SLAC E165 Fluorescence from Air in Showers FLASH
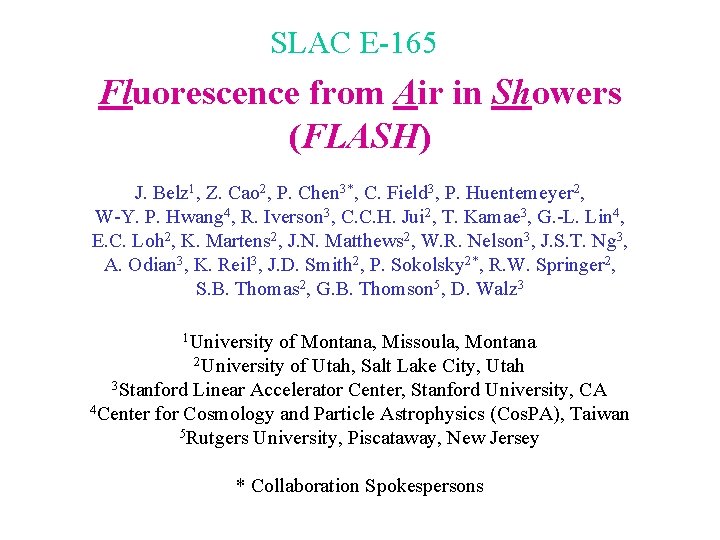
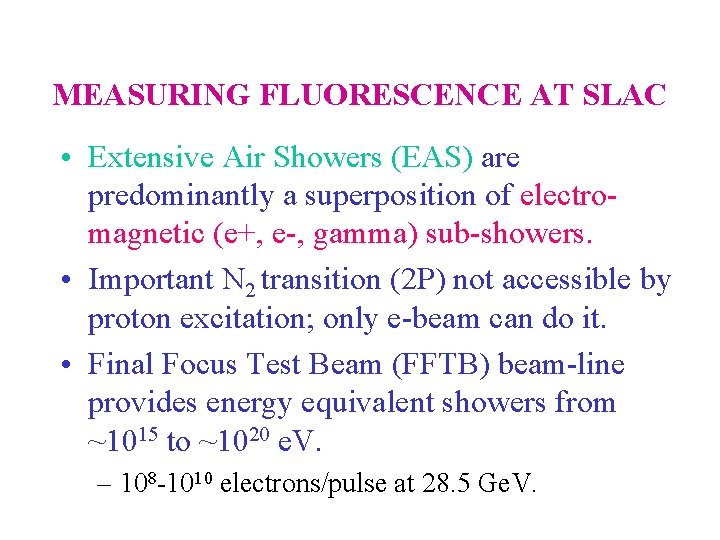
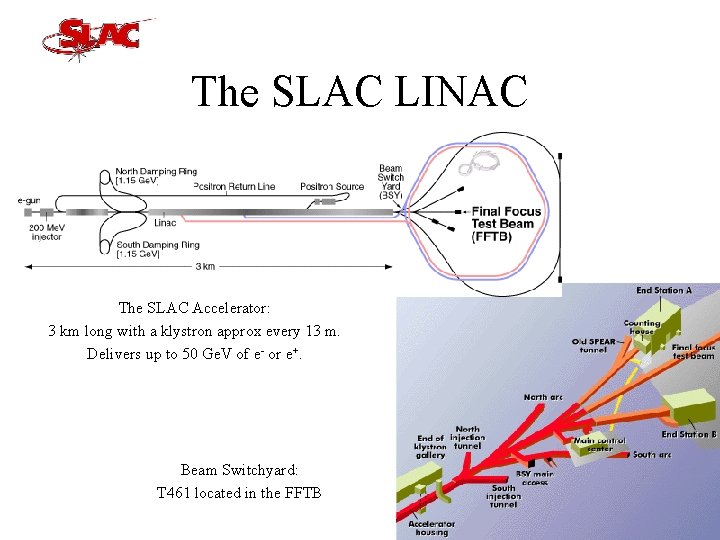
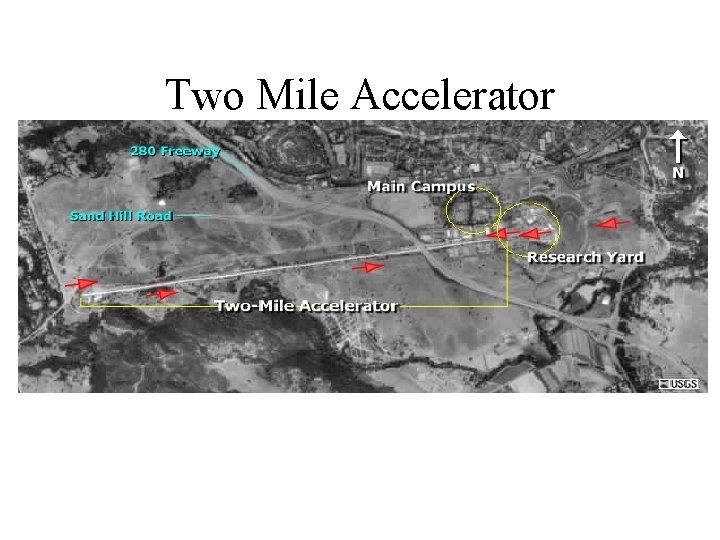
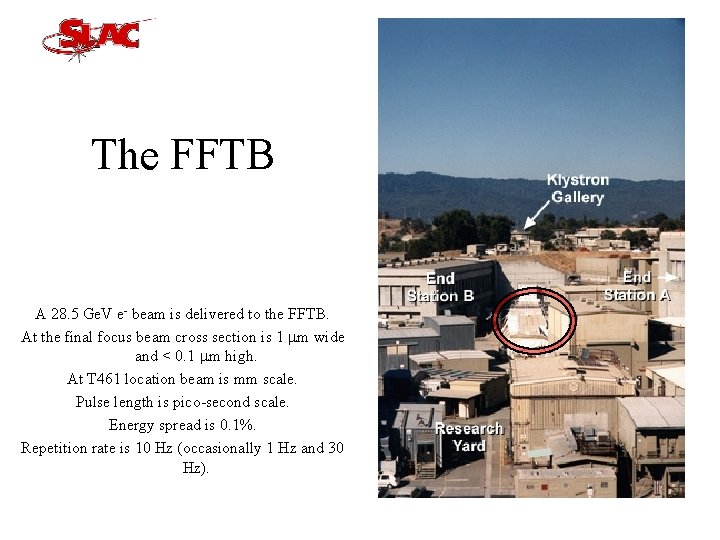
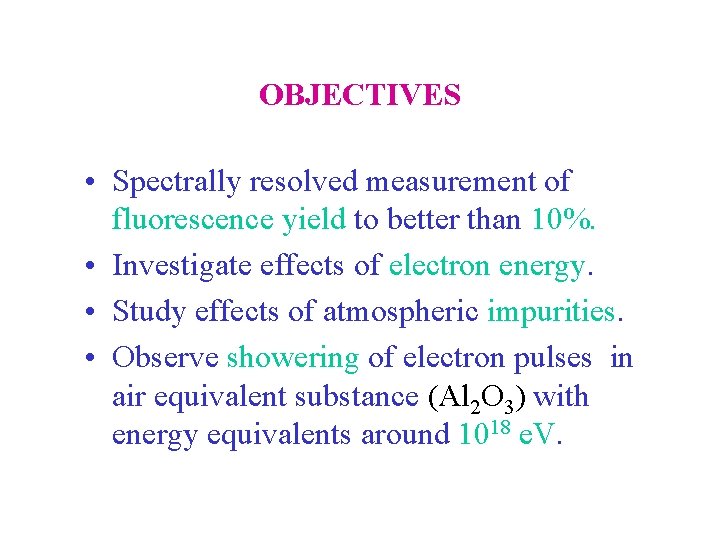
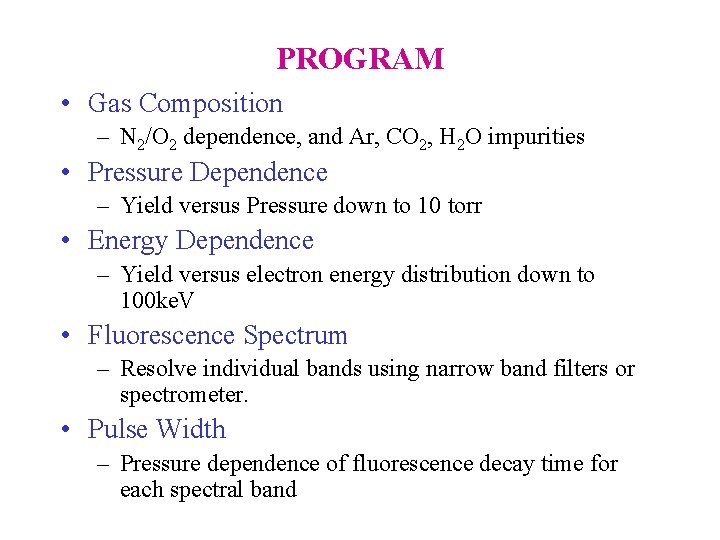
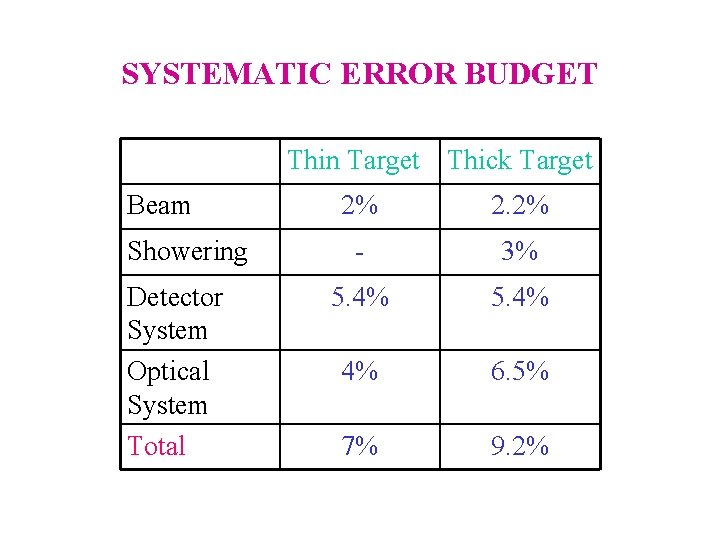
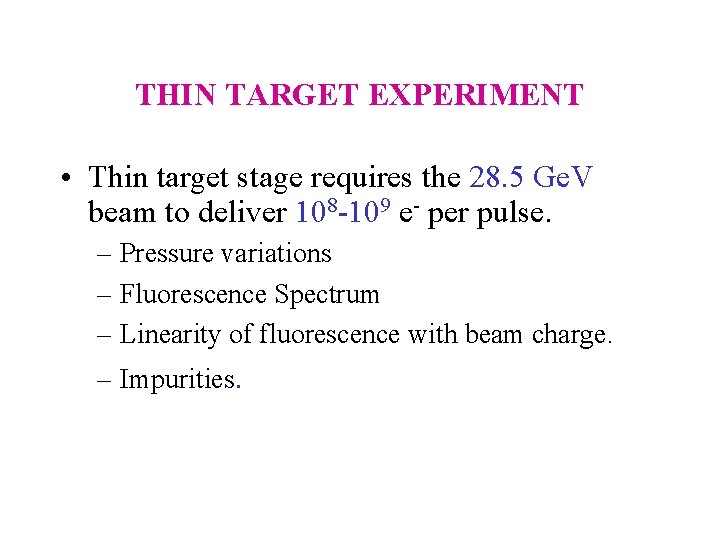
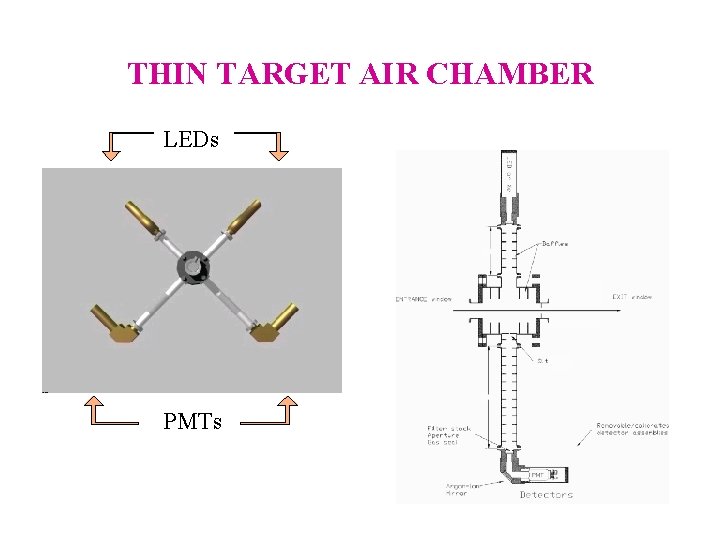
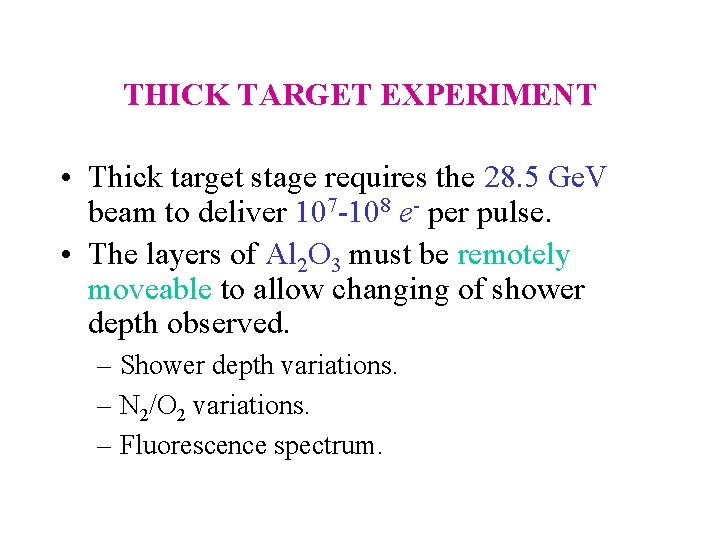
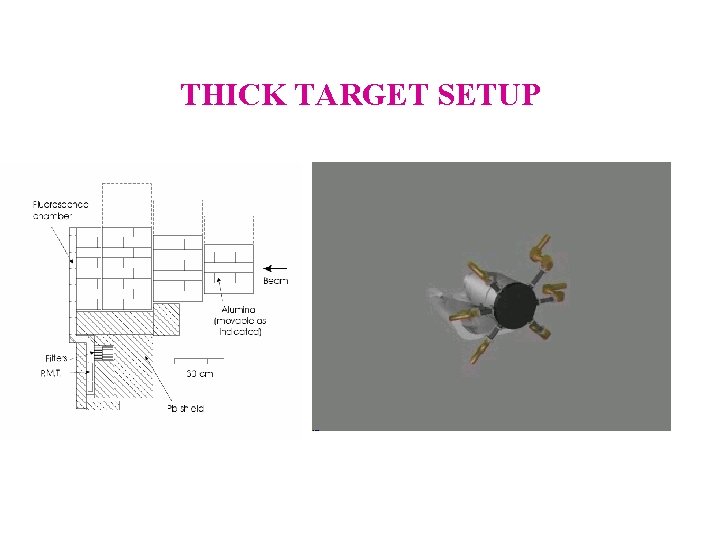
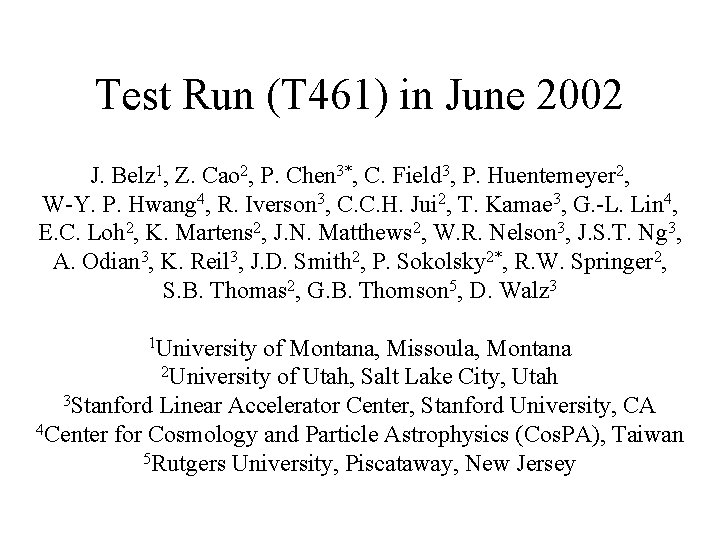
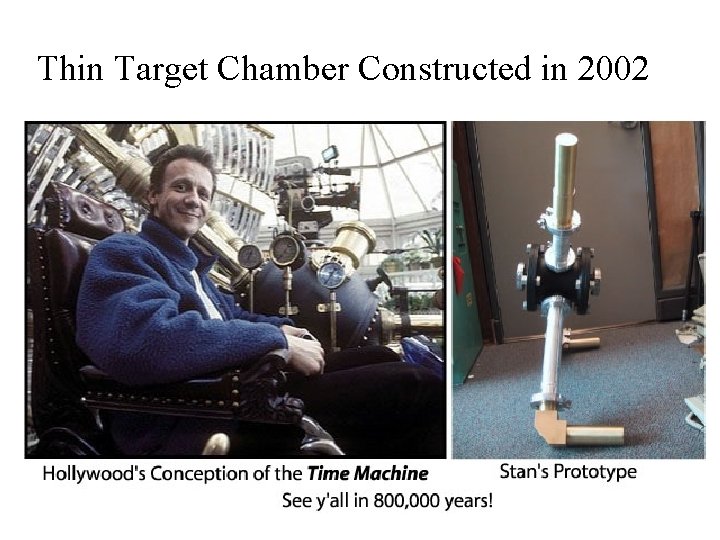
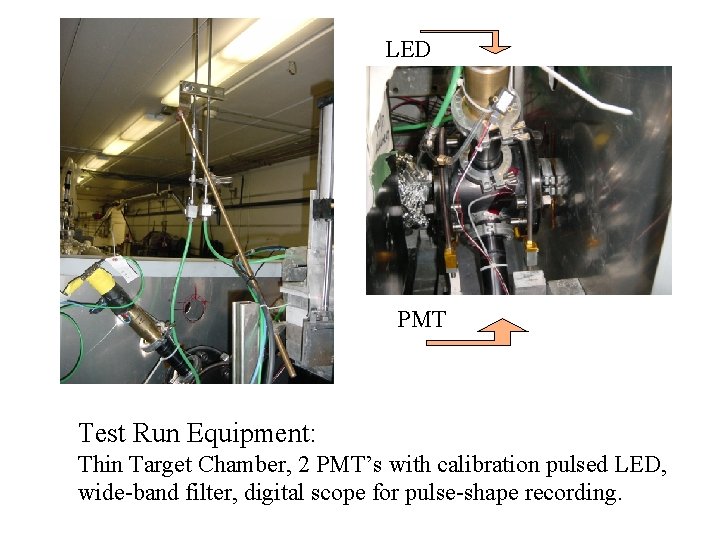
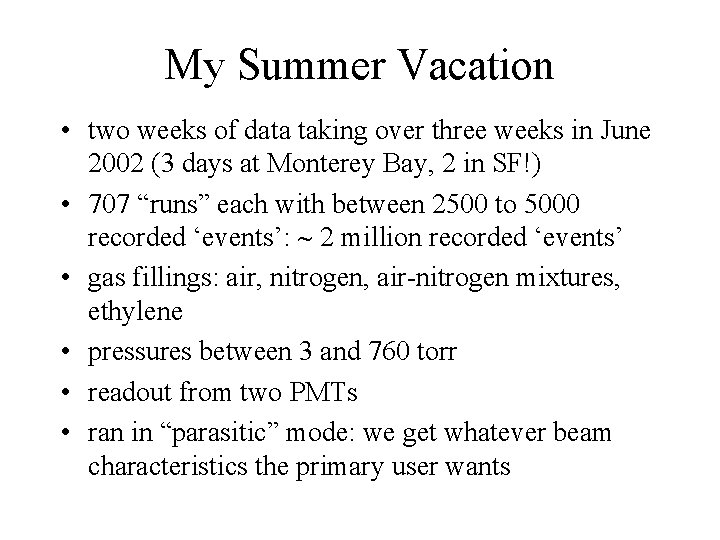
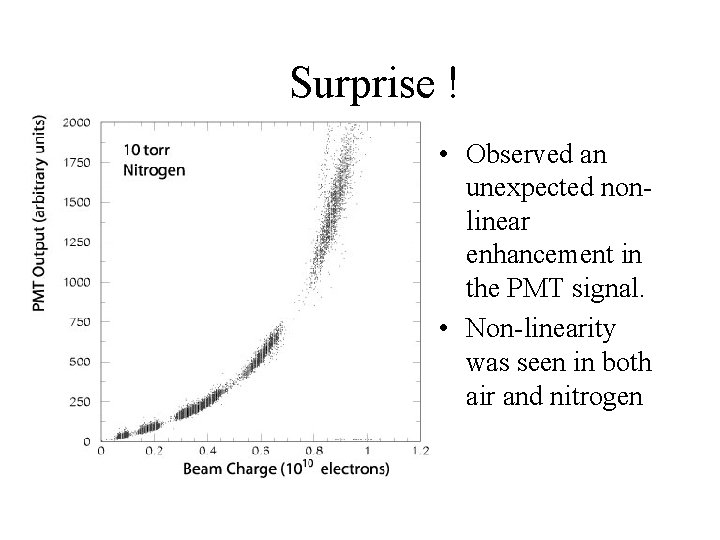
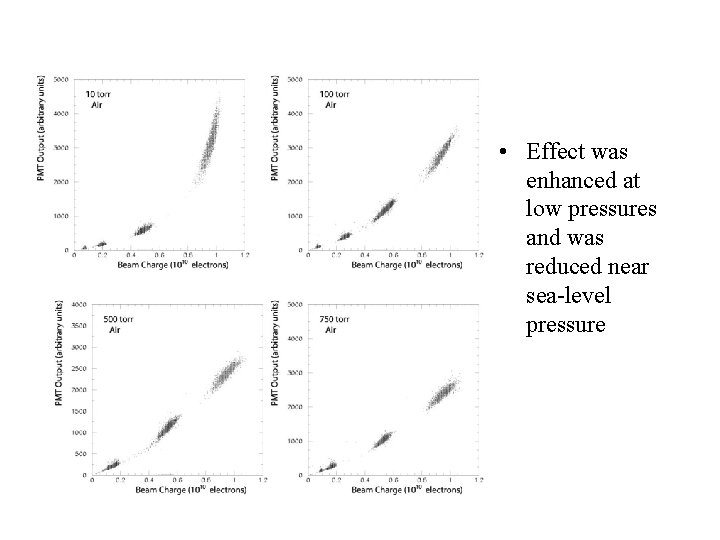
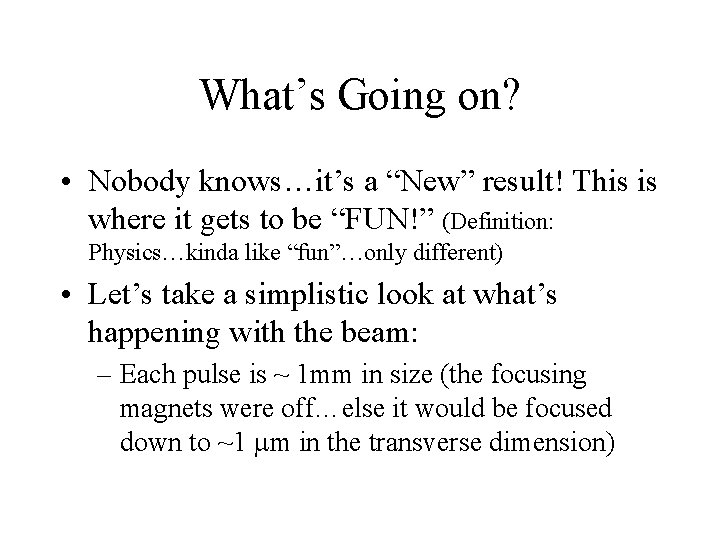
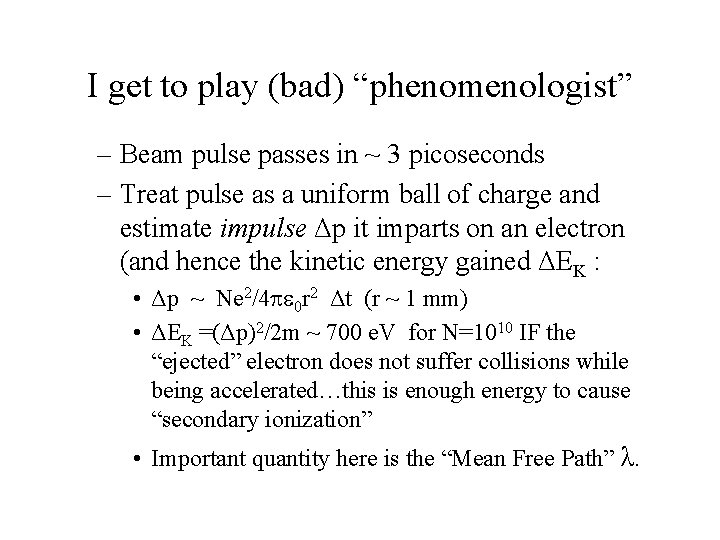
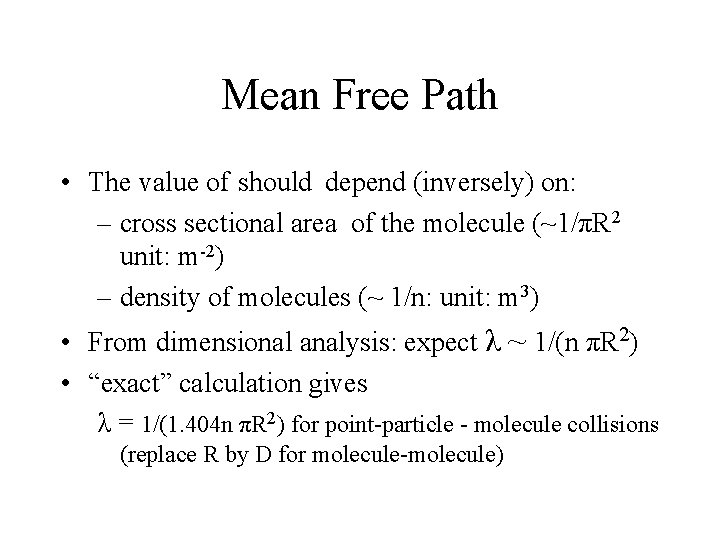
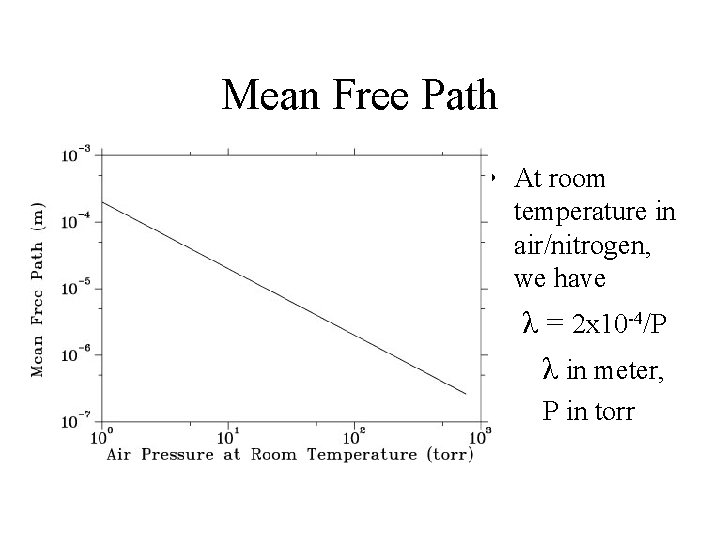
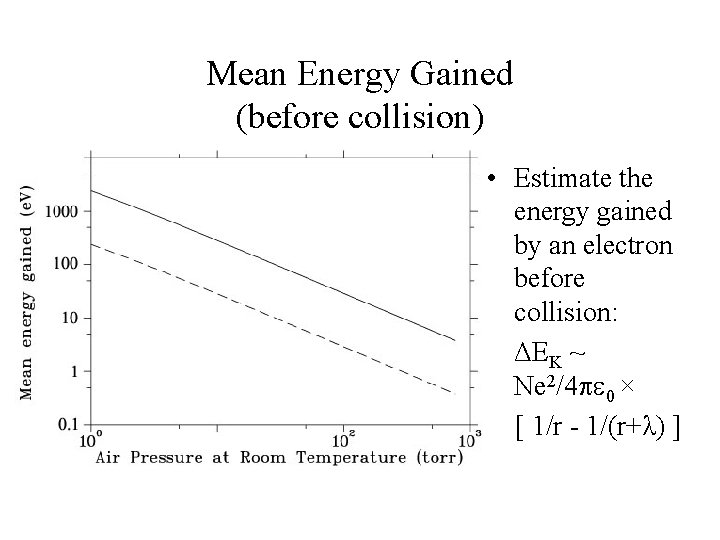
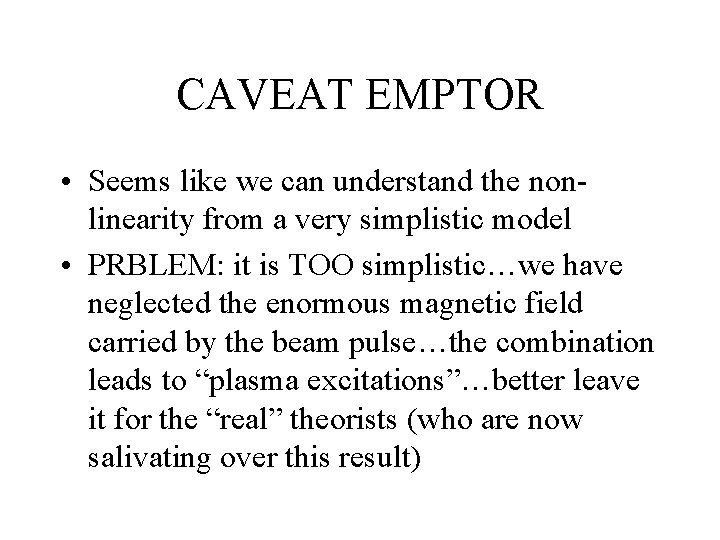
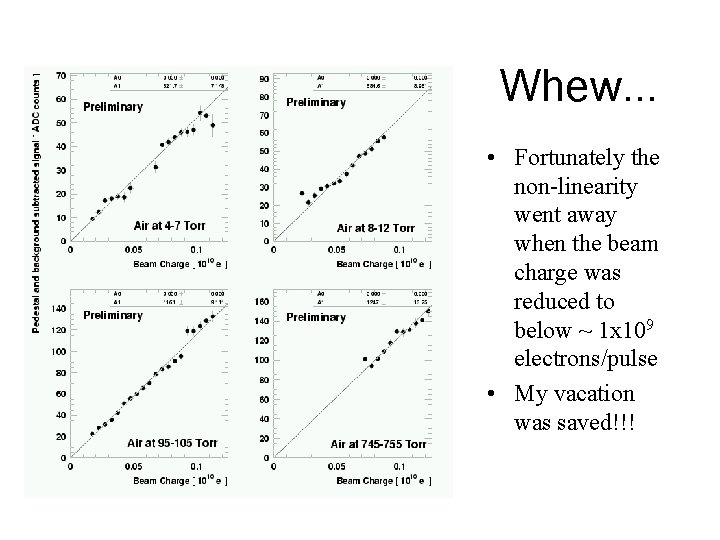
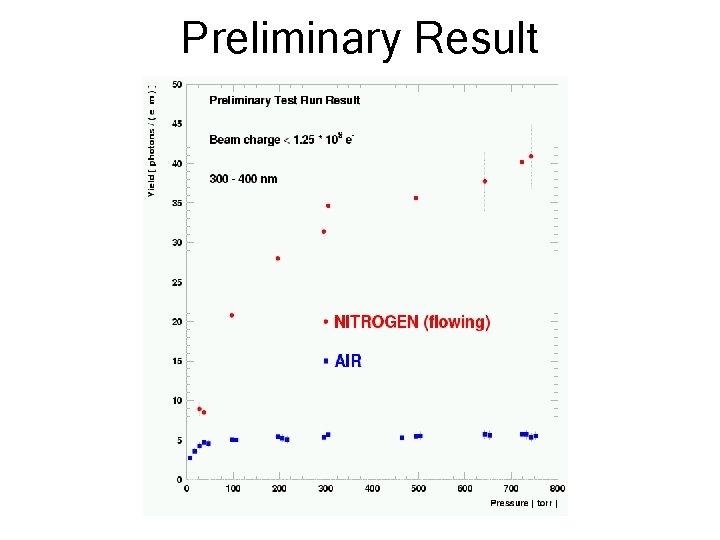
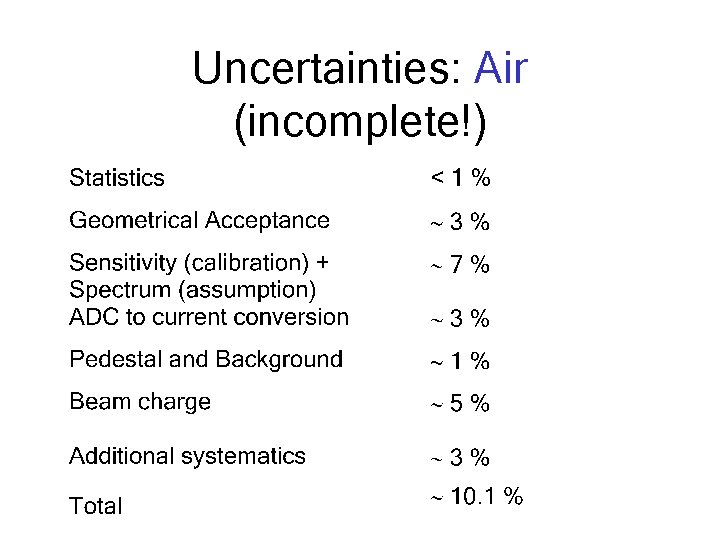
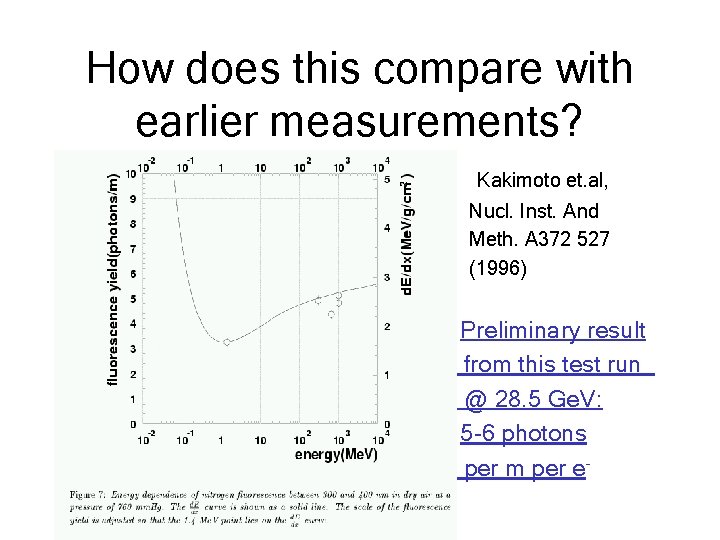
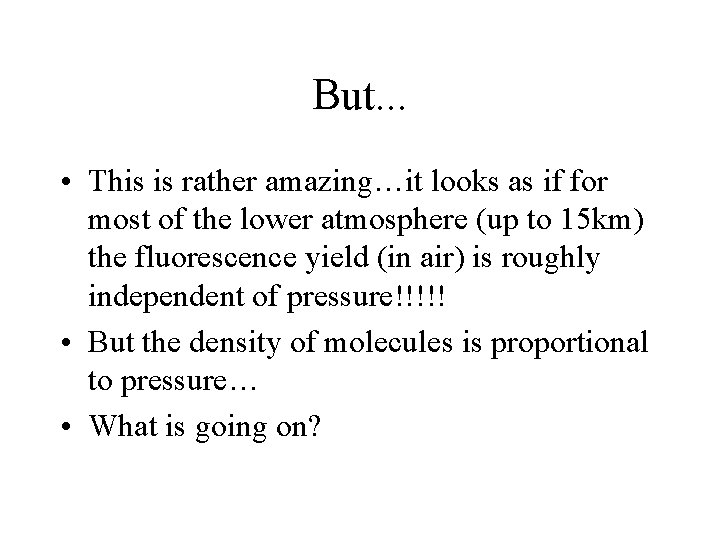
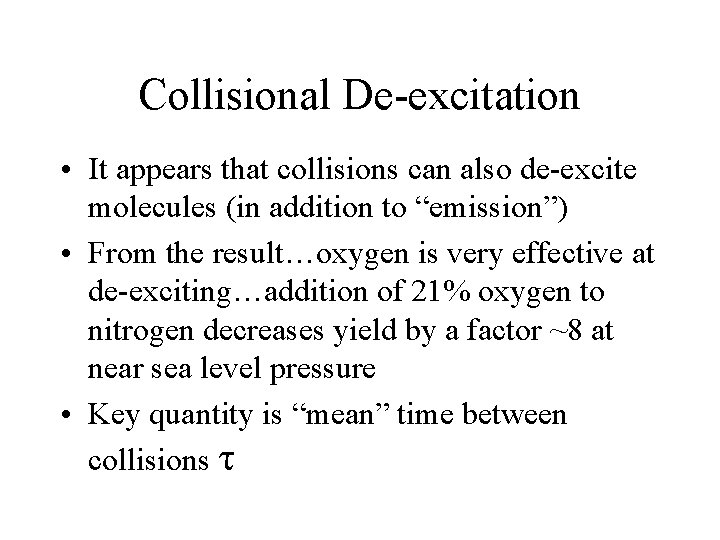
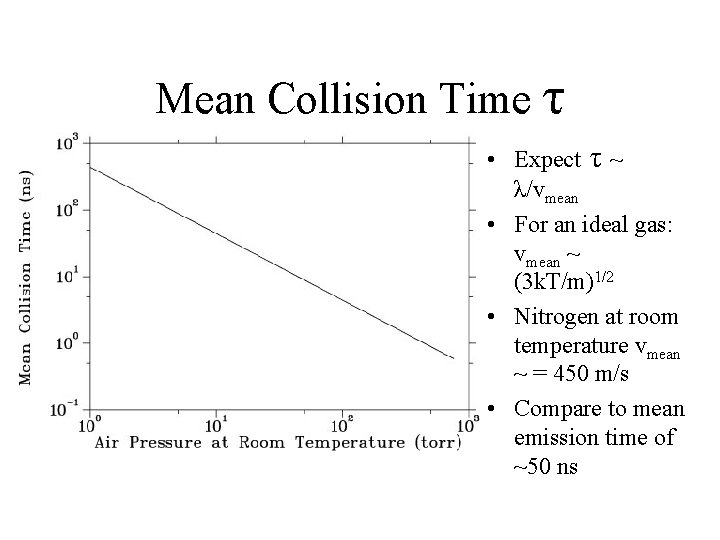
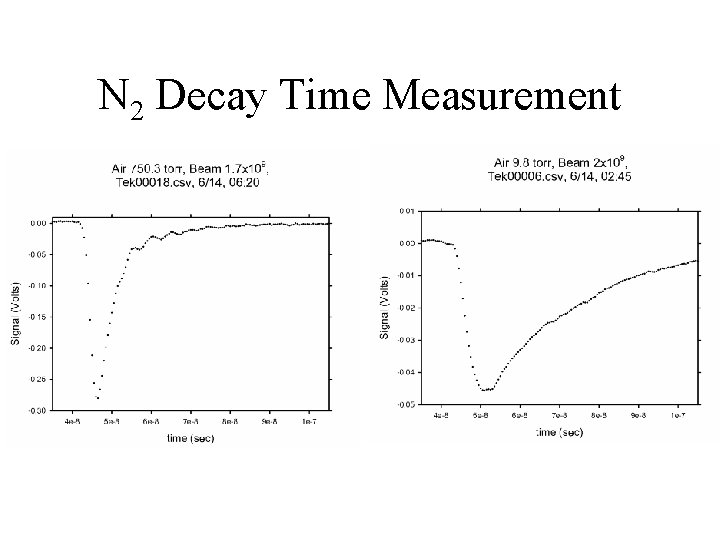
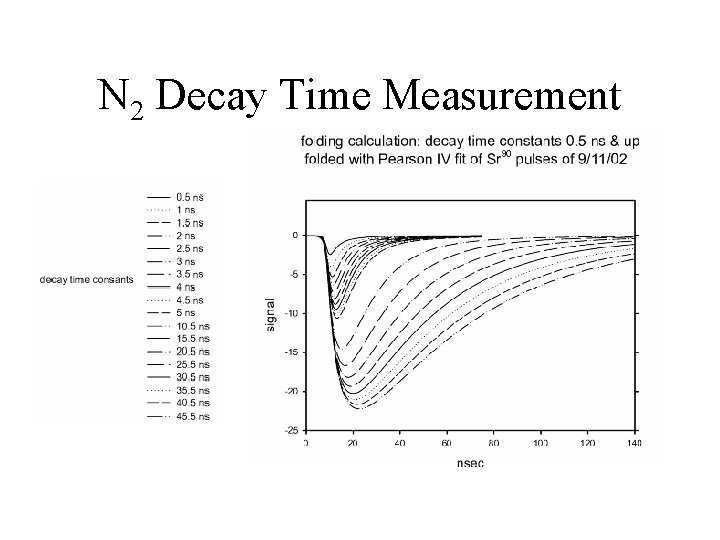
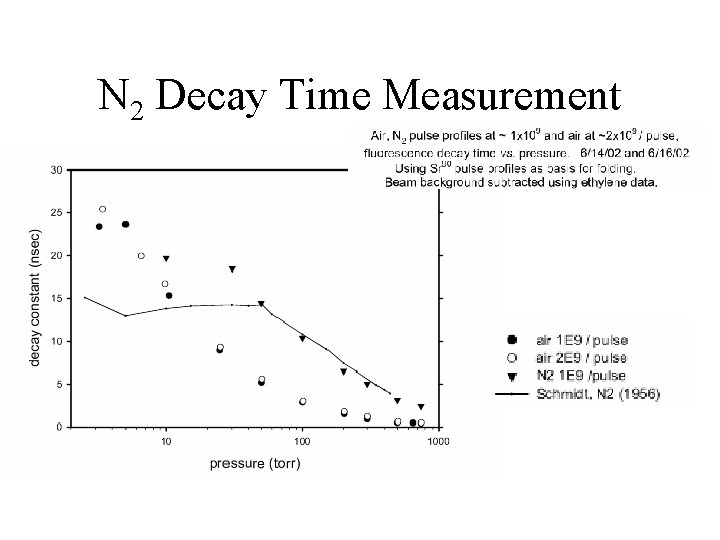
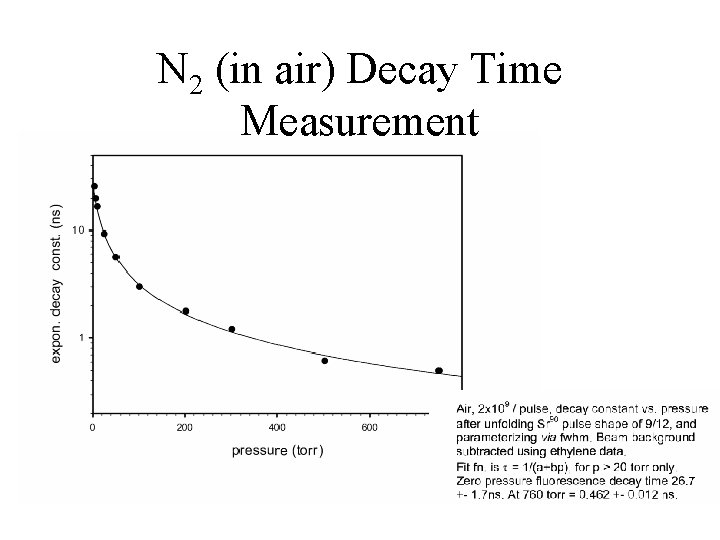
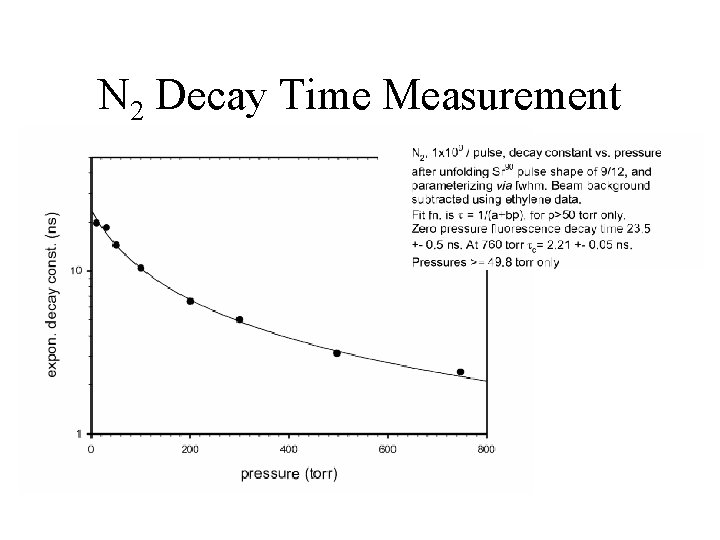
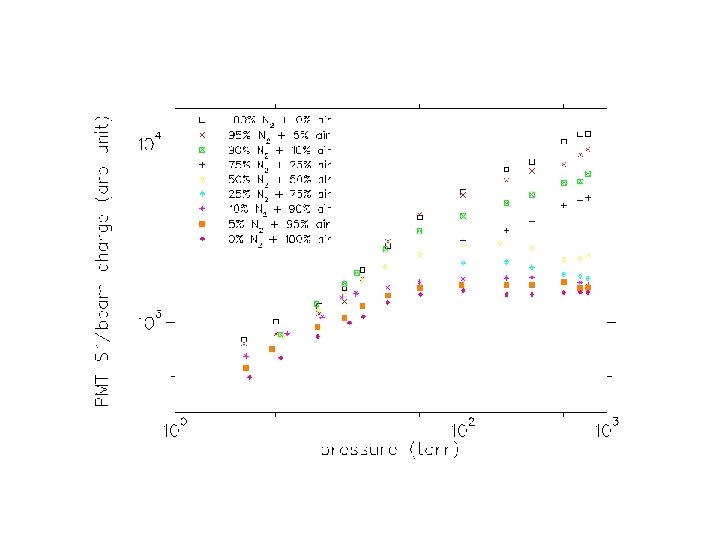
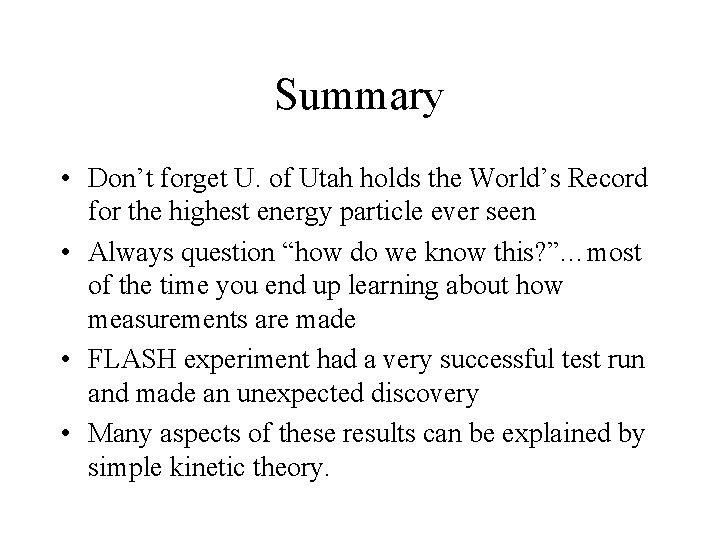
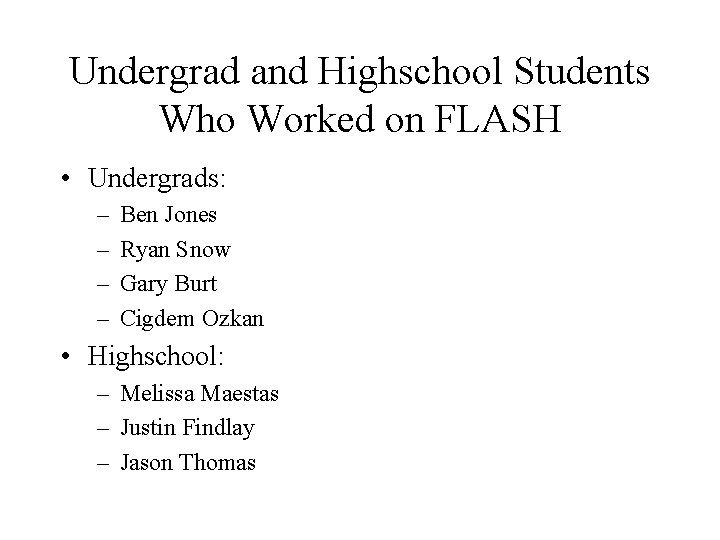
- Slides: 39
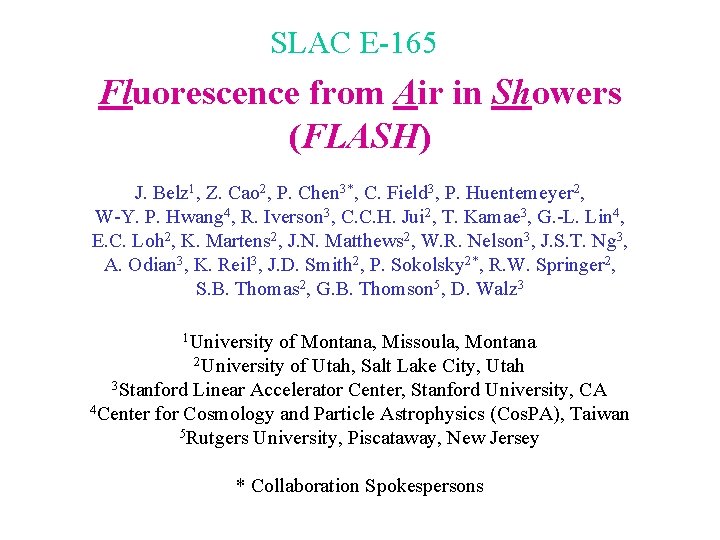
SLAC E-165 Fluorescence from Air in Showers (FLASH) J. Belz 1, Z. Cao 2, P. Chen 3*, C. Field 3, P. Huentemeyer 2, W-Y. P. Hwang 4, R. Iverson 3, C. C. H. Jui 2, T. Kamae 3, G. -L. Lin 4, E. C. Loh 2, K. Martens 2, J. N. Matthews 2, W. R. Nelson 3, J. S. T. Ng 3, A. Odian 3, K. Reil 3, J. D. Smith 2, P. Sokolsky 2*, R. W. Springer 2, S. B. Thomas 2, G. B. Thomson 5, D. Walz 3 1 University of Montana, Missoula, Montana 2 University of Utah, Salt Lake City, Utah 3 Stanford Linear Accelerator Center, Stanford University, CA 4 Center for Cosmology and Particle Astrophysics (Cos. PA), Taiwan 5 Rutgers University, Piscataway, New Jersey * Collaboration Spokespersons
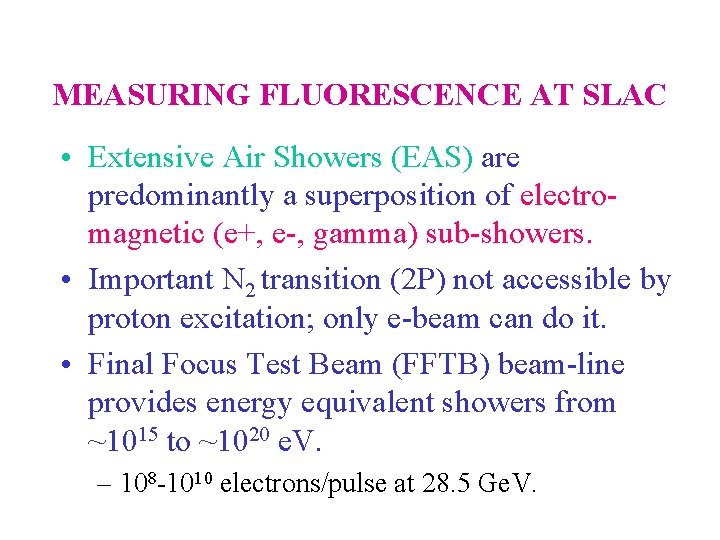
MEASURING FLUORESCENCE AT SLAC • Extensive Air Showers (EAS) are predominantly a superposition of electromagnetic (e+, e-, gamma) sub-showers. • Important N 2 transition (2 P) not accessible by proton excitation; only e-beam can do it. • Final Focus Test Beam (FFTB) beam-line provides energy equivalent showers from ~1015 to ~1020 e. V. – 108 -1010 electrons/pulse at 28. 5 Ge. V.
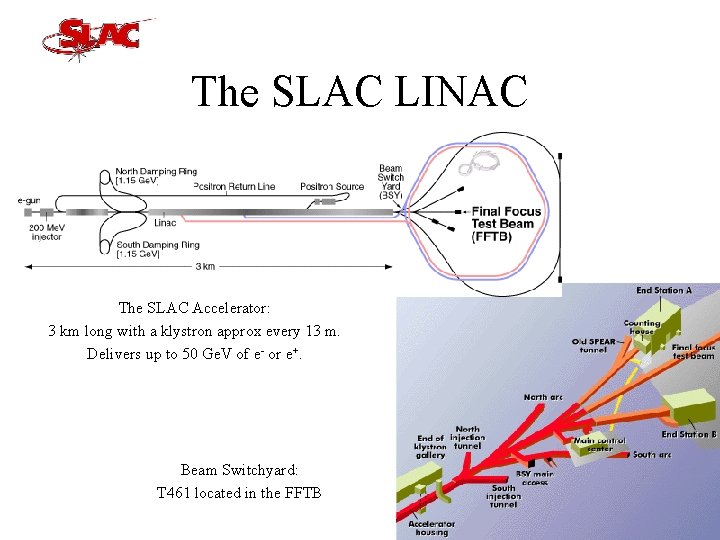
The SLAC LINAC The SLAC Accelerator: 3 km long with a klystron approx every 13 m. Delivers up to 50 Ge. V of e- or e+. Beam Switchyard: T 461 located in the FFTB
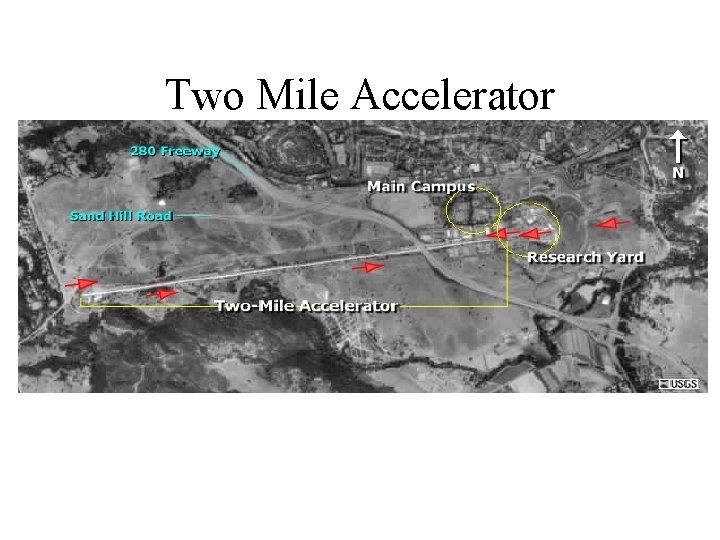
Two Mile Accelerator
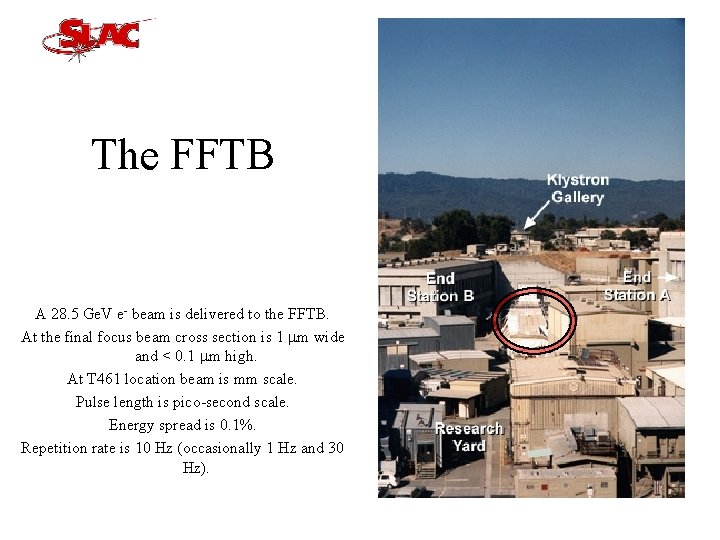
The FFTB A 28. 5 Ge. V e- beam is delivered to the FFTB. At the final focus beam cross section is 1 m wide and < 0. 1 m high. At T 461 location beam is mm scale. Pulse length is pico-second scale. Energy spread is 0. 1%. Repetition rate is 10 Hz (occasionally 1 Hz and 30 Hz).
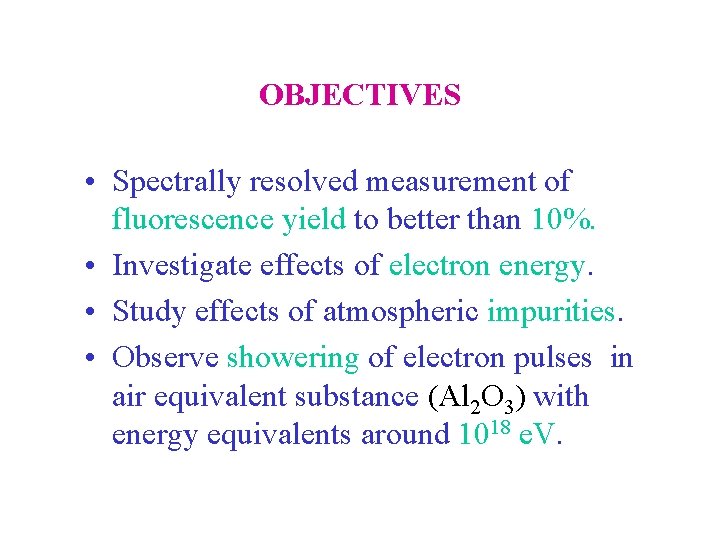
OBJECTIVES • Spectrally resolved measurement of fluorescence yield to better than 10%. • Investigate effects of electron energy. • Study effects of atmospheric impurities. • Observe showering of electron pulses in air equivalent substance (Al 2 O 3) with energy equivalents around 1018 e. V.
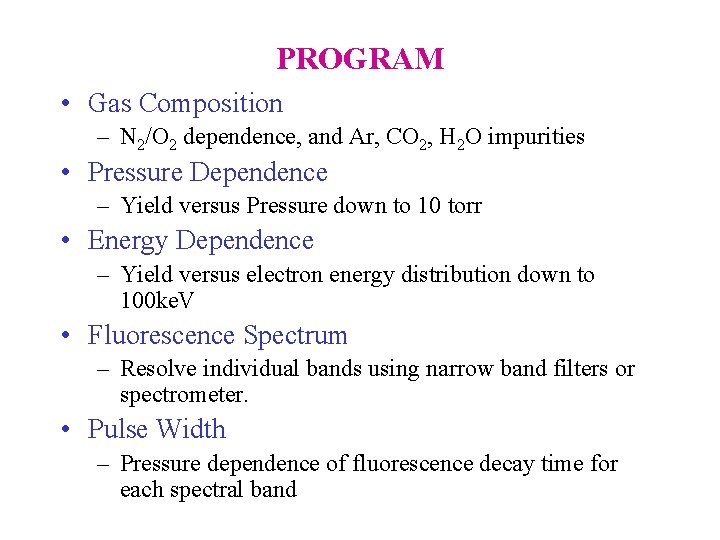
PROGRAM • Gas Composition – N 2/O 2 dependence, and Ar, CO 2, H 2 O impurities • Pressure Dependence – Yield versus Pressure down to 10 torr • Energy Dependence – Yield versus electron energy distribution down to 100 ke. V • Fluorescence Spectrum – Resolve individual bands using narrow band filters or spectrometer. • Pulse Width – Pressure dependence of fluorescence decay time for each spectral band
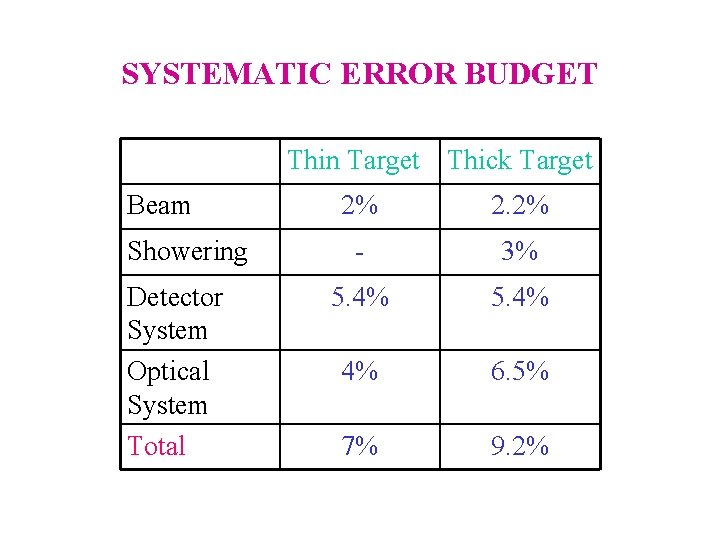
SYSTEMATIC ERROR BUDGET Thin Target Thick Target Beam Showering Detector System Optical System Total 2% 2. 2% - 3% 5. 4% 4% 6. 5% 7% 9. 2%
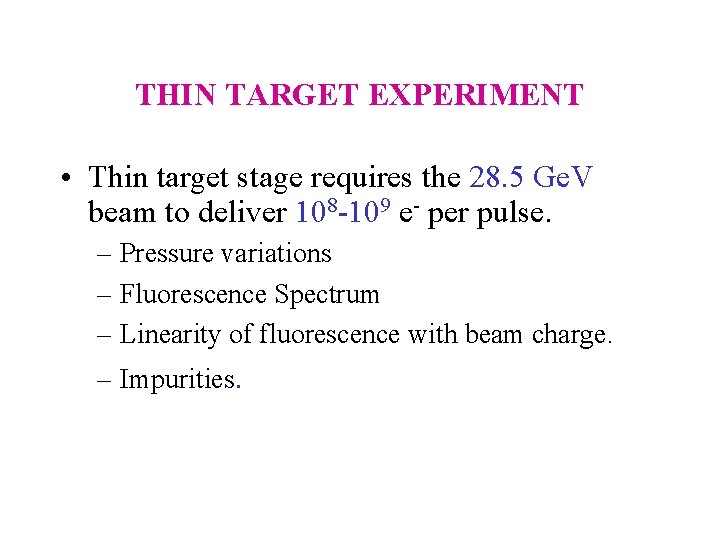
THIN TARGET EXPERIMENT • Thin target stage requires the 28. 5 Ge. V beam to deliver 108 -109 e- per pulse. – Pressure variations – Fluorescence Spectrum – Linearity of fluorescence with beam charge. – Impurities.
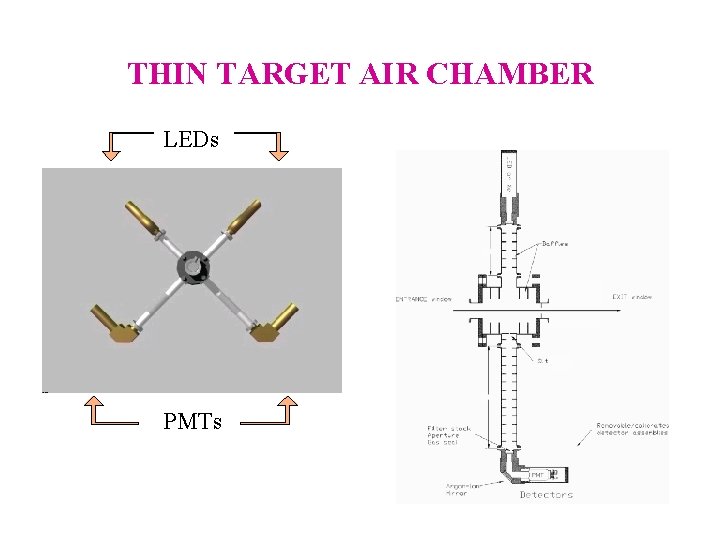
THIN TARGET AIR CHAMBER LEDs PMTs
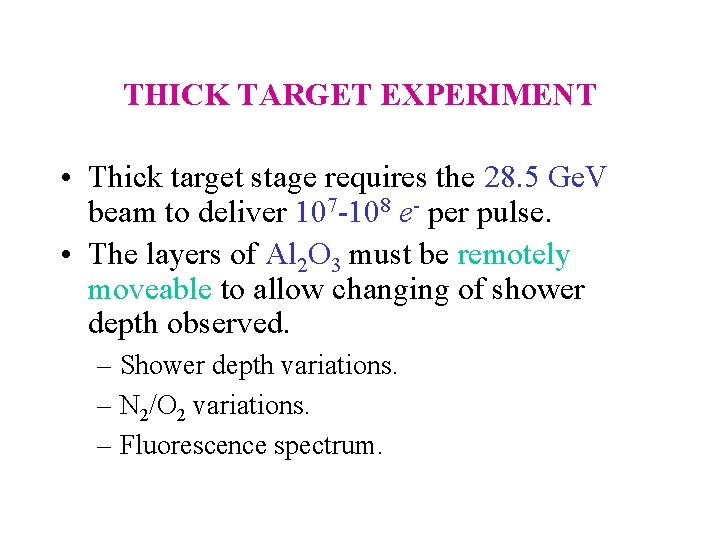
THICK TARGET EXPERIMENT • Thick target stage requires the 28. 5 Ge. V beam to deliver 107 -108 e- per pulse. • The layers of Al 2 O 3 must be remotely moveable to allow changing of shower depth observed. – Shower depth variations. – N 2/O 2 variations. – Fluorescence spectrum.
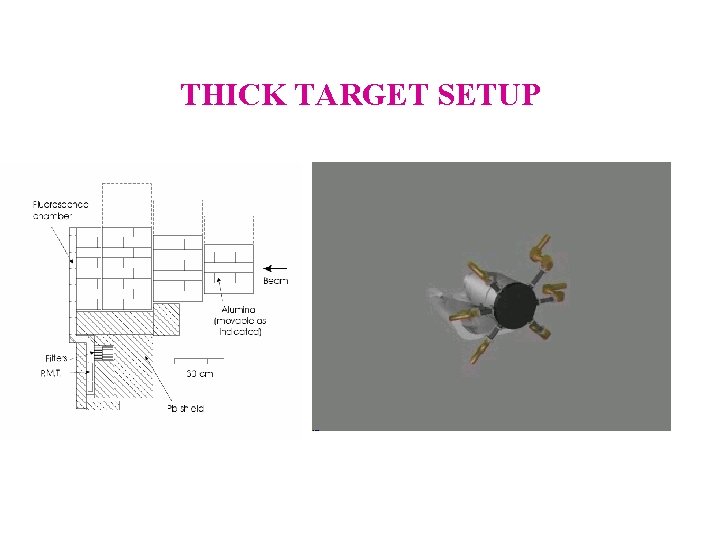
THICK TARGET SETUP
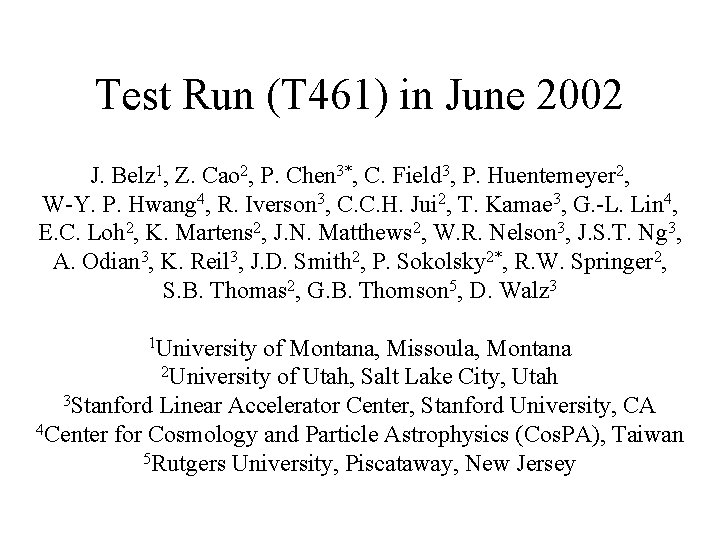
Test Run (T 461) in June 2002 J. Belz 1, Z. Cao 2, P. Chen 3*, C. Field 3, P. Huentemeyer 2, W-Y. P. Hwang 4, R. Iverson 3, C. C. H. Jui 2, T. Kamae 3, G. -L. Lin 4, E. C. Loh 2, K. Martens 2, J. N. Matthews 2, W. R. Nelson 3, J. S. T. Ng 3, A. Odian 3, K. Reil 3, J. D. Smith 2, P. Sokolsky 2*, R. W. Springer 2, S. B. Thomas 2, G. B. Thomson 5, D. Walz 3 1 University of Montana, Missoula, Montana 2 University of Utah, Salt Lake City, Utah 3 Stanford Linear Accelerator Center, Stanford University, CA 4 Center for Cosmology and Particle Astrophysics (Cos. PA), Taiwan 5 Rutgers University, Piscataway, New Jersey
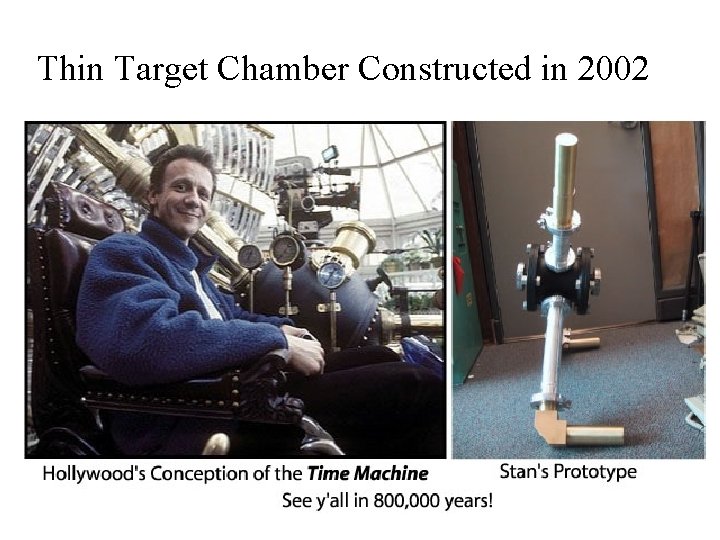
Thin Target Chamber Constructed in 2002
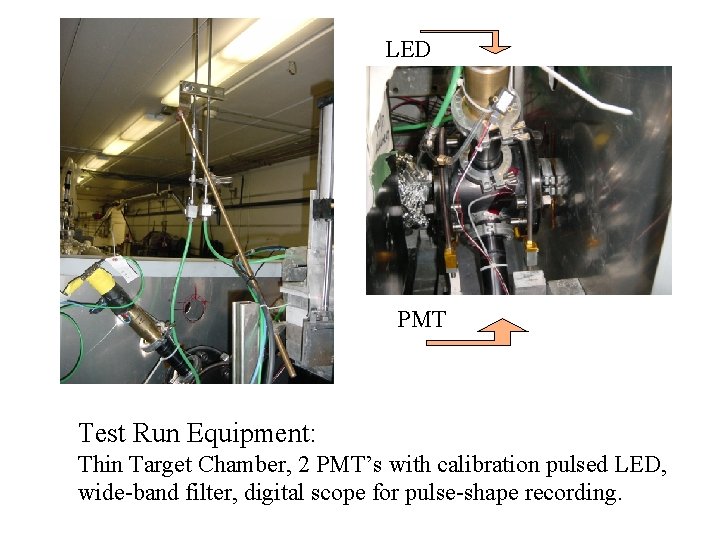
LED PMT Test Run Equipment: Thin Target Chamber, 2 PMT’s with calibration pulsed LED, wide-band filter, digital scope for pulse-shape recording.
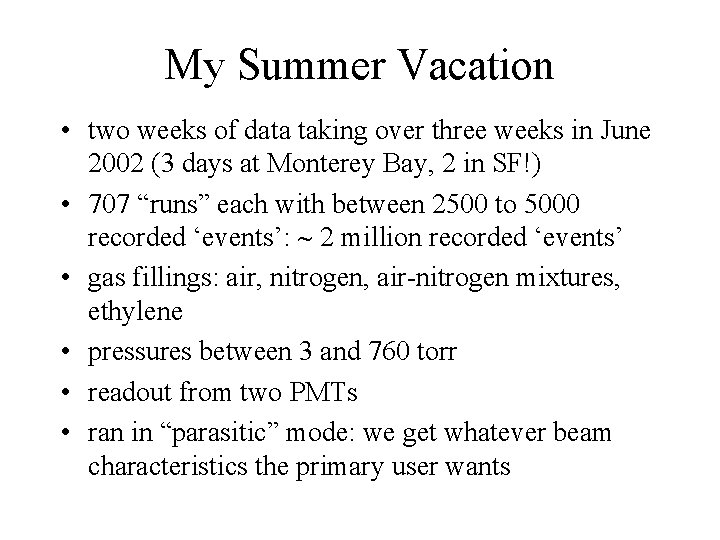
My Summer Vacation • two weeks of data taking over three weeks in June 2002 (3 days at Monterey Bay, 2 in SF!) • 707 “runs” each with between 2500 to 5000 recorded ‘events’: 2 million recorded ‘events’ • gas fillings: air, nitrogen, air-nitrogen mixtures, ethylene • pressures between 3 and 760 torr • readout from two PMTs • ran in “parasitic” mode: we get whatever beam characteristics the primary user wants
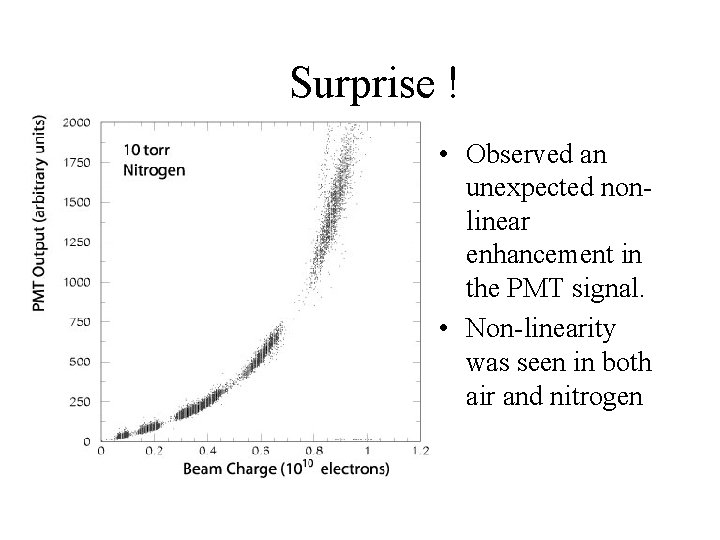
Surprise ! • Observed an unexpected nonlinear enhancement in the PMT signal. • Non-linearity was seen in both air and nitrogen
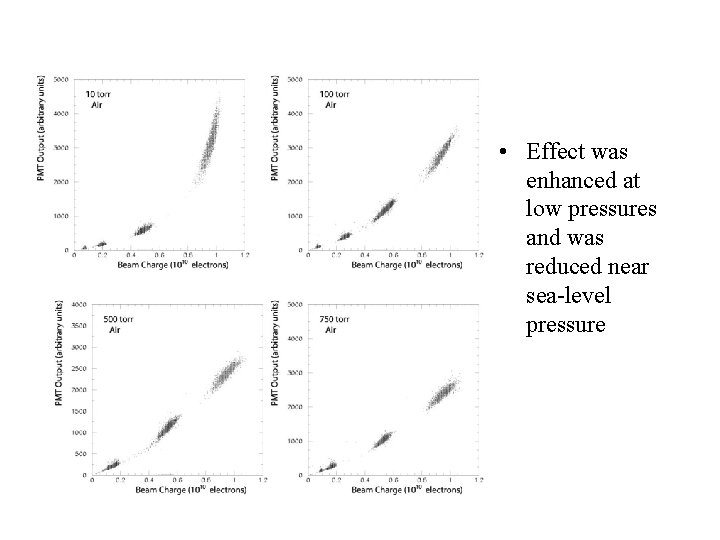
• Effect was enhanced at low pressures and was reduced near sea-level pressure
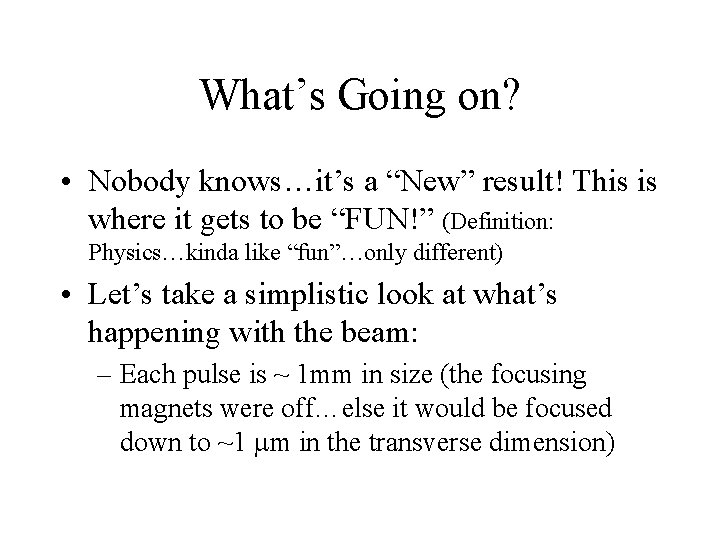
What’s Going on? • Nobody knows…it’s a “New” result! This is where it gets to be “FUN!” (Definition: Physics…kinda like “fun”…only different) • Let’s take a simplistic look at what’s happening with the beam: – Each pulse is ~ 1 mm in size (the focusing magnets were off…else it would be focused down to ~1 m in the transverse dimension)
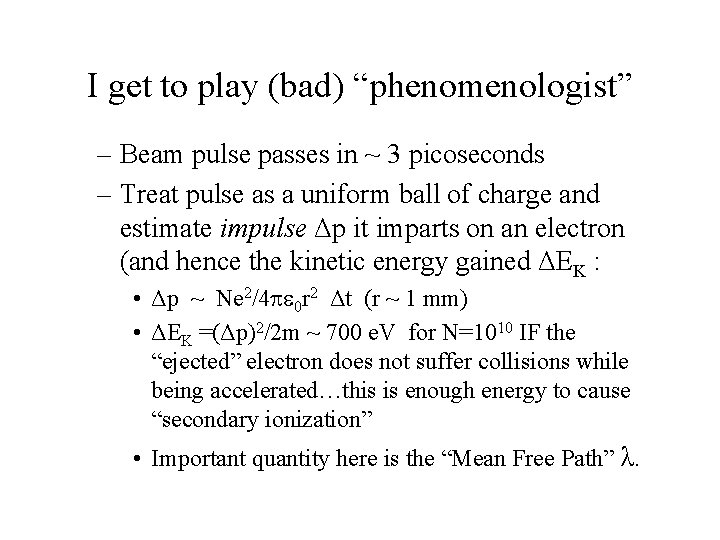
I get to play (bad) “phenomenologist” – Beam pulse passes in ~ 3 picoseconds – Treat pulse as a uniform ball of charge and estimate impulse Δp it imparts on an electron (and hence the kinetic energy gained ΔEK : • Δp ~ Ne 2/4 pe 0 r 2 Δt (r ~ 1 mm) • ΔEK =(Δp)2/2 m ~ 700 e. V for N=1010 IF the “ejected” electron does not suffer collisions while being accelerated…this is enough energy to cause “secondary ionization” • Important quantity here is the “Mean Free Path” λ.
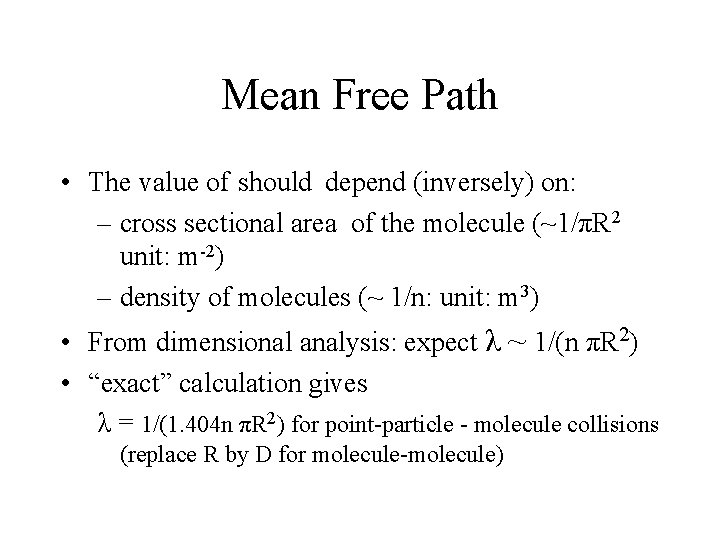
Mean Free Path • The value of should depend (inversely) on: – cross sectional area of the molecule (~1/πR 2 unit: m-2) – density of molecules (~ 1/n: unit: m 3) • From dimensional analysis: expect λ ~ 1/(n πR 2) • “exact” calculation gives λ = 1/(1. 404 n πR 2) for point-particle - molecule collisions (replace R by D for molecule-molecule)
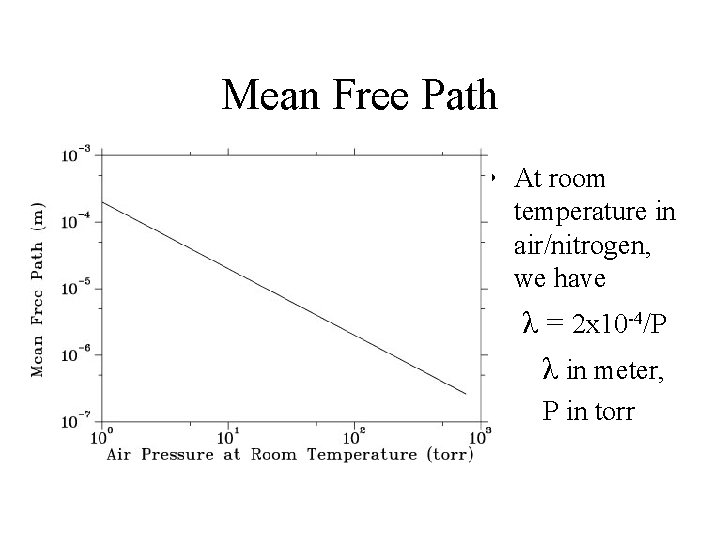
Mean Free Path • At room temperature in air/nitrogen, we have λ = 2 x 10 -4/P λ in meter, P in torr
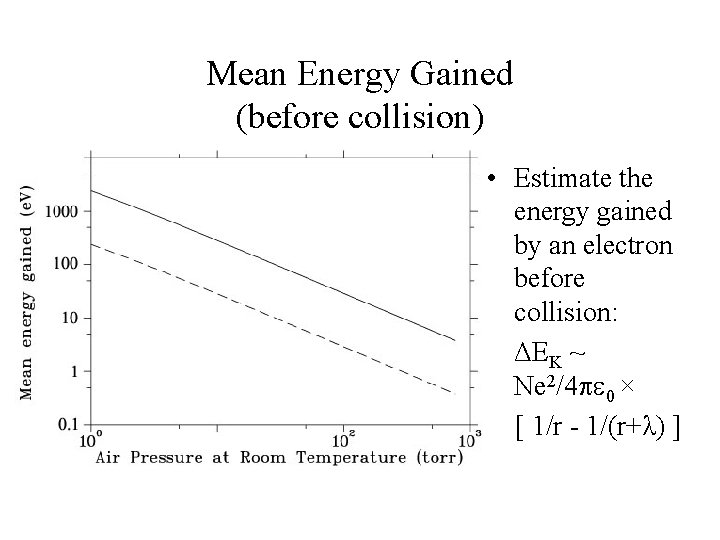
Mean Energy Gained (before collision) • Estimate the energy gained by an electron before collision: ΔEK ~ Ne 2/4 pe 0 × [ 1/r - 1/(r+λ) ]
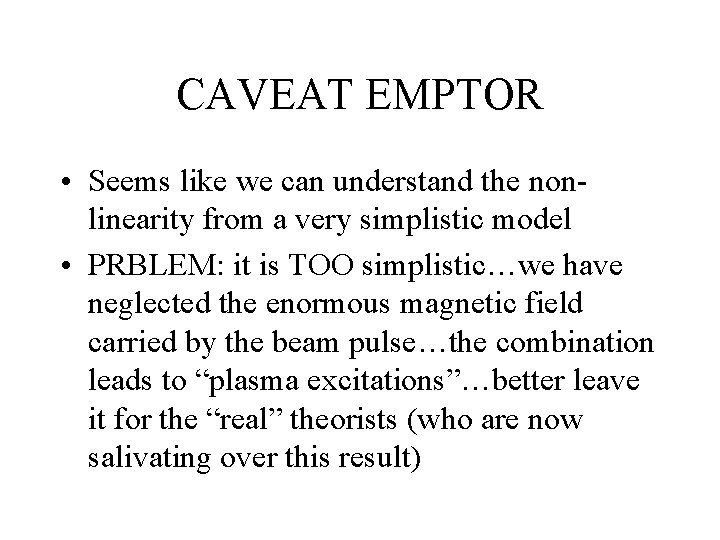
CAVEAT EMPTOR • Seems like we can understand the nonlinearity from a very simplistic model • PRBLEM: it is TOO simplistic…we have neglected the enormous magnetic field carried by the beam pulse…the combination leads to “plasma excitations”…better leave it for the “real” theorists (who are now salivating over this result)
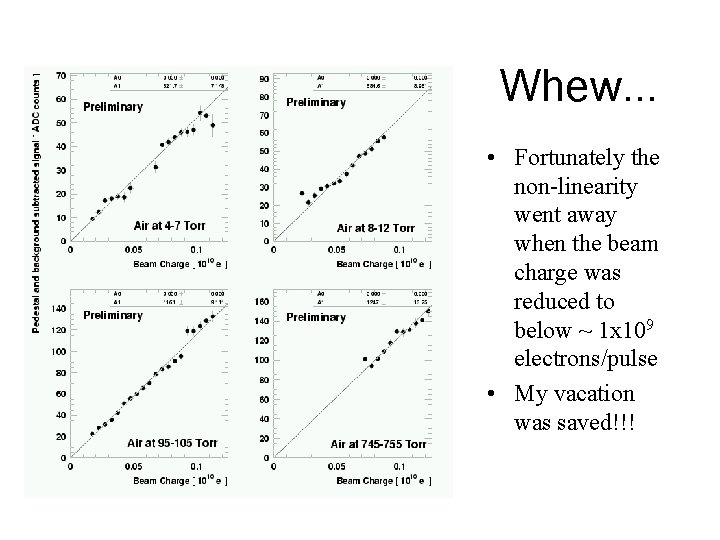
Whew. . . • Fortunately the non-linearity went away when the beam charge was reduced to below ~ 1 x 109 electrons/pulse • My vacation was saved!!!
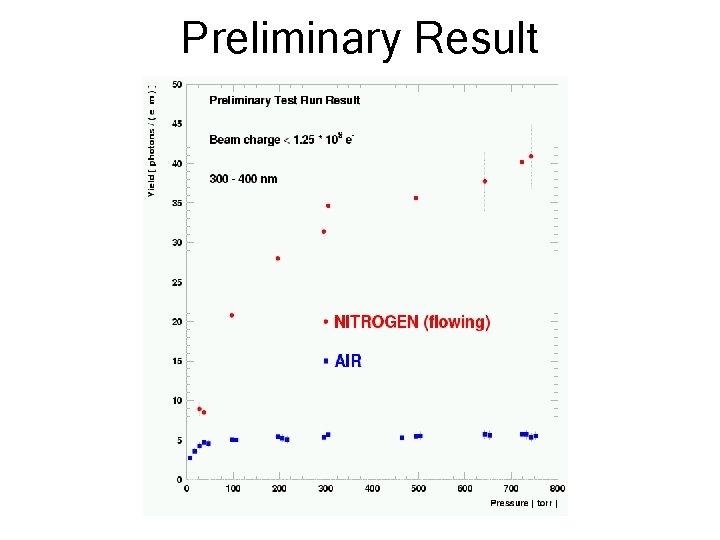
Preliminary Result
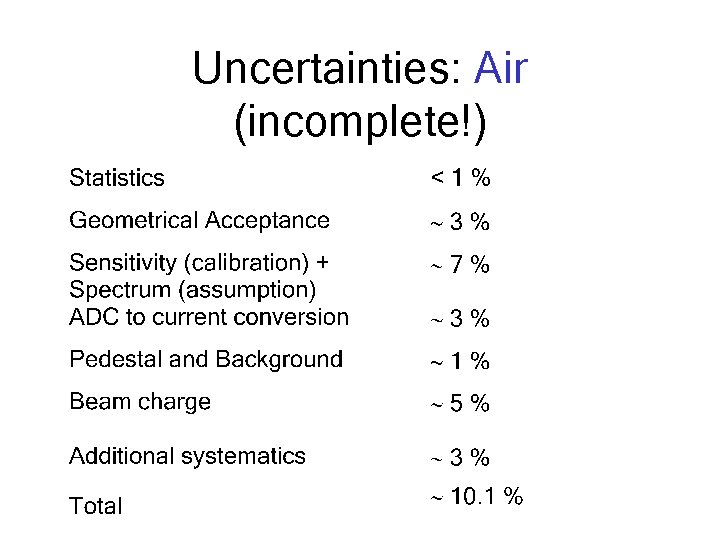
Uncertainties: Air (incomplete!)
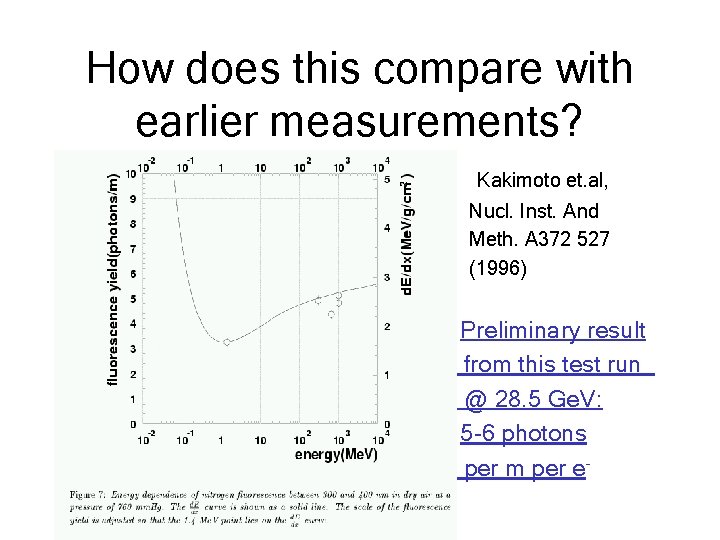
How does this compare with earlier measurements? Kakimoto et. al, Nucl. Inst. And Meth. A 372 527 (1996) Preliminary result from this test run @ 28. 5 Ge. V: 5 -6 photons per m per e-
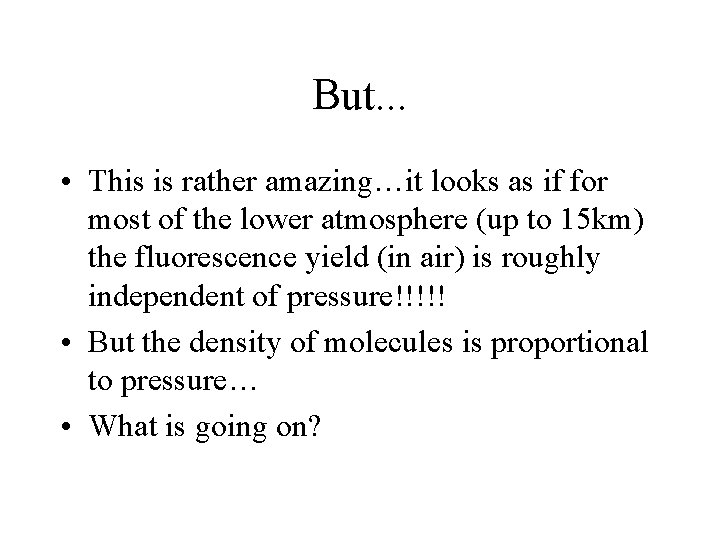
But. . . • This is rather amazing…it looks as if for most of the lower atmosphere (up to 15 km) the fluorescence yield (in air) is roughly independent of pressure!!!!! • But the density of molecules is proportional to pressure… • What is going on?
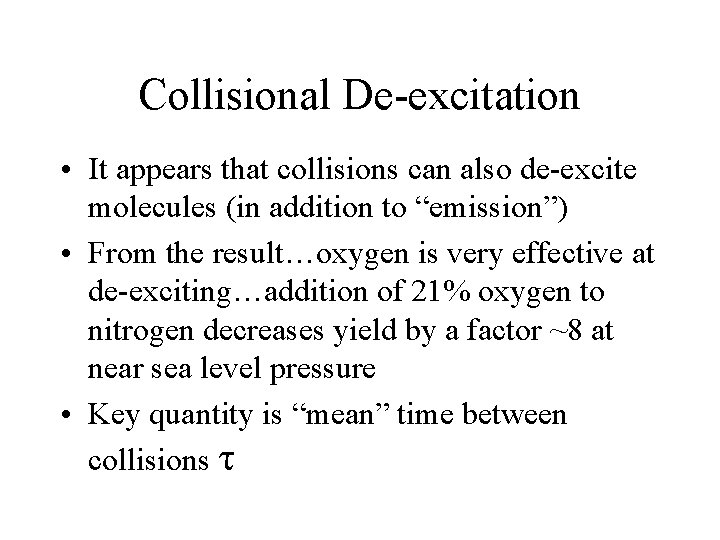
Collisional De-excitation • It appears that collisions can also de-excite molecules (in addition to “emission”) • From the result…oxygen is very effective at de-exciting…addition of 21% oxygen to nitrogen decreases yield by a factor ~8 at near sea level pressure • Key quantity is “mean” time between collisions τ
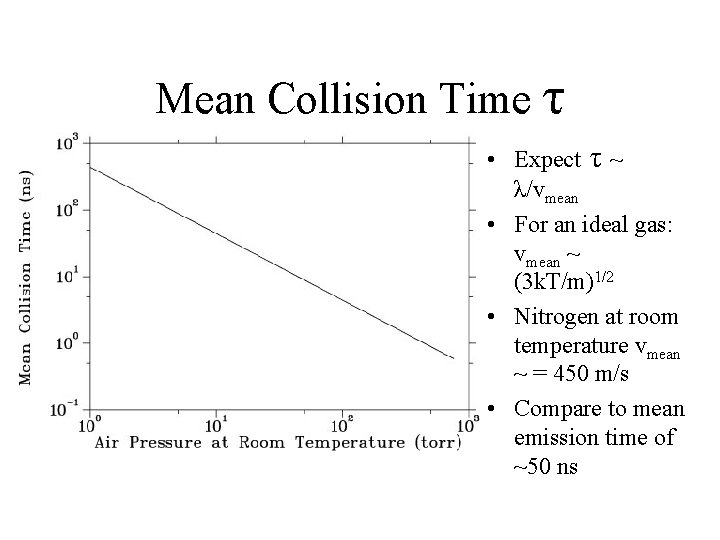
Mean Collision Time τ • Expect τ ~ λ/vmean • For an ideal gas: vmean ~ (3 k. T/m)1/2 • Nitrogen at room temperature vmean ~ = 450 m/s • Compare to mean emission time of ~50 ns
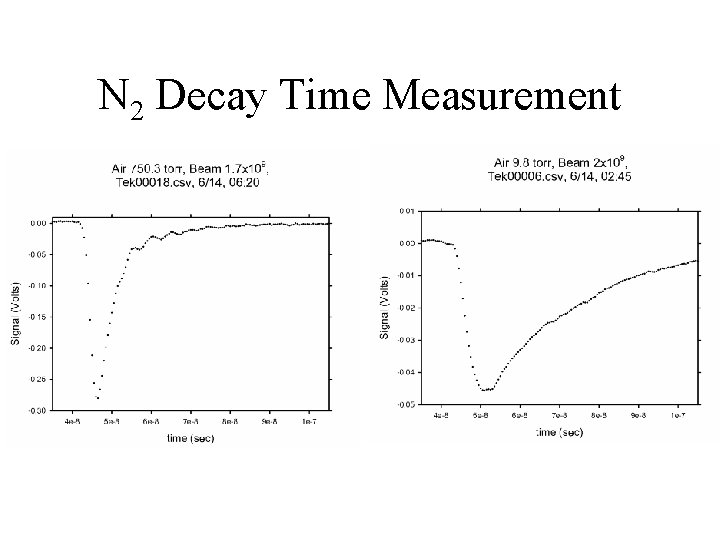
N 2 Decay Time Measurement
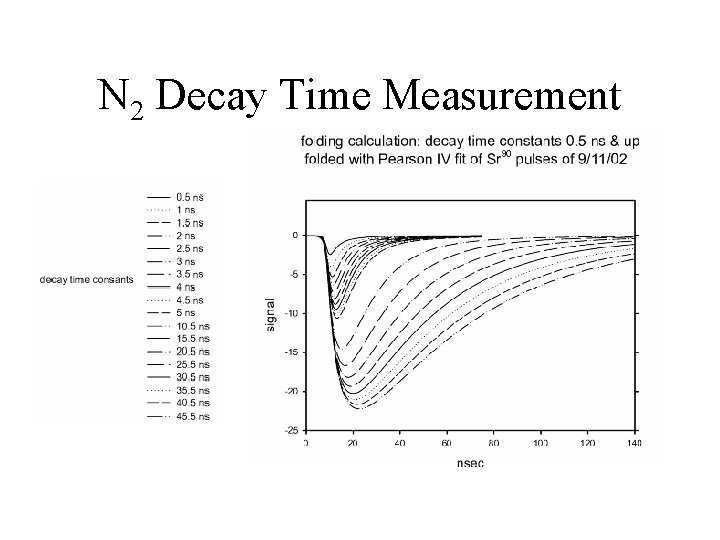
N 2 Decay Time Measurement
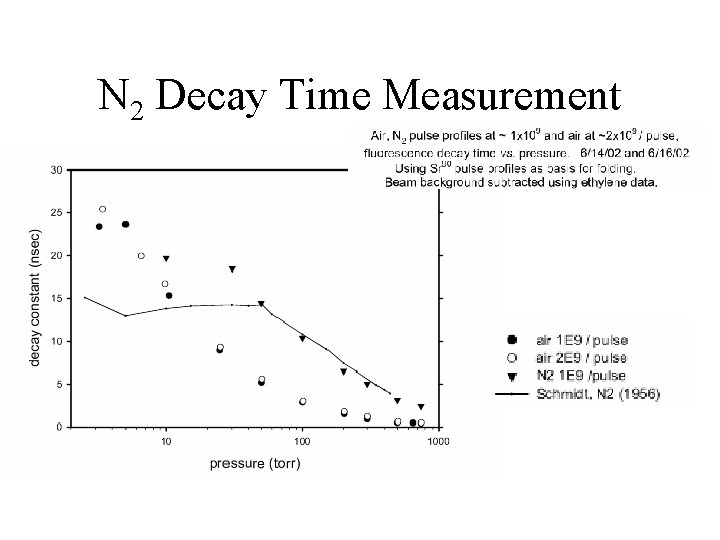
N 2 Decay Time Measurement
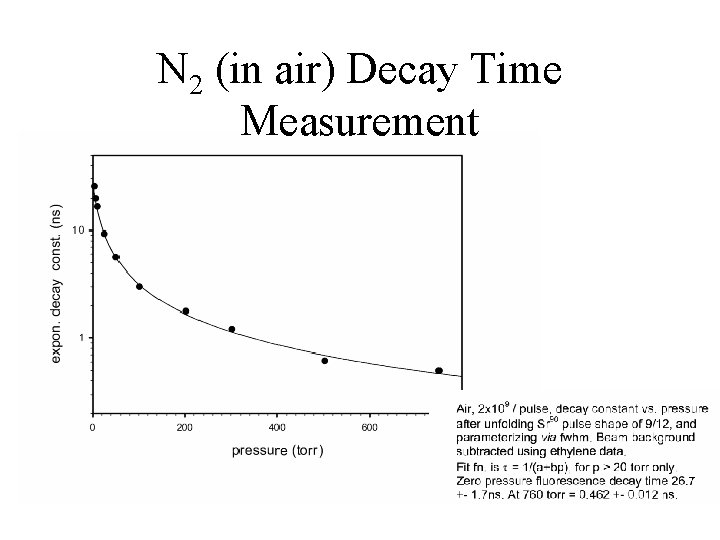
N 2 (in air) Decay Time Measurement
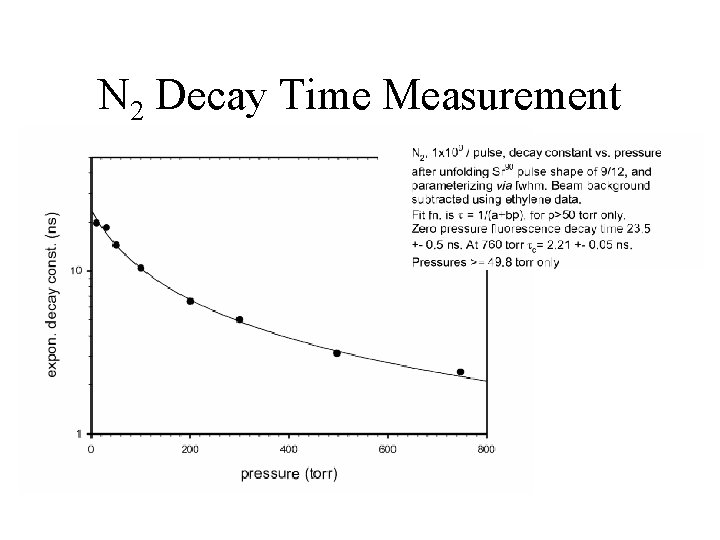
N 2 Decay Time Measurement
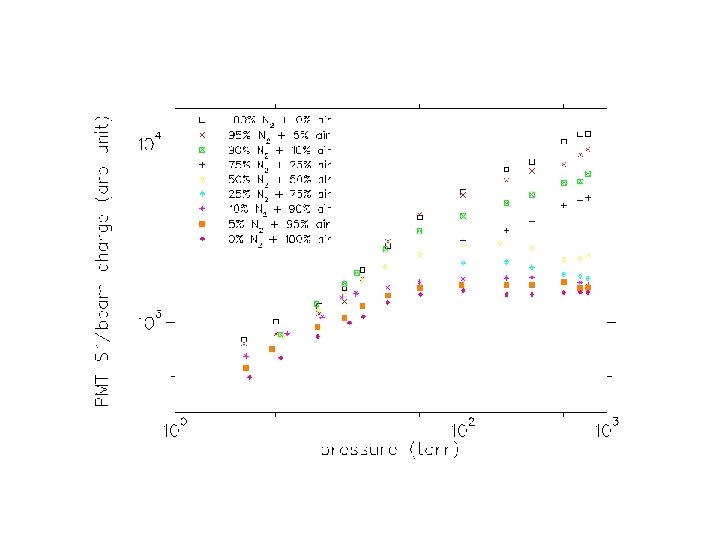
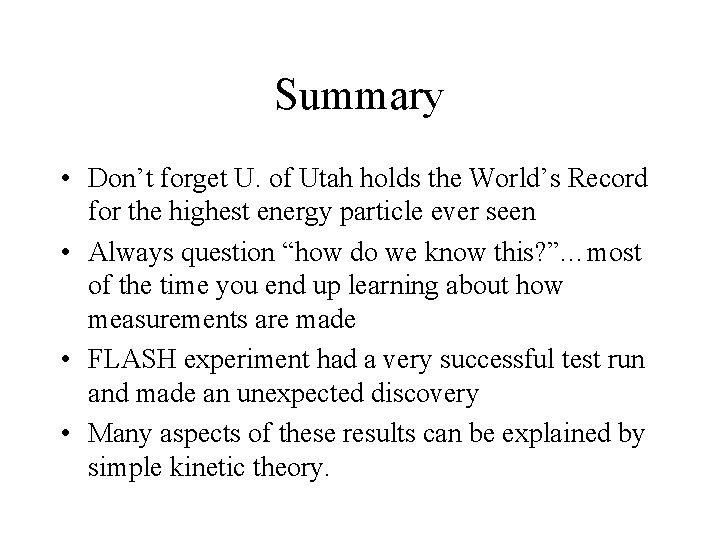
Summary • Don’t forget U. of Utah holds the World’s Record for the highest energy particle ever seen • Always question “how do we know this? ”…most of the time you end up learning about how measurements are made • FLASH experiment had a very successful test run and made an unexpected discovery • Many aspects of these results can be explained by simple kinetic theory.
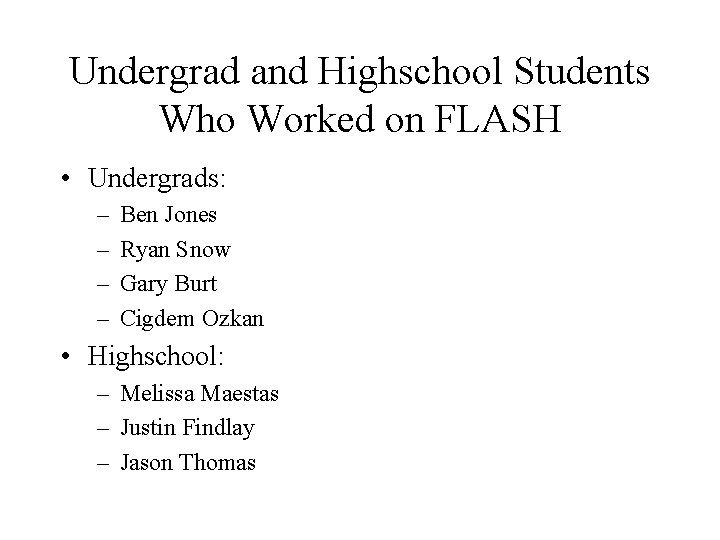
Undergrad and Highschool Students Who Worked on FLASH • Undergrads: – – Ben Jones Ryan Snow Gary Burt Cigdem Ozkan • Highschool: – Melissa Maestas – Justin Findlay – Jason Thomas
Extensive air showers
Language
Cantebury tales prologue
Patrick showers
Cosmic ray showers
Digital photography with flash and no-flash image pairs
Air higroskopis air kapiler dan air gravitasi
Principle of fluorescence spectroscopy
So4ex
Cold vapor atomic fluorescence spectrometry
Time to amplitude converter tac
Fluorescence microscope uses
Fluorescence-activated cell sorting (facs)
Fluorescence bandpass filter
Confocal fluorescence microscopy
Fluorescence recovery after photobleaching
Xrf theory ppt
Application of fluorescence spectroscopy
Jablonski diagrams
Atomic fluorescence spectroscopy principle
Cold vapor atomic fluorescence spectrometry
Compound microscope
Laser confocal microscopy
Limitations of the beer lambert law
Fluorescence activated cell sorting
Chemiluminescence vs fluorescence
F=p/2
Light sources for fluorescence microscopy
Fluorescence recovery after photobleaching
Fluorescence units
Principles of fluorescence spectroscopy
Fluorescence activated cell sorting
Slac spires
Slac citrix
Confluence slac
Andrew burrill slac
Slac
Slac summer institute
Network data collection at slac
Ip fragmentation