New Methods for Precision Mller Polarimetry Dave Mack
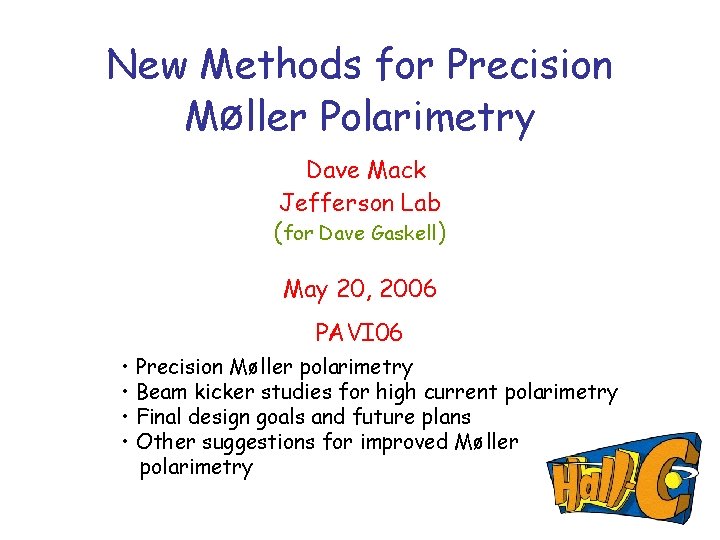
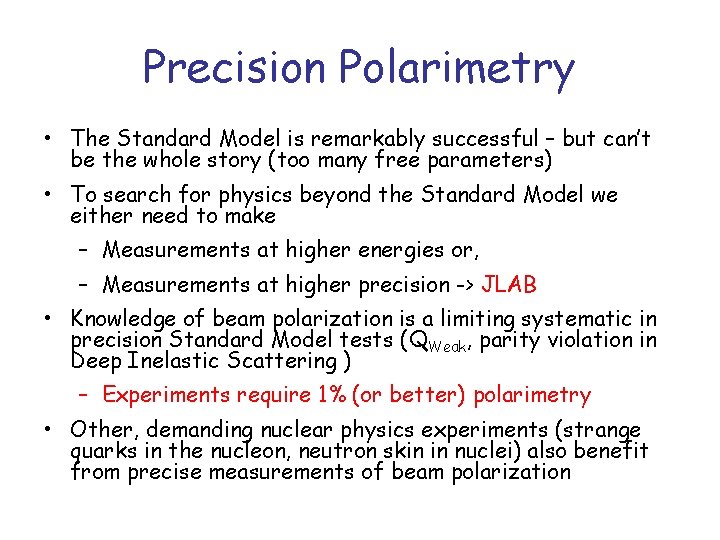
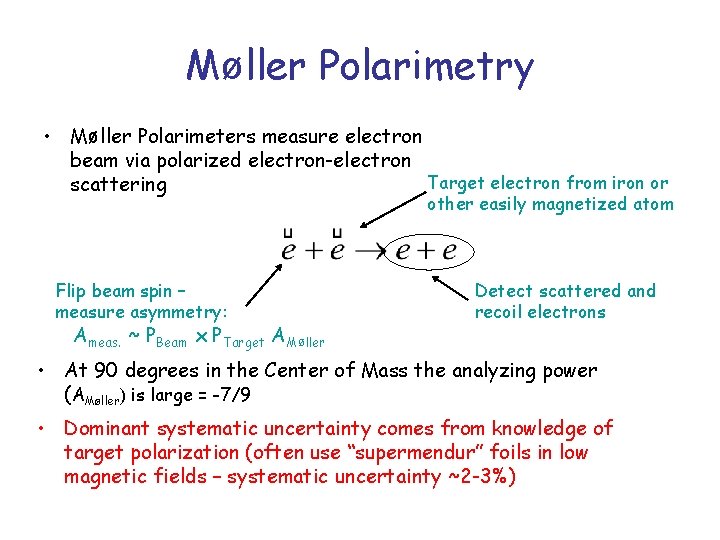
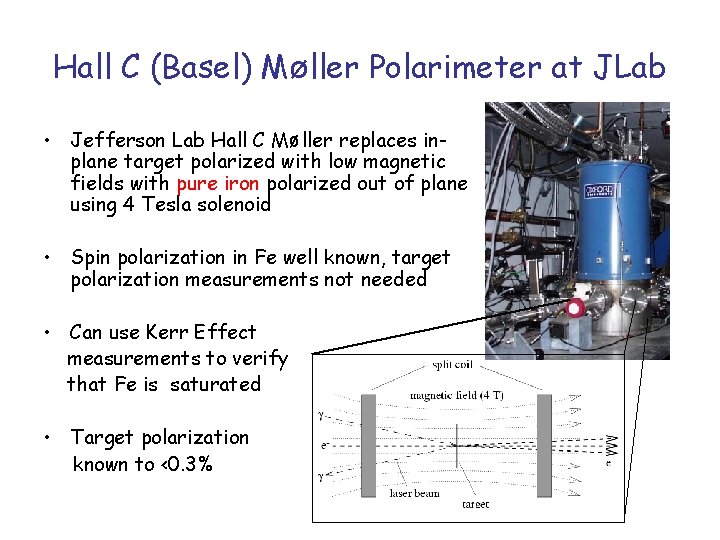
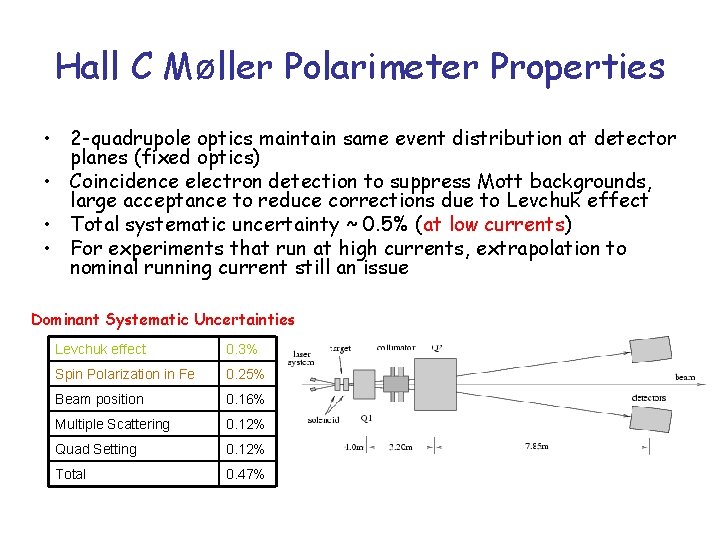
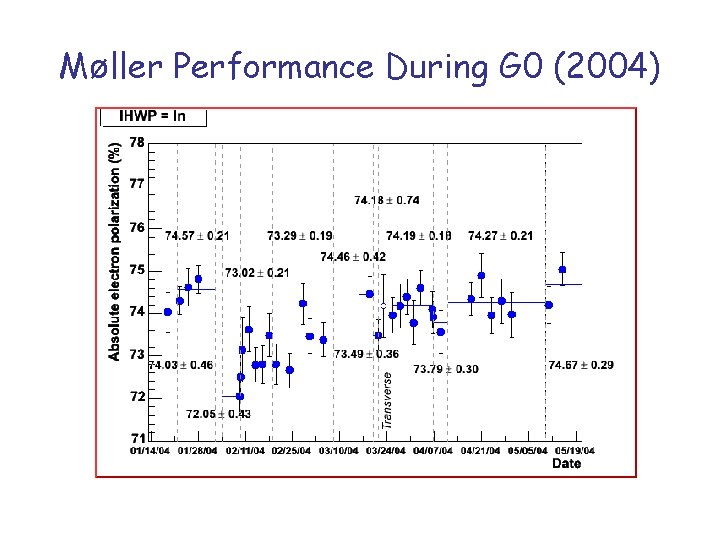
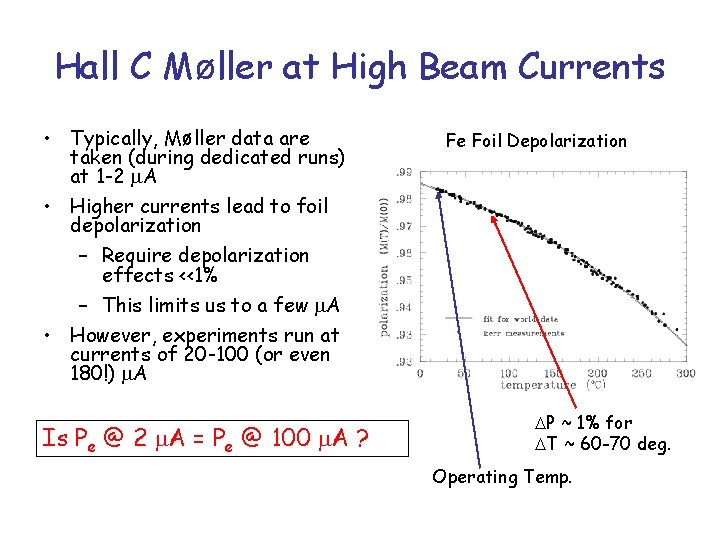
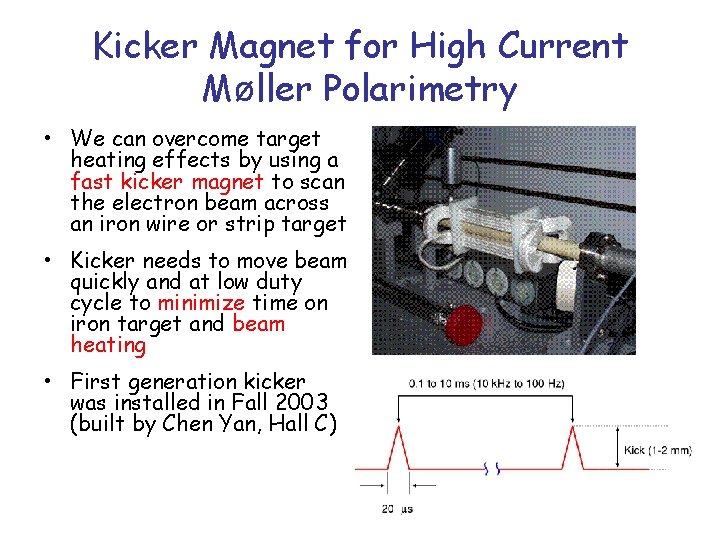
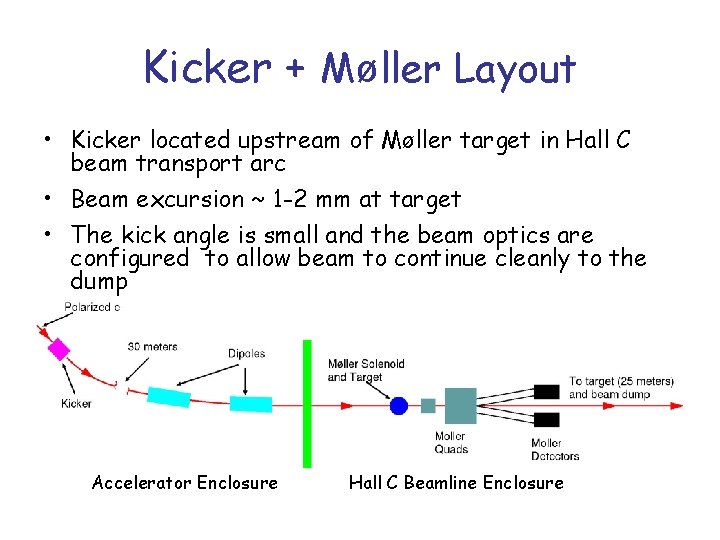
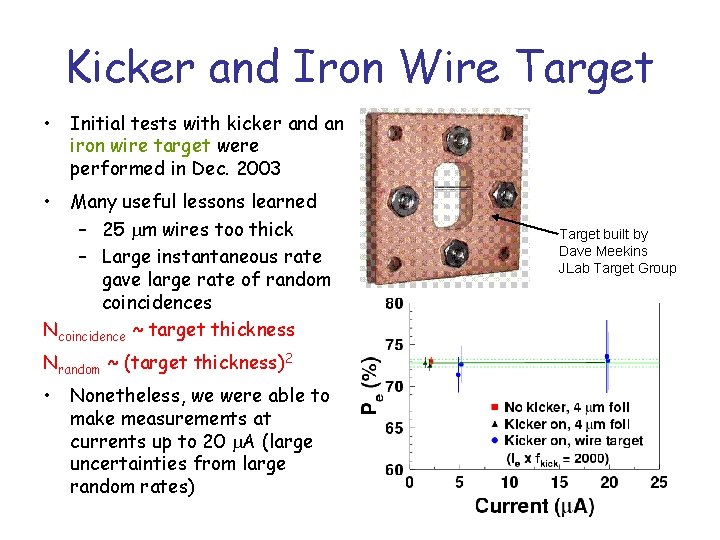
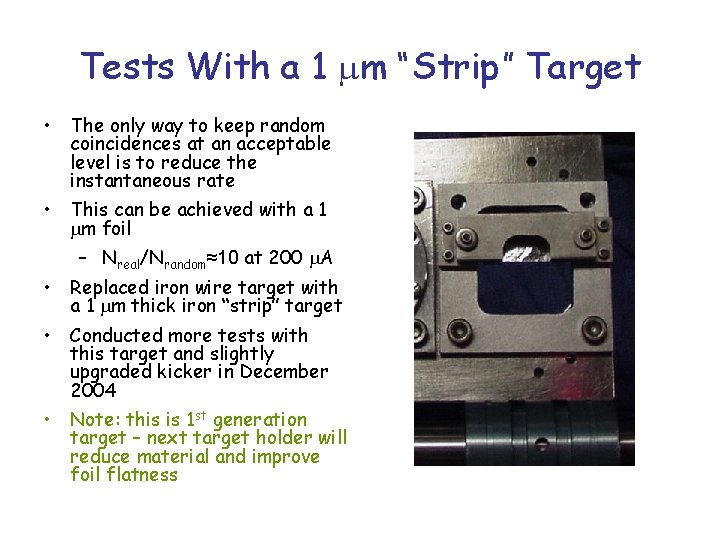
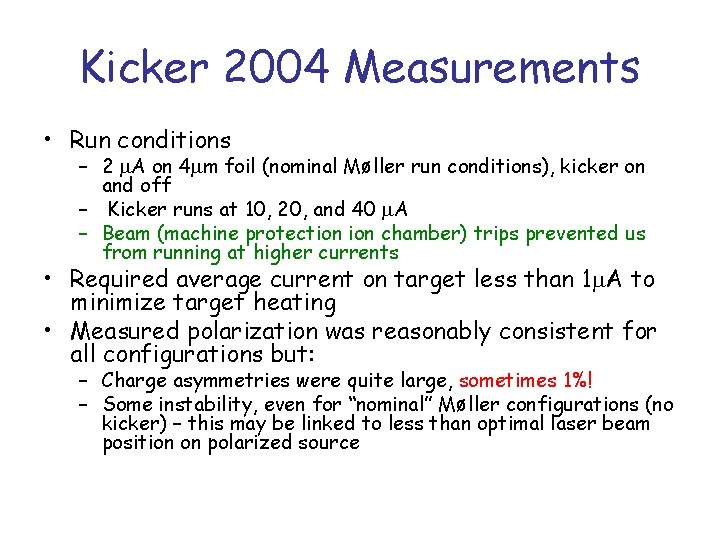
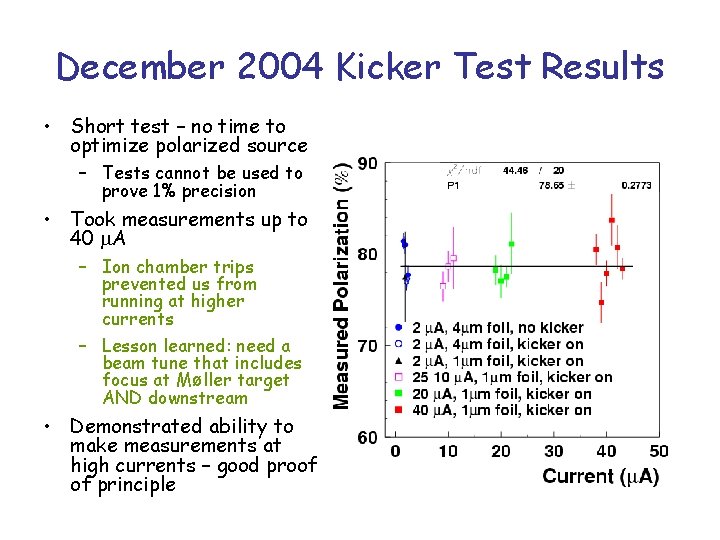
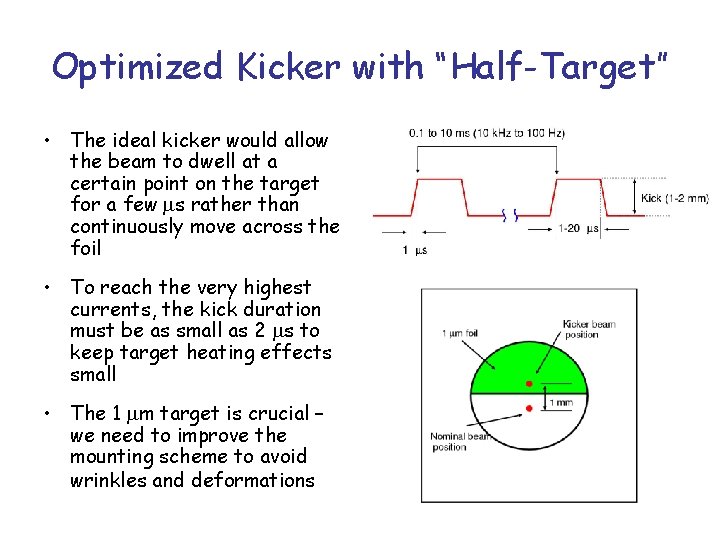
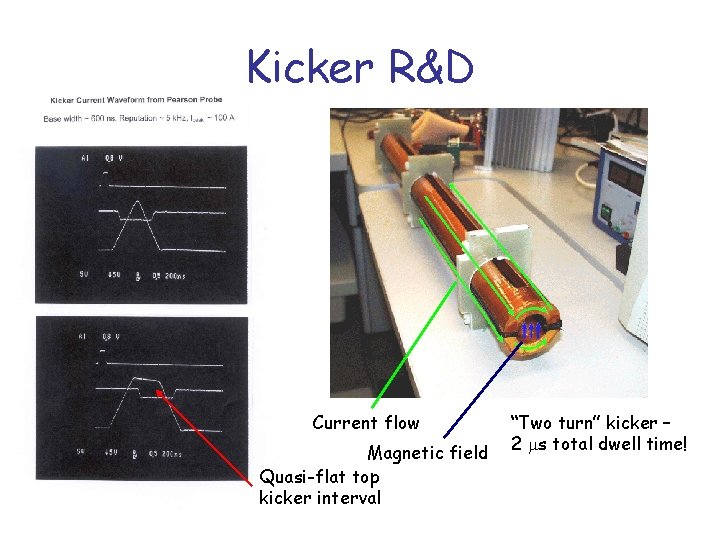
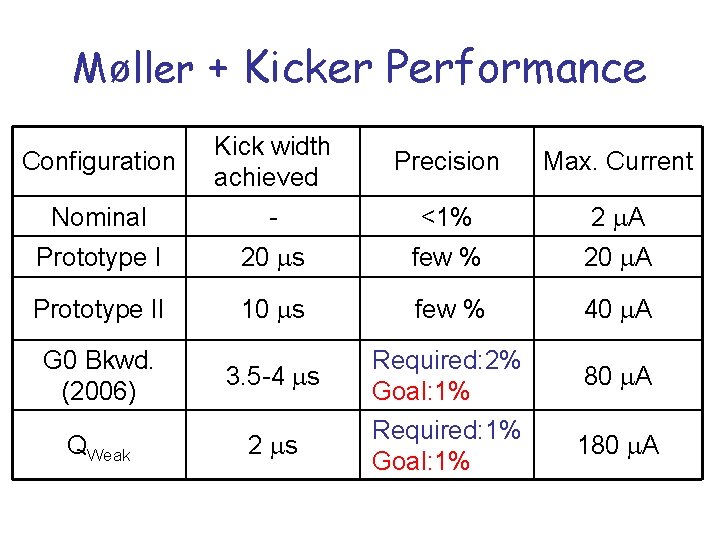
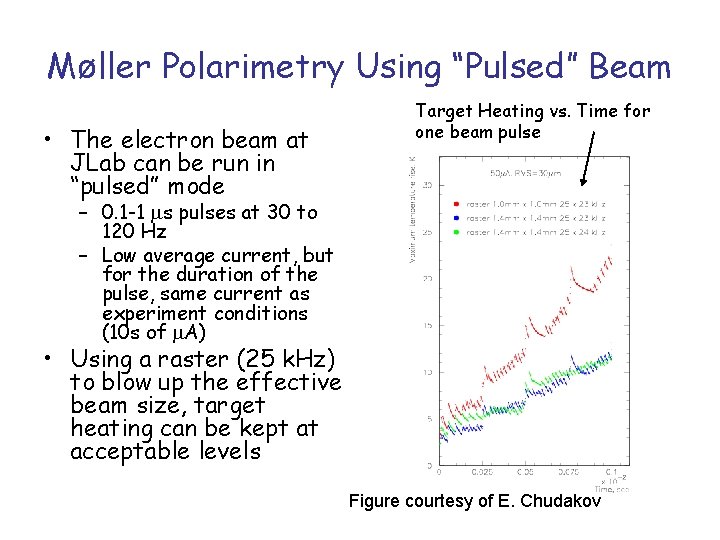
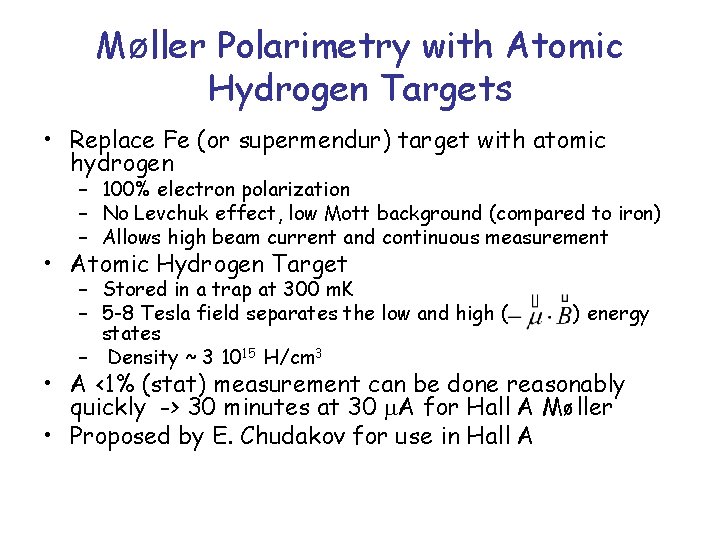
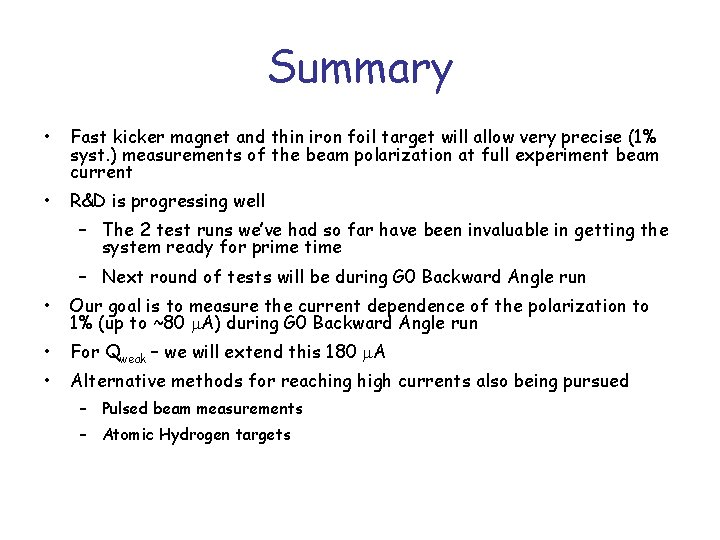
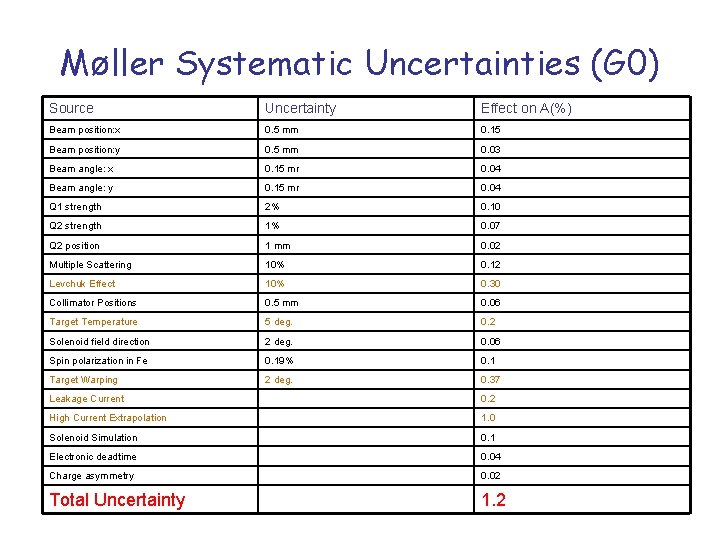
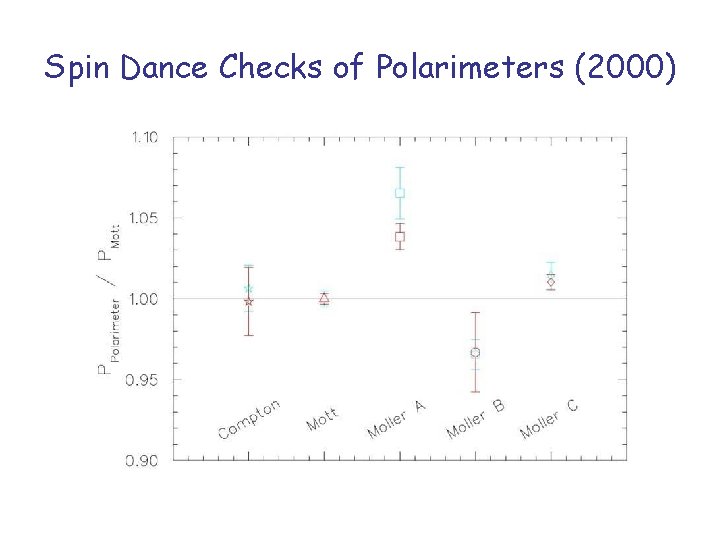
- Slides: 21
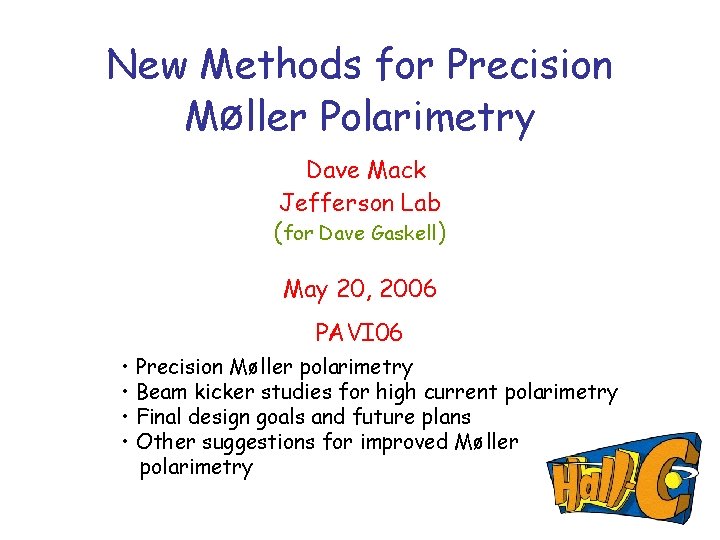
New Methods for Precision Møller Polarimetry Dave Mack Jefferson Lab (for Dave Gaskell) May 20, 2006 PAVI 06 • Precision Møller polarimetry • Beam kicker studies for high current polarimetry • Final design goals and future plans • Other suggestions for improved Møller polarimetry
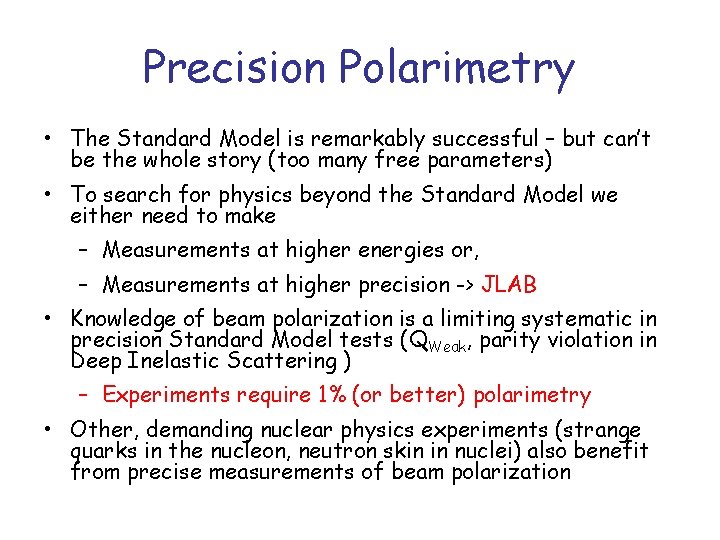
Precision Polarimetry • The Standard Model is remarkably successful – but can’t be the whole story (too many free parameters) • To search for physics beyond the Standard Model we either need to make – Measurements at higher energies or, – Measurements at higher precision -> JLAB • Knowledge of beam polarization is a limiting systematic in precision Standard Model tests (QWeak, parity violation in Deep Inelastic Scattering ) – Experiments require 1% (or better) polarimetry • Other, demanding nuclear physics experiments (strange quarks in the nucleon, neutron skin in nuclei) also benefit from precise measurements of beam polarization
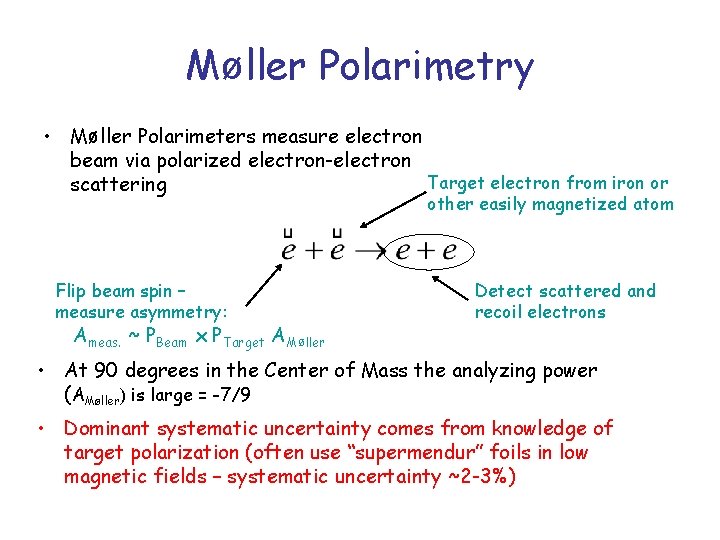
Møller Polarimetry • Møller Polarimeters measure electron beam via polarized electron-electron Target electron from iron or scattering other easily magnetized atom Flip beam spin – measure asymmetry: Ameas. ~ PBeam x PTarget AMøller Detect scattered and recoil electrons • At 90 degrees in the Center of Mass the analyzing power (AMøller) is large = -7/9 • Dominant systematic uncertainty comes from knowledge of target polarization (often use “supermendur” foils in low magnetic fields – systematic uncertainty ~2 -3%)
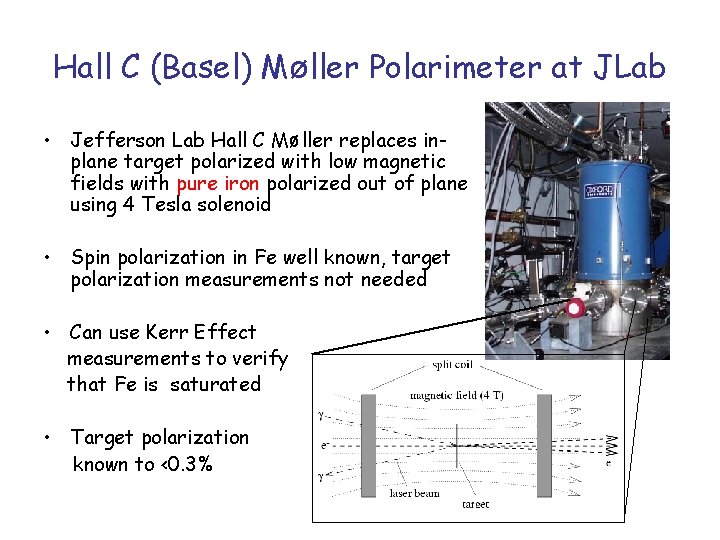
Hall C (Basel) Møller Polarimeter at JLab • Jefferson Lab Hall C Møller replaces inplane target polarized with low magnetic fields with pure iron polarized out of plane using 4 Tesla solenoid • Spin polarization in Fe well known, target polarization measurements not needed • Can use Kerr Effect measurements to verify that Fe is saturated • Target polarization known to <0. 3%
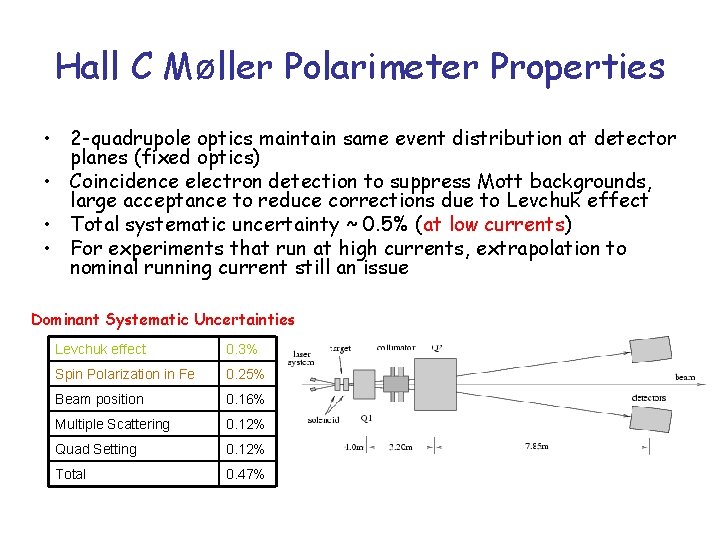
Hall C Møller Polarimeter Properties • 2 -quadrupole optics maintain same event distribution at detector planes (fixed optics) • Coincidence electron detection to suppress Mott backgrounds, large acceptance to reduce corrections due to Levchuk effect • Total systematic uncertainty ~ 0. 5% (at low currents) • For experiments that run at high currents, extrapolation to nominal running current still an issue Dominant Systematic Uncertainties Levchuk effect 0. 3% Spin Polarization in Fe 0. 25% Beam position 0. 16% Multiple Scattering 0. 12% Quad Setting 0. 12% Total 0. 47%
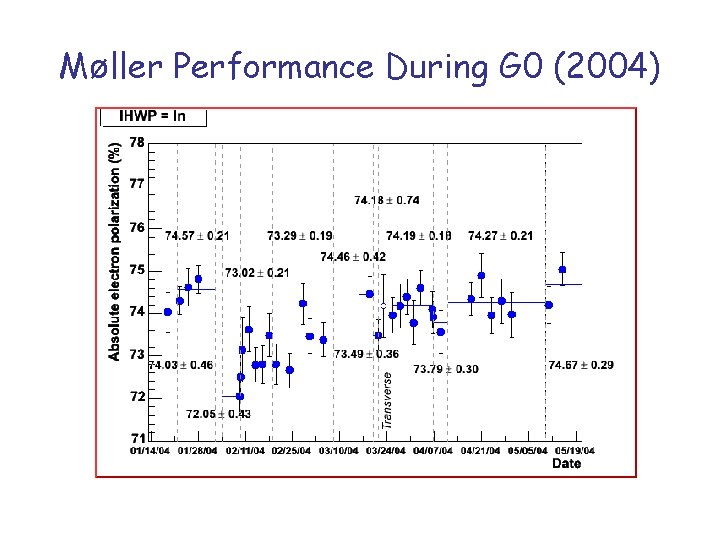
Møller Performance During G 0 (2004)
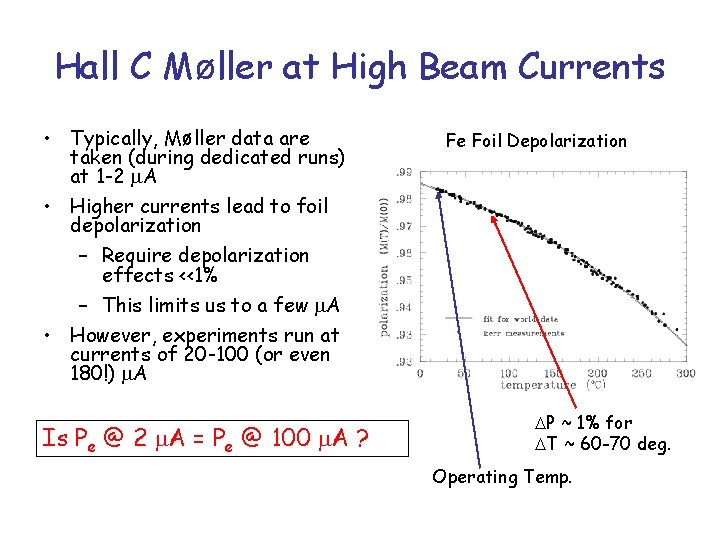
Hall C Møller at High Beam Currents • Typically, Møller data are taken (during dedicated runs) at 1 -2 m. A • Higher currents lead to foil depolarization – Require depolarization effects <<1% – This limits us to a few m. A • However, experiments run at currents of 20 -100 (or even 180!) m. A Is Pe @ 2 m. A = Pe @ 100 m. A ? Fe Foil Depolarization DP ~ 1% for DT ~ 60 -70 deg. Operating Temp.
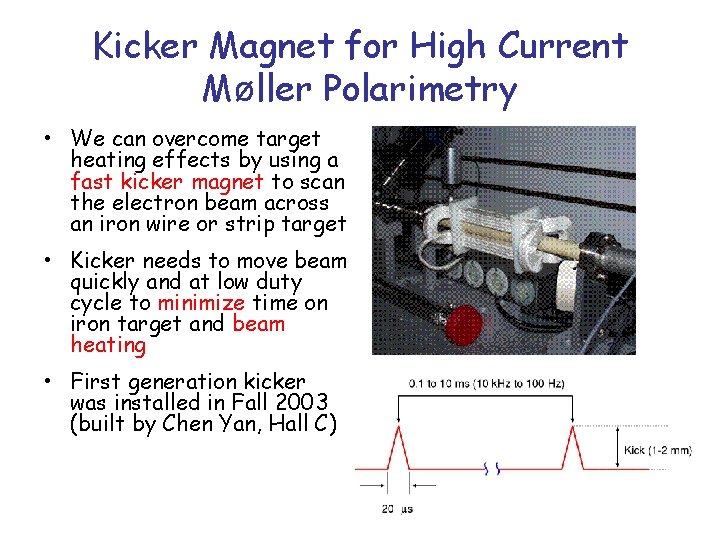
Kicker Magnet for High Current Møller Polarimetry • We can overcome target heating effects by using a fast kicker magnet to scan the electron beam across an iron wire or strip target • Kicker needs to move beam quickly and at low duty cycle to minimize time on iron target and beam heating • First generation kicker was installed in Fall 2003 (built by Chen Yan, Hall C)
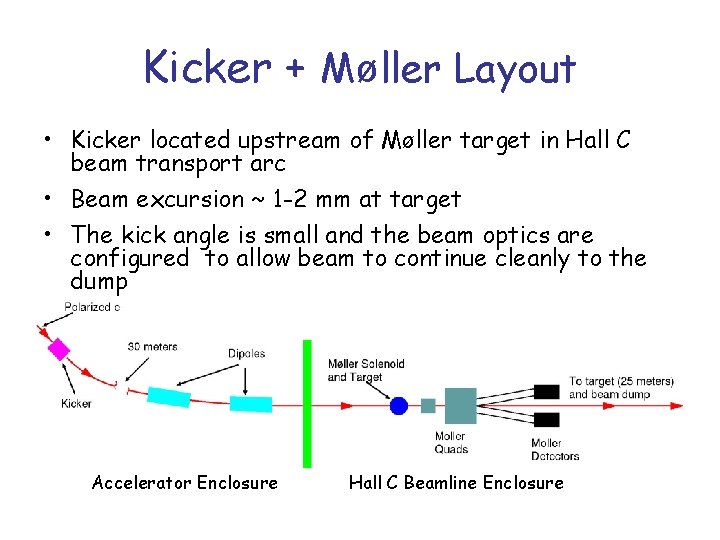
Kicker + Møller Layout • Kicker located upstream of Møller target in Hall C beam transport arc • Beam excursion ~ 1 -2 mm at target • The kick angle is small and the beam optics are configured to allow beam to continue cleanly to the dump Accelerator Enclosure Hall C Beamline Enclosure
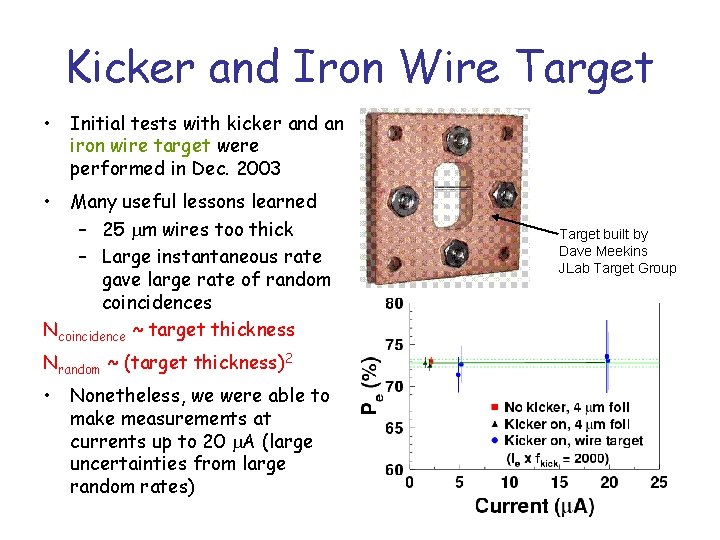
Kicker and Iron Wire Target • Initial tests with kicker and an iron wire target were performed in Dec. 2003 • Many useful lessons learned – 25 mm wires too thick – Large instantaneous rate gave large rate of random coincidences Ncoincidence ~ target thickness Nrandom ~ (target thickness)2 • Nonetheless, we were able to make measurements at currents up to 20 m. A (large uncertainties from large random rates) Target built by Dave Meekins JLab Target Group
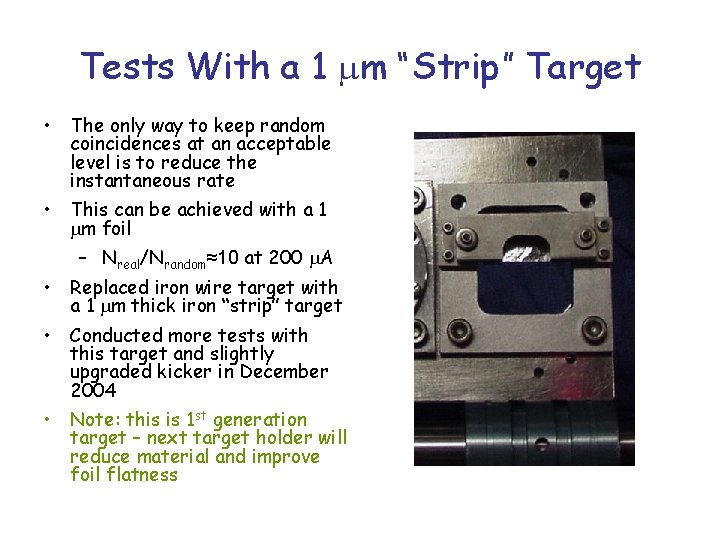
Tests With a 1 mm “Strip” Target • The only way to keep random coincidences at an acceptable level is to reduce the instantaneous rate • This can be achieved with a 1 mm foil – Nreal/Nrandom≈10 at 200 m. A • Replaced iron wire target with a 1 mm thick iron “strip” target • Conducted more tests with this target and slightly upgraded kicker in December 2004 • Note: this is 1 st generation target – next target holder will reduce material and improve foil flatness
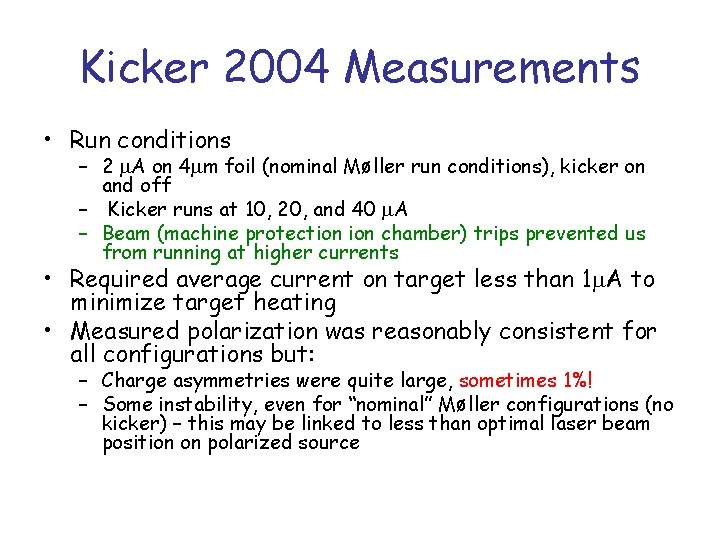
Kicker 2004 Measurements • Run conditions – 2 m. A on 4 mm foil (nominal Møller run conditions), kicker on and off – Kicker runs at 10, 20, and 40 m. A – Beam (machine protection chamber) trips prevented us from running at higher currents • Required average current on target less than 1 m. A to minimize target heating • Measured polarization was reasonably consistent for all configurations but: – Charge asymmetries were quite large, sometimes 1%! – Some instability, even for “nominal” Møller configurations (no kicker) – this may be linked to less than optimal laser beam position on polarized source
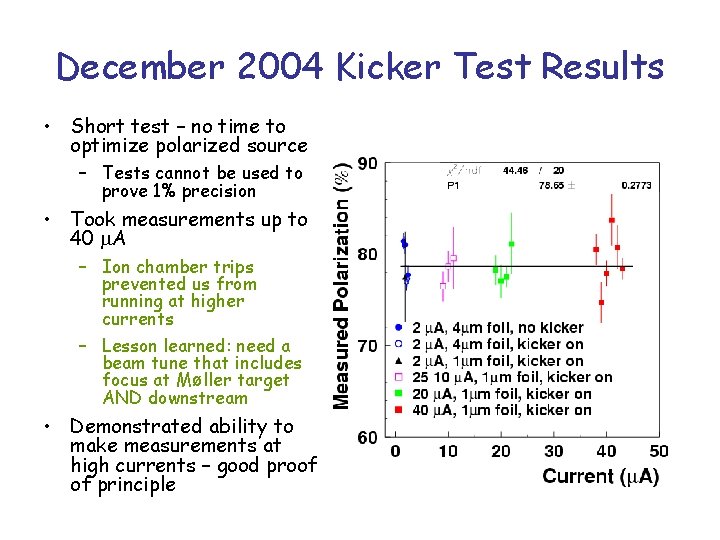
December 2004 Kicker Test Results • Short test – no time to optimize polarized source – Tests cannot be used to prove 1% precision • Took measurements up to 40 m. A – Ion chamber trips prevented us from running at higher currents – Lesson learned: need a beam tune that includes focus at Møller target AND downstream • Demonstrated ability to make measurements at high currents – good proof of principle
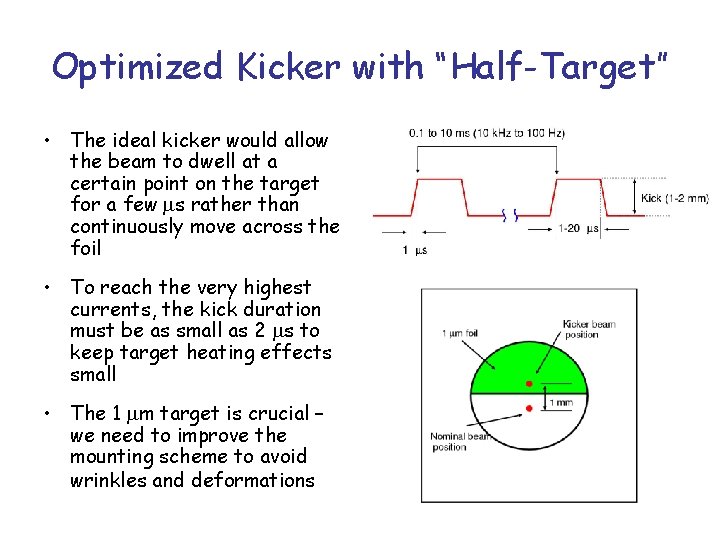
Optimized Kicker with “Half-Target” • The ideal kicker would allow the beam to dwell at a certain point on the target for a few ms rather than continuously move across the foil • To reach the very highest currents, the kick duration must be as small as 2 ms to keep target heating effects small • The 1 mm target is crucial – we need to improve the mounting scheme to avoid wrinkles and deformations
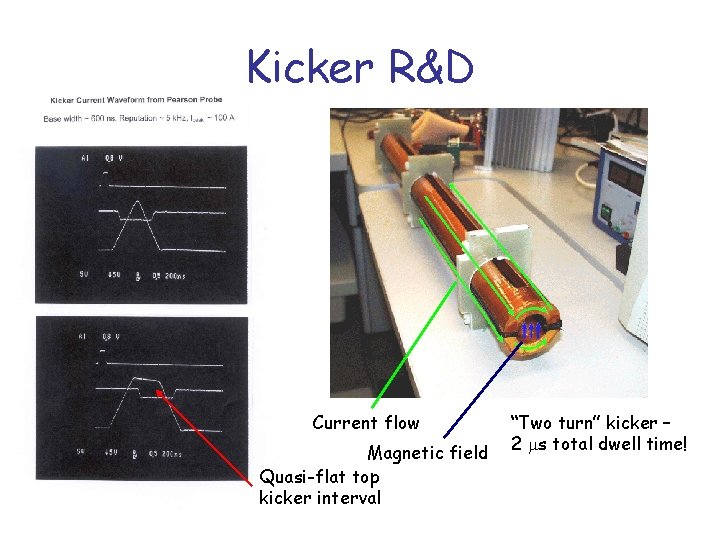
Kicker R&D Current flow Magnetic field Quasi-flat top kicker interval “Two turn” kicker – 2 ms total dwell time!
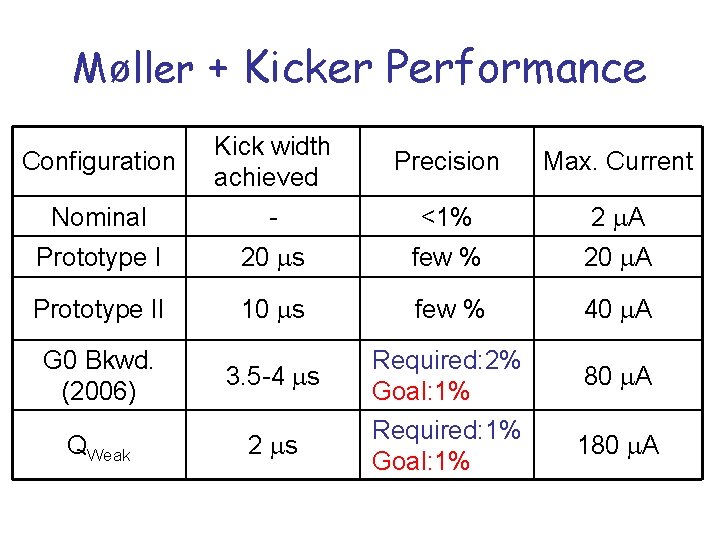
Møller + Kicker Performance Configuration Kick width achieved Precision Max. Current Nominal - <1% 2 m. A Prototype I 20 ms few % 20 m. A Prototype II 10 ms few % 40 m. A G 0 Bkwd. (2006) 3. 5 -4 ms QWeak 2 ms Required: 2% Goal: 1% Required: 1% Goal: 1% 80 m. A 180 m. A
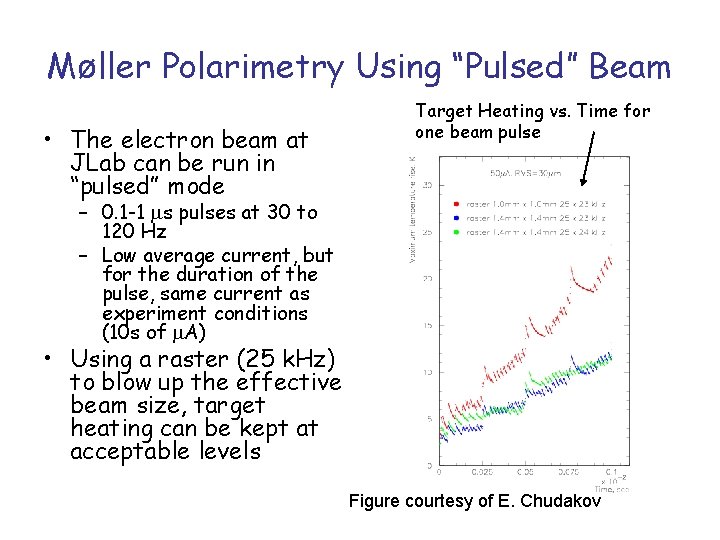
Møller Polarimetry Using “Pulsed” Beam • The electron beam at JLab can be run in “pulsed” mode Target Heating vs. Time for one beam pulse – 0. 1 -1 ms pulses at 30 to 120 Hz – Low average current, but for the duration of the pulse, same current as experiment conditions (10 s of m. A) • Using a raster (25 k. Hz) to blow up the effective beam size, target heating can be kept at acceptable levels Figure courtesy of E. Chudakov
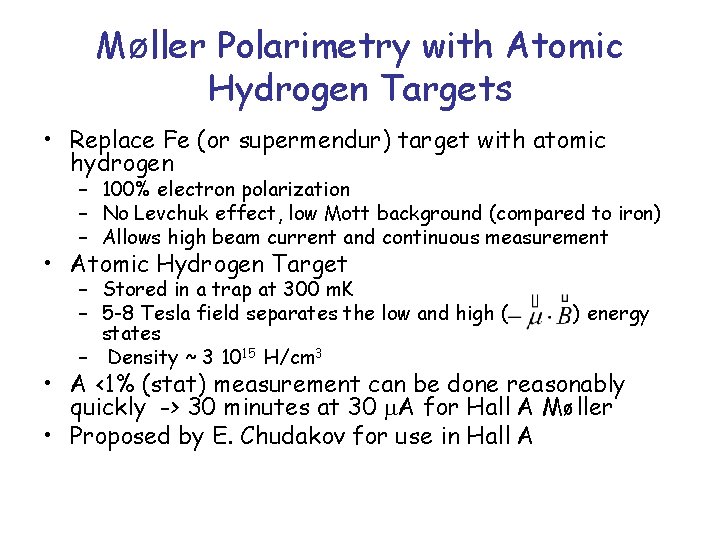
Møller Polarimetry with Atomic Hydrogen Targets • Replace Fe (or supermendur) target with atomic hydrogen – 100% electron polarization – No Levchuk effect, low Mott background (compared to iron) – Allows high beam current and continuous measurement • Atomic Hydrogen Target – Stored in a trap at 300 m. K – 5 -8 Tesla field separates the low and high ( states – Density ~ 3 1015 H/cm 3 ) energy • A <1% (stat) measurement can be done reasonably quickly -> 30 minutes at 30 m. A for Hall A Møller • Proposed by E. Chudakov for use in Hall A
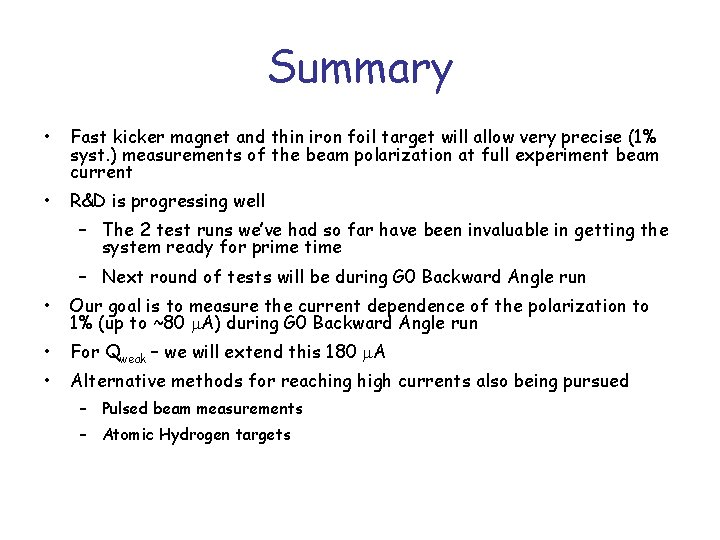
Summary • Fast kicker magnet and thin iron foil target will allow very precise (1% syst. ) measurements of the beam polarization at full experiment beam current • R&D is progressing well – The 2 test runs we’ve had so far have been invaluable in getting the system ready for prime time – Next round of tests will be during G 0 Backward Angle run • Our goal is to measure the current dependence of the polarization to 1% (up to ~80 m. A) during G 0 Backward Angle run • For Qweak – we will extend this 180 m. A • Alternative methods for reaching high currents also being pursued – Pulsed beam measurements – Atomic Hydrogen targets
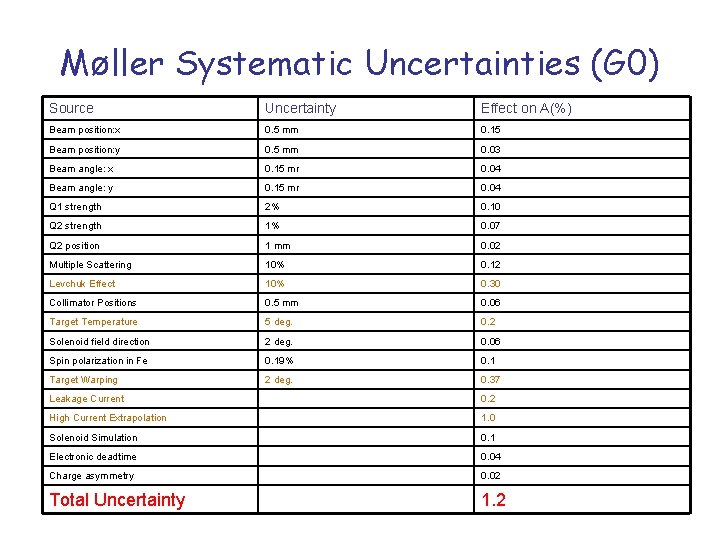
Møller Systematic Uncertainties (G 0) Source Uncertainty Effect on A(%) Beam position: x 0. 5 mm 0. 15 Beam position: y 0. 5 mm 0. 03 Beam angle: x 0. 15 mr 0. 04 Beam angle: y 0. 15 mr 0. 04 Q 1 strength 2% 0. 10 Q 2 strength 1% 0. 07 Q 2 position 1 mm 0. 02 Multiple Scattering 10% 0. 12 Levchuk Effect 10% 0. 30 Collimator Positions 0. 5 mm 0. 06 Target Temperature 5 deg. 0. 2 Solenoid field direction 2 deg. 0. 06 Spin polarization in Fe 0. 19% 0. 1 Target Warping 2 deg. 0. 37 Leakage Current 0. 2 High Current Extrapolation 1. 0 Solenoid Simulation 0. 1 Electronic deadtime 0. 04 Charge asymmetry 0. 02 Total Uncertainty 1. 2
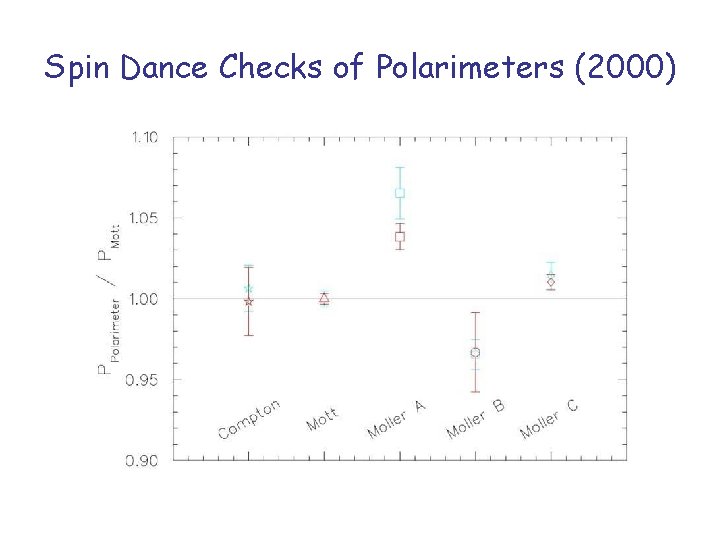
Spin Dance Checks of Polarimeters (2000)
Polarimetry definition in chemistry
Biots law polarimetry
Polarimetry organic chemistry
Difference between precision and non precision instruments
Precision vs semi precision attachment
Bcd gösterimi
Mack gipson jr
One24 webinar
Michael mack md
Cinderella dressed in yellow game
Mack gipson jr
Testament of gideon mack
Streck mack schwedhelm
Judith mack
Reinhard mack konstanz
Camp seph mack map
Szonek
Mack
Mack
Lithography
Antonia roybal mack
Metal coping fpd