Parallel Simulation of Electron Cooling Physics for Relativistic
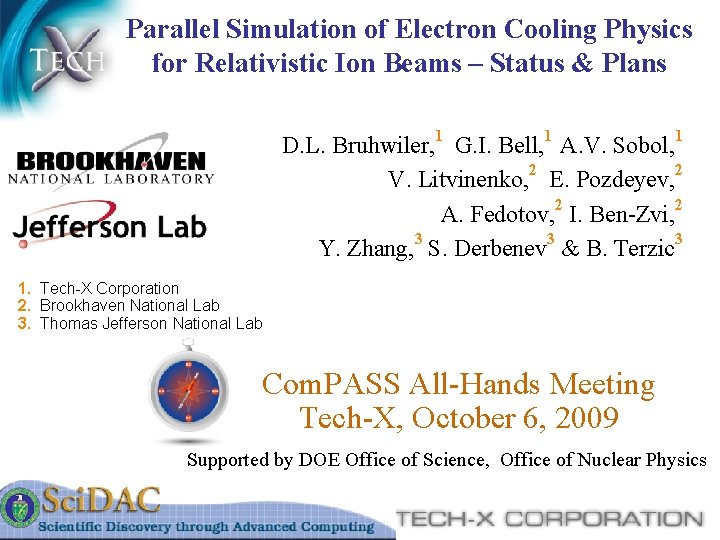
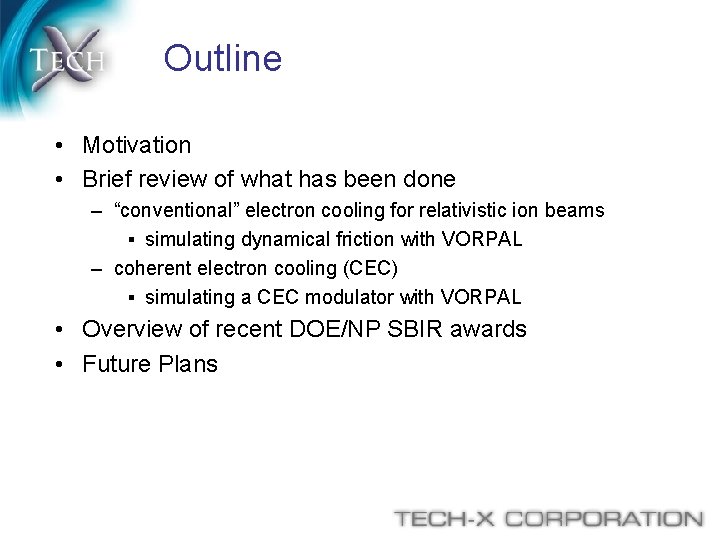
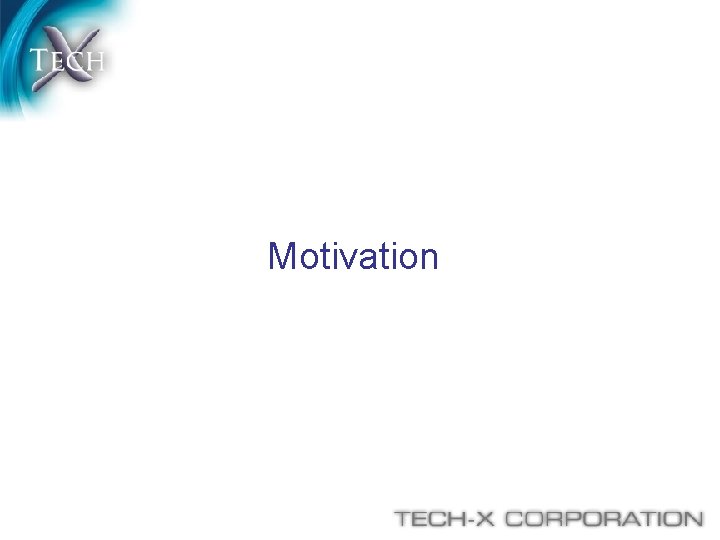
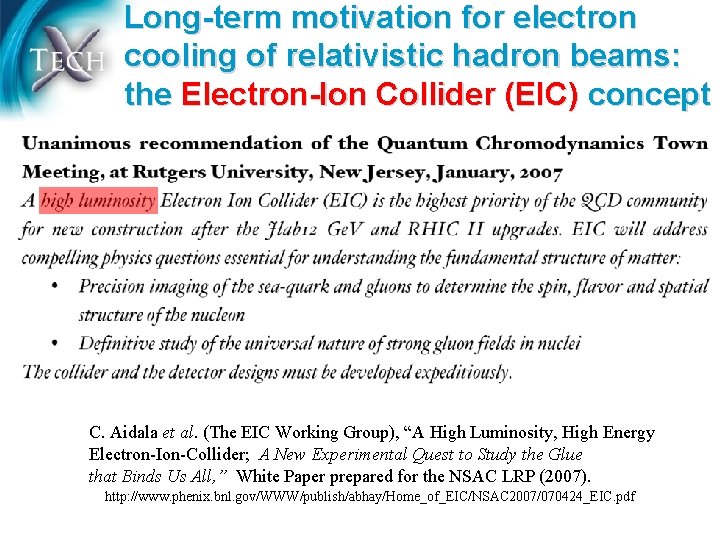
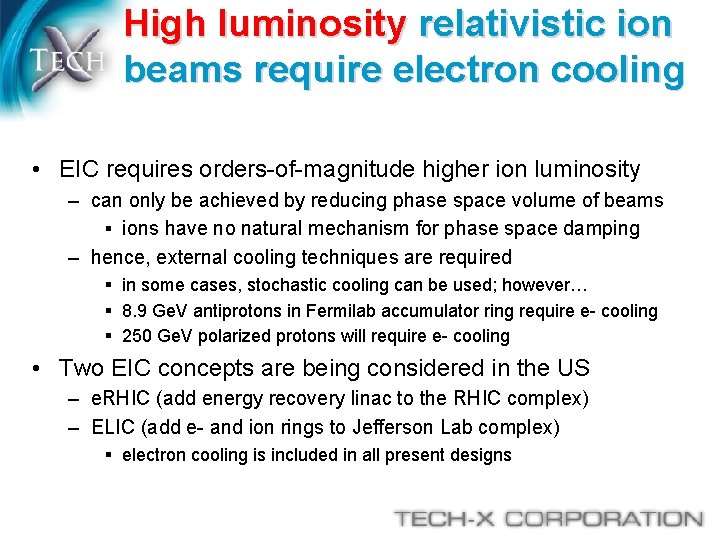
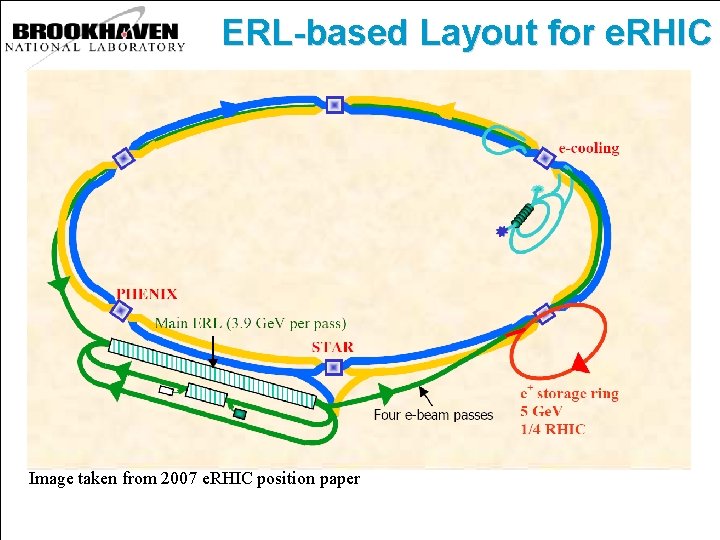
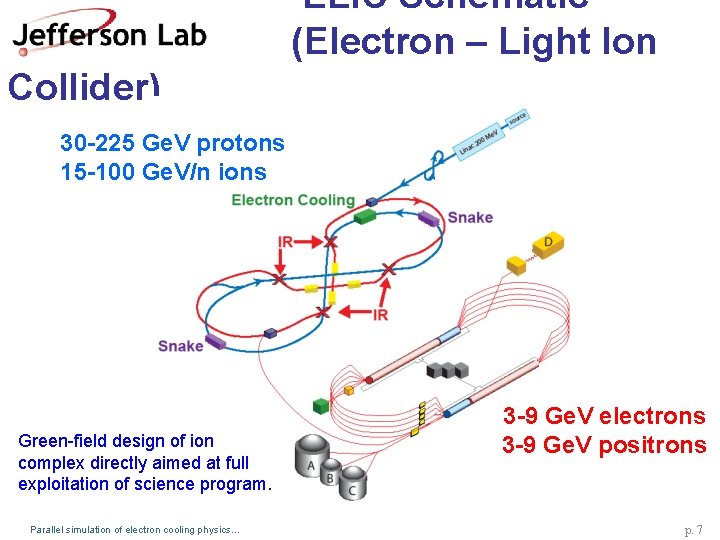
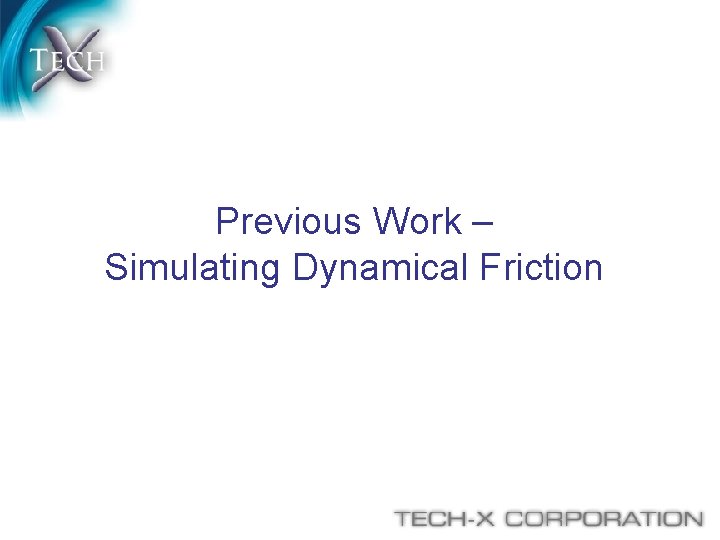
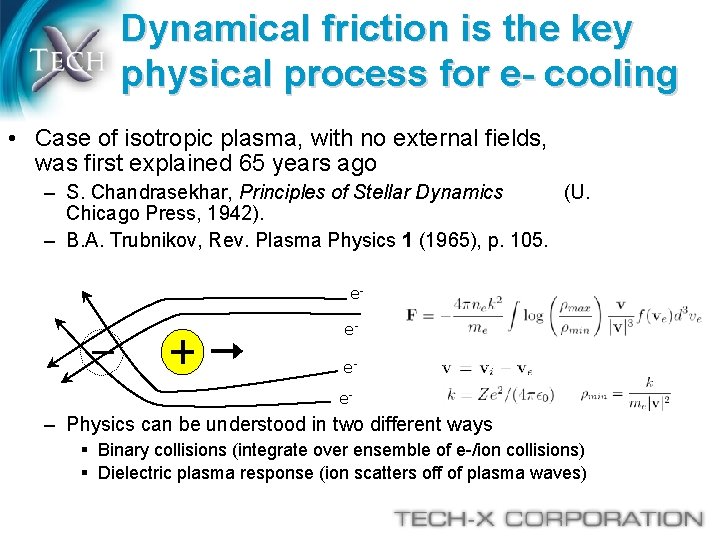
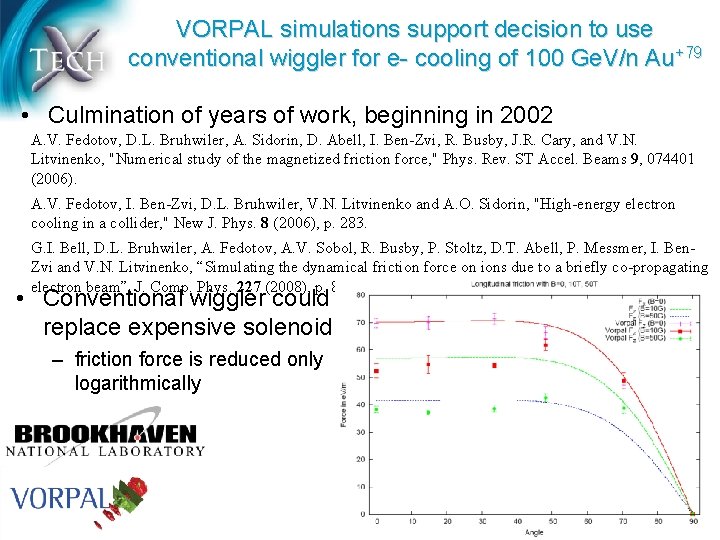
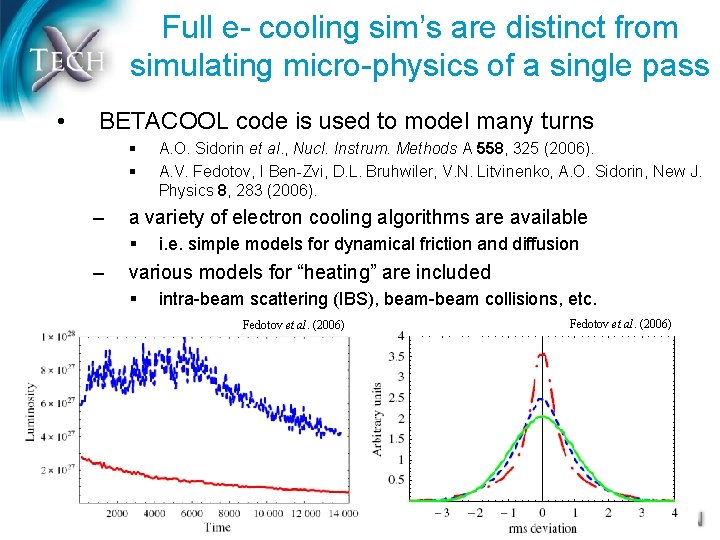
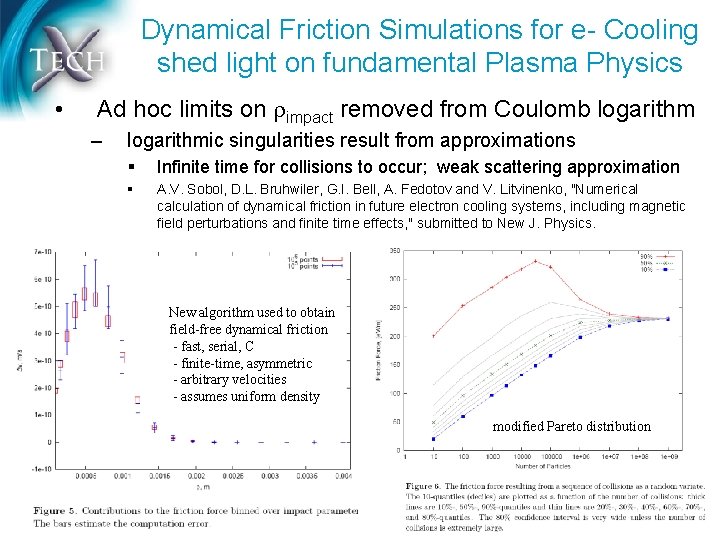
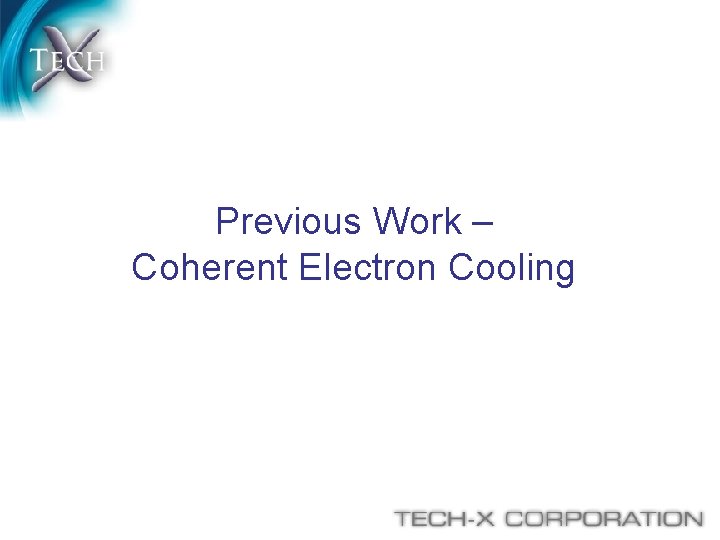
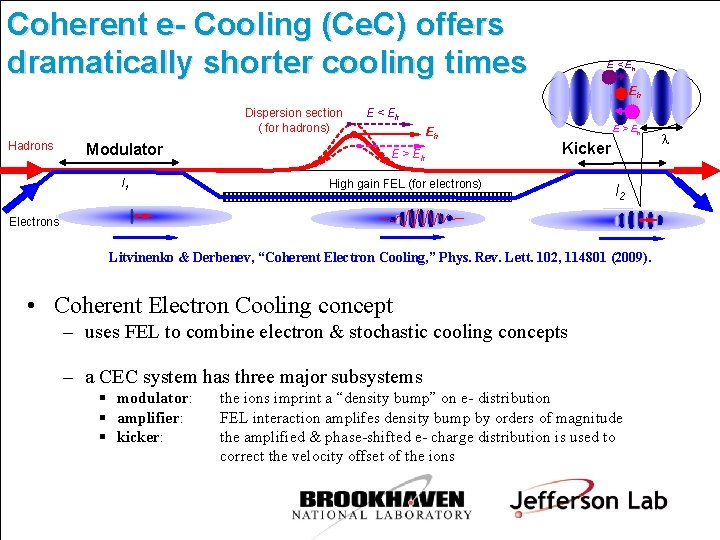
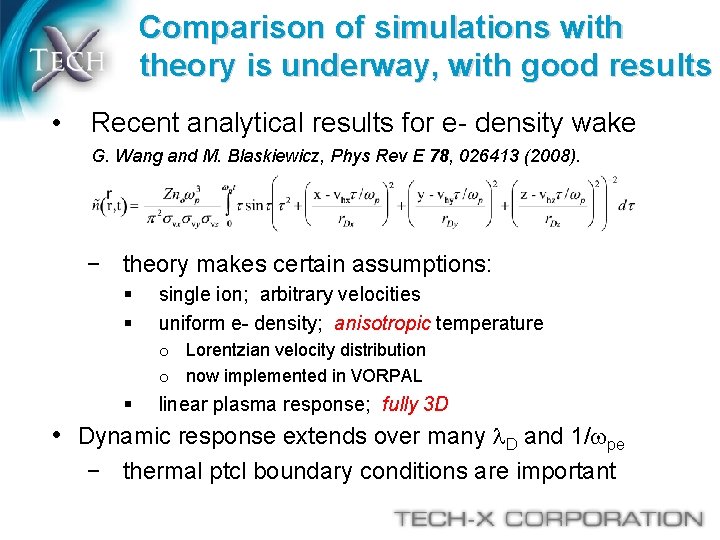
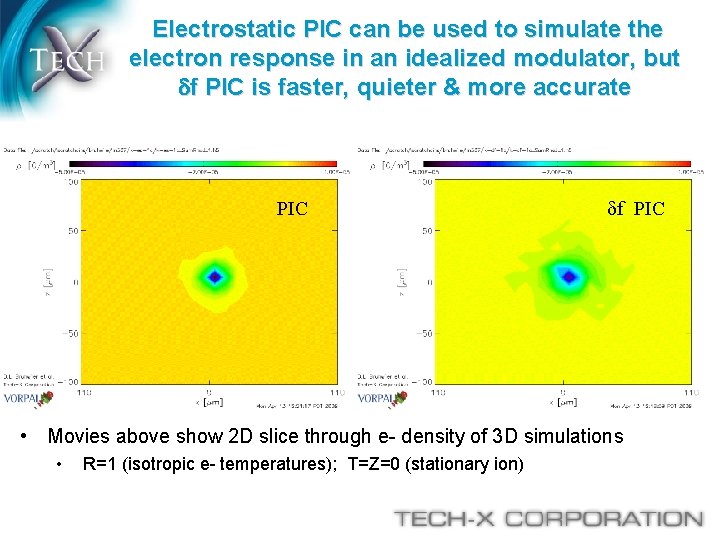
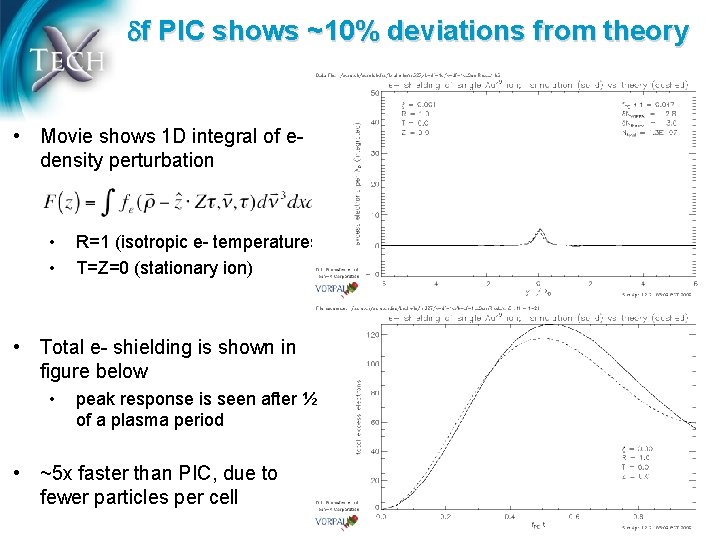
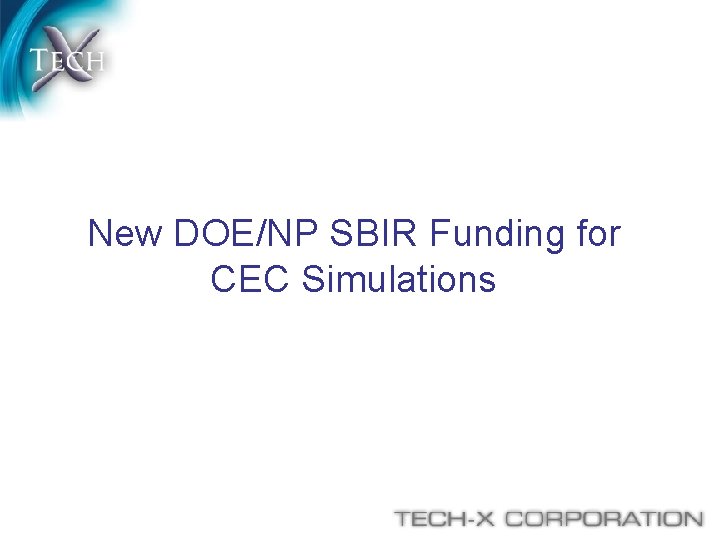
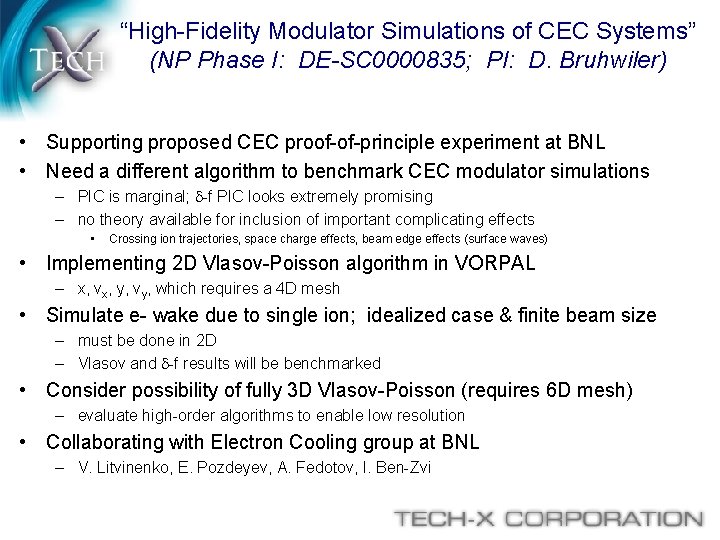
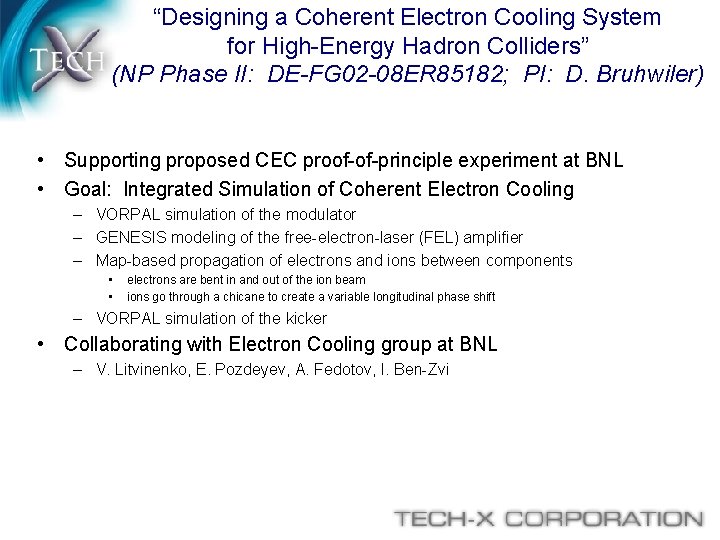
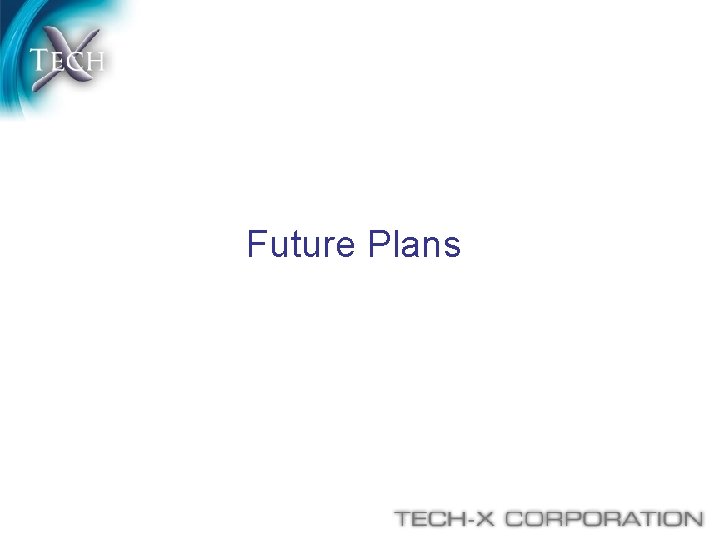
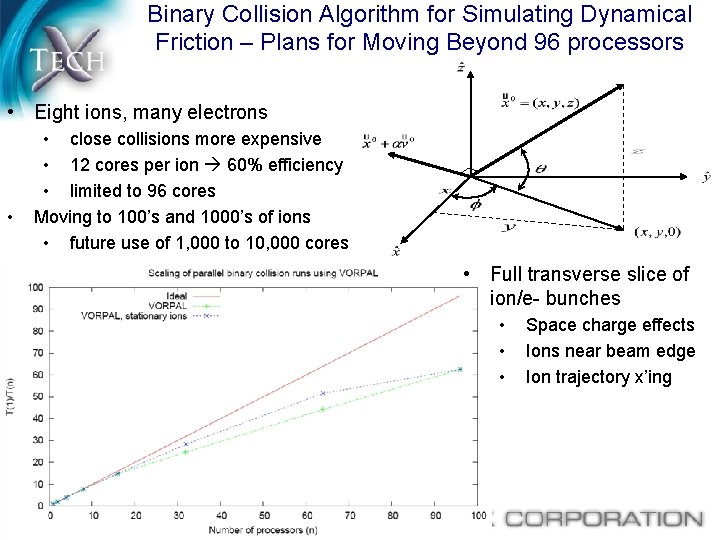
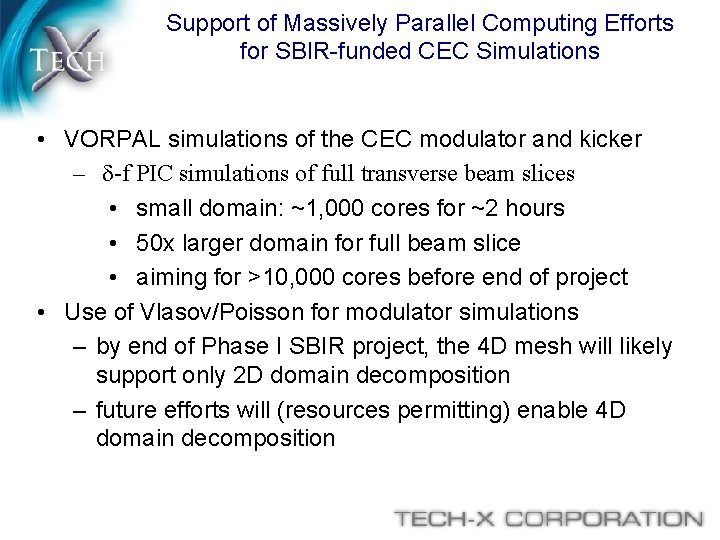
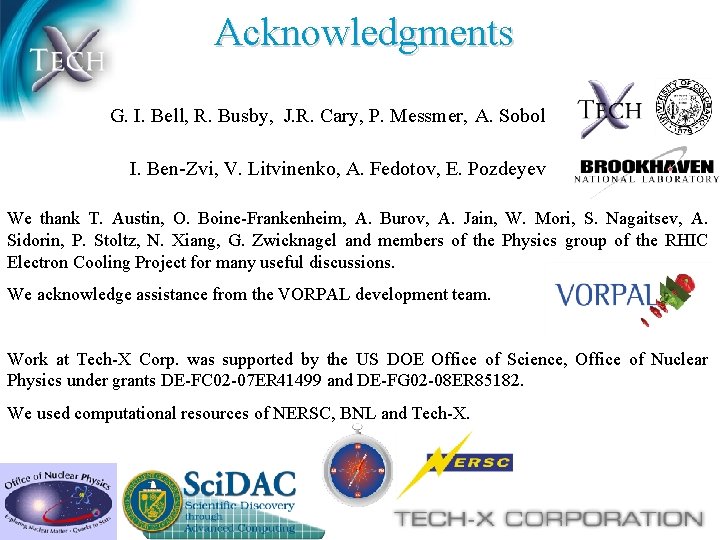
- Slides: 24
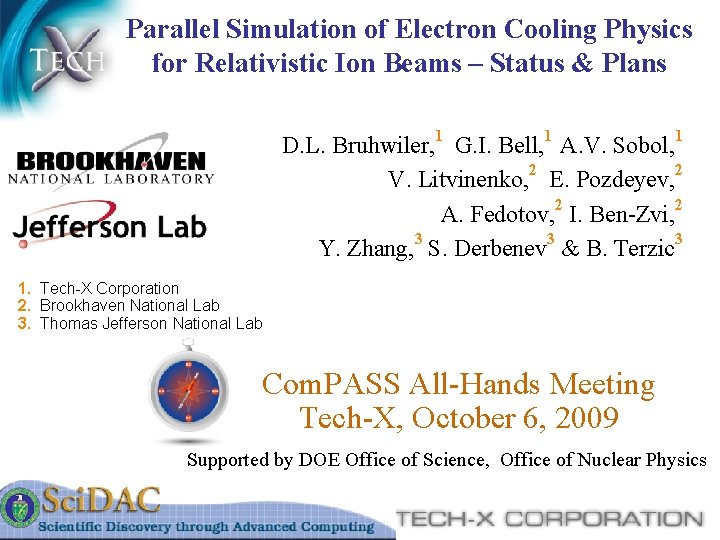
Parallel Simulation of Electron Cooling Physics for Relativistic Ion Beams – Status & Plans D. L. Bruhwiler, 1 G. I. Bell, 1 A. V. Sobol, 1 V. Litvinenko, 2 E. Pozdeyev, 2 A. Fedotov, 2 I. Ben-Zvi, 2 Y. Zhang, 3 S. Derbenev 3 & B. Terzic 3 1. Tech-X Corporation 2. Brookhaven National Lab 3. Thomas Jefferson National Lab Com. PASS All-Hands Meeting Tech-X, October 6, 2009 Supported by DOE Office of Science, Office of Nuclear Physics
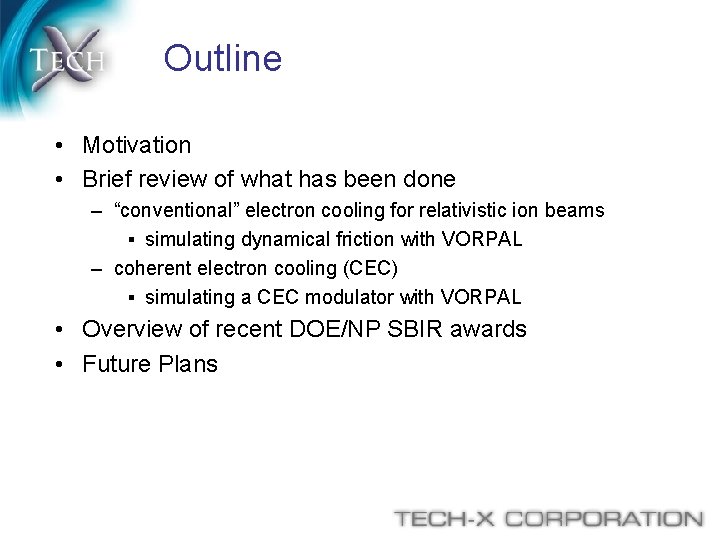
Outline • Motivation • Brief review of what has been done – “conventional” electron cooling for relativistic ion beams § simulating dynamical friction with VORPAL – coherent electron cooling (CEC) § simulating a CEC modulator with VORPAL • Overview of recent DOE/NP SBIR awards • Future Plans
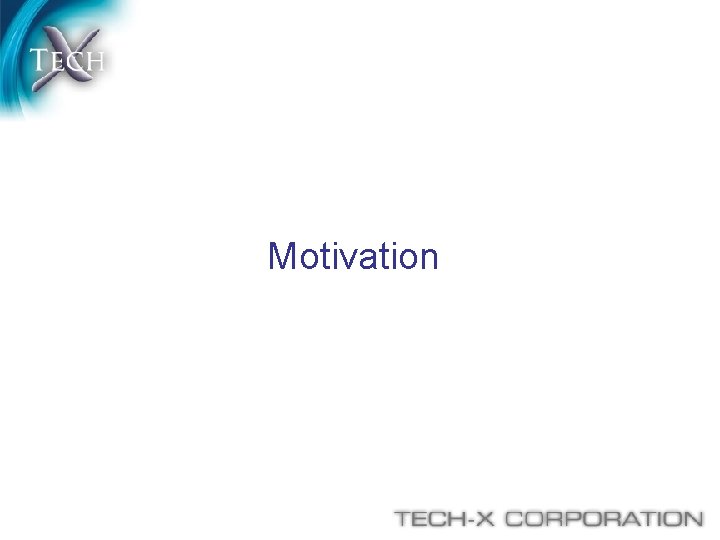
Motivation
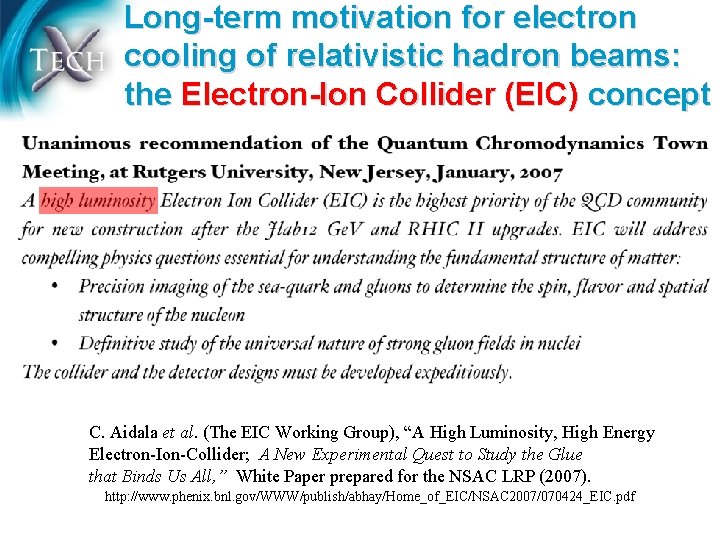
Long-term motivation for electron cooling of relativistic hadron beams: the Electron-Ion Collider (EIC) concept C. Aidala et al. (The EIC Working Group), “A High Luminosity, High Energy Electron-Ion-Collider; A New Experimental Quest to Study the Glue that Binds Us All, ” White Paper prepared for the NSAC LRP (2007). http: //www. phenix. bnl. gov/WWW/publish/abhay/Home_of_EIC/NSAC 2007/070424_EIC. pdf
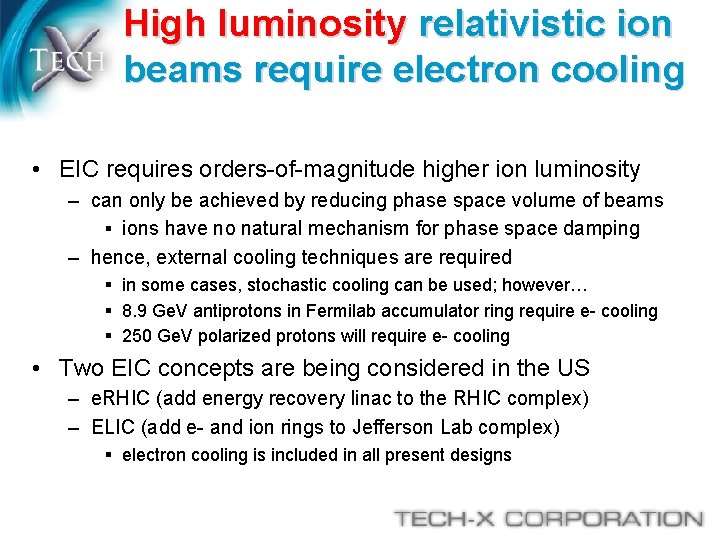
High luminosity relativistic ion beams require electron cooling • EIC requires orders-of-magnitude higher ion luminosity – can only be achieved by reducing phase space volume of beams § ions have no natural mechanism for phase space damping – hence, external cooling techniques are required § in some cases, stochastic cooling can be used; however… § 8. 9 Ge. V antiprotons in Fermilab accumulator ring require e- cooling § 250 Ge. V polarized protons will require e- cooling • Two EIC concepts are being considered in the US – e. RHIC (add energy recovery linac to the RHIC complex) – ELIC (add e- and ion rings to Jefferson Lab complex) § electron cooling is included in all present designs
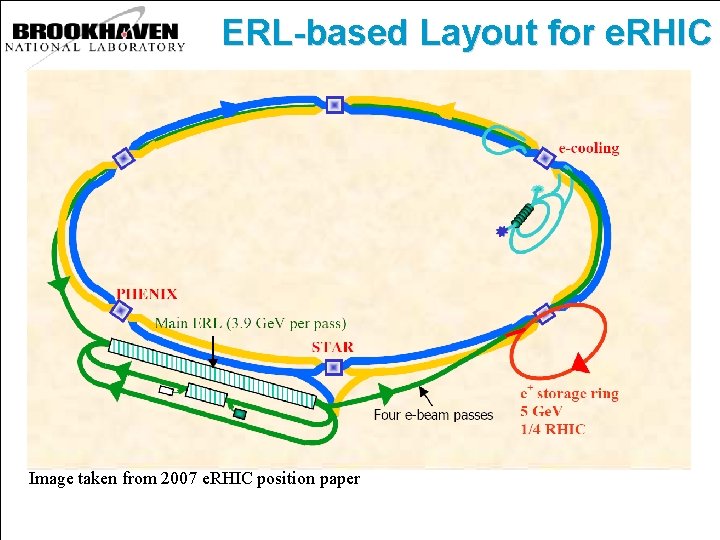
ERL-based Layout for e. RHIC Image taken from 2007 e. RHIC position paper
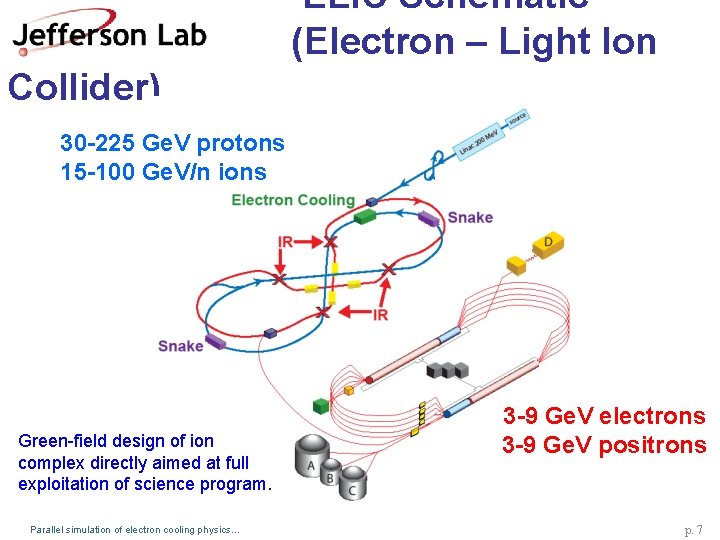
ELIC Schematic (Electron – Light Ion Collider) 30 -225 Ge. V protons 15 -100 Ge. V/n ions Green-field design of ion complex directly aimed at full exploitation of science program. Parallel simulation of electron cooling physics… 3 -9 Ge. V electrons 3 -9 Ge. V positrons p. 7
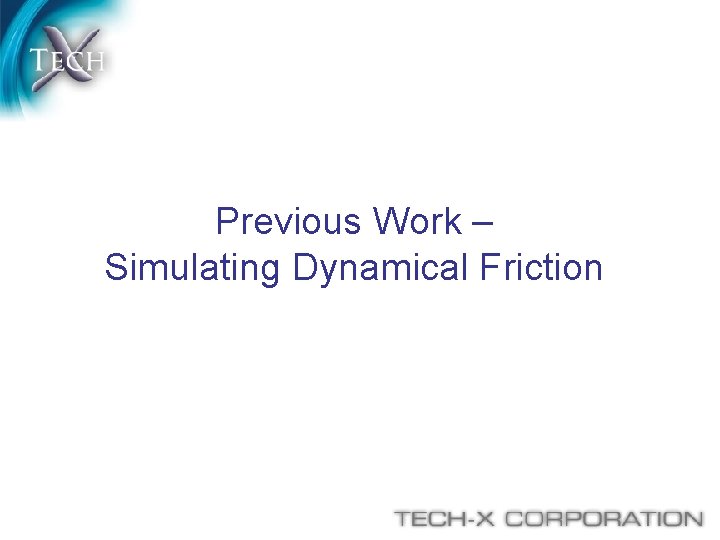
Previous Work – Simulating Dynamical Friction
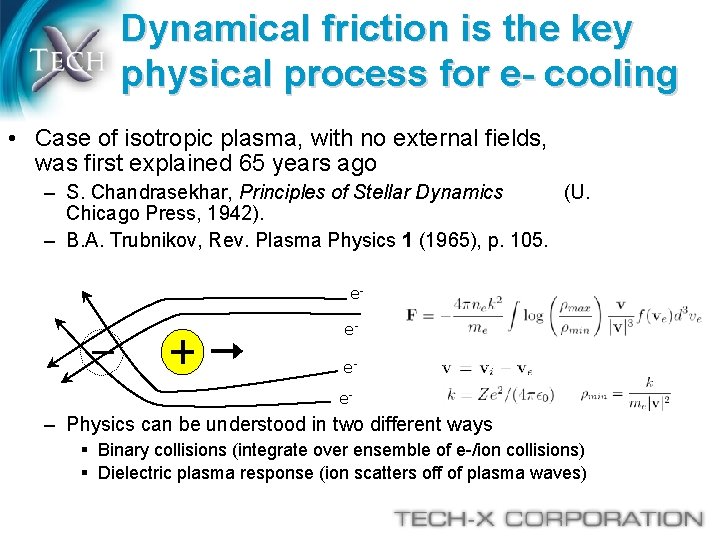
Dynamical friction is the key physical process for e- cooling • Case of isotropic plasma, with no external fields, was first explained 65 years ago – S. Chandrasekhar, Principles of Stellar Dynamics (U. Chicago Press, 1942). – B. A. Trubnikov, Rev. Plasma Physics 1 (1965), p. 105. eeee- – Physics can be understood in two different ways § Binary collisions (integrate over ensemble of e-/ion collisions) § Dielectric plasma response (ion scatters off of plasma waves)
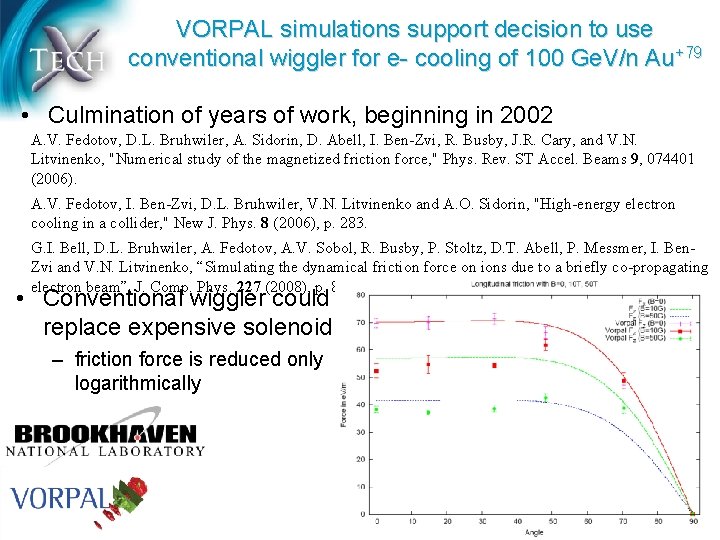
VORPAL simulations support decision to use conventional wiggler for e- cooling of 100 Ge. V/n Au+79 • Culmination of years of work, beginning in 2002 A. V. Fedotov, D. L. Bruhwiler, A. Sidorin, D. Abell, I. Ben-Zvi, R. Busby, J. R. Cary, and V. N. Litvinenko, "Numerical study of the magnetized friction force, " Phys. Rev. ST Accel. Beams 9, 074401 (2006). A. V. Fedotov, I. Ben-Zvi, D. L. Bruhwiler, V. N. Litvinenko and A. O. Sidorin, "High-energy electron cooling in a collider, " New J. Phys. 8 (2006), p. 283. G. I. Bell, D. L. Bruhwiler, A. Fedotov, A. V. Sobol, R. Busby, P. Stoltz, D. T. Abell, P. Messmer, I. Ben. Zvi and V. N. Litvinenko, “Simulating the dynamical friction force on ions due to a briefly co-propagating electron beam”, J. Comp. Phys. 227 (2008), p. 8714. • Conventional wiggler could replace expensive solenoid – friction force is reduced only logarithmically
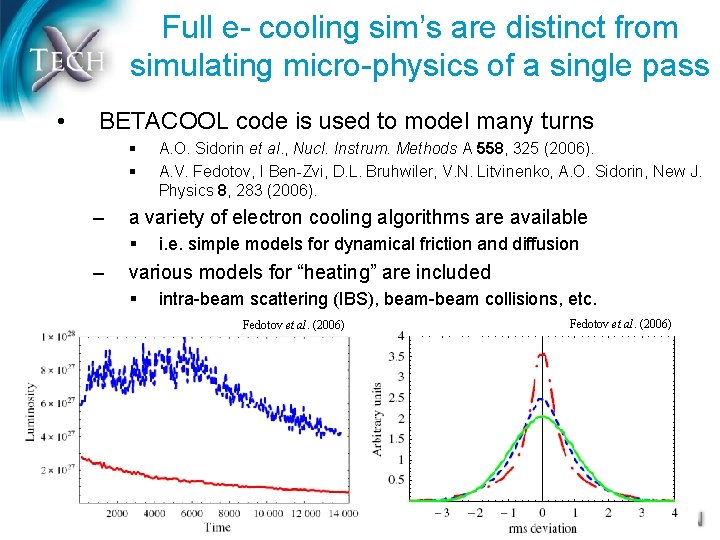
Full e- cooling sim’s are distinct from simulating micro-physics of a single pass • BETACOOL code is used to model many turns § § – a variety of electron cooling algorithms are available § – A. O. Sidorin et al. , Nucl. Instrum. Methods A 558, 325 (2006). A. V. Fedotov, I Ben-Zvi, D. L. Bruhwiler, V. N. Litvinenko, A. O. Sidorin, New J. Physics 8, 283 (2006). i. e. simple models for dynamical friction and diffusion various models for “heating” are included § intra-beam scattering (IBS), beam-beam collisions, etc. Fedotov et al. (2006)
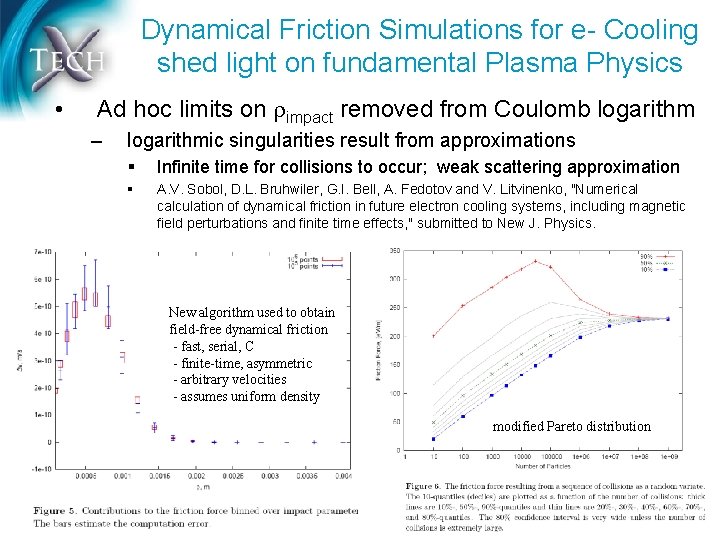
Dynamical Friction Simulations for e- Cooling shed light on fundamental Plasma Physics • Ad hoc limits on rimpact removed from Coulomb logarithm – logarithmic singularities result from approximations § Infinite time for collisions to occur; weak scattering approximation § A. V. Sobol, D. L. Bruhwiler, G. I. Bell, A. Fedotov and V. Litvinenko, "Numerical calculation of dynamical friction in future electron cooling systems, including magnetic field perturbations and finite time effects, " submitted to New J. Physics. New algorithm used to obtain field-free dynamical friction - fast, serial, C - finite-time, asymmetric - arbitrary velocities - assumes uniform density modified Pareto distribution
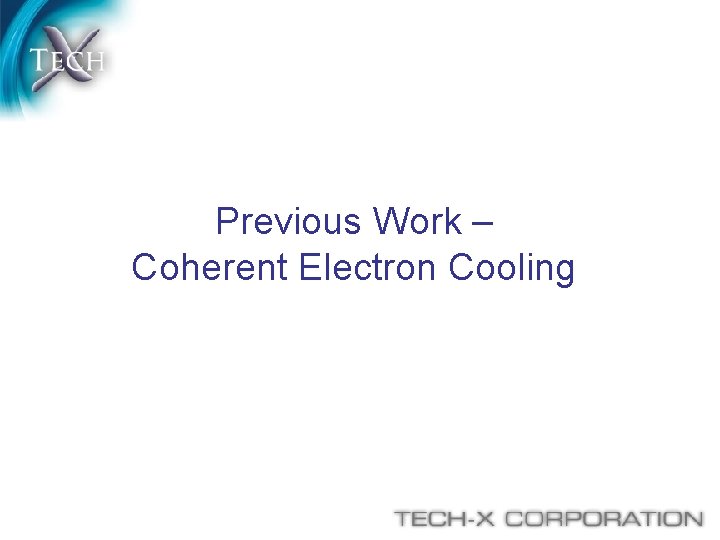
Previous Work – Coherent Electron Cooling
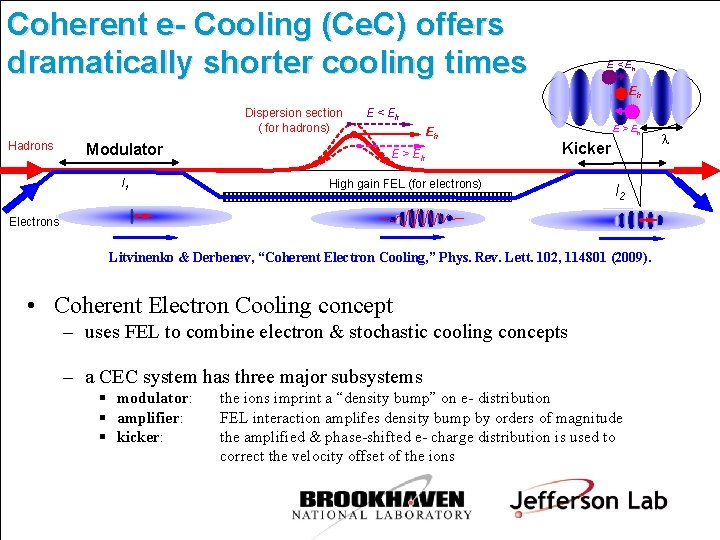
Coherent e- Cooling (Ce. C) offers dramatically shorter cooling times E < Eh Eh Dispersion section ( for hadrons) Hadrons E < Eh Eh Modulator E > Eh l 1 High gain FEL (for electrons) E > Eh Kicker l 2 Electrons Litvinenko & Derbenev, “Coherent Electron Cooling, ” Phys. Rev. Lett. 102, 114801 (2009). • Coherent Electron Cooling concept – uses FEL to combine electron & stochastic cooling concepts – a CEC system has three major subsystems § modulator: § amplifier: § kicker: the ions imprint a “density bump” on e- distribution FEL interaction amplifes density bump by orders of magnitude the amplified & phase-shifted e- charge distribution is used to correct the velocity offset of the ions
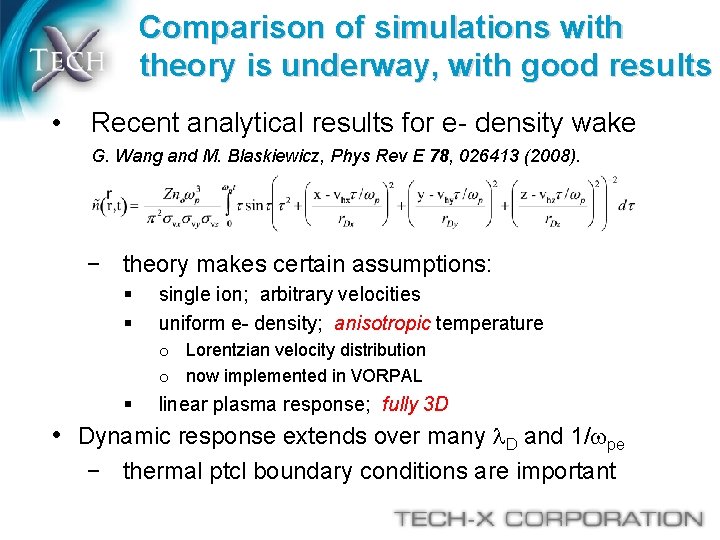
Comparison of simulations with theory is underway, with good results • Recent analytical results for e- density wake G. Wang and M. Blaskiewicz, Phys Rev E 78, 026413 (2008). − theory makes certain assumptions: § § single ion; arbitrary velocities uniform e- density; anisotropic temperature o Lorentzian velocity distribution o now implemented in VORPAL § linear plasma response; fully 3 D • Dynamic response extends over many D and 1/wpe − thermal ptcl boundary conditions are important
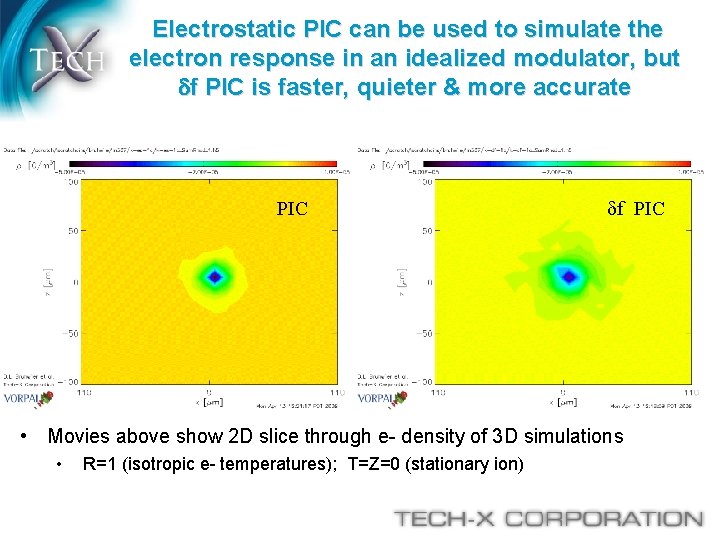
Electrostatic PIC can be used to simulate the electron response in an idealized modulator, but df PIC is faster, quieter & more accurate PIC df PIC • Movies above show 2 D slice through e- density of 3 D simulations • R=1 (isotropic e- temperatures); T=Z=0 (stationary ion)
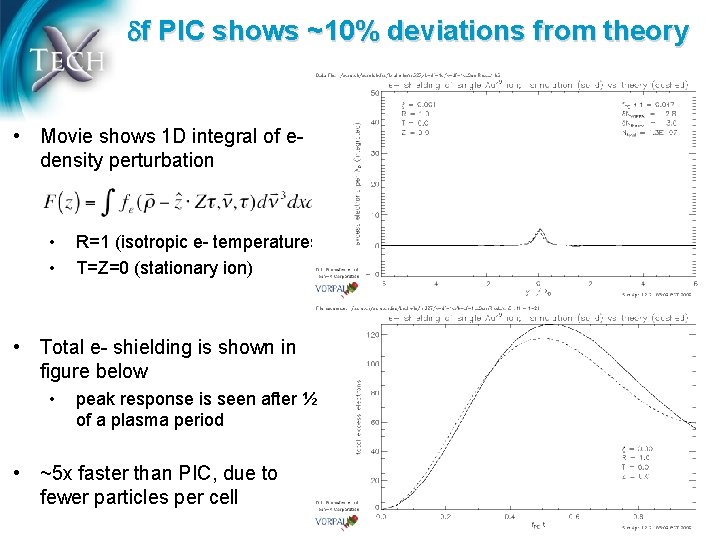
df PIC shows ~10% deviations from theory • Movie shows 1 D integral of edensity perturbation • • R=1 (isotropic e- temperatures) T=Z=0 (stationary ion) • Total e- shielding is shown in figure below • peak response is seen after ½ of a plasma period • ~5 x faster than PIC, due to fewer particles per cell
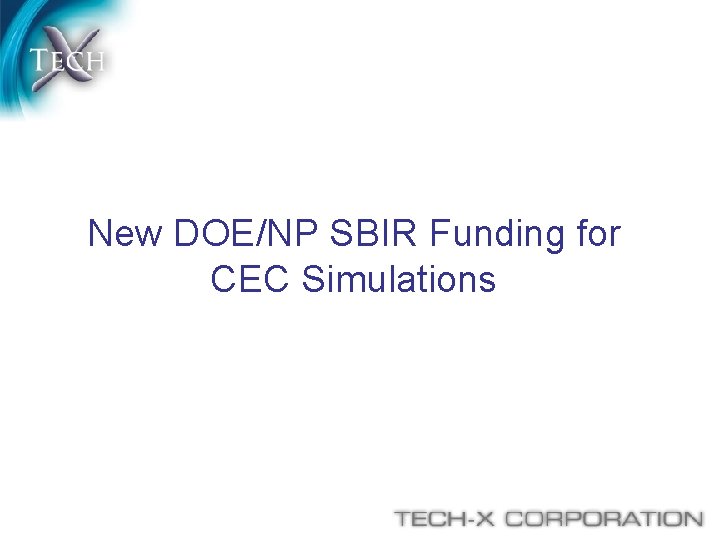
New DOE/NP SBIR Funding for CEC Simulations
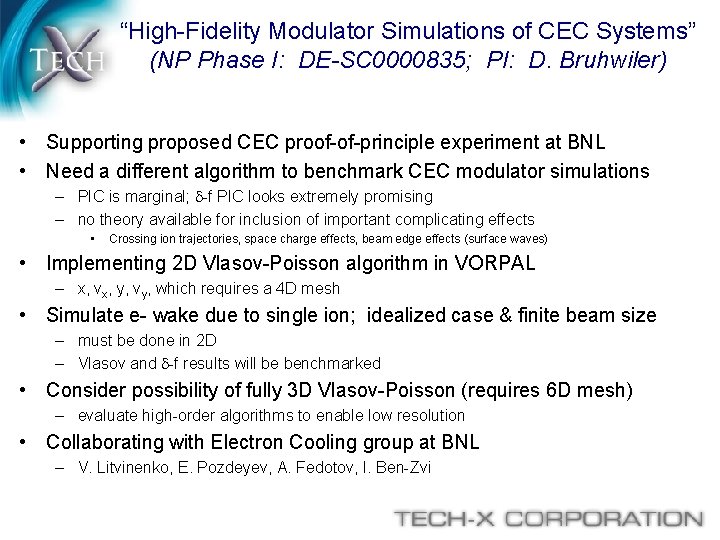
“High-Fidelity Modulator Simulations of CEC Systems” (NP Phase I: DE-SC 0000835; PI: D. Bruhwiler) • Supporting proposed CEC proof-of-principle experiment at BNL • Need a different algorithm to benchmark CEC modulator simulations – PIC is marginal; d-f PIC looks extremely promising – no theory available for inclusion of important complicating effects • Crossing ion trajectories, space charge effects, beam edge effects (surface waves) • Implementing 2 D Vlasov-Poisson algorithm in VORPAL – x, vx, y, vy, which requires a 4 D mesh • Simulate e- wake due to single ion; idealized case & finite beam size – must be done in 2 D – Vlasov and d-f results will be benchmarked • Consider possibility of fully 3 D Vlasov-Poisson (requires 6 D mesh) – evaluate high-order algorithms to enable low resolution • Collaborating with Electron Cooling group at BNL – V. Litvinenko, E. Pozdeyev, A. Fedotov, I. Ben-Zvi
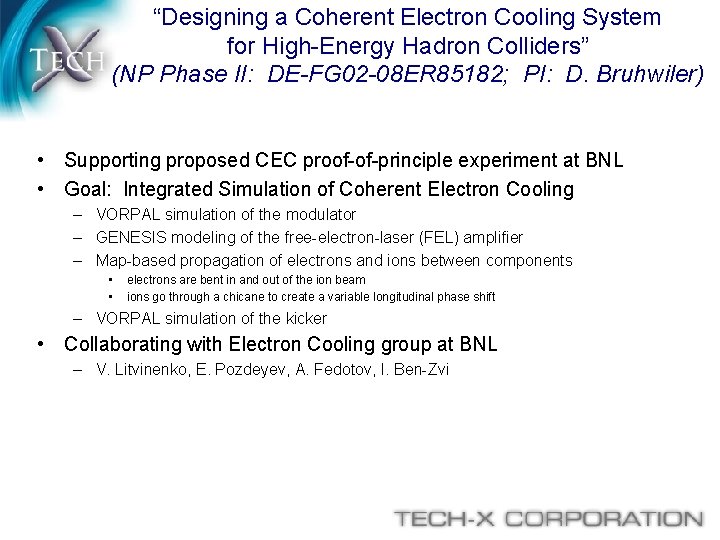
“Designing a Coherent Electron Cooling System for High-Energy Hadron Colliders” (NP Phase II: DE-FG 02 -08 ER 85182; PI: D. Bruhwiler) • Supporting proposed CEC proof-of-principle experiment at BNL • Goal: Integrated Simulation of Coherent Electron Cooling – VORPAL simulation of the modulator – GENESIS modeling of the free-electron-laser (FEL) amplifier – Map-based propagation of electrons and ions between components • • electrons are bent in and out of the ion beam ions go through a chicane to create a variable longitudinal phase shift – VORPAL simulation of the kicker • Collaborating with Electron Cooling group at BNL – V. Litvinenko, E. Pozdeyev, A. Fedotov, I. Ben-Zvi
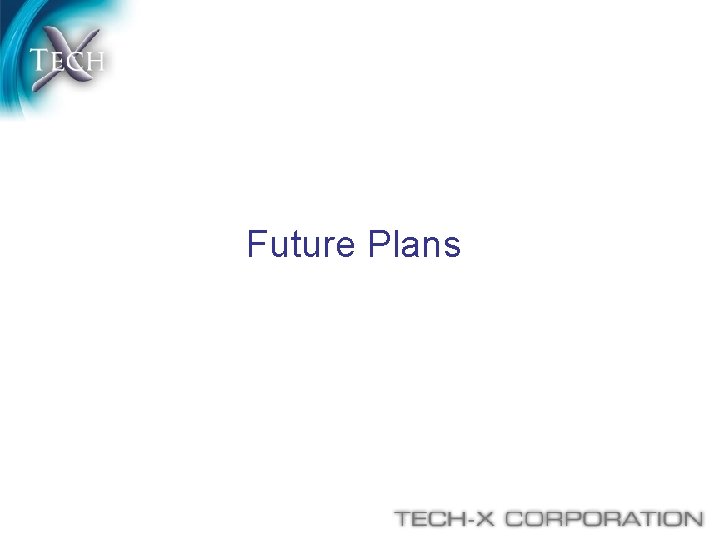
Future Plans
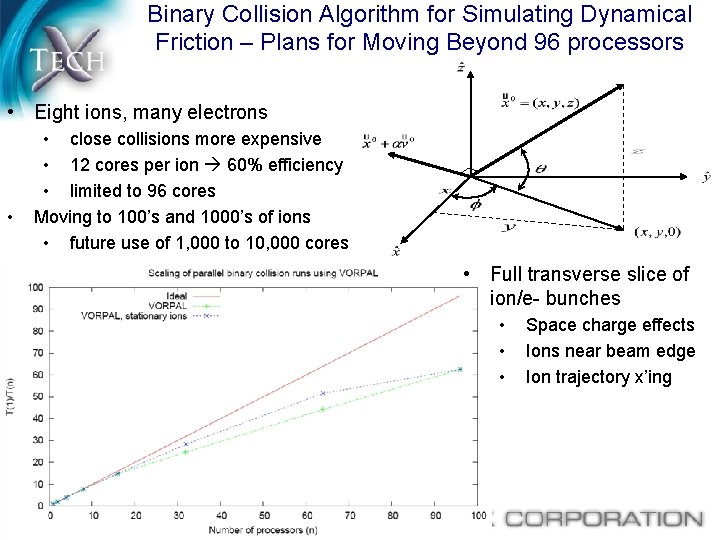
Binary Collision Algorithm for Simulating Dynamical Friction – Plans for Moving Beyond 96 processors • Eight ions, many electrons • • close collisions more expensive • 12 cores per ion 60% efficiency • limited to 96 cores Moving to 100’s and 1000’s of ions • future use of 1, 000 to 10, 000 cores • Full transverse slice of ion/e- bunches • • • Space charge effects Ions near beam edge Ion trajectory x’ing
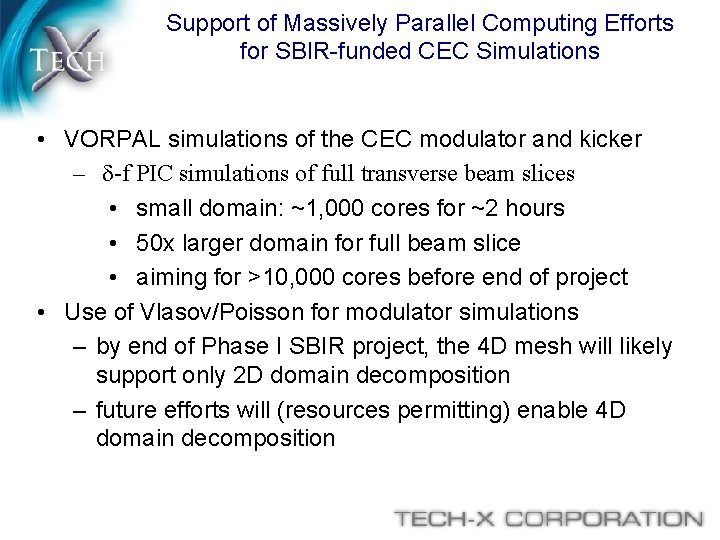
Support of Massively Parallel Computing Efforts for SBIR-funded CEC Simulations • VORPAL simulations of the CEC modulator and kicker – d-f PIC simulations of full transverse beam slices • small domain: ~1, 000 cores for ~2 hours • 50 x larger domain for full beam slice • aiming for >10, 000 cores before end of project • Use of Vlasov/Poisson for modulator simulations – by end of Phase I SBIR project, the 4 D mesh will likely support only 2 D domain decomposition – future efforts will (resources permitting) enable 4 D domain decomposition
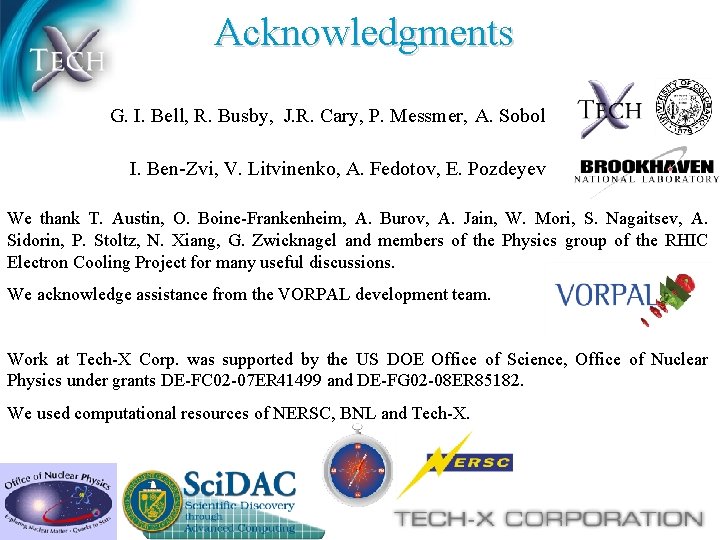
Acknowledgments G. I. Bell, R. Busby, J. R. Cary, P. Messmer, A. Sobol I. Ben-Zvi, V. Litvinenko, A. Fedotov, E. Pozdeyev We thank T. Austin, O. Boine-Frankenheim, A. Burov, A. Jain, W. Mori, S. Nagaitsev, A. Sidorin, P. Stoltz, N. Xiang, G. Zwicknagel and members of the Physics group of the RHIC Electron Cooling Project for many useful discussions. We acknowledge assistance from the VORPAL development team. Work at Tech-X Corp. was supported by the US DOE Office of Science, Office of Nuclear Physics under grants DE-FC 02 -07 ER 41499 and DE-FG 02 -08 ER 85182. We used computational resources of NERSC, BNL and Tech-X.
Specific heat capacity
Relativistic circular motion
Relativistic thinking example
Michelson morley experiment
Bertrand postulate
General relativity equation
Relativistic momentum
Relativistic thinking example
Relativistic mass formula
Labouvie-vief pragmatic thought
Relativistic kinetic energy
Relativistic kinetic energy
Relativity
Relative speed of approach
Clairaut equation
A physical education chapter 28
Cognitive development early adulthood
Relativistic mean field theory
Doppler effect animation ppt
Relativistic momentum
Ib physics doc
Concezio bozzi
Parallel and distributed simulation systems
Manual auditing and computerized auditing
Parallel discrete event simulation