PTYS 554 Evolution of Planetary Surfaces Planetary Heating
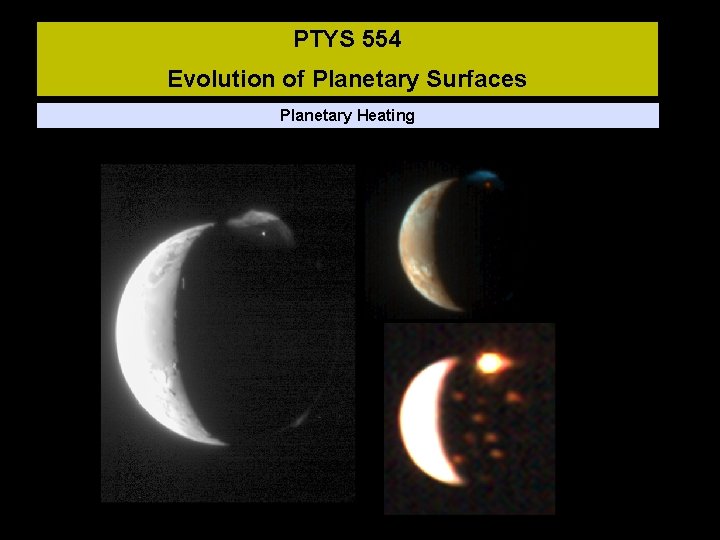
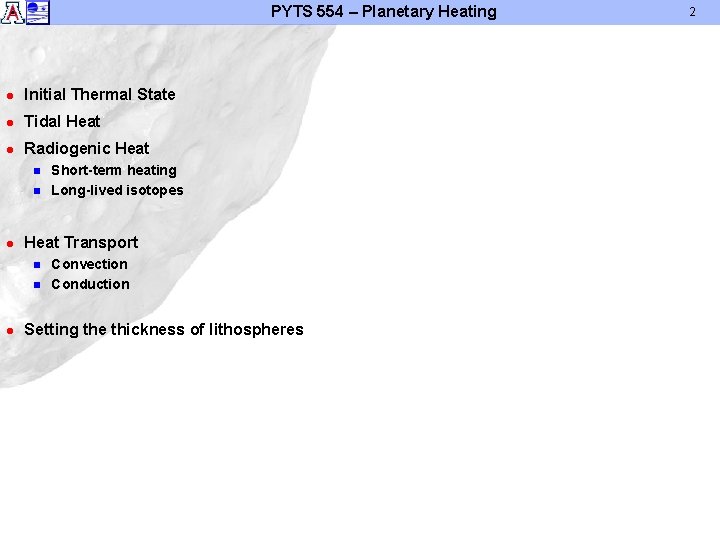
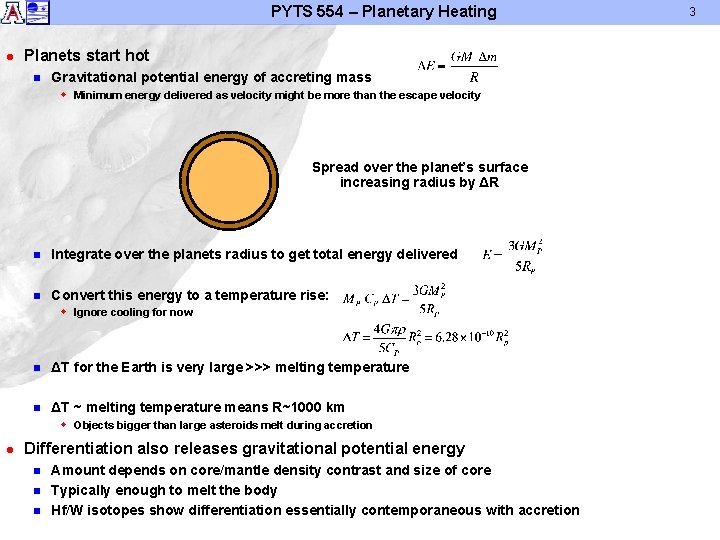
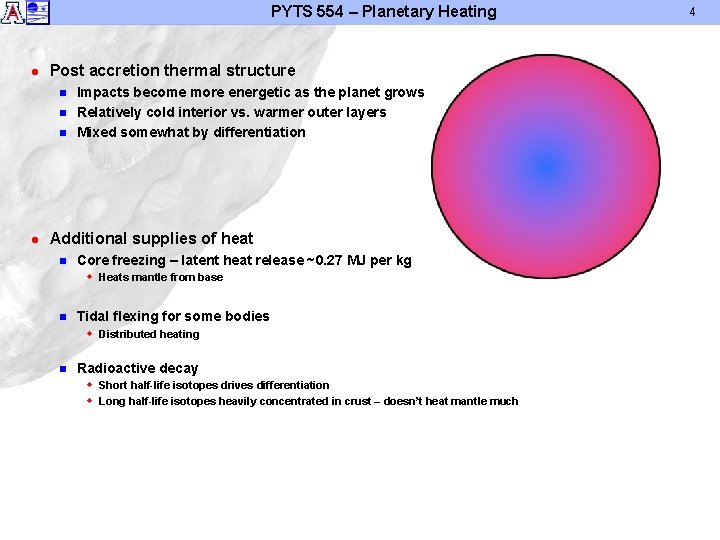
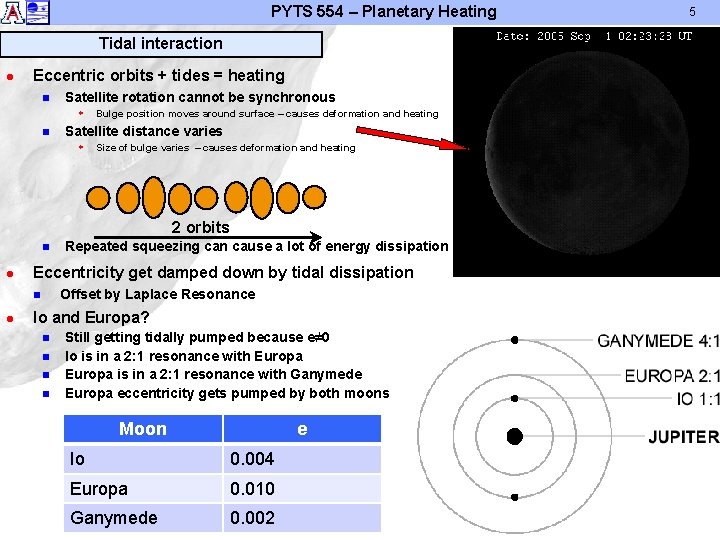
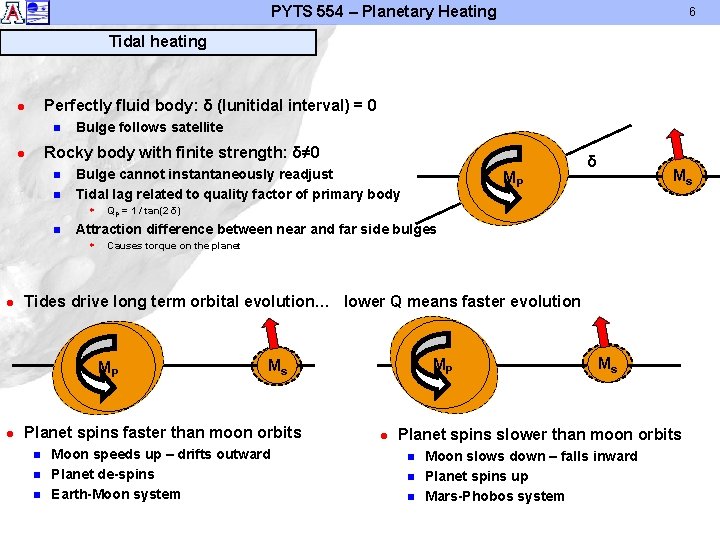
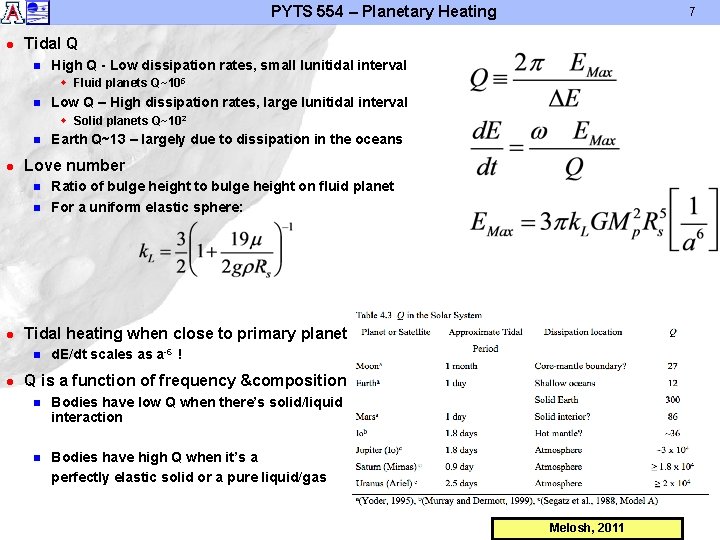
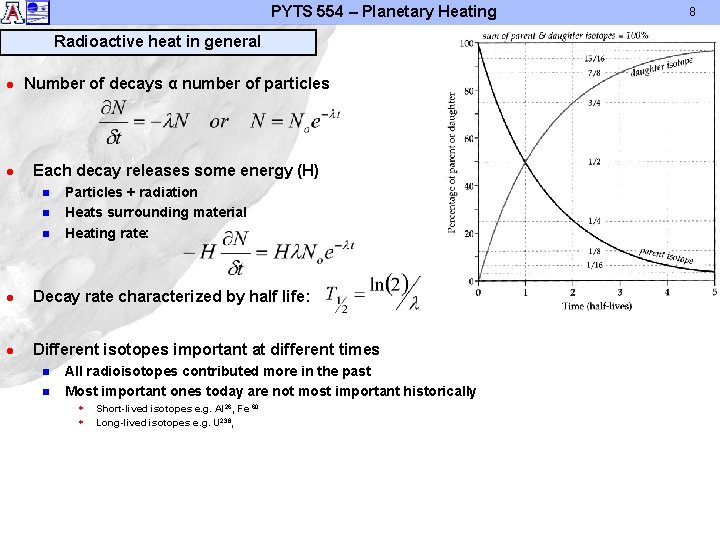
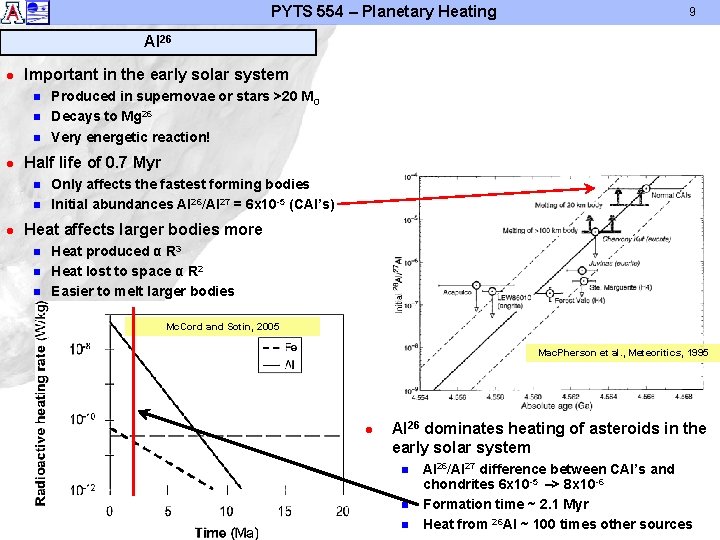
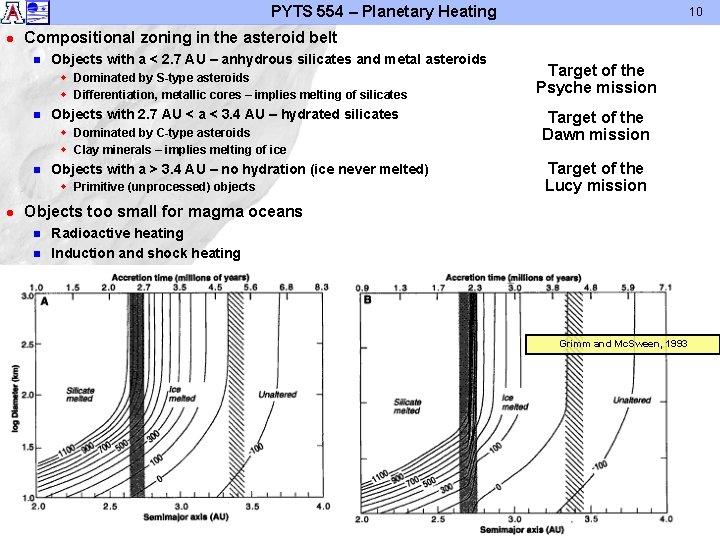
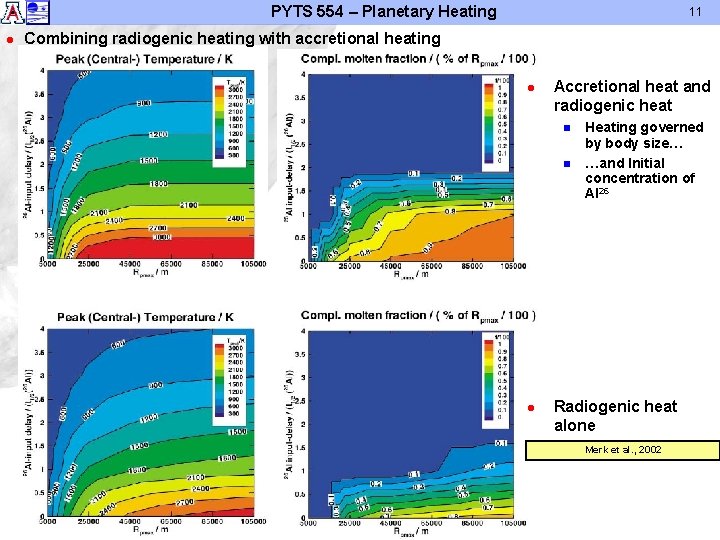
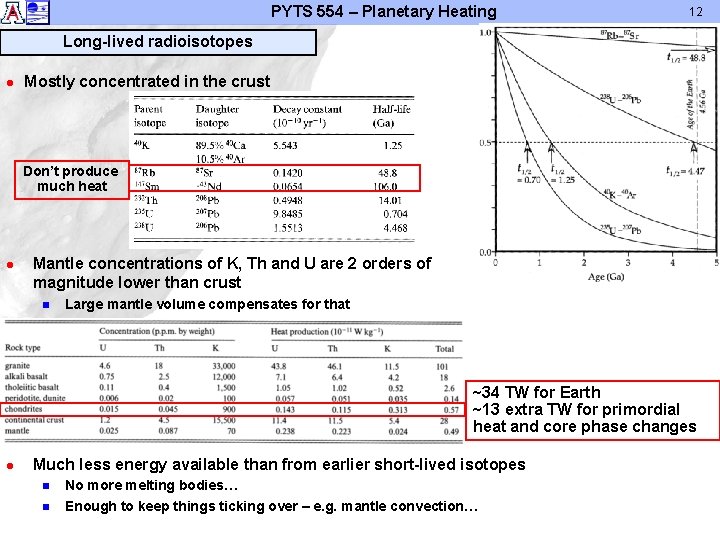
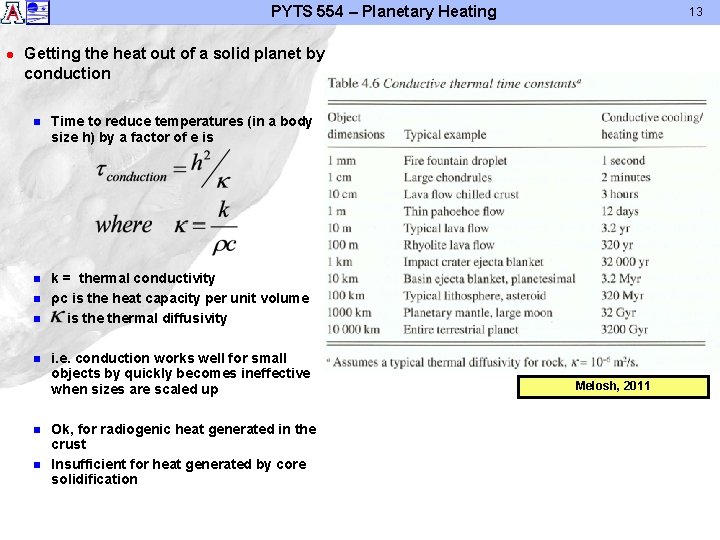
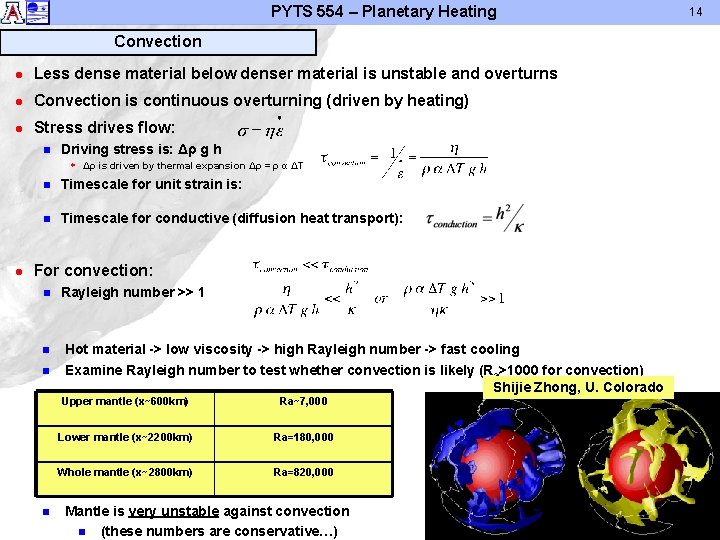
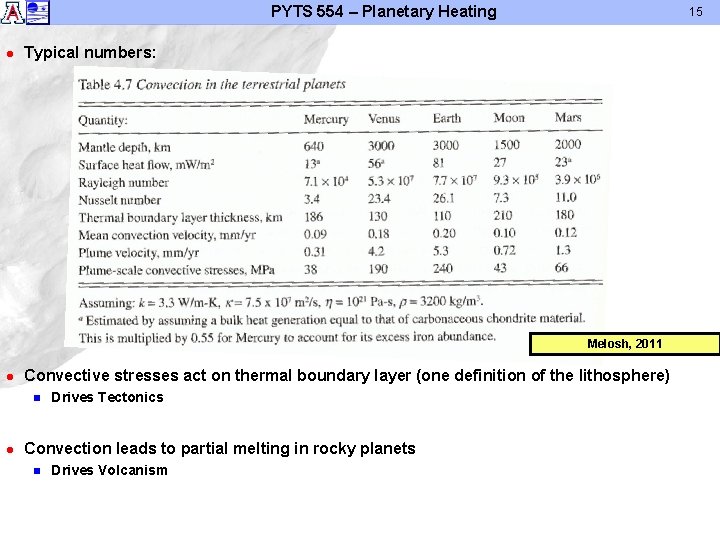
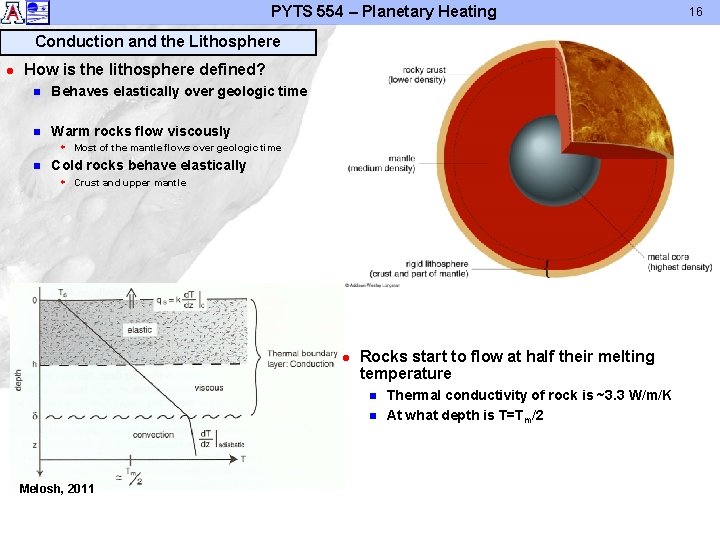
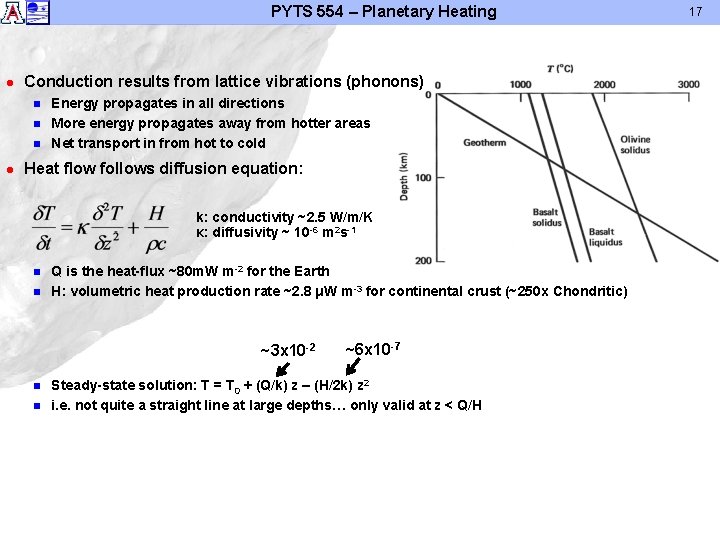
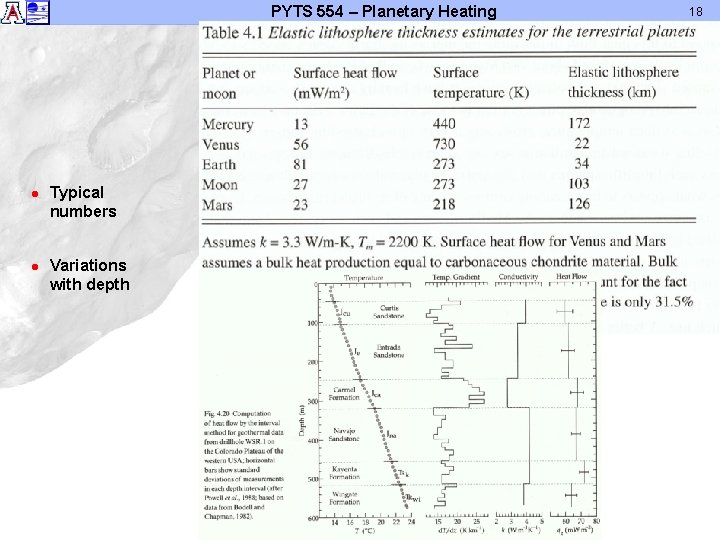
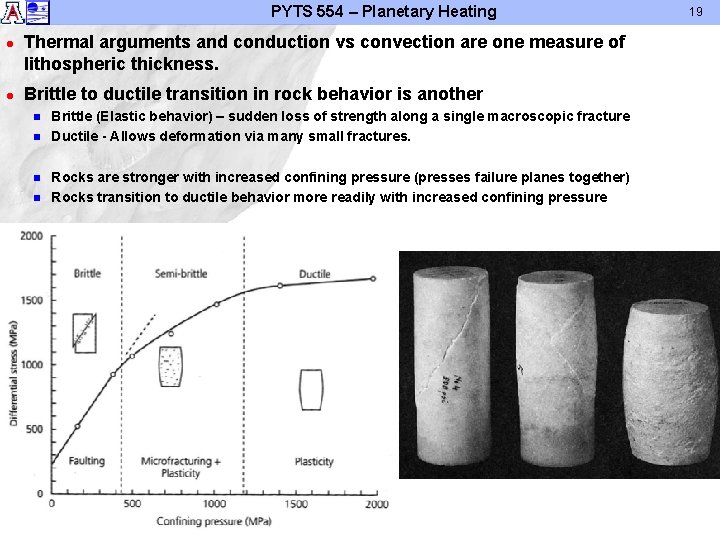
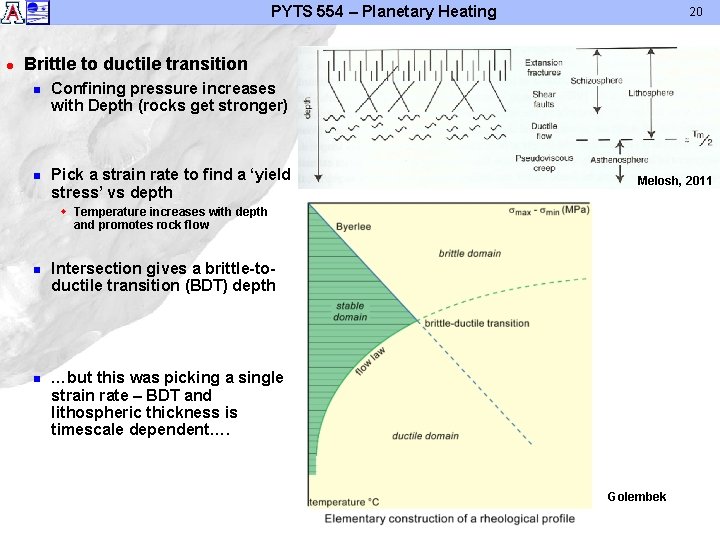
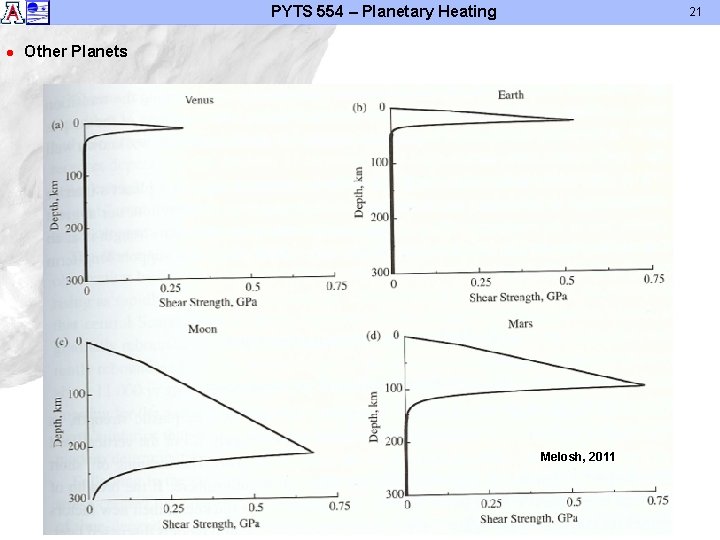
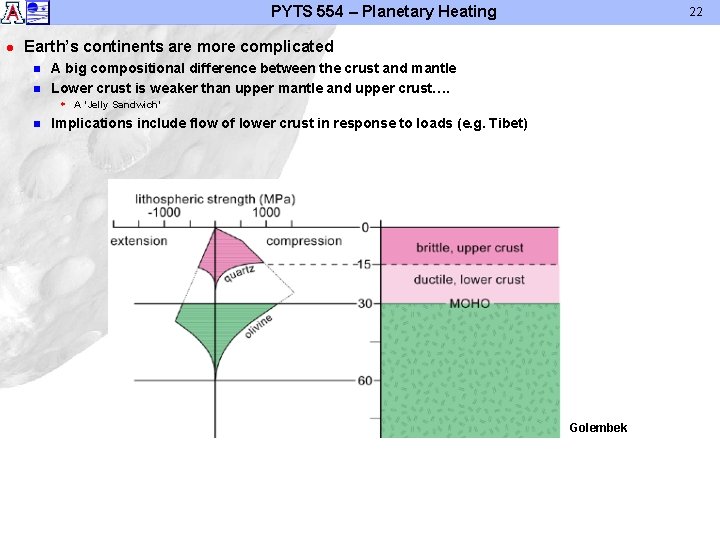
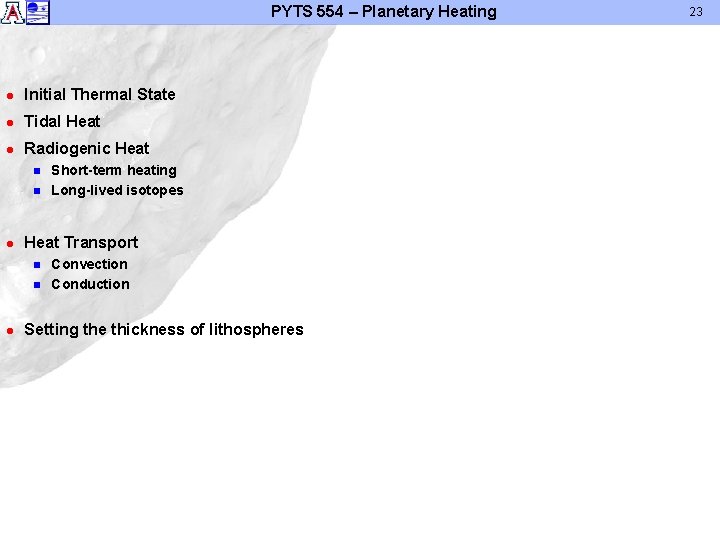
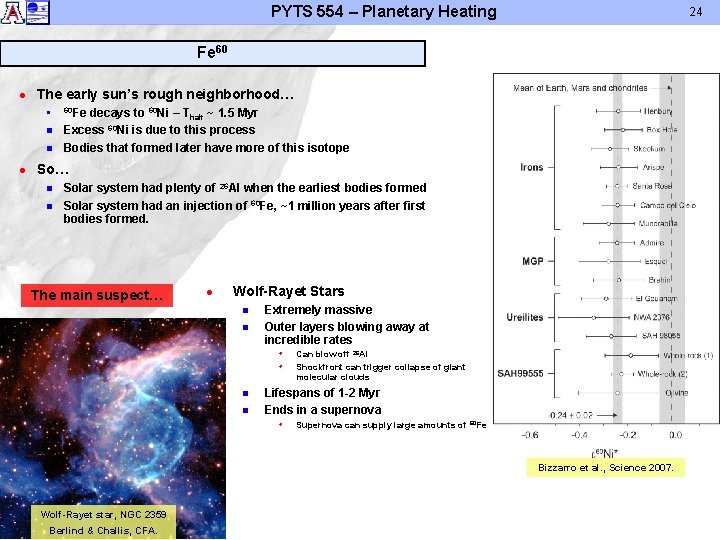
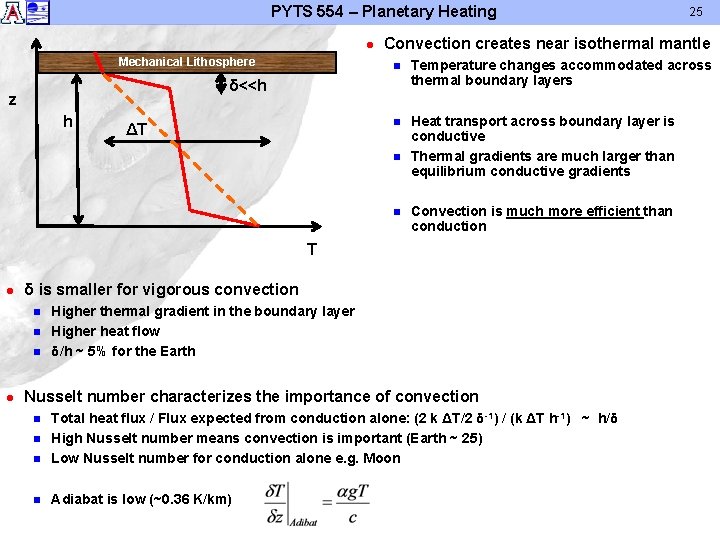
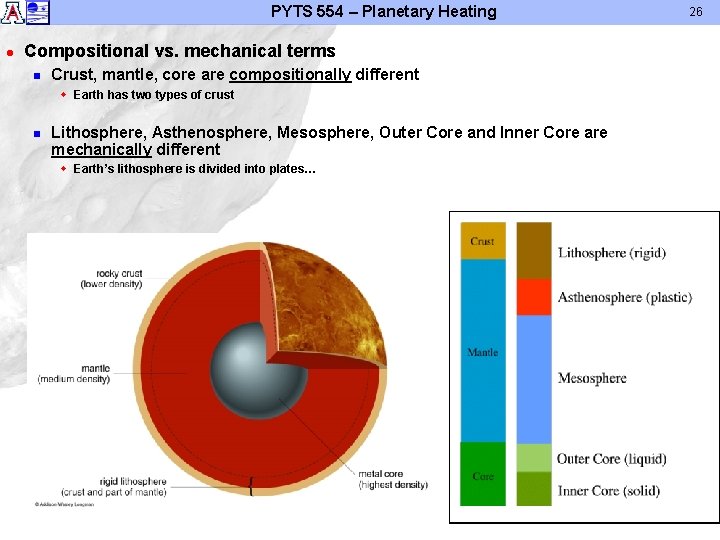
- Slides: 26
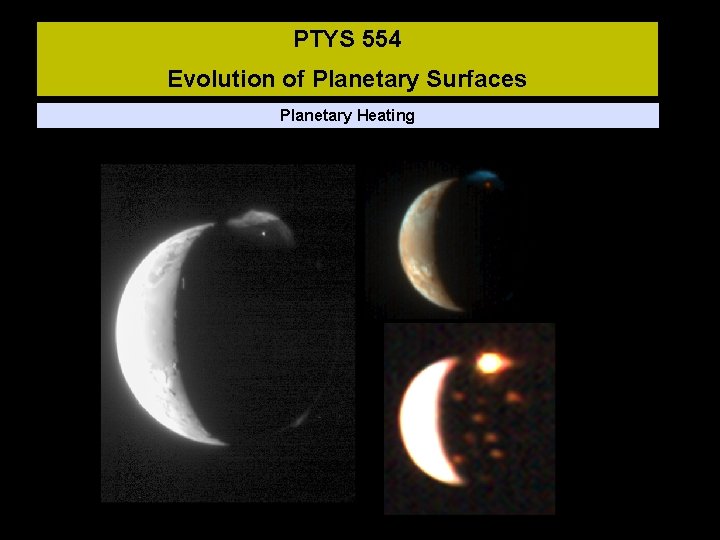
PTYS 554 Evolution of Planetary Surfaces Planetary Heating
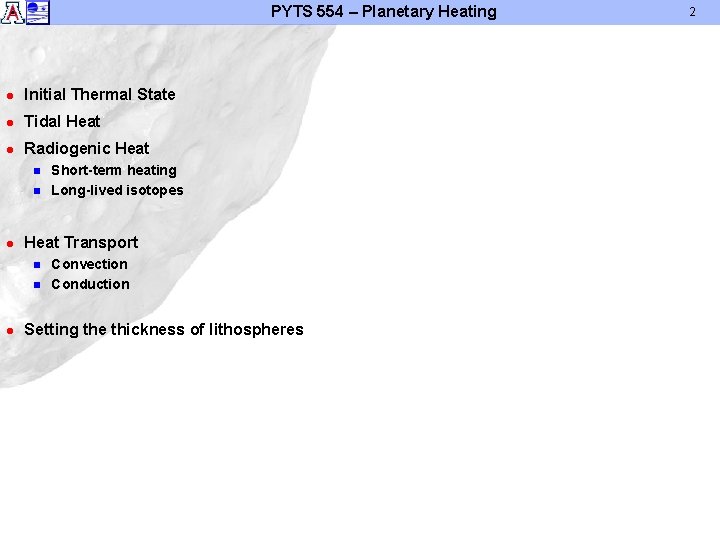
PYTS 554 – Planetary Heating l Initial Thermal State l Tidal Heat l Radiogenic Heat n n l Heat Transport n n l Short-term heating Long-lived isotopes Convection Conduction Setting the thickness of lithospheres 2
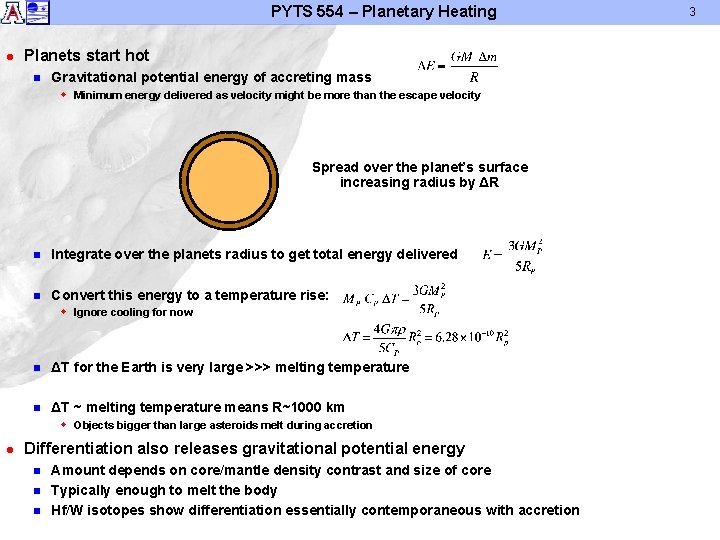
PYTS 554 – Planetary Heating l Planets start hot n Gravitational potential energy of accreting mass w Minimum energy delivered as velocity might be more than the escape velocity Spread over the planet’s surface increasing radius by ΔR n Integrate over the planets radius to get total energy delivered n Convert this energy to a temperature rise: w Ignore cooling for now n ΔT for the Earth is very large >>> melting temperature n ΔT ~ melting temperature means R~1000 km w Objects bigger than large asteroids melt during accretion l Differentiation also releases gravitational potential energy n n n Amount depends on core/mantle density contrast and size of core Typically enough to melt the body Hf/W isotopes show differentiation essentially contemporaneous with accretion 3
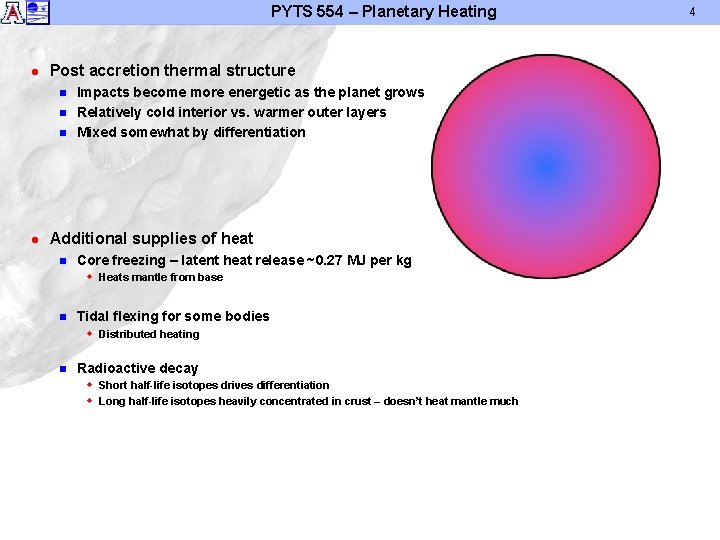
PYTS 554 – Planetary Heating l Post accretion thermal structure n n n l Impacts become more energetic as the planet grows Relatively cold interior vs. warmer outer layers Mixed somewhat by differentiation Additional supplies of heat n Core freezing – latent heat release ~0. 27 MJ per kg w Heats mantle from base n Tidal flexing for some bodies w Distributed heating n Radioactive decay w Short half-life isotopes drives differentiation w Long half-life isotopes heavily concentrated in crust – doesn’t heat mantle much 4
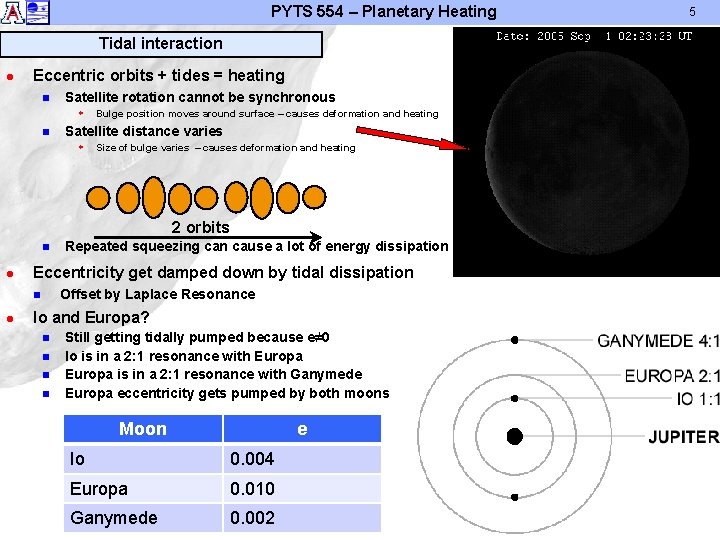
PYTS 554 – Planetary Heating Tidal interaction l Eccentric orbits + tides = heating n Satellite rotation cannot be synchronous w n Bulge position moves around surface – causes deformation and heating Satellite distance varies w Size of bulge varies – causes deformation and heating 2 orbits n l Eccentricity get damped down by tidal dissipation Offset by Laplace Resonance n l Repeated squeezing can cause a lot of energy dissipation Io and Europa? n n Still getting tidally pumped because e≠ 0 Io is in a 2: 1 resonance with Europa is in a 2: 1 resonance with Ganymede Europa eccentricity gets pumped by both moons Moon e Io 0. 004 Europa 0. 010 Ganymede 0. 002 5
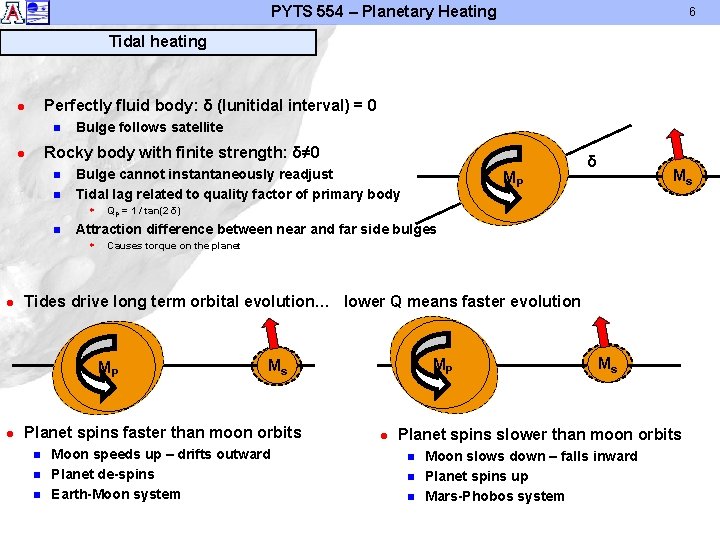
PYTS 554 – Planetary Heating 6 Tidal heating Perfectly fluid body: δ (lunitidal interval) = 0 l n Bulge follows satellite Rocky body with finite strength: δ≠ 0 l n n Bulge cannot instantaneously readjust Tidal lag related to quality factor of primary body w n QP = 1 / tan(2 δ) Causes torque on the planet Tides drive long term orbital evolution… lower Q means faster evolution MP l Ms Attraction difference between near and far side bulges w l MP δ Planet spins faster than moon orbits n n n MP Ms Moon speeds up – drifts outward Planet de-spins Earth-Moon system l Ms Planet spins slower than moon orbits n n n Moon slows down – falls inward Planet spins up Mars-Phobos system
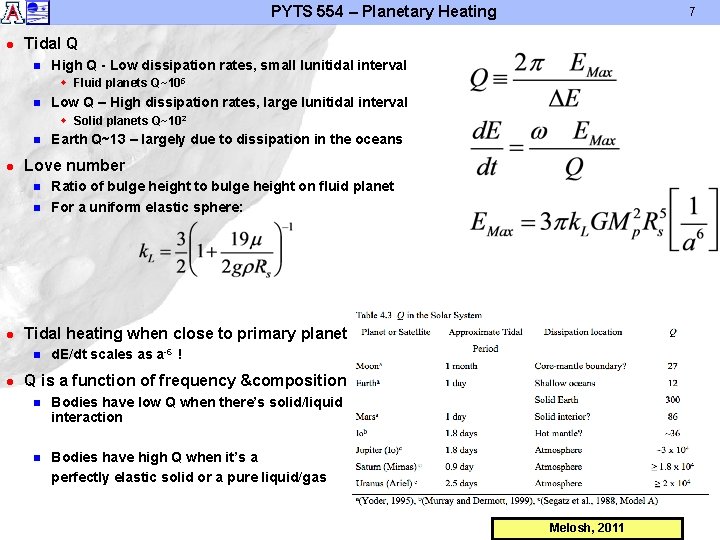
PYTS 554 – Planetary Heating l 7 Tidal Q n High Q - Low dissipation rates, small lunitidal interval w Fluid planets Q~105 n Low Q – High dissipation rates, large lunitidal interval w Solid planets Q~102 n l Love number n n l Ratio of bulge height to bulge height on fluid planet For a uniform elastic sphere: Tidal heating when close to primary planet n l Earth Q~13 – largely due to dissipation in the oceans d. E/dt scales as a-6 ! Q is a function of frequency &composition n Bodies have low Q when there’s solid/liquid interaction n Bodies have high Q when it’s a perfectly elastic solid or a pure liquid/gas Melosh, 2011
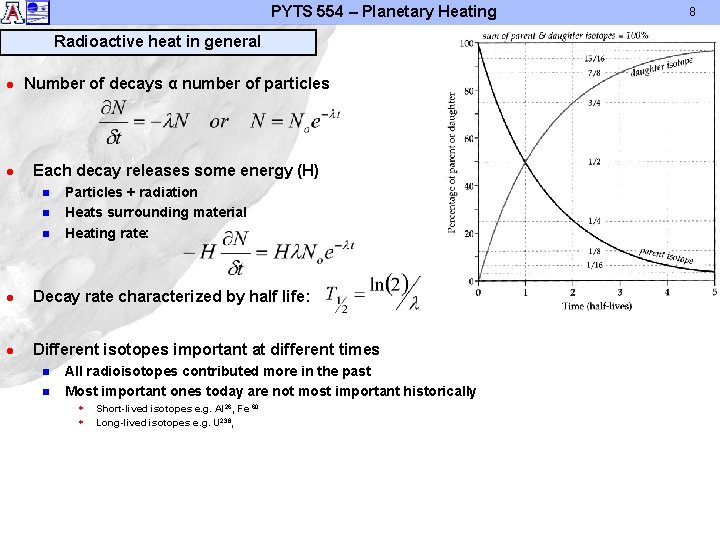
PYTS 554 – Planetary Heating Radioactive heat in general l Number of decays α number of particles l Each decay releases some energy (H) n n n Particles + radiation Heats surrounding material Heating rate: l Decay rate characterized by half life: l Different isotopes important at different times n n All radioisotopes contributed more in the past Most important ones today are not most important historically w w Short-lived isotopes e. g. Al 26, Fe 60 Long-lived isotopes e. g. U 238, 8
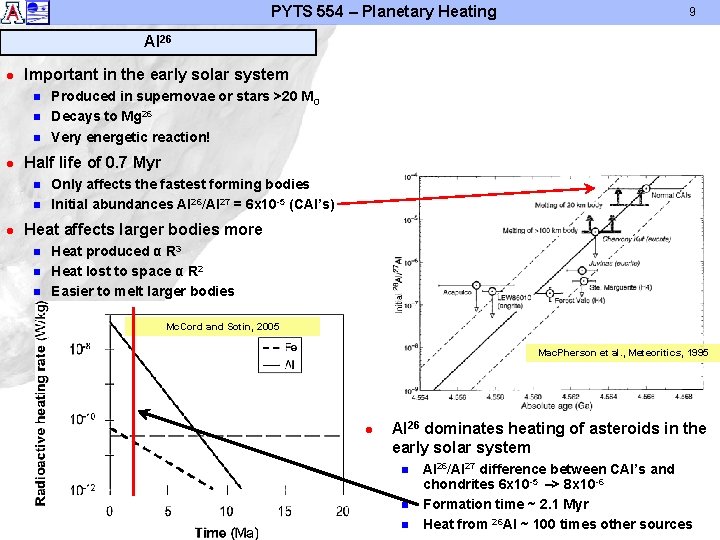
PYTS 554 – Planetary Heating 9 Al 26 l Important in the early solar system n n n l Half life of 0. 7 Myr n n l Produced in supernovae or stars >20 MO Decays to Mg 26 Very energetic reaction! Only affects the fastest forming bodies Initial abundances Al 26/Al 27 = 6 x 10 -5 (CAI’s) Heat affects larger bodies more n n n Heat produced α R 3 Heat lost to space α R 2 Easier to melt larger bodies Mc. Cord and Sotin, 2005 Mac. Pherson et al. , Meteoritics, 1995 l Al 26 dominates heating of asteroids in the early solar system n n n Al 26/Al 27 difference between CAI’s and chondrites 6 x 10 -5 –> 8 x 10 -6 Formation time ~ 2. 1 Myr Heat from 26 Al ~ 100 times other sources
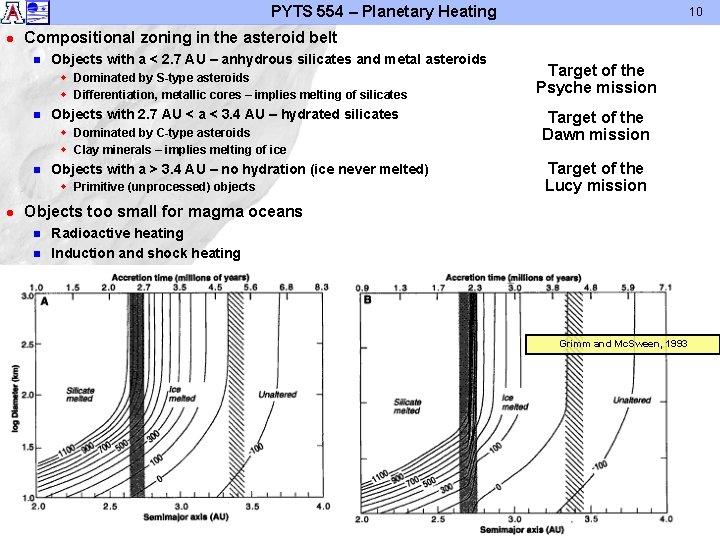
PYTS 554 – Planetary Heating l Compositional zoning in the asteroid belt n Objects with a < 2. 7 AU – anhydrous silicates and metal asteroids w Dominated by S-type asteroids w Differentiation, metallic cores – implies melting of silicates n Objects with 2. 7 AU < a < 3. 4 AU – hydrated silicates w Dominated by C-type asteroids w Clay minerals – implies melting of ice n Objects with a > 3. 4 AU – no hydration (ice never melted) w Primitive (unprocessed) objects l 10 Target of the Psyche mission Target of the Dawn mission Target of the Lucy mission Objects too small for magma oceans n n Radioactive heating Induction and shock heating Grimm and Mc. Sween, 1993
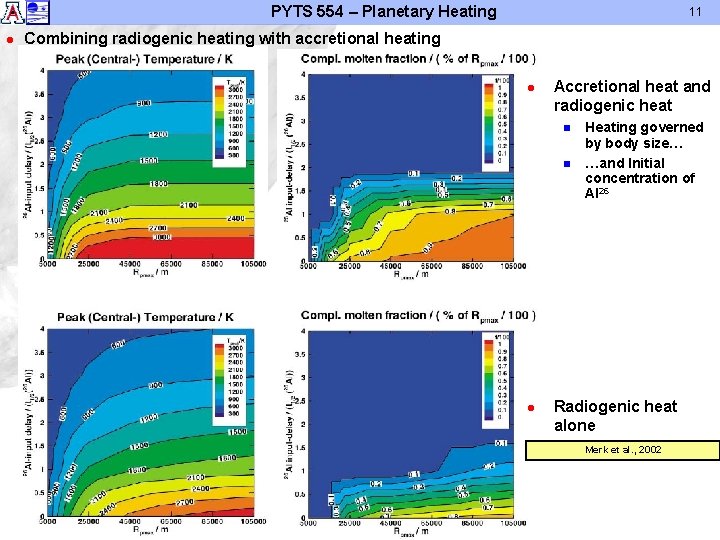
PYTS 554 – Planetary Heating l 11 Combining radiogenic heating with accretional heating l Accretional heat and radiogenic heat n n l Heating governed by body size… …and Initial concentration of Al 26 Radiogenic heat alone Merk et al. , 2002
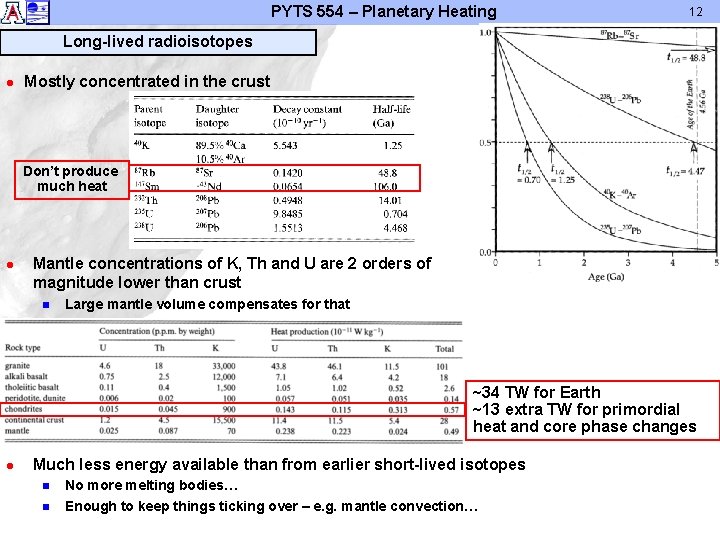
PYTS 554 – Planetary Heating 12 Long-lived radioisotopes l Mostly concentrated in the crust Don’t produce much heat l Mantle concentrations of K, Th and U are 2 orders of magnitude lower than crust n Large mantle volume compensates for that ~34 TW for Earth ~13 extra TW for primordial heat and core phase changes l Much less energy available than from earlier short-lived isotopes n n No more melting bodies… Enough to keep things ticking over – e. g. mantle convection…
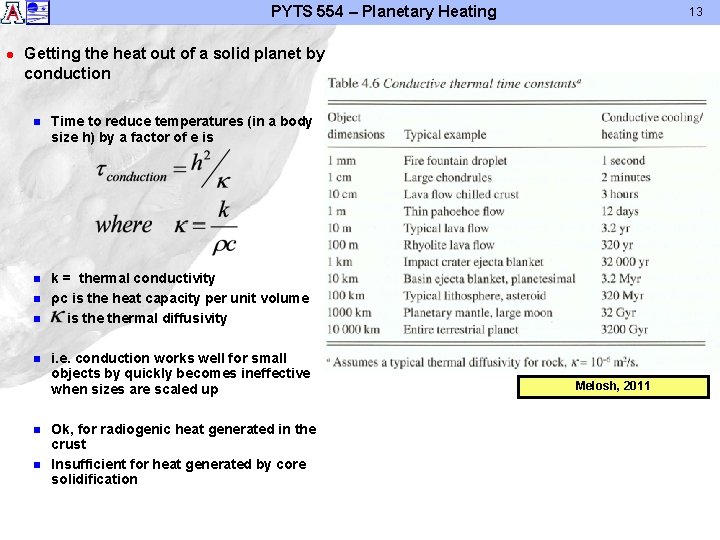
PYTS 554 – Planetary Heating l 13 Getting the heat out of a solid planet by conduction n Time to reduce temperatures (in a body size h) by a factor of e is n k = thermal conductivity ρc is the heat capacity per unit volume is thermal diffusivity n n n i. e. conduction works well for small objects by quickly becomes ineffective when sizes are scaled up Ok, for radiogenic heat generated in the crust Insufficient for heat generated by core solidification Melosh, 2011
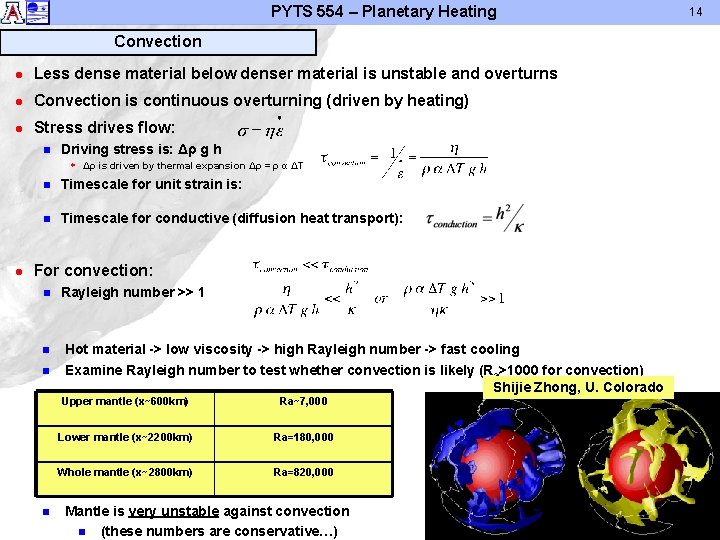
PYTS 554 – Planetary Heating Convection l Less dense material below denser material is unstable and overturns l Convection is continuous overturning (driven by heating) l Stress drives flow: n Driving stress is: Δρ g h w Δρ is driven by thermal expansion Δρ = ρ α ΔT l n Timescale for unit strain is: n Timescale for conductive (diffusion heat transport): For convection: n n Rayleigh number >> 1 Hot material -> low viscosity -> high Rayleigh number -> fast cooling Examine Rayleigh number to test whether convection is likely (R a>1000 for convection) Shijie Zhong, U. Colorado Upper mantle (x~600 km) Ra~7, 000 Lower mantle (x~2200 km) Ra=180, 000 Whole mantle (x~2800 km) Ra=820, 000 Mantle is very unstable against convection n (these numbers are conservative…) 14
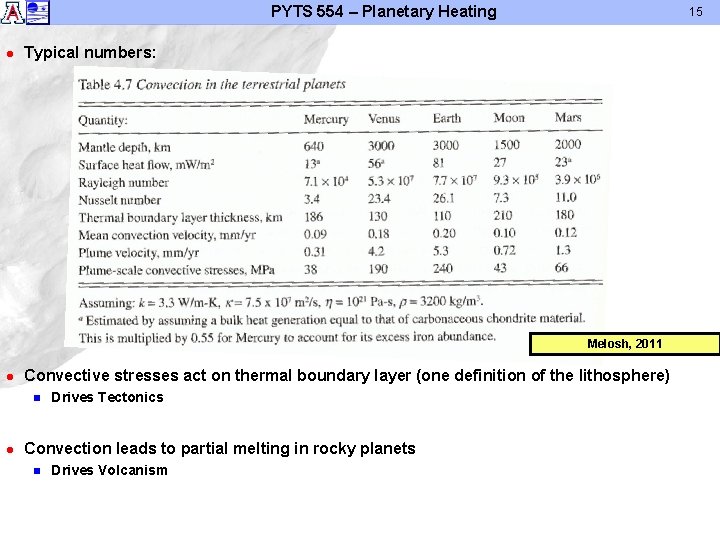
PYTS 554 – Planetary Heating l 15 Typical numbers: Melosh, 2011 l Convective stresses act on thermal boundary layer (one definition of the lithosphere) n l Drives Tectonics Convection leads to partial melting in rocky planets n Drives Volcanism
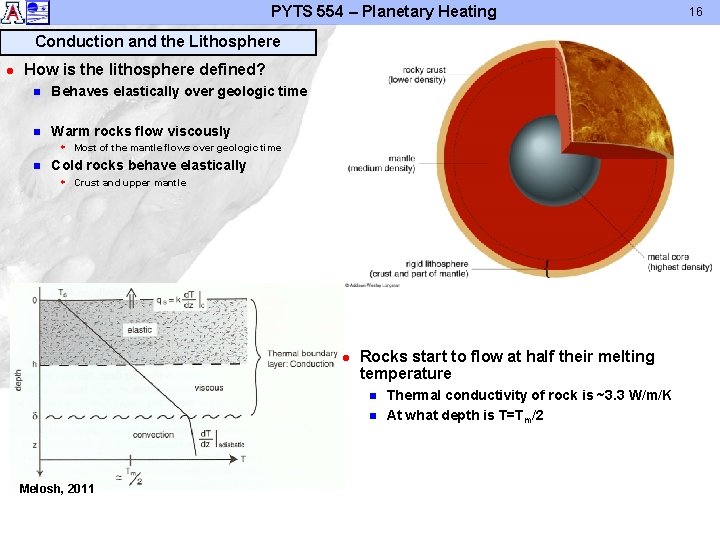
PYTS 554 – Planetary Heating Conduction and the Lithosphere l How is the lithosphere defined? n Behaves elastically over geologic time n Warm rocks flow viscously w Most of the mantle flows over geologic time n Cold rocks behave elastically w Crust and upper mantle l Rocks start to flow at half their melting temperature n n Melosh, 2011 Thermal conductivity of rock is ~3. 3 W/m/K At what depth is T=Tm/2 16
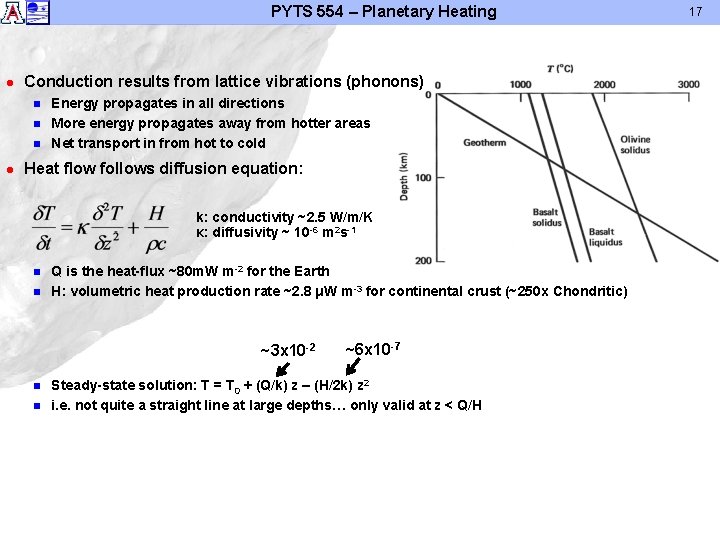
PYTS 554 – Planetary Heating l Conduction results from lattice vibrations (phonons) n n n l Energy propagates in all directions More energy propagates away from hotter areas Net transport in from hot to cold Heat flow follows diffusion equation: k: conductivity ~2. 5 W/m/K κ: diffusivity ~ 10 -6 m 2 s-1 n n Q is the heat-flux ~80 m. W m-2 for the Earth H: volumetric heat production rate ~2. 8 μW m-3 for continental crust (~250 x Chondritic) ~3 x 10 -2 n n ~6 x 10 -7 Steady-state solution: T = T 0 + (Q/k) z – (H/2 k) z 2 i. e. not quite a straight line at large depths… only valid at z < Q/H 17
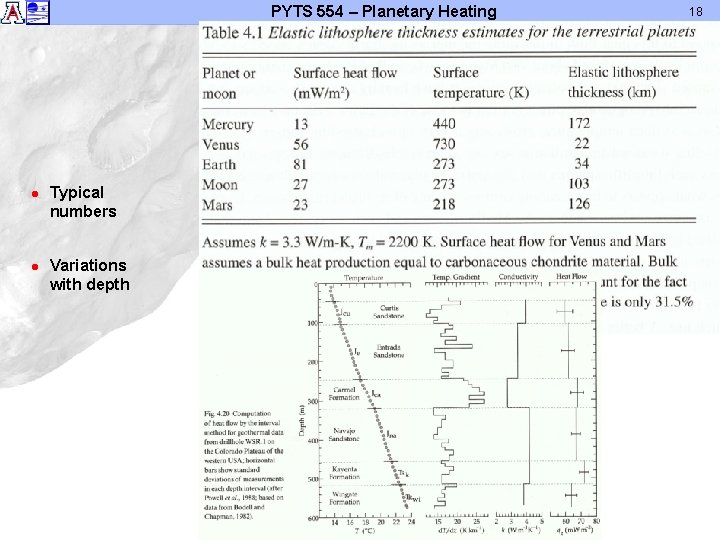
PYTS 554 – Planetary Heating l l Typical numbers Variations with depth 18
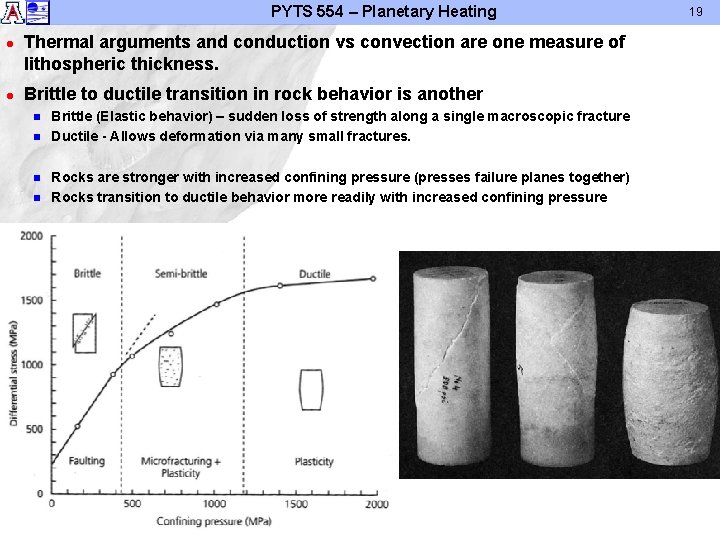
PYTS 554 – Planetary Heating l l Thermal arguments and conduction vs convection are one measure of lithospheric thickness. Brittle to ductile transition in rock behavior is another n n Brittle (Elastic behavior) – sudden loss of strength along a single macroscopic fracture Ductile - Allows deformation via many small fractures. Rocks are stronger with increased confining pressure (presses failure planes together) Rocks transition to ductile behavior more readily with increased confining pressure 19
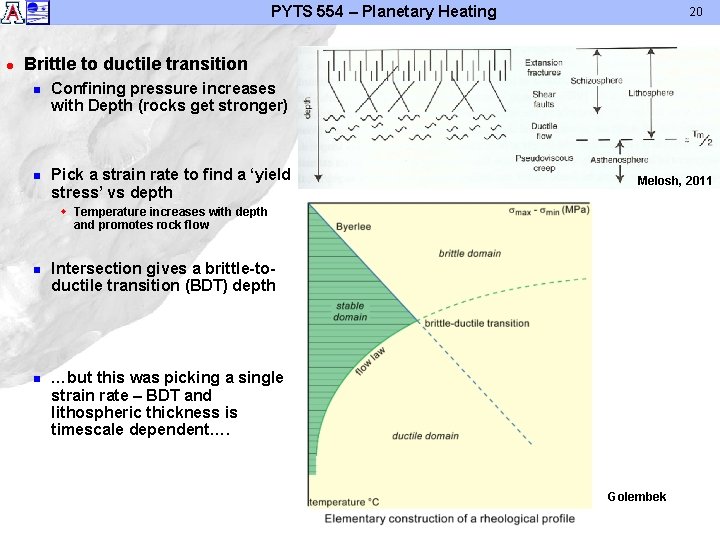
PYTS 554 – Planetary Heating l 20 Brittle to ductile transition n Confining pressure increases with Depth (rocks get stronger) n Pick a strain rate to find a ‘yield stress’ vs depth Melosh, 2011 w Temperature increases with depth and promotes rock flow n Intersection gives a brittle-toductile transition (BDT) depth n …but this was picking a single strain rate – BDT and lithospheric thickness is timescale dependent…. Golembek
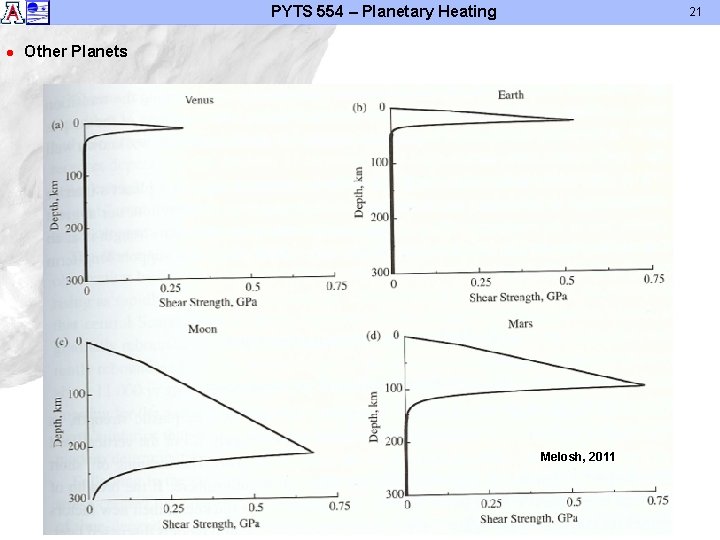
PYTS 554 – Planetary Heating l 21 Other Planets Melosh, 2011
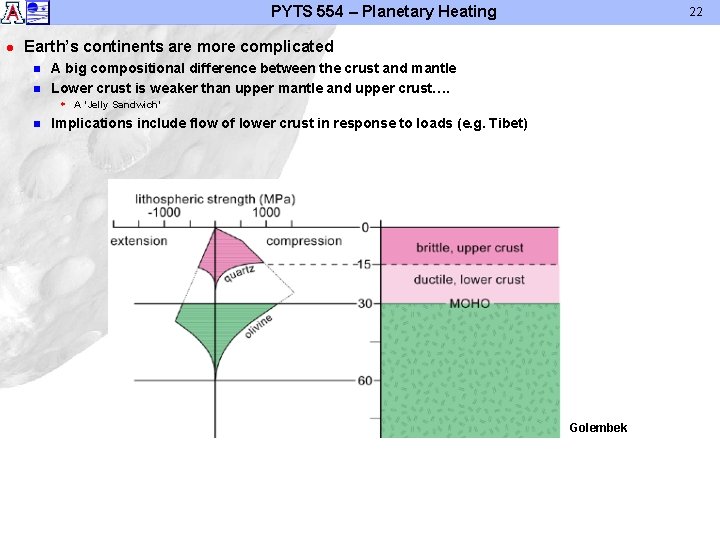
PYTS 554 – Planetary Heating l 22 Earth’s continents are more complicated n n A big compositional difference between the crust and mantle Lower crust is weaker than upper mantle and upper crust…. w A ‘Jelly Sandwich’ n Implications include flow of lower crust in response to loads (e. g. Tibet) Golembek
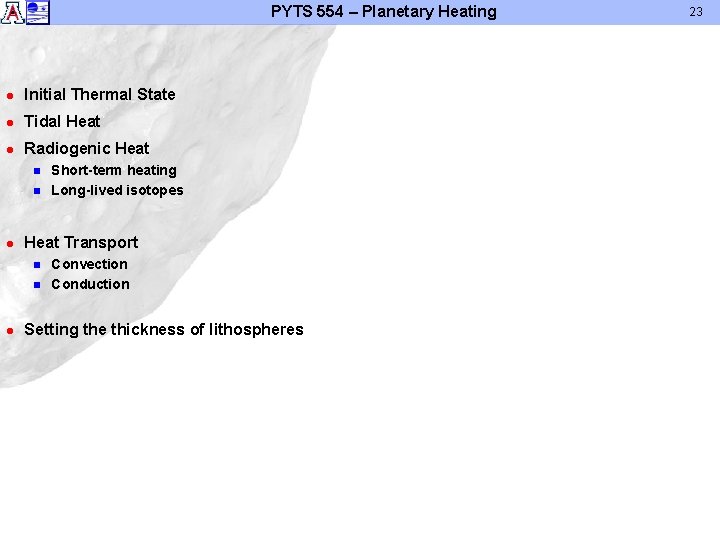
PYTS 554 – Planetary Heating l Initial Thermal State l Tidal Heat l Radiogenic Heat n n l Heat Transport n n l Short-term heating Long-lived isotopes Convection Conduction Setting the thickness of lithospheres 23
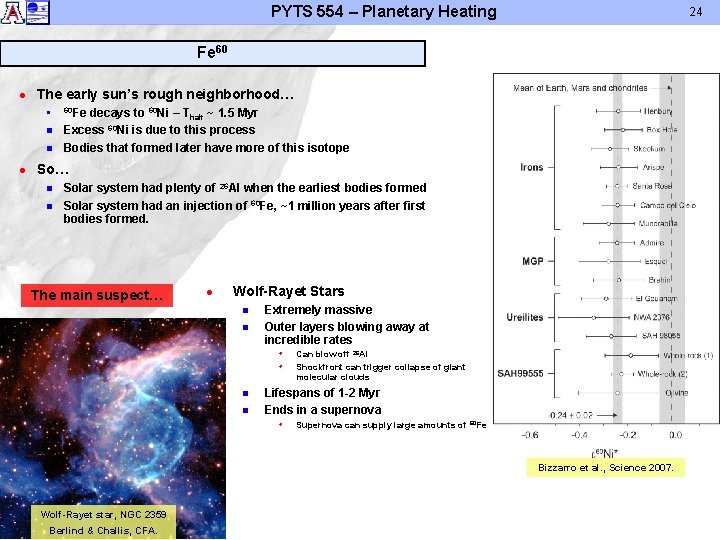
PYTS 554 – Planetary Heating 24 Fe 60 l The early sun’s rough neighborhood… n n n l 60 Fe decays to 60 Ni – Thalf ~ 1. 5 Myr Excess 60 Ni is due to this process Bodies that formed later have more of this isotope So… n n Solar system had plenty of 26 Al when the earliest bodies formed Solar system had an injection of 60 Fe, ~1 million years after first bodies formed. The main suspect… l Wolf-Rayet Stars n n Extremely massive Outer layers blowing away at incredible rates w w n n Can blow off 26 Al Shockfront can trigger collapse of giant molecular clouds Lifespans of 1 -2 Myr Ends in a supernova w Supernova can supply large amounts of 60 Fe Bizzarro et al. , Science 2007. Wolf-Rayet star, NGC 2359 Berlind & Challis, CFA.
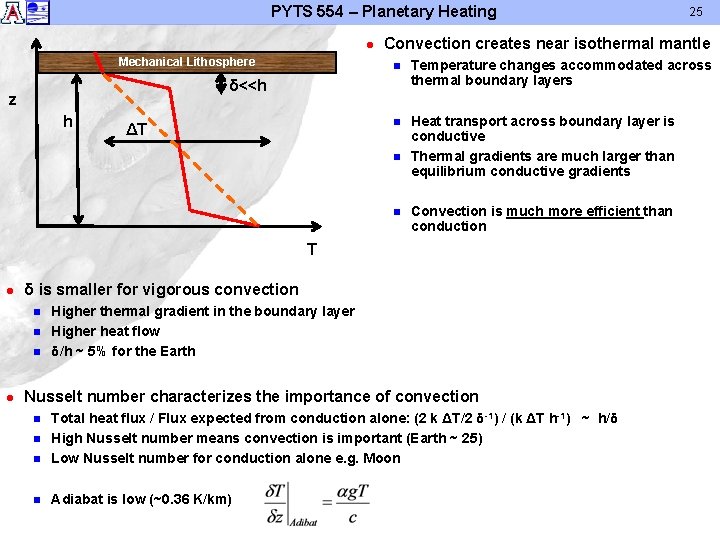
PYTS 554 – Planetary Heating l Mechanical Lithosphere Convection creates near isothermal mantle n Temperature changes accommodated across thermal boundary layers n Heat transport across boundary layer is conductive Thermal gradients are much larger than equilibrium conductive gradients δ<<h z h ΔT n n Convection is much more efficient than conduction T l δ is smaller for vigorous convection n l Higher thermal gradient in the boundary layer Higher heat flow δ/h ~ 5% for the Earth Nusselt number characterizes the importance of convection n Total heat flux / Flux expected from conduction alone: (2 k ΔT/2 δ -1) / (k ΔT h-1) ~ h/δ High Nusselt number means convection is important (Earth ~ 25) Low Nusselt number for conduction alone e. g. Moon n Adiabat is low (~0. 36 K/km) n n 25
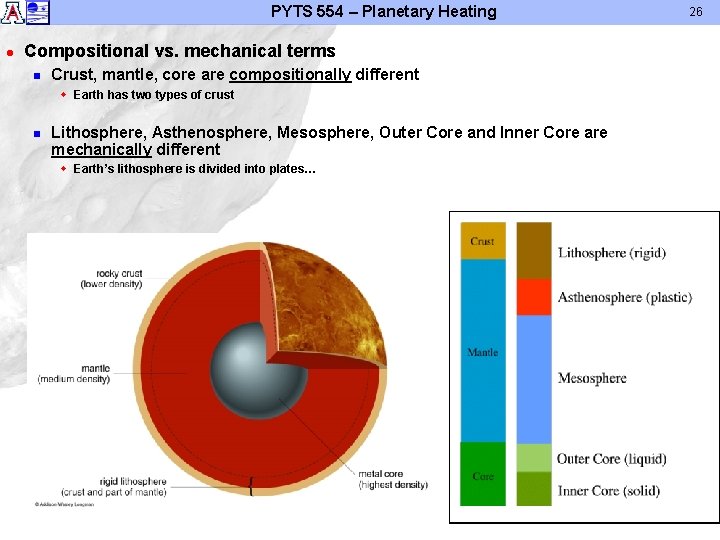
PYTS 554 – Planetary Heating l Compositional vs. mechanical terms n Crust, mantle, core are compositionally different w Earth has two types of crust n Lithosphere, Asthenosphere, Mesosphere, Outer Core and Inner Core are mechanically different w Earth’s lithosphere is divided into plates… 26
Ptys
Ptys
Dielectric heating
En 554
554 romeinse cijfers
Ece 554
Planetary data analysis
Kepler's three laws of planetary motion
Planetary protection
Association of lunar and planetary observers
Environmental wisdom worldview
Planetary systems
Planetary model
Wide field and planetary camera 2
Nasa planetary science
Prius planetary gear animation
Law of planetary motion
Hr 8799
Planetary disc
Mars jupiter and saturn show retrograde motion because
Kepler's law of planetary motion
Planetary temperature calculator
Planetary mixer definition
Today planets position
Gravitational force images
Alchemical language
The prevailing winds at 45˚s latitude are from the