Fuel Effect on Compression Ignition Yuanjiang Pei Aramco
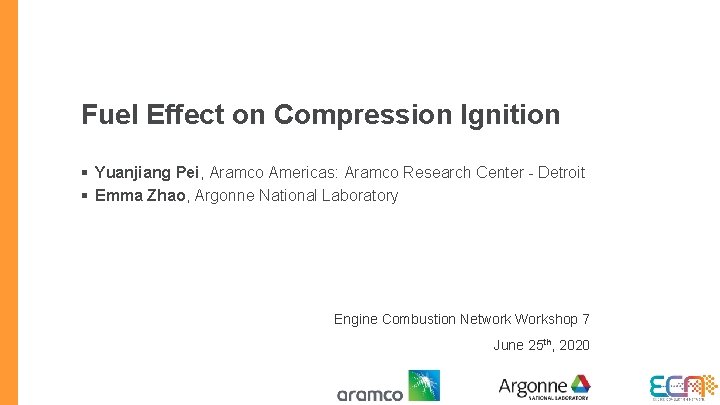
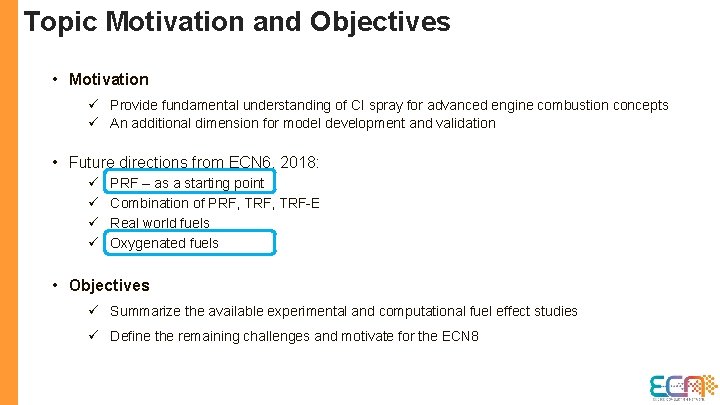
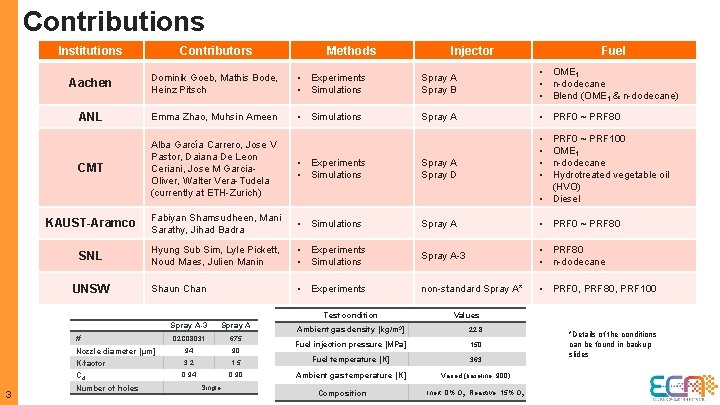
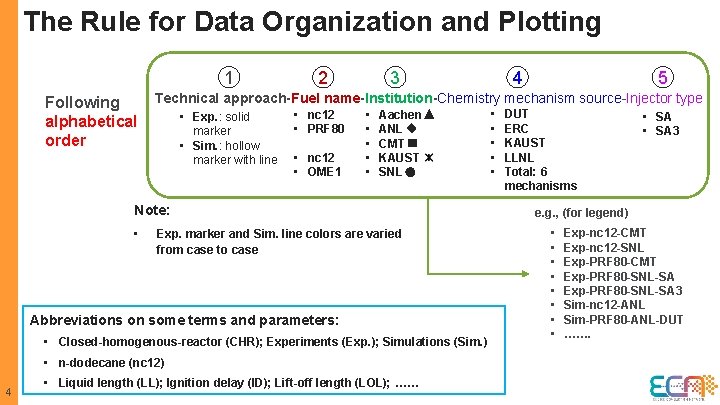
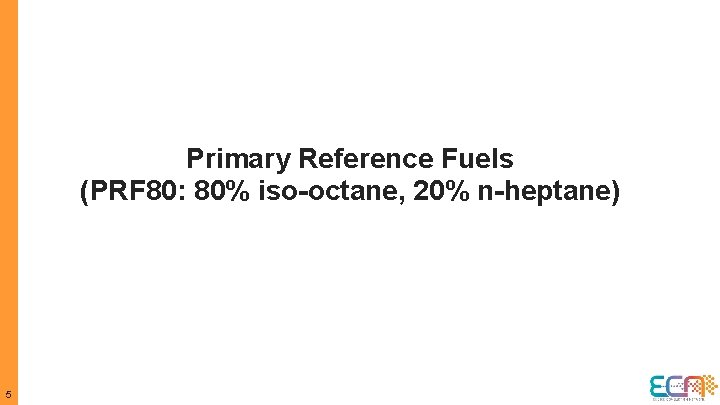
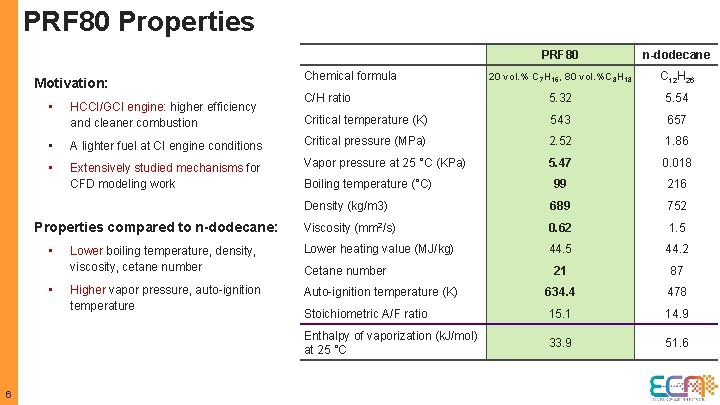
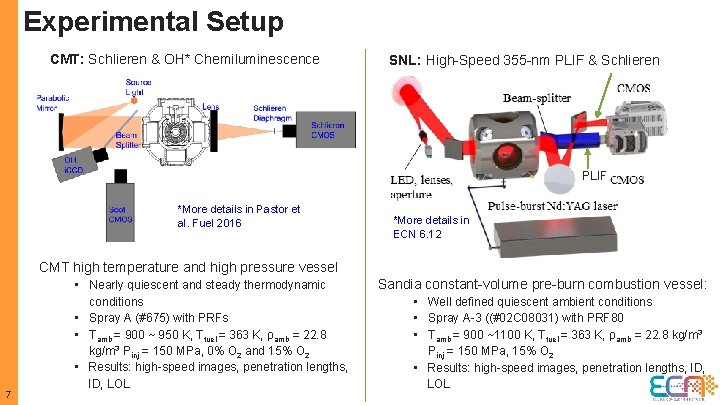
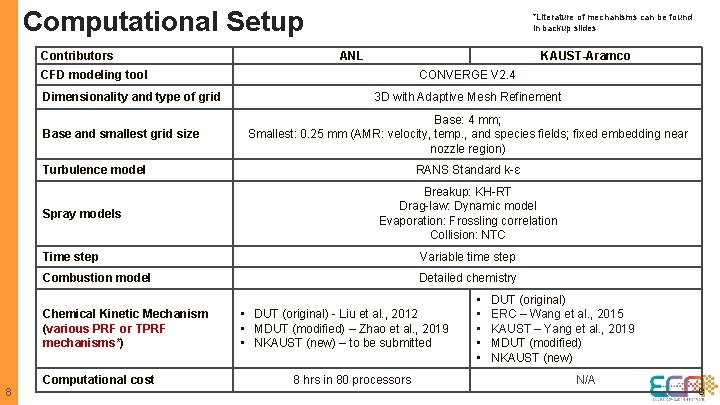
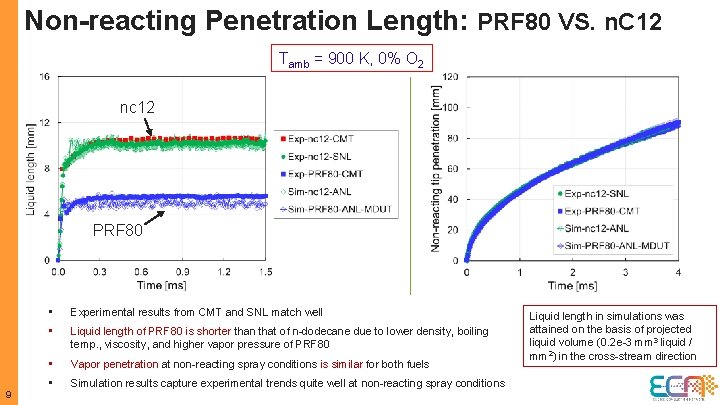
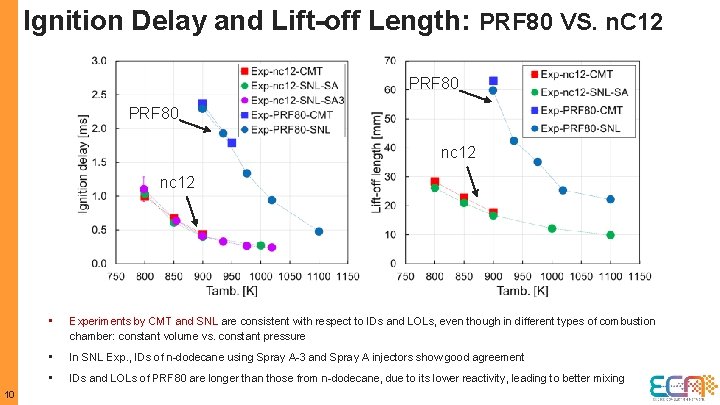
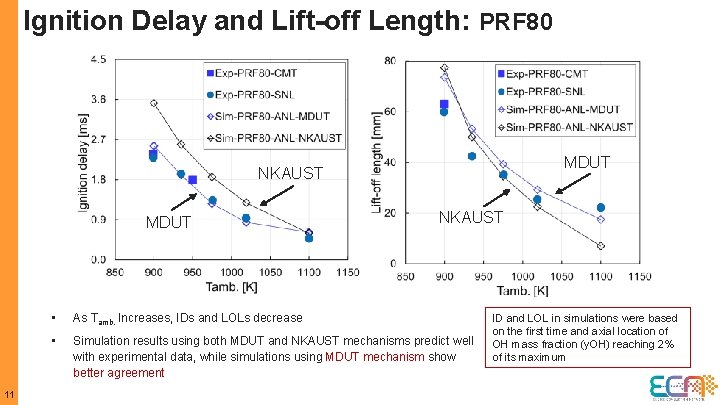
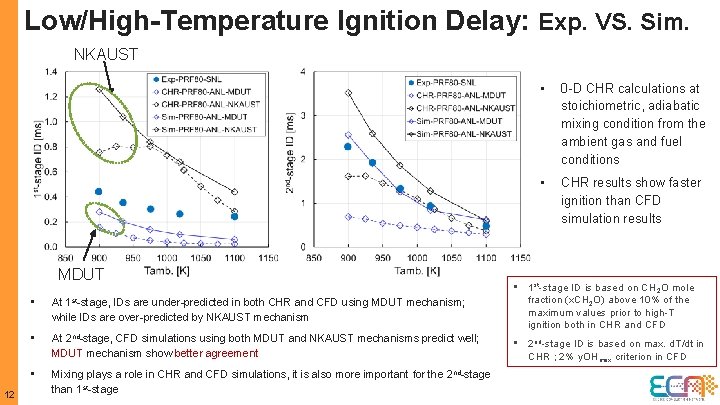
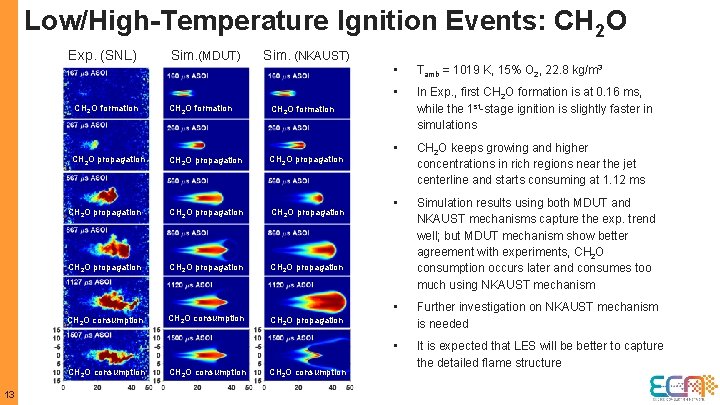
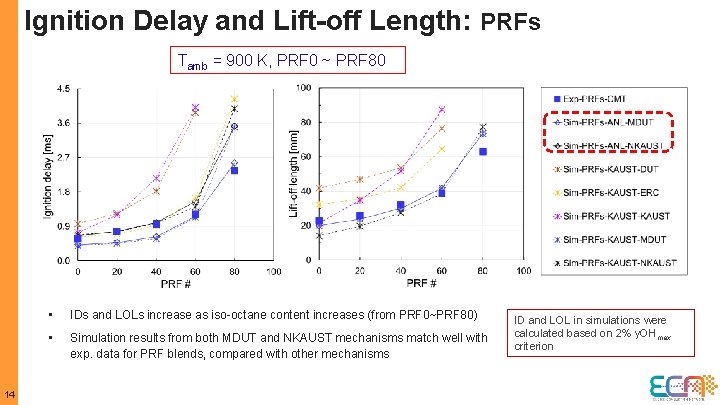
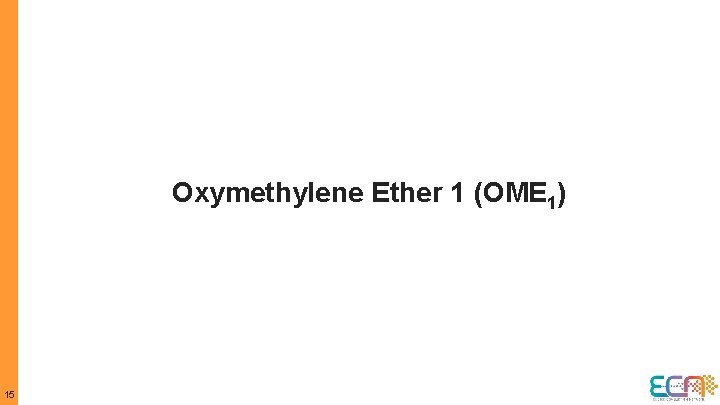
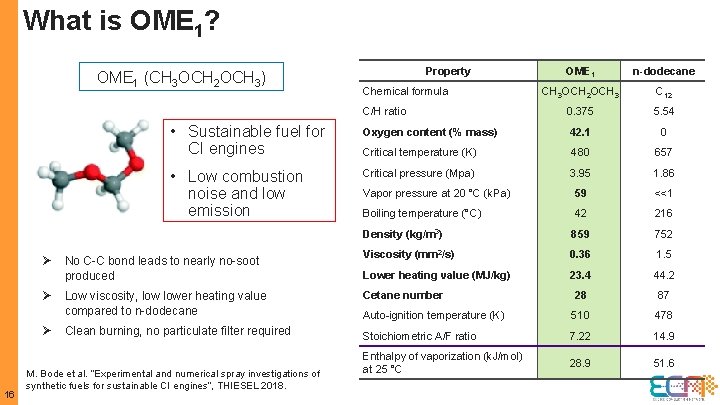
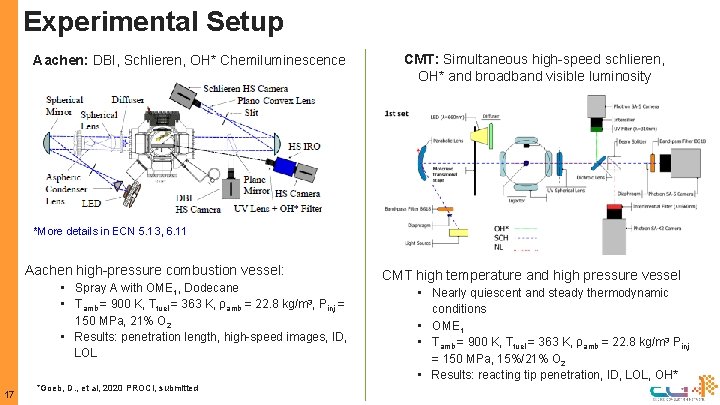
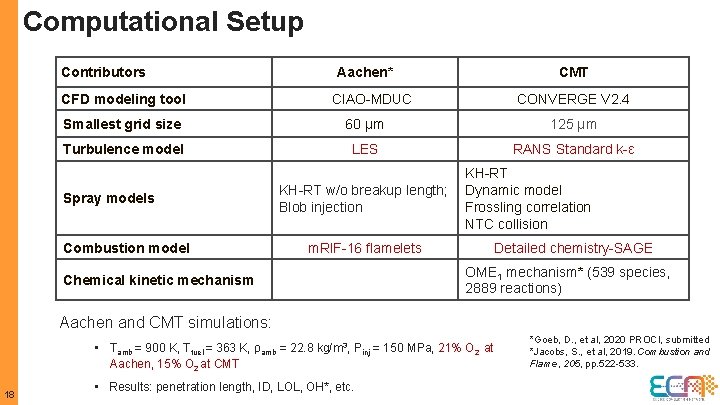
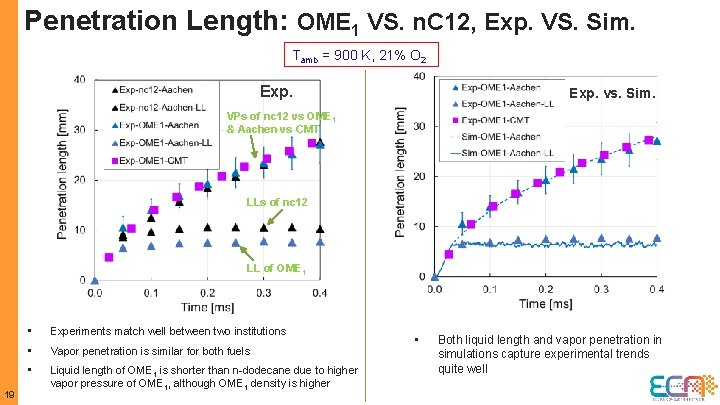
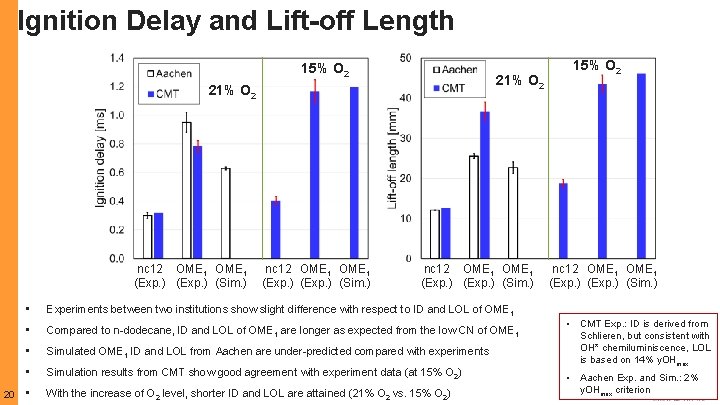
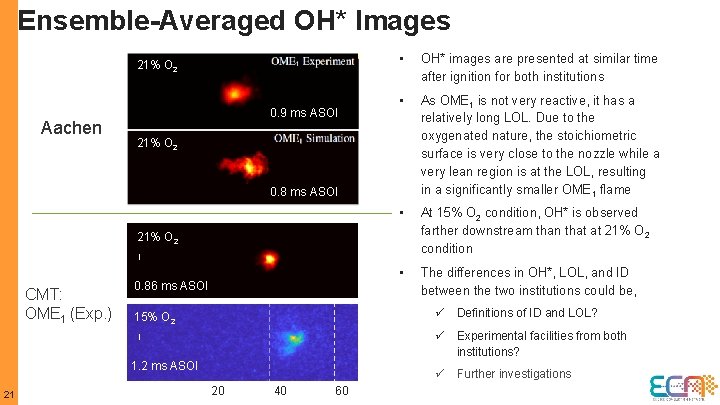
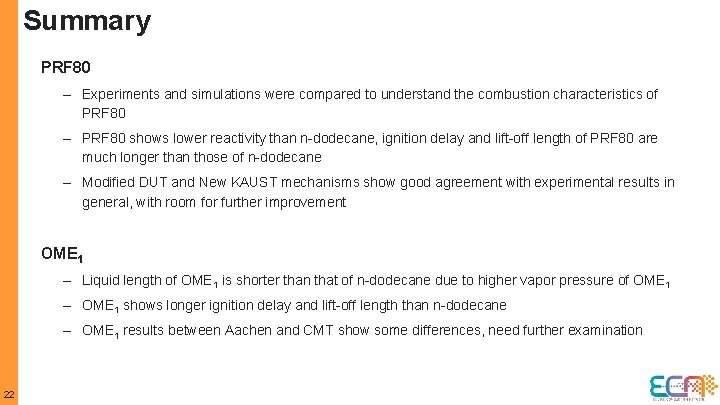
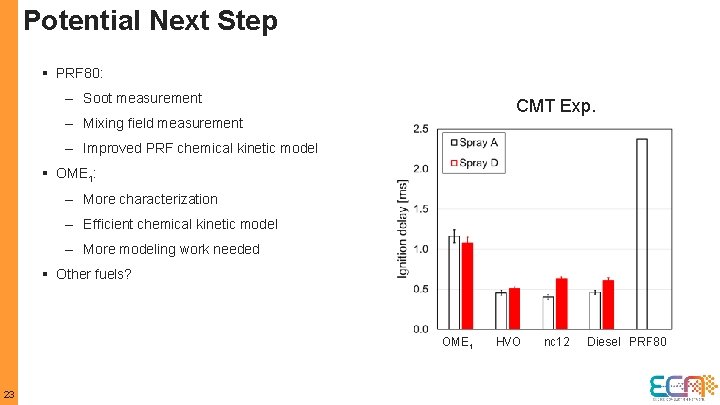
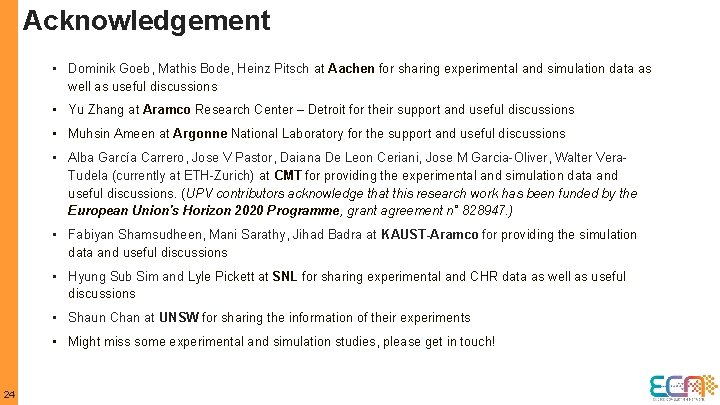
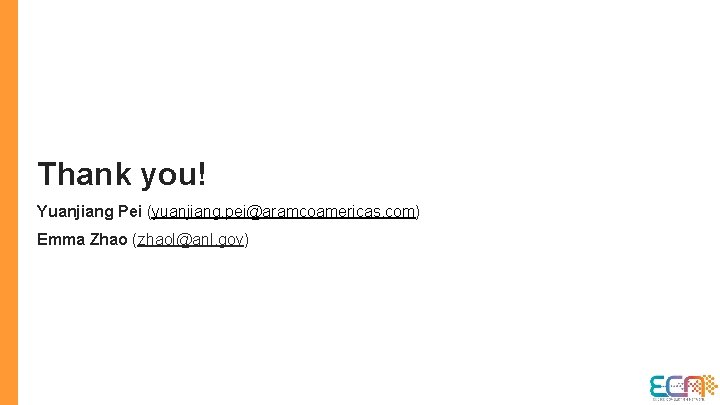
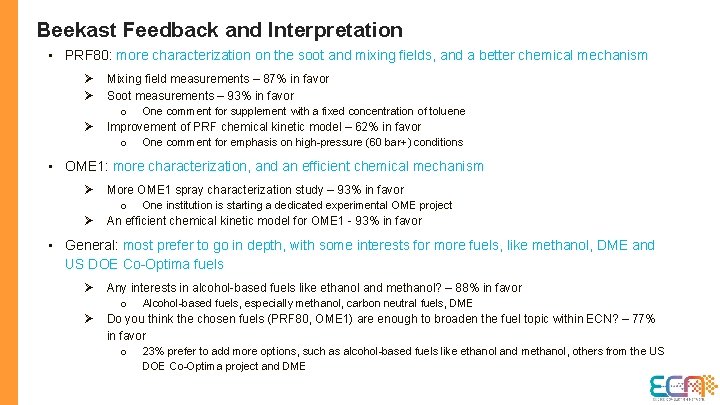
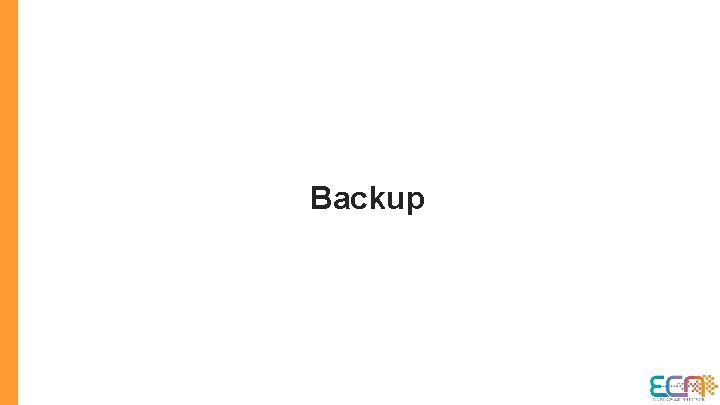
![UNSW PRF BLENDS EXPERIMENTAL CONDITIONS Injection conditions Injection duration [ms] Injection pressure [bar] Nozzle UNSW PRF BLENDS EXPERIMENTAL CONDITIONS Injection conditions Injection duration [ms] Injection pressure [bar] Nozzle](https://slidetodoc.com/presentation_image_h/f380dee1350276c4196e3a518abb6d69/image-28.jpg)
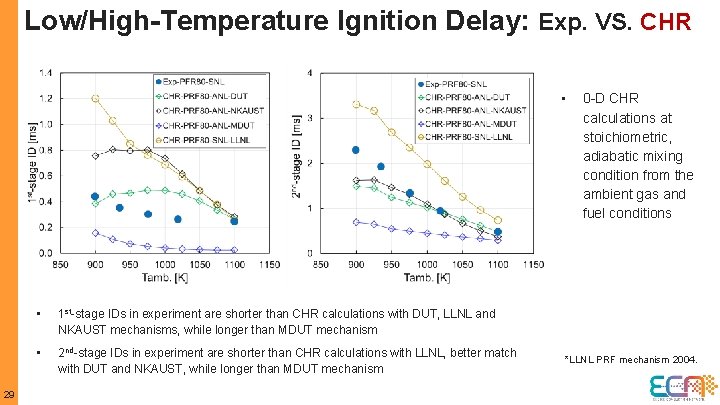
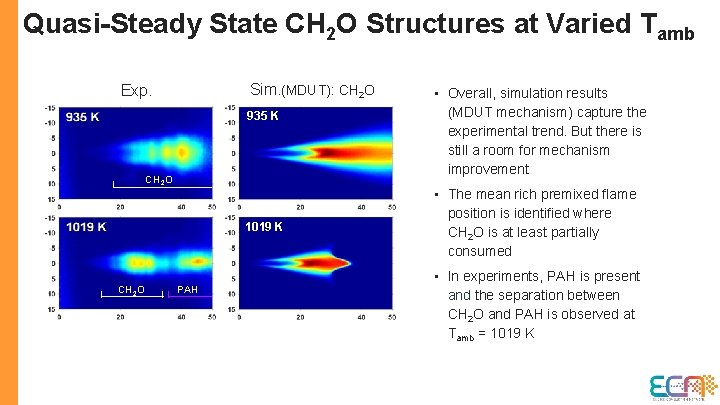
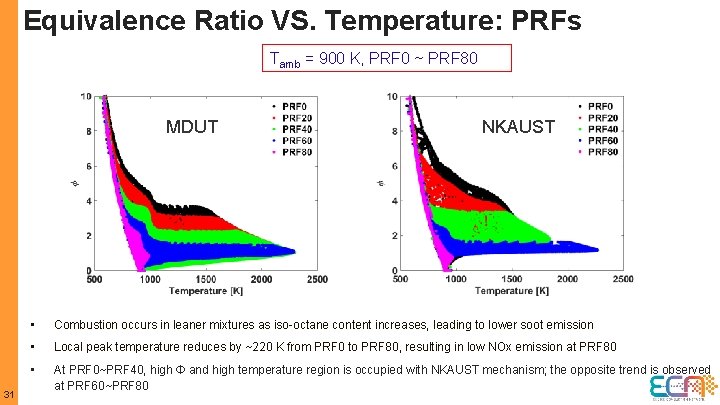
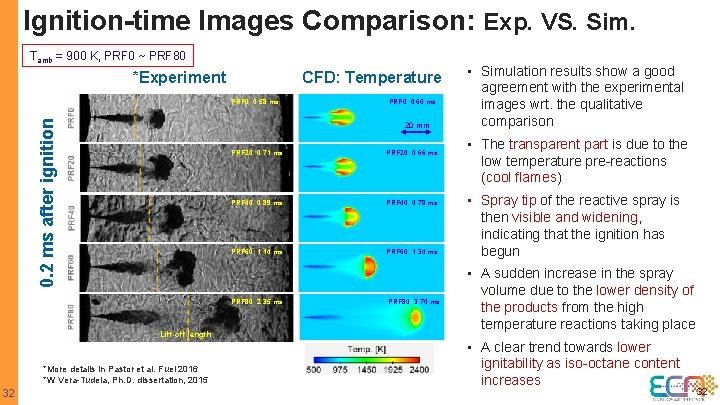
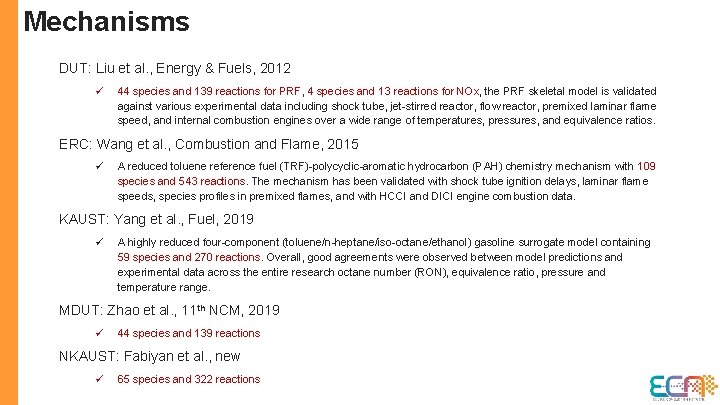
- Slides: 33
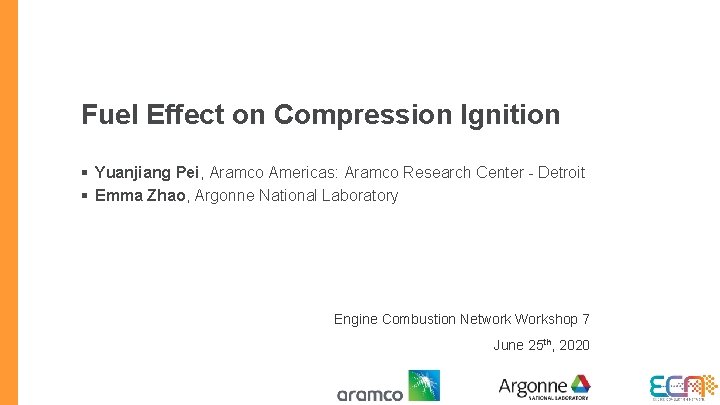
Fuel Effect on Compression Ignition § Yuanjiang Pei, Aramco Americas: Aramco Research Center - Detroit § Emma Zhao, Argonne National Laboratory Engine Combustion Network Workshop 7 June 25 th, 2020
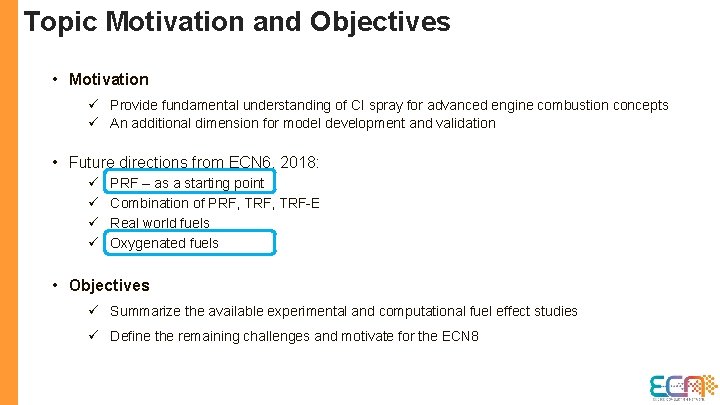
Topic Motivation and Objectives • Motivation ü Provide fundamental understanding of CI spray for advanced engine combustion concepts ü An additional dimension for model development and validation • Future directions from ECN 6, 2018: ü ü PRF – as a starting point Combination of PRF, TRF-E Real world fuels Oxygenated fuels • Objectives ü Summarize the available experimental and computational fuel effect studies ü Define the remaining challenges and motivate for the ECN 8
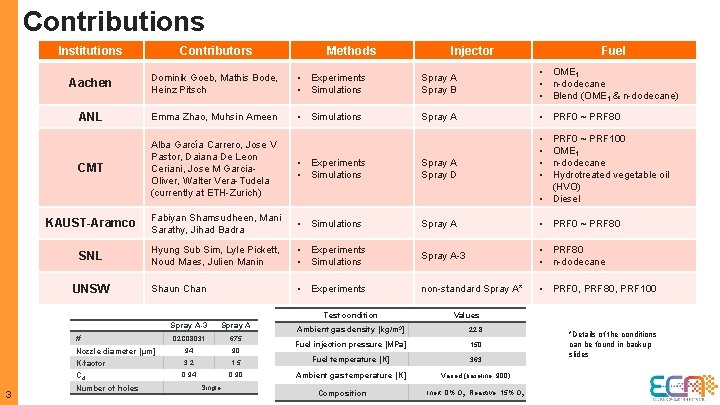
Contributions Institutions Contributors Aachen Dominik Goeb, Mathis Bode, Heinz Pitsch • Experiments • Simulations Spray A Spray B • OME 1 • n-dodecane • Blend (OME 1 & n-dodecane) ANL Emma Zhao, Muhsin Ameen • Simulations Spray A • PRF 0 ~ PRF 80 CMT Alba García Carrero, Jose V Pastor, Daiana De Leon Ceriani, Jose M Garcia. Oliver, Walter Vera-Tudela (currently at ETH-Zurich) • Experiments • Simulations Spray A Spray D • • KAUST-Aramco Fabiyan Shamsudheen, Mani Sarathy, Jihad Badra • Simulations Spray A • PRF 0 ~ PRF 80 SNL Hyung Sub Sim, Lyle Pickett, Noud Maes, Julien Manin • Experiments • Simulations Spray A-3 • PRF 80 • n-dodecane Shaun Chan • Experiments non-standard Spray A* • PRF 0, PRF 80, PRF 100 UNSW Methods Test condition # 3 Spray A-3 Spray A 02 C 08031 675 Injector PRF 0 ~ PRF 100 OME 1 n-dodecane Hydrotreated vegetable oil (HVO) • Diesel Values Ambient gas density [kg/m 3] 22. 8 Fuel injection pressure [MPa] 150 Nozzle diameter [µm] K-factor 94 90 3. 2 1. 5 Fuel temperature [K] 363 Cd 0. 94 0. 90 Ambient gas temperature [K] Varied (baseline: 900) Number of holes Single Composition Fuel Inert: 0% O 2; Reactive: 15% O 2 *Details of the conditions can be found in backup slides
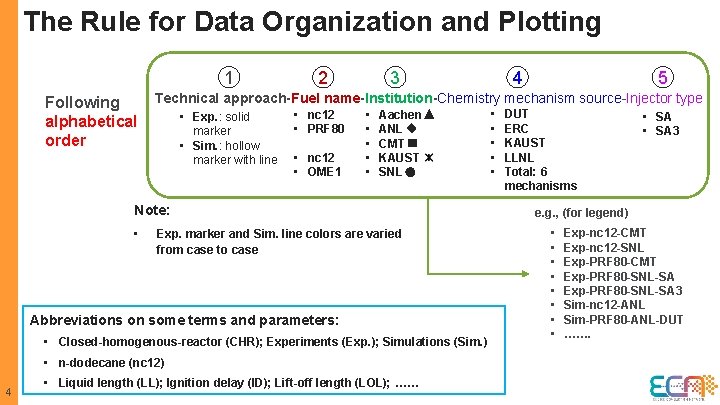
The Rule for Data Organization and Plotting 1 2 3 4 5 Technical approach-Fuel name-Institution-Chemistry mechanism source-Injector type Following • DUT • nc 12 • Aachen • Exp. : solid • SA alphabetical • ERC • PRF 80 • ANL marker • SA 3 order • KAUST • CMT • Sim. : hollow marker with line • nc 12 • OME 1 • KAUST • SNL Note: • Exp. marker and Sim. line colors are varied from case to case Abbreviations on some terms and parameters: • Closed-homogenous-reactor (CHR); Experiments (Exp. ); Simulations (Sim. ) • n-dodecane (nc 12) 4 • Liquid length (LL); Ignition delay (ID); Lift-off length (LOL); …… • LLNL • Total: 6 mechanisms e. g. , (for legend) • • Exp-nc 12 -CMT Exp-nc 12 -SNL Exp-PRF 80 -CMT Exp-PRF 80 -SNL-SA 3 Sim-nc 12 -ANL Sim-PRF 80 -ANL-DUT …….
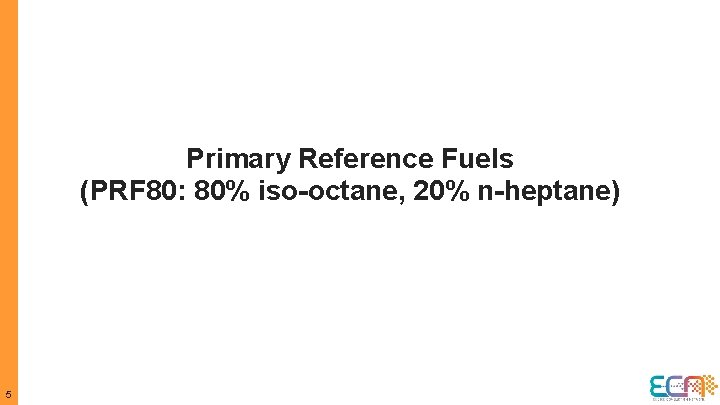
Primary Reference Fuels (PRF 80: 80% iso-octane, 20% n-heptane) 5
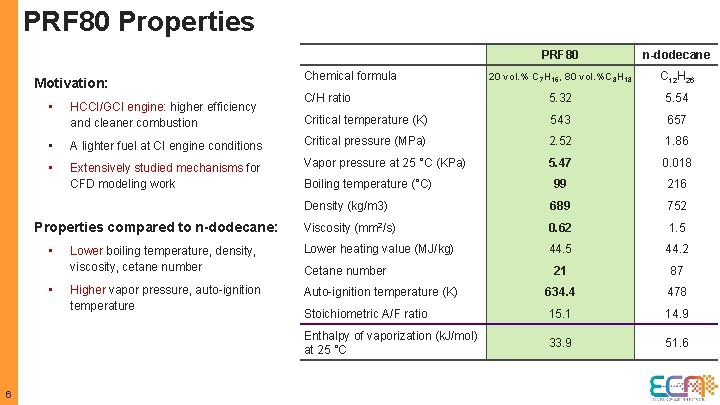
PRF 80 Properties Motivation: n-dodecane 20 vol. % C 7 H 16, 80 vol. %C 8 H 18 C 12 H 26 C/H ratio 5. 32 5. 54 Critical temperature (K) 543 657 Chemical formula • HCCI/GCI engine: higher efficiency and cleaner combustion • A lighter fuel at CI engine conditions Critical pressure (MPa) 2. 52 1. 86 • Extensively studied mechanisms for CFD modeling work Vapor pressure at 25 °C (KPa) 5. 47 0. 018 Boiling temperature (°C) 99 216 Density (kg/m 3) 689 752 Viscosity (mm 2/s) 0. 62 1. 5 Lower boiling temperature, density, viscosity, cetane number Lower heating value (MJ/kg) 44. 5 44. 2 21 87 Higher vapor pressure, auto-ignition temperature Auto-ignition temperature (K) 634. 4 478 Stoichiometric A/F ratio 15. 1 14. 9 Enthalpy of vaporization (k. J/mol) at 25 °C 33. 9 51. 6 Properties compared to n-dodecane: • • 6 PRF 80 Cetane number
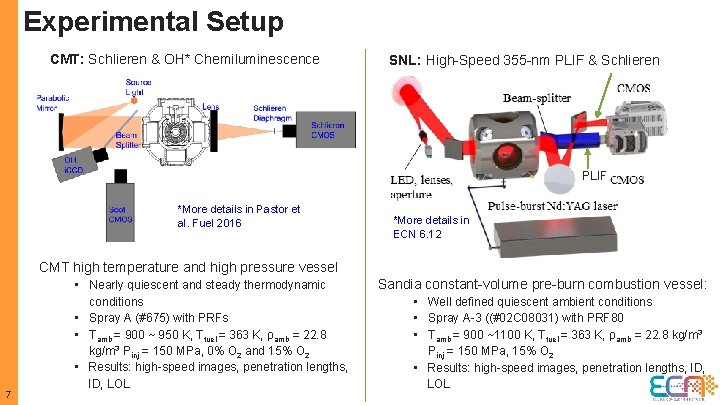
Experimental Setup CMT: Schlieren & OH* Chemiluminescence SNL: High-Speed 355 -nm PLIF & Schlieren PLIF *More details in Pastor et al. Fuel 2016 *More details in ECN 6. 12 CMT high temperature and high pressure vessel 7 • Nearly quiescent and steady thermodynamic conditions • Spray A (#675) with PRFs • Tamb = 900 ~ 950 K, Tfuel = 363 K, ρamb = 22. 8 kg/m 3 Pinj = 150 MPa, 0% O 2 and 15% O 2 • Results: high-speed images, penetration lengths, ID, LOL Sandia constant-volume pre-burn combustion vessel: • Well defined quiescent ambient conditions • Spray A-3 ((#02 C 08031) with PRF 80 • Tamb = 900 ~1100 K, Tfuel = 363 K, ρamb = 22. 8 kg/m 3 Pinj = 150 MPa, 15% O 2 • Results: high-speed images, penetration lengths, ID, LOL
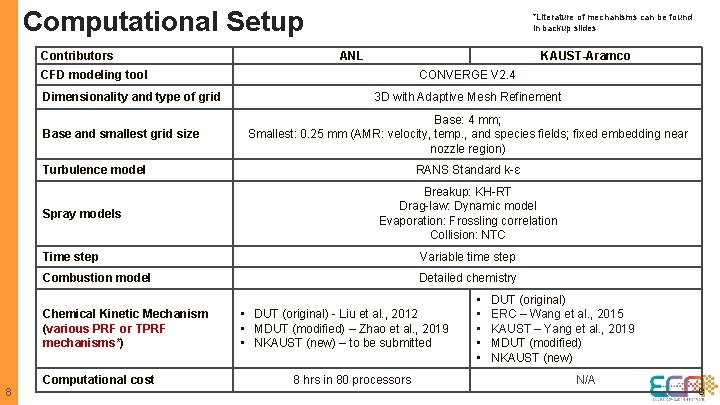
Computational Setup Contributors *Literature of mechanisms can be found in backup slides ANL KAUST-Aramco CFD modeling tool Dimensionality and type of grid Base and smallest grid size CONVERGE V 2. 4 3 D with Adaptive Mesh Refinement Base: 4 mm; Smallest: 0. 25 mm (AMR: velocity, temp. , and species fields; fixed embedding near nozzle region) Turbulence model Spray models Breakup: KH-RT Drag-law: Dynamic model Evaporation: Frossling correlation Collision: NTC Time step Variable time step Combustion model Detailed chemistry Chemical Kinetic Mechanism (various PRF or TPRF mechanisms*) 8 RANS Standard k-ɛ Computational cost • DUT (original) - Liu et al. , 2012 • MDUT (modified) – Zhao et al. , 2019 • NKAUST (new) – to be submitted 8 hrs in 80 processors • • • DUT (original) ERC – Wang et al. , 2015 KAUST – Yang et al. , 2019 MDUT (modified) NKAUST (new) N/A 8
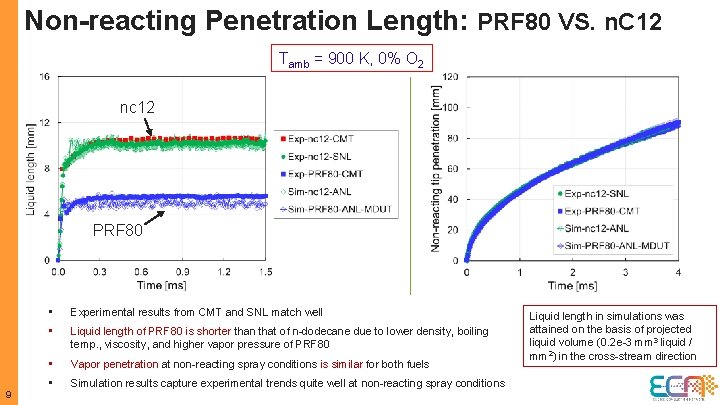
Non-reacting Penetration Length: PRF 80 VS. n. C 12 Tamb = 900 K, 0% O 2 nc 12 PRF 80 9 • Experimental results from CMT and SNL match well • Liquid length of PRF 80 is shorter than that of n-dodecane due to lower density, boiling temp. , viscosity, and higher vapor pressure of PRF 80 • Vapor penetration at non-reacting spray conditions is similar for both fuels • Simulation results capture experimental trends quite well at non-reacting spray conditions Liquid length in simulations was attained on the basis of projected liquid volume (0. 2 e-3 mm 3 liquid / mm 2) in the cross-stream direction
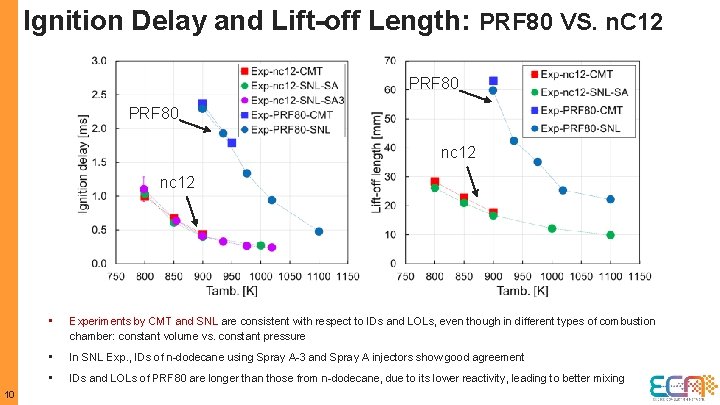
Ignition Delay and Lift-off Length: PRF 80 VS. n. C 12 PRF 80 nc 12 10 • Experiments by CMT and SNL are consistent with respect to IDs and LOLs, even though in different types of combustion chamber: constant volume vs. constant pressure • In SNL Exp. , IDs of n-dodecane using Spray A-3 and Spray A injectors show good agreement • IDs and LOLs of PRF 80 are longer than those from n-dodecane, due to its lower reactivity, leading to better mixing
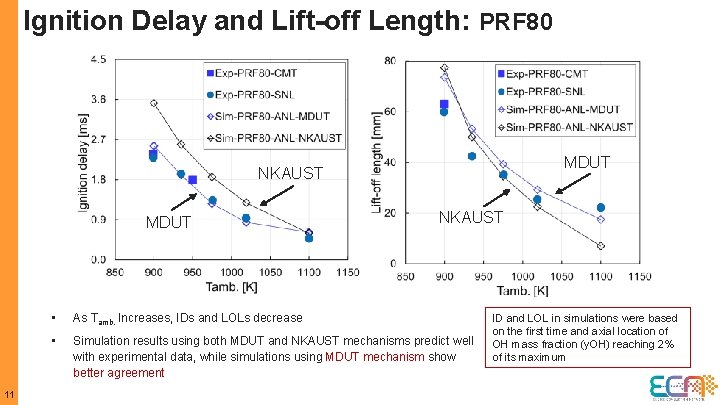
Ignition Delay and Lift-off Length: PRF 80 MDUT NKAUST MDUT 11 NKAUST • As Tamb. Increases, IDs and LOLs decrease • Simulation results using both MDUT and NKAUST mechanisms predict well with experimental data, while simulations using MDUT mechanism show better agreement ID and LOL in simulations were based on the first time and axial location of OH mass fraction (y. OH) reaching 2% of its maximum
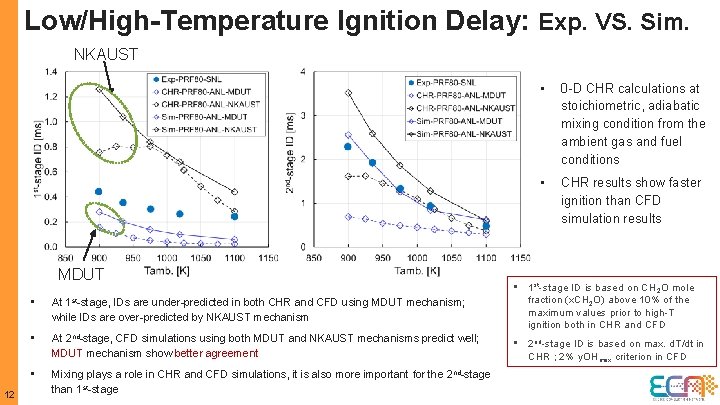
Low/High-Temperature Ignition Delay: Exp. VS. Sim. NKAUST MDUT 12 • 0 -D CHR calculations at stoichiometric, adiabatic mixing condition from the ambient gas and fuel conditions • CHR results show faster ignition than CFD simulation results • At 1 st-stage, IDs are under-predicted in both CHR and CFD using MDUT mechanism; while IDs are over-predicted by NKAUST mechanism • 1 st-stage ID is based on CH 2 O mole fraction (x. CH 2 O) above 10% of the maximum values prior to high-T ignition both in CHR and CFD • At 2 nd-stage, CFD simulations using both MDUT and NKAUST mechanisms predict well; MDUT mechanism show better agreement • 2 nd-stage ID is based on max. d. T/dt in CHR ; 2% y. OHmax criterion in CFD • Mixing plays a role in CHR and CFD simulations, it is also more important for the 2 nd-stage than 1 st-stage
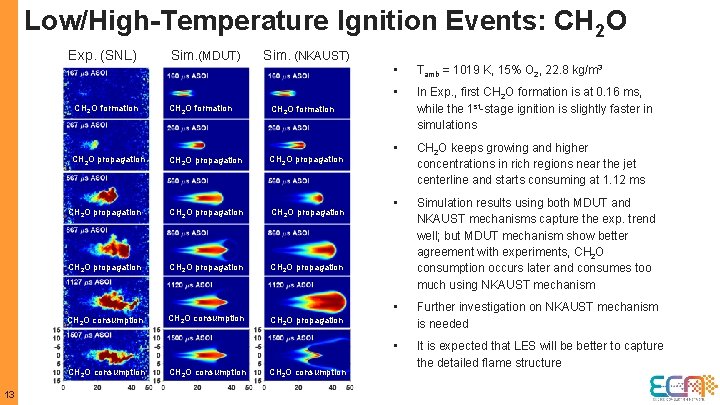
Low/High-Temperature Ignition Events: CH 2 O Exp. (SNL) CH 2 O formation Sim. (NKAUST) CH 2 O formation CH 2 O propagation CH 2 O propagation CH 2 O consumption CH 2 O propagation CH 2 O consumption 13 Sim. (MDUT) CH 2 O consumption • Tamb = 1019 K, 15% O 2, 22. 8 kg/m 3 • In Exp. , first CH 2 O formation is at 0. 16 ms, while the 1 st-stage ignition is slightly faster in simulations • CH 2 O keeps growing and higher concentrations in rich regions near the jet centerline and starts consuming at 1. 12 ms • Simulation results using both MDUT and NKAUST mechanisms capture the exp. trend well; but MDUT mechanism show better agreement with experiments, CH 2 O consumption occurs later and consumes too much using NKAUST mechanism • Further investigation on NKAUST mechanism is needed • It is expected that LES will be better to capture the detailed flame structure
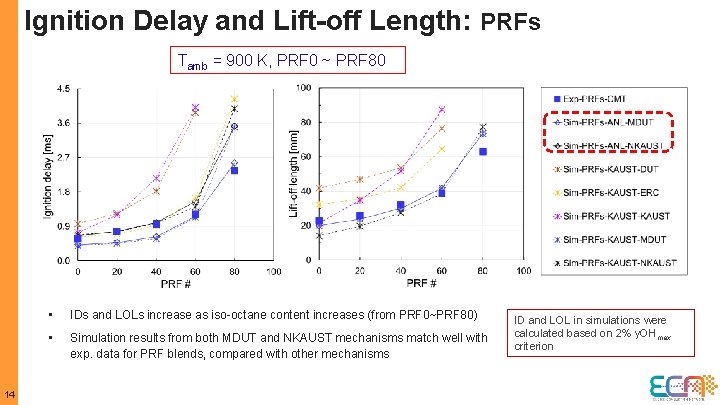
Ignition Delay and Lift-off Length: PRFs Tamb = 900 K, PRF 0 ~ PRF 80 14 • IDs and LOLs increase as iso-octane content increases (from PRF 0~PRF 80) • Simulation results from both MDUT and NKAUST mechanisms match well with exp. data for PRF blends, compared with other mechanisms ID and LOL in simulations were calculated based on 2% y. OHmax criterion
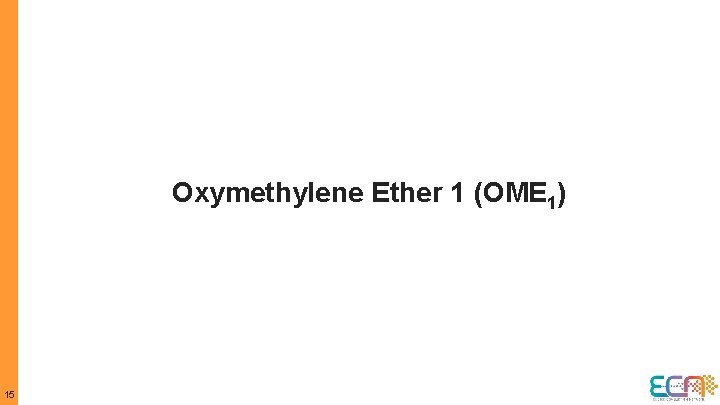
Oxymethylene Ether 1 (OME 1) 15
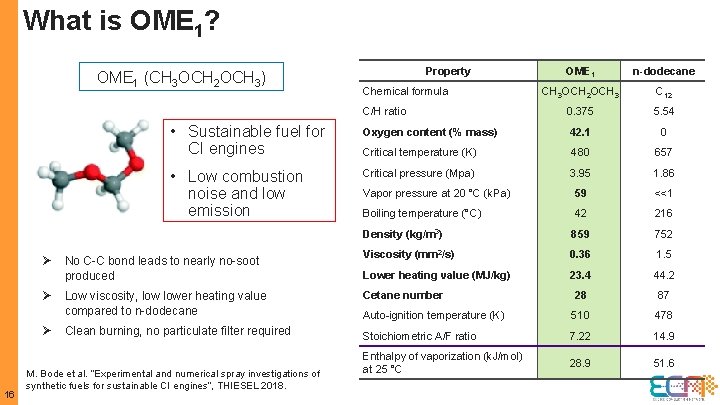
What is OME 1? OME 1 n-dodecane CH 3 OCH 2 OCH 3 C 12 C/H ratio 0. 375 5. 54 • Sustainable fuel for CI engines Oxygen content (% mass) 42. 1 0 Critical temperature (K) 480 657 • Low combustion noise and low emission Critical pressure (Mpa) 3. 95 1. 86 Vapor pressure at 20 °C (k. Pa) 59 <<1 Boiling temperature (°C) 42 216 Density (kg/m 3) 859 752 Viscosity (mm 2/s) 0. 36 1. 5 Lower heating value (MJ/kg) 23. 4 44. 2 Cetane number 28 87 Auto-ignition temperature (K) 510 478 Stoichiometric A/F ratio 7. 22 14. 9 Enthalpy of vaporization (k. J/mol) at 25 °C 28. 9 51. 6 OME 1 (CH 3 OCH 2 OCH 3) Ø Ø Ø 16 No C-C bond leads to nearly no-soot produced Low viscosity, lower heating value compared to n-dodecane Clean burning, no particulate filter required M. Bode et al. “Experimental and numerical spray investigations of synthetic fuels for sustainable CI engines”, THIESEL 2018. Property Chemical formula
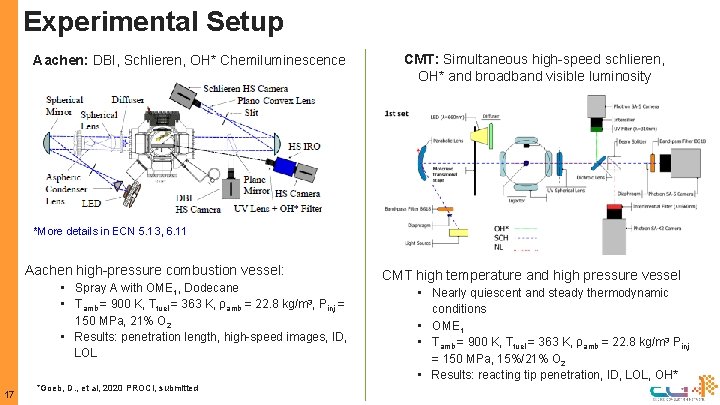
Experimental Setup Aachen: DBI, Schlieren, OH* Chemiluminescence CMT: Simultaneous high-speed schlieren, OH* and broadband visible luminosity *More details in ECN 5. 13, 6. 11 Aachen high-pressure combustion vessel: • Spray A with OME 1, Dodecane • Tamb = 900 K, Tfuel = 363 K, ρamb = 22. 8 kg/m 3, Pinj = 150 MPa, 21% O 2 • Results: penetration length, high-speed images, ID, LOL 17 *Goeb, D. , et al, 2020 PROCI, submitted CMT high temperature and high pressure vessel • Nearly quiescent and steady thermodynamic conditions • OME 1 • Tamb = 900 K, Tfuel = 363 K, ρamb = 22. 8 kg/m 3 Pinj = 150 MPa, 15%/21% O 2 • Results: reacting tip penetration, ID, LOL, OH*
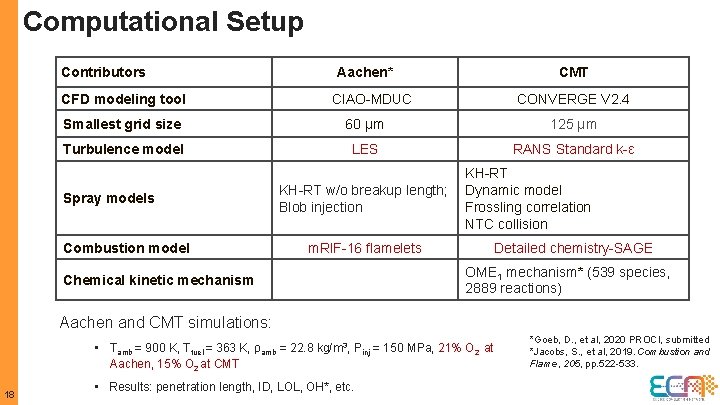
Computational Setup Contributors CFD modeling tool Aachen* CMT CONVERGE V 2. 4 CIAO-MDUC Smallest grid size 60 µm 125 µm Turbulence model LES RANS Standard k-ɛ Spray models Combustion model KH-RT w/o breakup length; Blob injection m. RIF-16 flamelets Chemical kinetic mechanism KH-RT Dynamic model Frossling correlation NTC collision Detailed chemistry-SAGE OME 1 mechanism* (539 species, 2889 reactions) Aachen and CMT simulations: • Tamb = 900 K, Tfuel = 363 K, ρamb = 22. 8 kg/m 3, Pinj = 150 MPa, 21% O 2 at Aachen, 15% O 2 at CMT 18 • Results: penetration length, ID, LOL, OH*, etc. *Goeb, D. , et al, 2020 PROCI, submitted *Jacobs, S. , et al, 2019. Combustion and Flame, 205, pp. 522 -533.
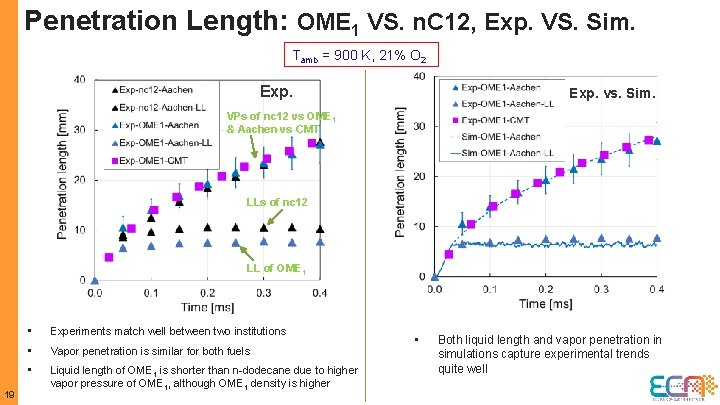
Penetration Length: OME 1 VS. n. C 12, Exp. VS. Sim. Tamb = 900 K, 21% O 2 Exp. vs. Sim. VPs of nc 12 vs OME 1 & Aachen vs CMT LLs of nc 12 LL of OME 1 19 • Experiments match well between two institutions • Vapor penetration is similar for both fuels • Liquid length of OME 1 is shorter than n-dodecane due to higher vapor pressure of OME 1, although OME 1 density is higher • Both liquid length and vapor penetration in simulations capture experimental trends quite well
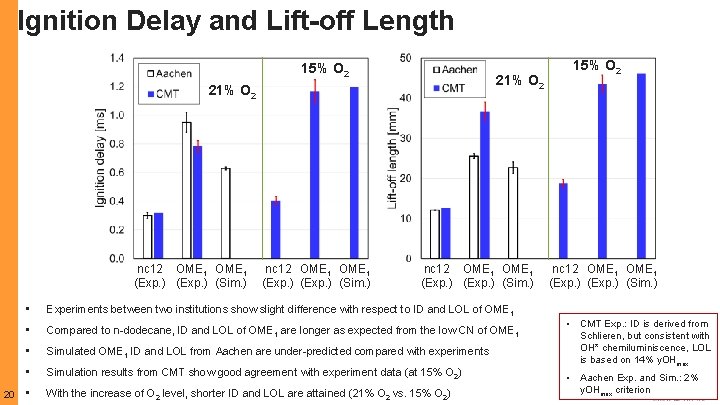
Ignition Delay and Lift-off Length 15% O 2 21% O 2 nc 12 OME 1 (Exp. ) (Sim. ) 20 15% O 2 nc 12 OME 1 (Exp. ) (Sim. ) • Experiments between two institutions show slight difference with respect to ID and LOL of OME 1 • Compared to n-dodecane, ID and LOL of OME 1 are longer as expected from the low CN of OME 1 • Simulated OME 1 ID and LOL from Aachen are under-predicted compared with experiments • Simulation results from CMT show good agreement with experiment data (at 15% O 2) • With the increase of O 2 level, shorter ID and LOL are attained (21% O 2 vs. 15% O 2) • CMT Exp. : ID is derived from Schlieren, but consistent with OH* chemiluminiscence, LOL is based on 14% y. OHmax • Aachen Exp. and Sim. : 2% y. OHmax criterion
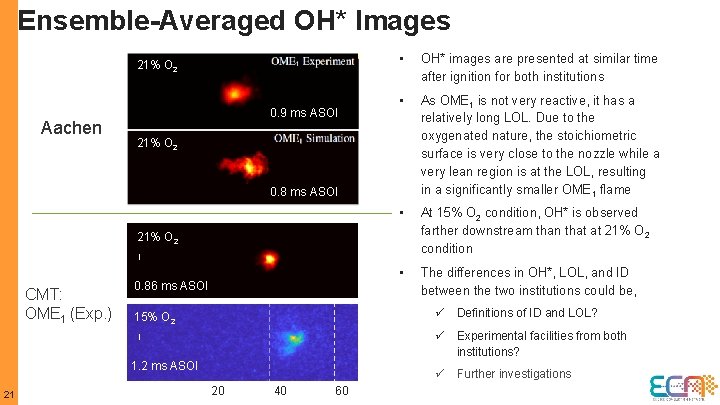
Ensemble-Averaged OH* Images 21% O 2 Aachen 0. 9 ms ASOI • OH* images are presented at similar time after ignition for both institutions • As OME 1 is not very reactive, it has a relatively long LOL. Due to the oxygenated nature, the stoichiometric surface is very close to the nozzle while a very lean region is at the LOL, resulting in a significantly smaller OME 1 flame • At 15% O 2 condition, OH* is observed farther downstream than that at 21% O 2 condition • The differences in OH*, LOL, and ID between the two institutions could be, 21% O 2 0. 8 ms ASOI 21% O 2 CMT: OME 1 (Exp. ) 0. 86 ms ASOI ü Definitions of ID and LOL? 15% O 2 ü Experimental facilities from both institutions? 1. 2 ms ASOI 21 ü Further investigations 20 40 60
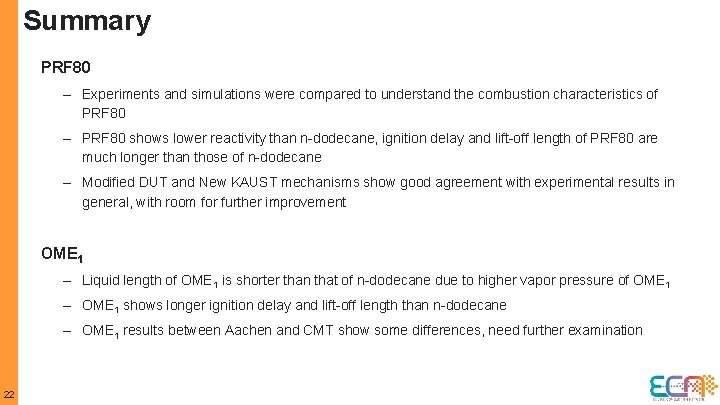
Summary PRF 80 – Experiments and simulations were compared to understand the combustion characteristics of PRF 80 – PRF 80 shows lower reactivity than n-dodecane, ignition delay and lift-off length of PRF 80 are much longer than those of n-dodecane – Modified DUT and New KAUST mechanisms show good agreement with experimental results in general, with room for further improvement OME 1 – Liquid length of OME 1 is shorter than that of n-dodecane due to higher vapor pressure of OME 1 – OME 1 shows longer ignition delay and lift-off length than n-dodecane – OME 1 results between Aachen and CMT show some differences, need further examination 22
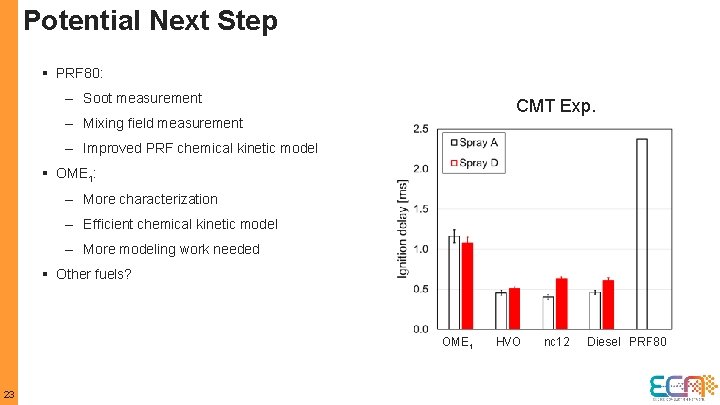
Potential Next Step § PRF 80: – Soot measurement CMT Exp. – Mixing field measurement – Improved PRF chemical kinetic model § OME 1: – More characterization – Efficient chemical kinetic model – More modeling work needed § Other fuels? OME 1 23 HVO nc 12 Diesel PRF 80
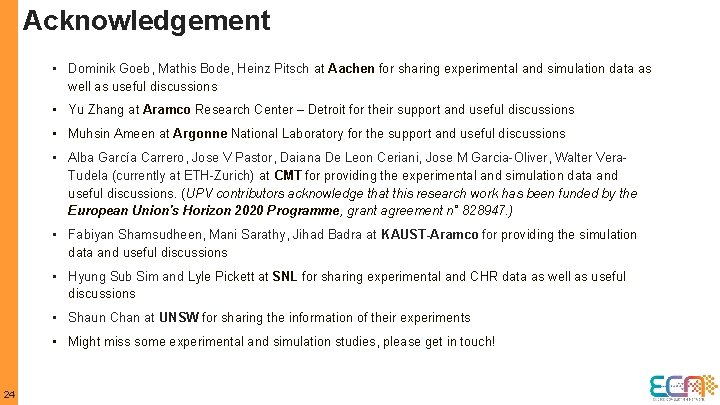
Acknowledgement • Dominik Goeb, Mathis Bode, Heinz Pitsch at Aachen for sharing experimental and simulation data as well as useful discussions • Yu Zhang at Aramco Research Center – Detroit for their support and useful discussions • Muhsin Ameen at Argonne National Laboratory for the support and useful discussions • Alba García Carrero, Jose V Pastor, Daiana De Leon Ceriani, Jose M Garcia-Oliver, Walter Vera. Tudela (currently at ETH-Zurich) at CMT for providing the experimental and simulation data and useful discussions. (UPV contributors acknowledge that this research work has been funded by the European Union's Horizon 2020 Programme, grant agreement n° 828947. ) • Fabiyan Shamsudheen, Mani Sarathy, Jihad Badra at KAUST-Aramco for providing the simulation data and useful discussions • Hyung Sub Sim and Lyle Pickett at SNL for sharing experimental and CHR data as well as useful discussions • Shaun Chan at UNSW for sharing the information of their experiments • Might miss some experimental and simulation studies, please get in touch! 24
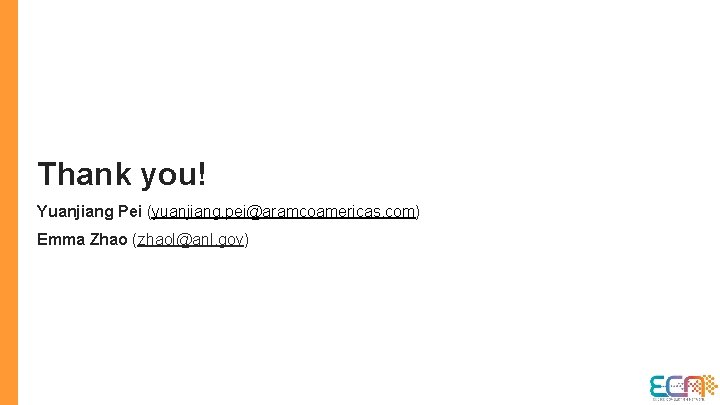
Thank you! Yuanjiang Pei (yuanjiang. pei@aramcoamericas. com) Emma Zhao (zhaol@anl. gov)
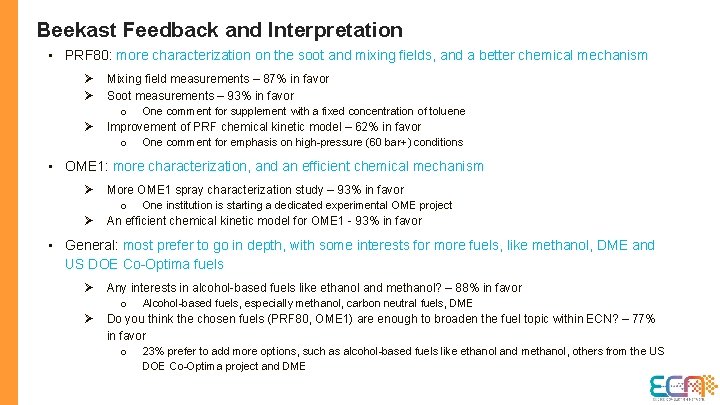
Beekast Feedback and Interpretation • PRF 80: more characterization on the soot and mixing fields, and a better chemical mechanism Ø Ø Ø Mixing field measurements – 87% in favor Soot measurements – 93% in favor o One comment for supplement with a fixed concentration of toluene Improvement of PRF chemical kinetic model – 62% in favor o One comment for emphasis on high-pressure (60 bar+) conditions • OME 1: more characterization, and an efficient chemical mechanism Ø Ø More OME 1 spray characterization study – 93% in favor o One institution is starting a dedicated experimental OME project An efficient chemical kinetic model for OME 1 - 93% in favor • General: most prefer to go in depth, with some interests for more fuels, like methanol, DME and US DOE Co-Optima fuels Ø Ø Any interests in alcohol-based fuels like ethanol and methanol? – 88% in favor o Alcohol-based fuels, especially methanol, carbon neutral fuels, DME Do you think the chosen fuels (PRF 80, OME 1) are enough to broaden the fuel topic within ECN? – 77% in favor o 23% prefer to add more options, such as alcohol-based fuels like ethanol and methanol, others from the US DOE Co-Optima project and DME
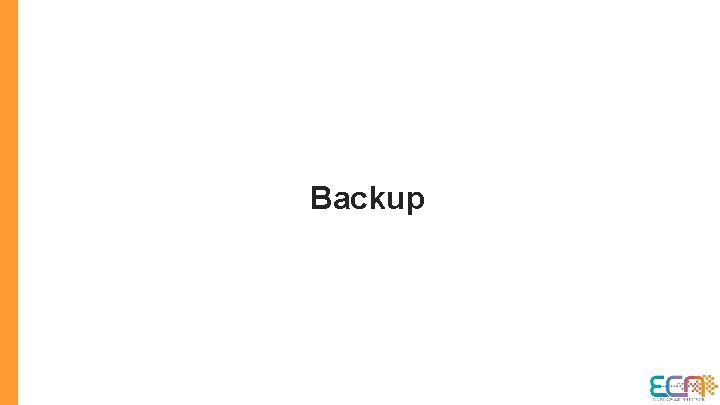
Backup
![UNSW PRF BLENDS EXPERIMENTAL CONDITIONS Injection conditions Injection duration ms Injection pressure bar Nozzle UNSW PRF BLENDS EXPERIMENTAL CONDITIONS Injection conditions Injection duration [ms] Injection pressure [bar] Nozzle](https://slidetodoc.com/presentation_image_h/f380dee1350276c4196e3a518abb6d69/image-28.jpg)
UNSW PRF BLENDS EXPERIMENTAL CONDITIONS Injection conditions Injection duration [ms] Injection pressure [bar] Nozzle diameter [μm] Fuel temperature [K] Test conditions Ambient density [kg/m 3] 8. 85 (hydraulic) 1000 105 403 22. 8 Ambient oxygen level [vol. %] 15 (reacting) 0 (non-reacting) Ambient temperature [K] 1150 (PRF 100) 1120 (PRF 80) 900 (PRF 0) Bulk temperature [K] 968 (PRF 100) 921 (PRF 80) 703 (PRF 0)
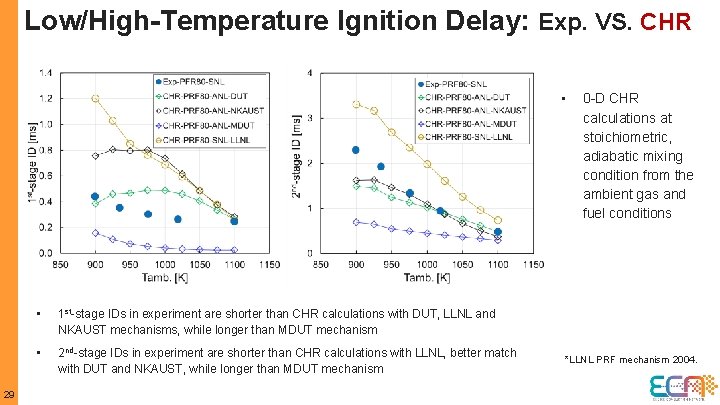
Low/High-Temperature Ignition Delay: Exp. VS. CHR • 29 • 1 st-stage IDs in experiment are shorter than CHR calculations with DUT, LLNL and NKAUST mechanisms, while longer than MDUT mechanism • 2 nd-stage IDs in experiment are shorter than CHR calculations with LLNL, better match with DUT and NKAUST, while longer than MDUT mechanism 0 -D CHR calculations at stoichiometric, adiabatic mixing condition from the ambient gas and fuel conditions *LLNL PRF mechanism 2004.
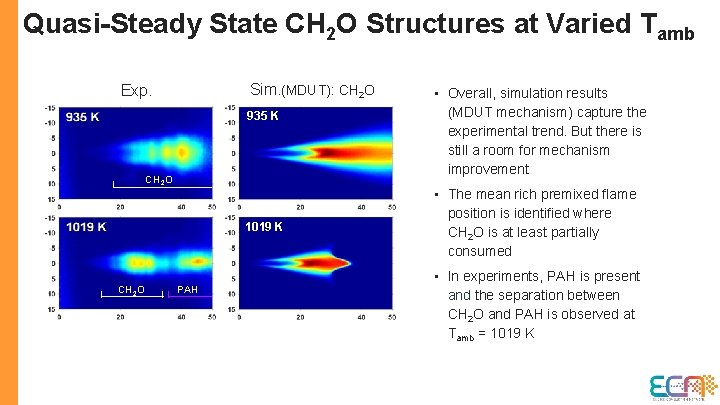
Quasi-Steady State CH 2 O Structures at Varied Tamb Sim. (MDUT): CH 2 O Exp. 935 K CH 2 O 1019 K CH 2 O PAH • Overall, simulation results (MDUT mechanism) capture the experimental trend. But there is still a room for mechanism improvement • The mean rich premixed flame position is identified where CH 2 O is at least partially consumed • In experiments, PAH is present and the separation between CH 2 O and PAH is observed at Tamb = 1019 K
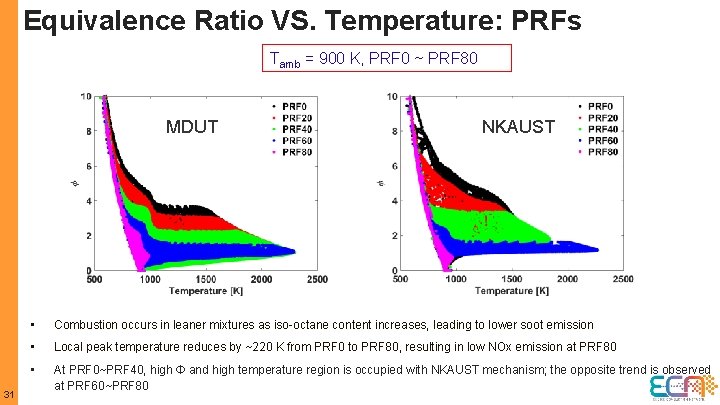
Equivalence Ratio VS. Temperature: PRFs Tamb = 900 K, PRF 0 ~ PRF 80 MDUT 31 NKAUST • Combustion occurs in leaner mixtures as iso-octane content increases, leading to lower soot emission • Local peak temperature reduces by ~220 K from PRF 0 to PRF 80, resulting in low NOx emission at PRF 80 • At PRF 0~PRF 40, high Ф and high temperature region is occupied with NKAUST mechanism; the opposite trend is observed at PRF 60~PRF 80
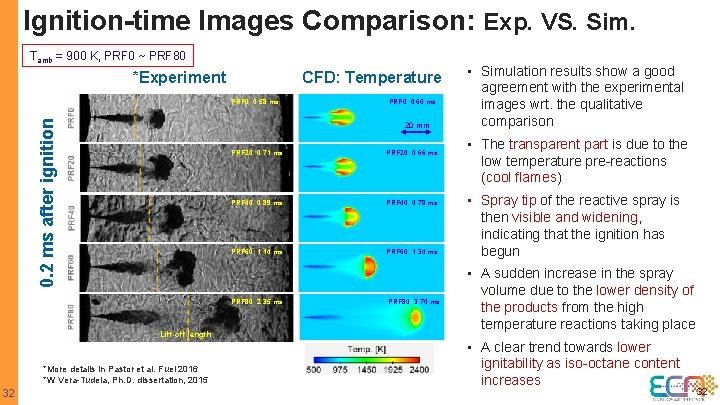
Ignition-time Images Comparison: Exp. VS. Sim. Tamb = 900 K, PRF 0 ~ PRF 80 *Experiment CFD: Temperature 0. 2 ms after ignition PRF 0: 0. 58 ms 20 mm PRF 20: 0. 71 ms PRF 20: 0. 66 ms PRF 40: 0. 89 ms PRF 40: 0. 78 ms PRF 60: 1. 14 ms PRF 60: 1. 34 ms PRF 80: 2. 35 ms Lift-off length *More details in Pastor et al. Fuel 2016 *W Vera-Tudela, Ph. D. dissertation, 2015 32 PRF 0: 0. 60 ms PRF 80: 3. 70 ms • Simulation results show a good agreement with the experimental images wrt. the qualitative comparison • The transparent part is due to the low temperature pre-reactions (cool flames) • Spray tip of the reactive spray is then visible and widening, indicating that the ignition has begun • A sudden increase in the spray volume due to the lower density of the products from the high temperature reactions taking place • A clear trend towards lower ignitability as iso-octane content increases 32
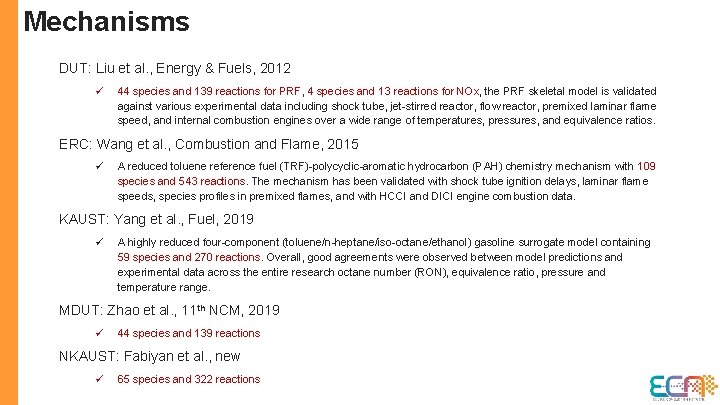
Mechanisms DUT: Liu et al. , Energy & Fuels, 2012 ü 44 species and 139 reactions for PRF, 4 species and 13 reactions for NOx, the PRF skeletal model is validated against various experimental data including shock tube, jet-stirred reactor, flow reactor, premixed laminar flame speed, and internal combustion engines over a wide range of temperatures, pressures, and equivalence ratios. ERC: Wang et al. , Combustion and Flame, 2015 ü A reduced toluene reference fuel (TRF)-polycyclic-aromatic hydrocarbon (PAH) chemistry mechanism with 109 species and 543 reactions. The mechanism has been validated with shock tube ignition delays, laminar flame speeds, species profiles in premixed flames, and with HCCI and DICI engine combustion data. KAUST: Yang et al. , Fuel, 2019 ü A highly reduced four-component (toluene/n-heptane/iso-octane/ethanol) gasoline surrogate model containing 59 species and 270 reactions. Overall, good agreements were observed between model predictions and experimental data across the entire research octane number (RON), equivalence ratio, pressure and temperature range. MDUT: Zhao et al. , 11 th NCM, 2019 ü 44 species and 139 reactions NKAUST: Fabiyan et al. , new ü 65 species and 322 reactions
Back work ratio formula
Safety and industrial security aramco
Abis saudi arabia
Universal electronic ignition south africa
Ignition extinction curve
Dtsi engine disadvantages
Dtsi engine advantages & disadvantages
Ignition coil windings
Chapter 10 ignition systems
7284u
Two technicians are discussing electromagnetic induction
Ignition timing diagram
Affordable ignition interlock
Ignition armature definition
Chapter 10 ignition systems
Blast off second ignition
Chapter 34 ignition system technology
Small engine ignition system
Sepasoft ignition
Hei and eis are examples of
Ignition interlock missouri
Ignition delay
Ignition temperature
Cheapest ignition interlock device
Experimental study of hydrogen release and ignition from
Ignition sql
Smart start breathalyzer hack
Ignition system adalah
An ignition coil operates using the principle of
Ignition interlock device program
Ignition remote tag provider
Minimum ignition current
Initially all rocks on earth were
Leandra's law