Finitesize effects on twoparticle production in continuous and
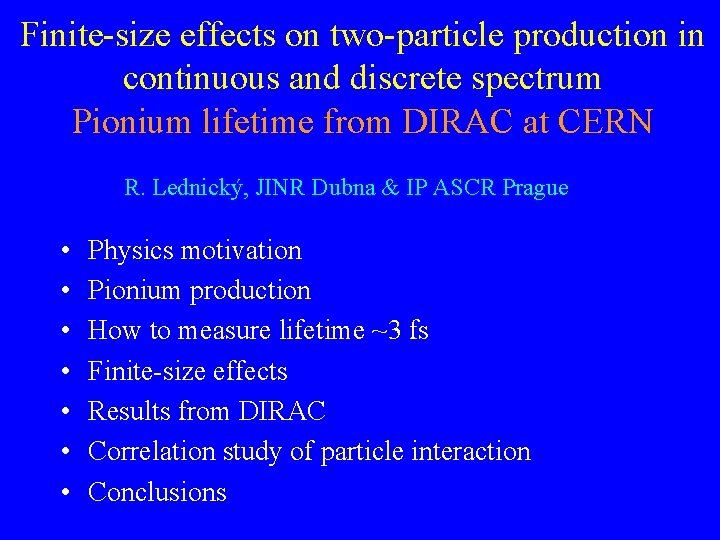
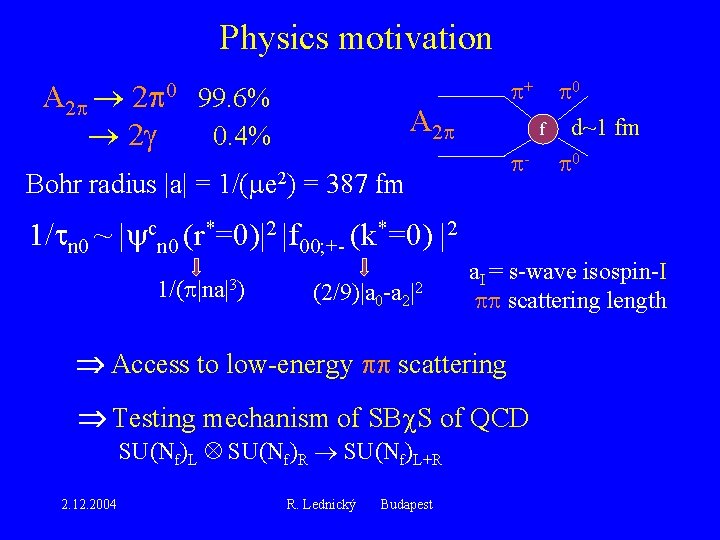
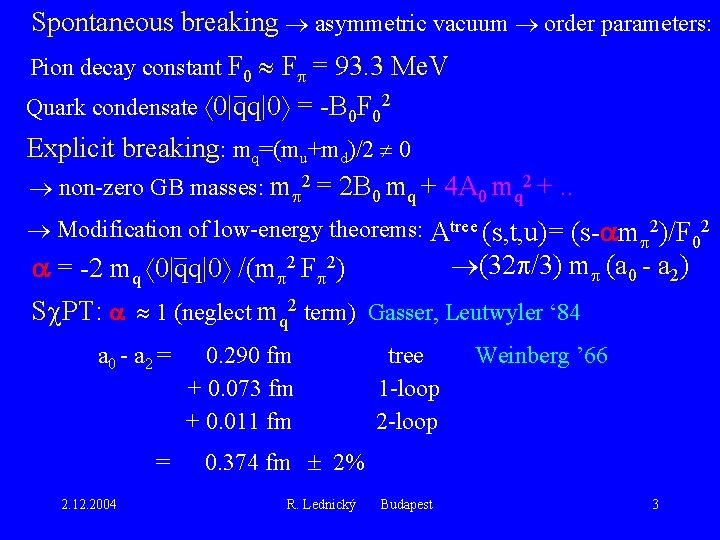
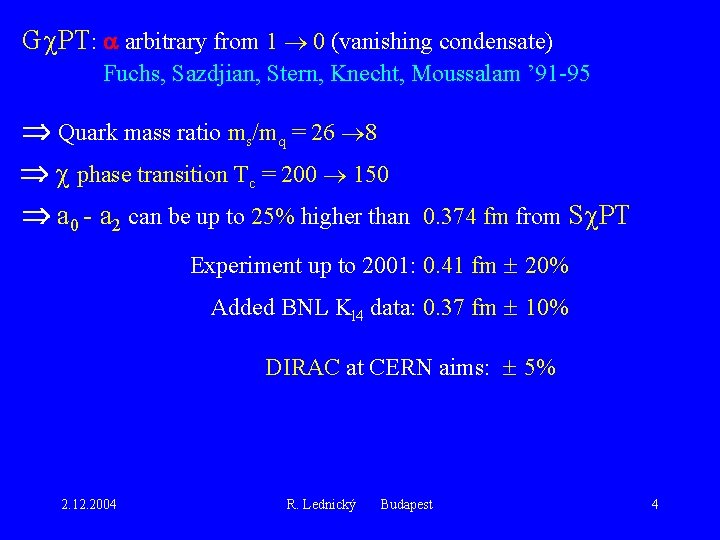
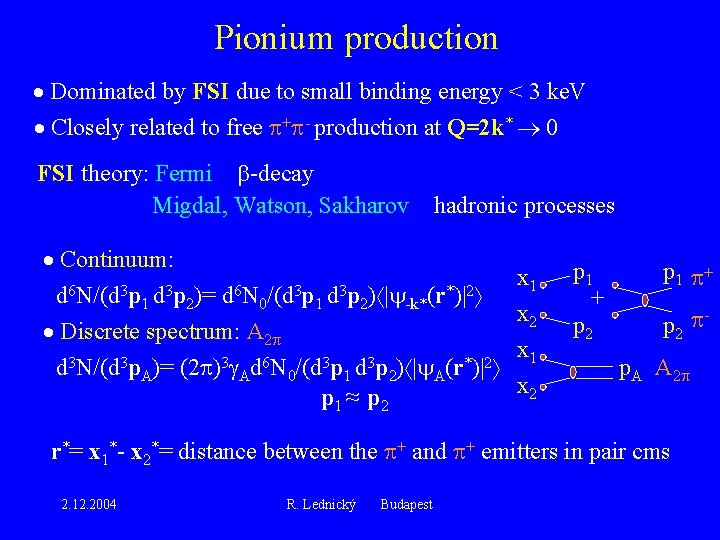
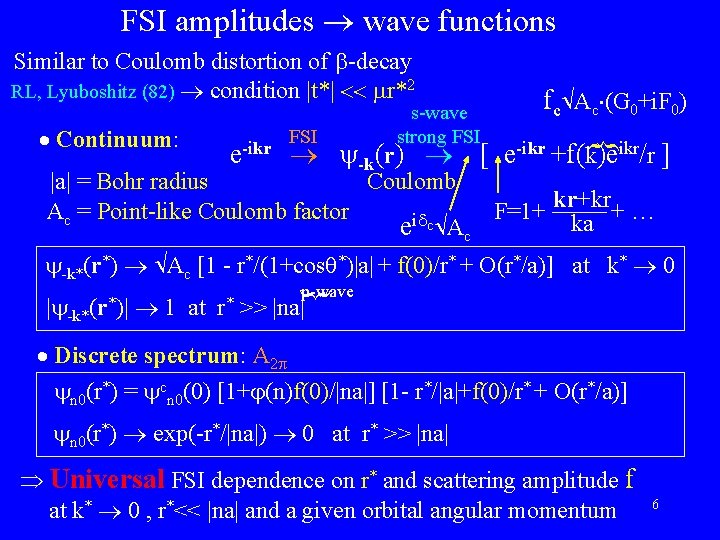
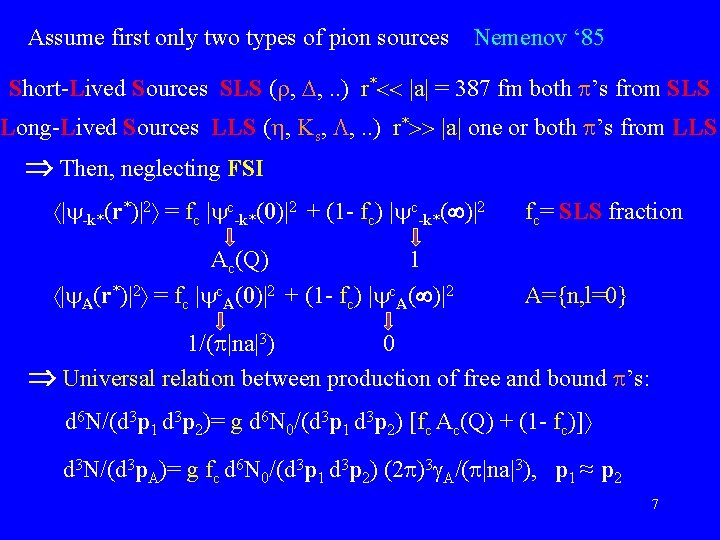
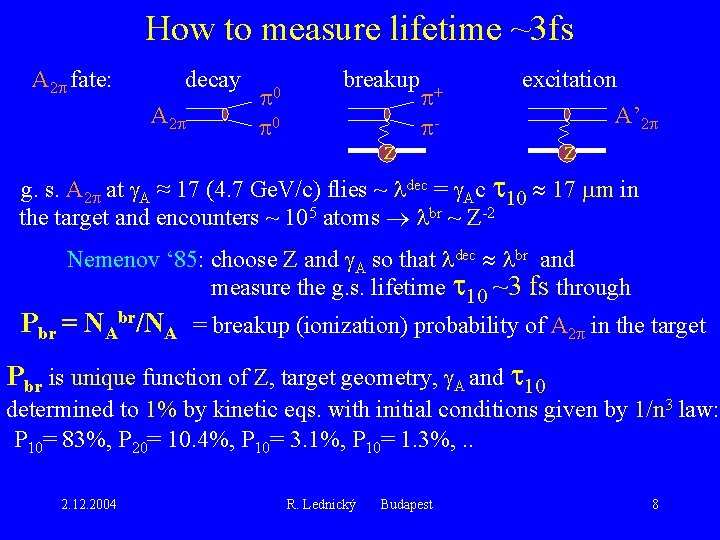
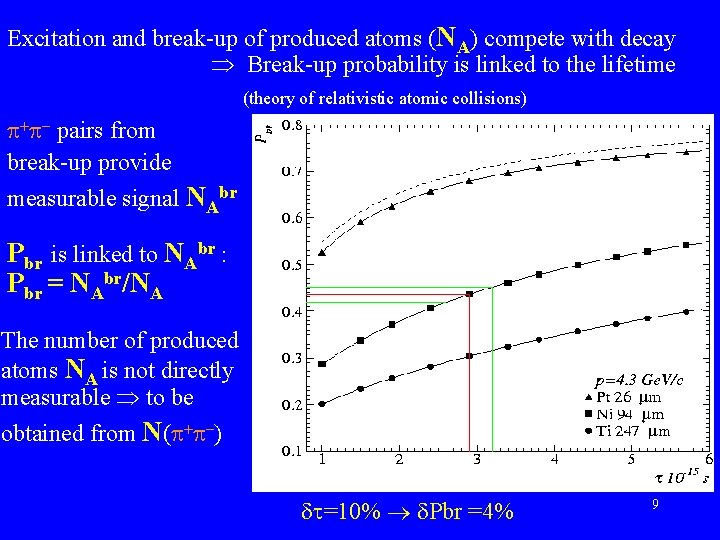
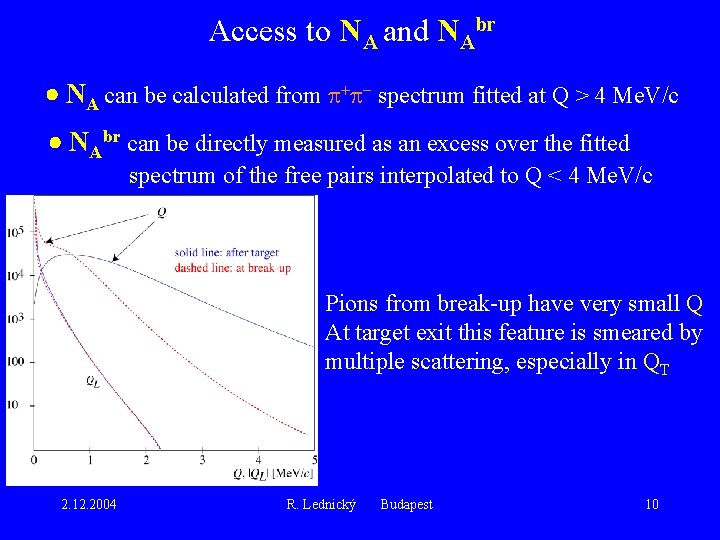
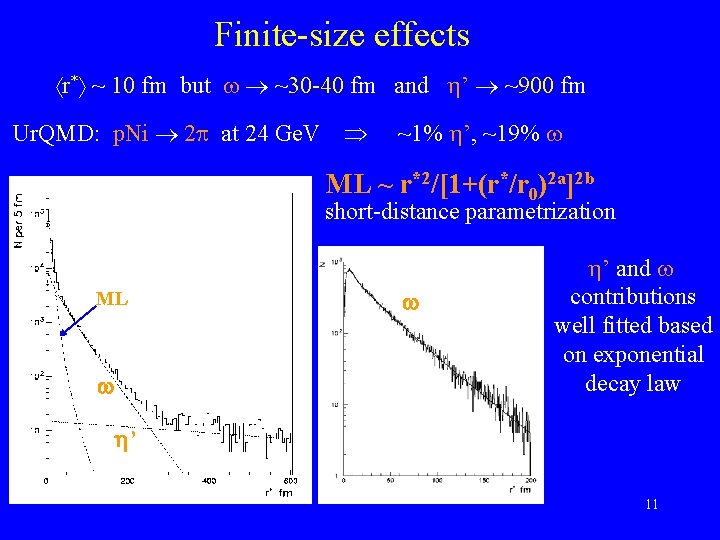
![DIRAC - - CF: CF=N{ | -k*(r*)|2 SLS +(1 - )}[1+s Q] SLS determined DIRAC - - CF: CF=N{ | -k*(r*)|2 SLS +(1 - )}[1+s Q] SLS determined](https://slidetodoc.com/presentation_image_h2/4499b46858b10c5712d5eb44ca3c1e5f/image-12.jpg)
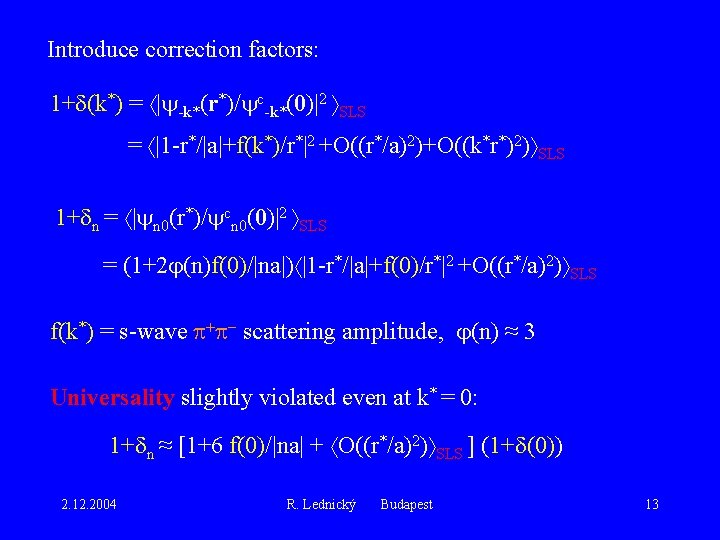
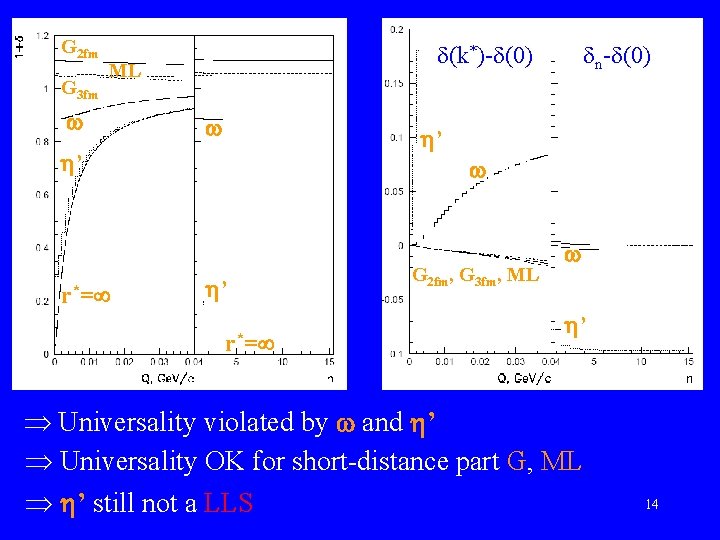
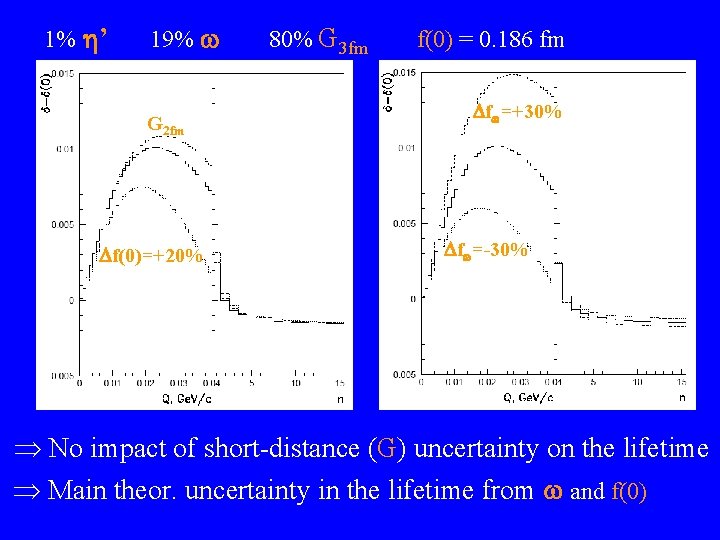
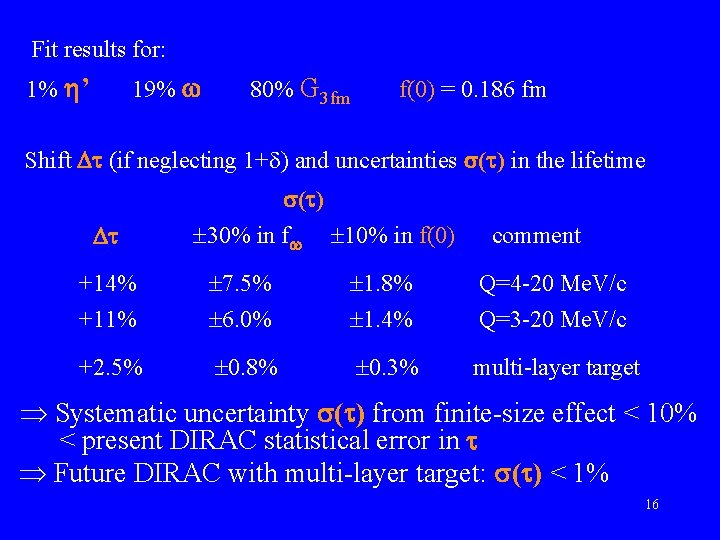
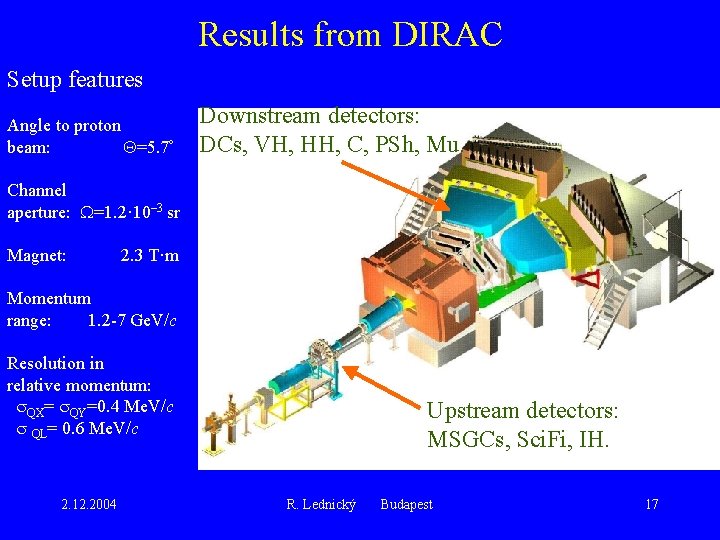
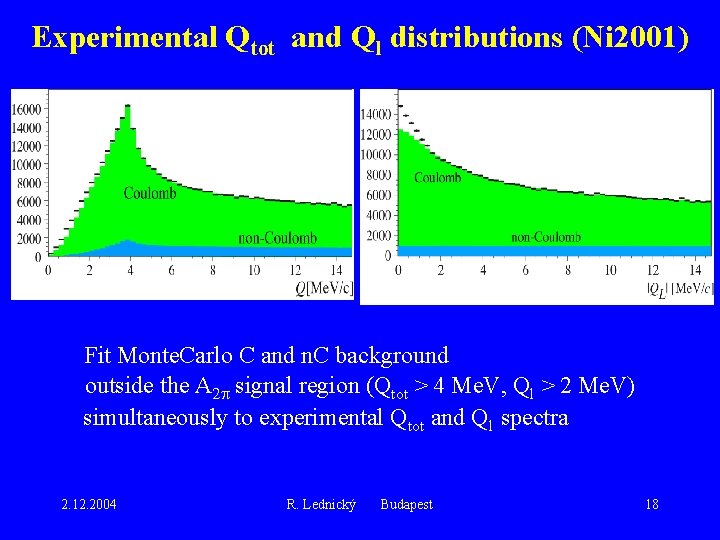
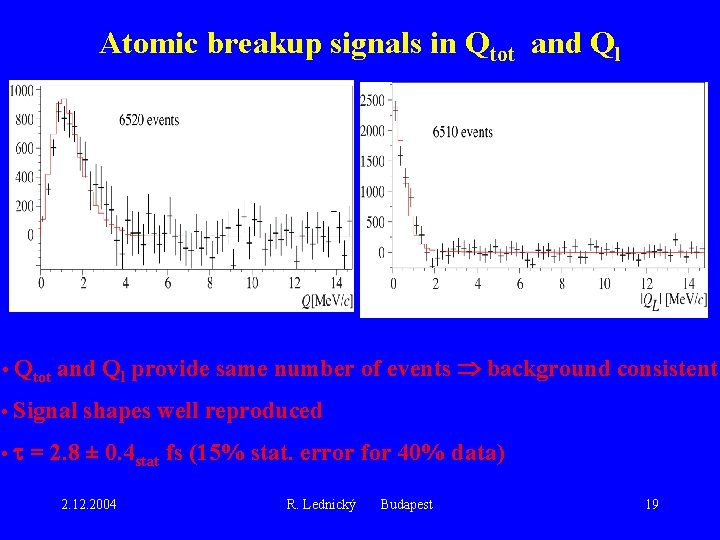
![Correlation study of particle interaction CF=Norm [Purity RQMD(r* Scale r*)+1 -Purity] + scattering length Correlation study of particle interaction CF=Norm [Purity RQMD(r* Scale r*)+1 -Purity] + scattering length](https://slidetodoc.com/presentation_image_h2/4499b46858b10c5712d5eb44ca3c1e5f/image-20.jpg)
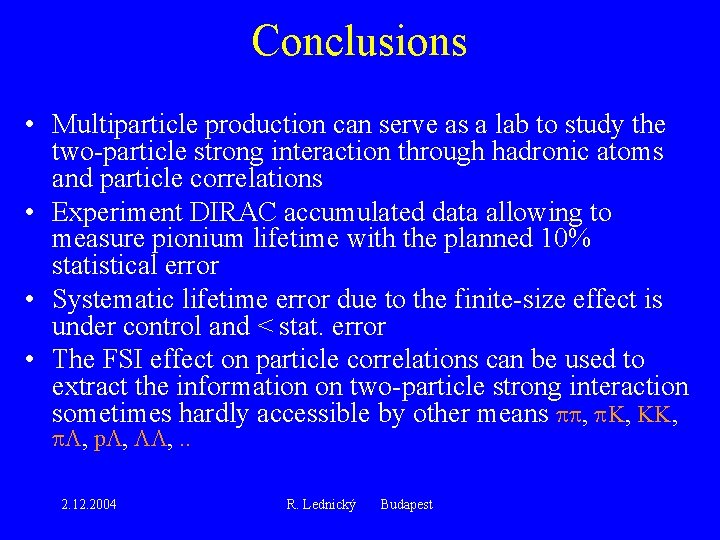
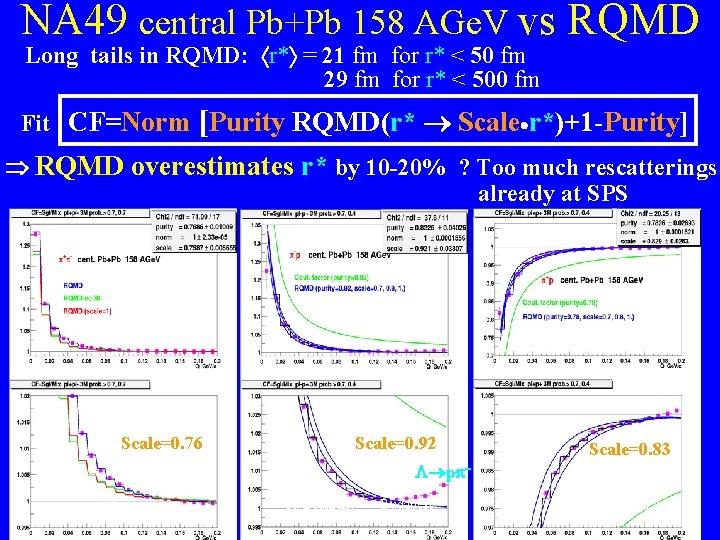
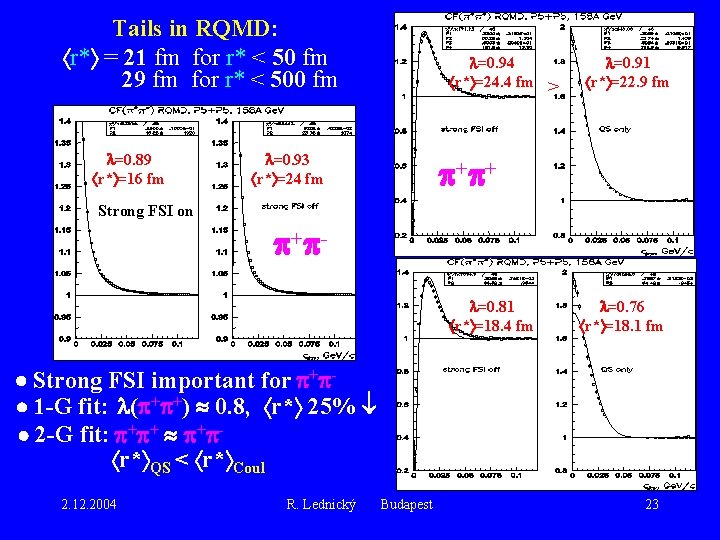
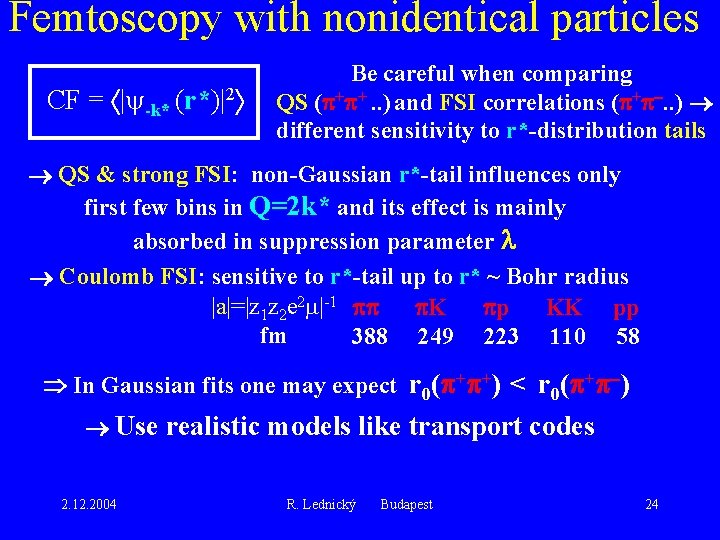
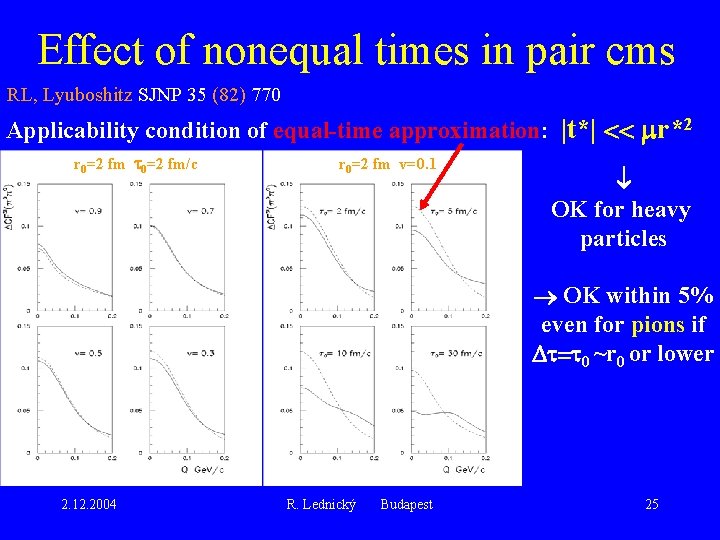
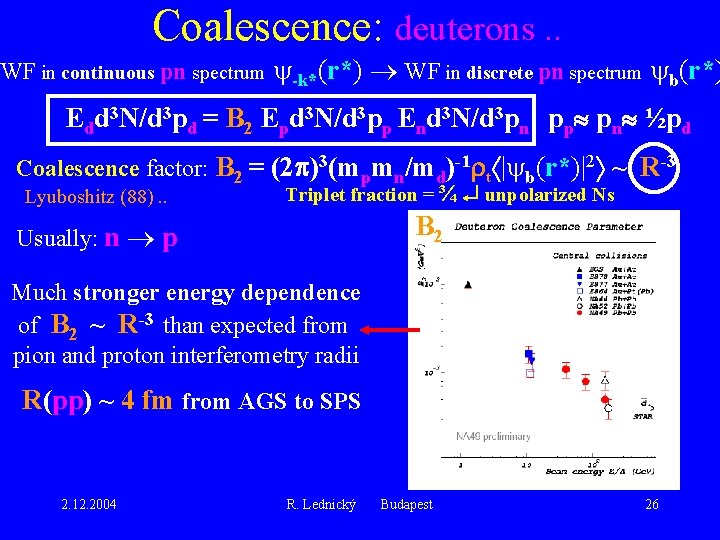
- Slides: 26
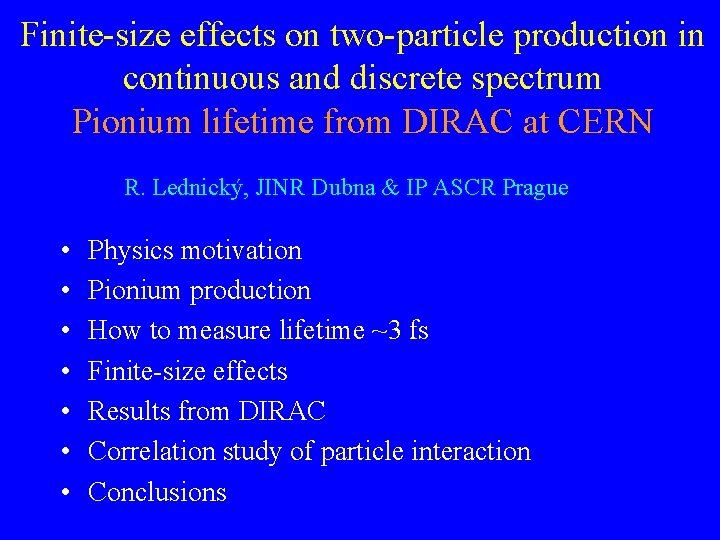
Finite-size effects on two-particle production in continuous and discrete spectrum Pionium lifetime from DIRAC at CERN R. Lednický, JINR Dubna & IP ASCR Prague • • Physics motivation Pionium production How to measure lifetime ~3 fs Finite-size effects Results from DIRAC Correlation study of particle interaction Conclusions
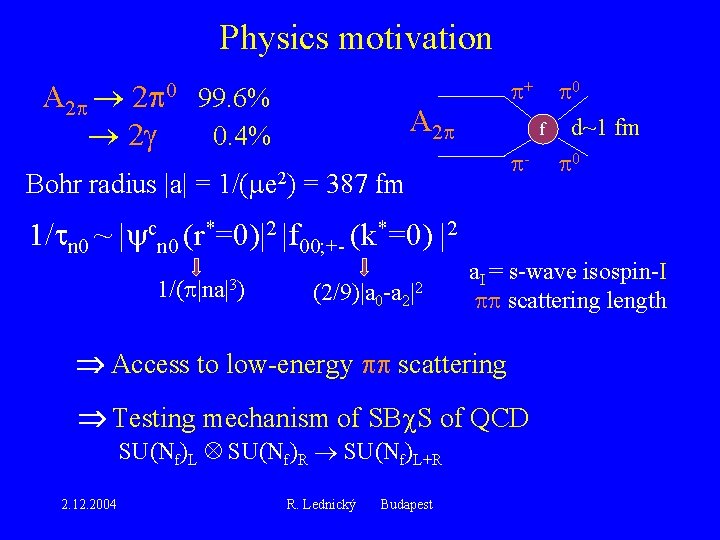
Physics motivation A 2 2 0 99. 6% 2 0. 4% + A 2 0 f - Bohr radius |a| = 1/( e 2) = 387 fm d~1 fm 0 1/ n 0 ~ | cn 0 (r*=0)|2 |f 00; +- (k*=0) |2 1/( |na|3) (2/9)|a 0 -a 2|2 a. I = s-wave isospin-I scattering length Access to low-energy scattering Testing mechanism of SB S of QCD SU(Nf)L SU(Nf)R SU(Nf)L+R 2. 12. 2004 R. Lednický Budapest
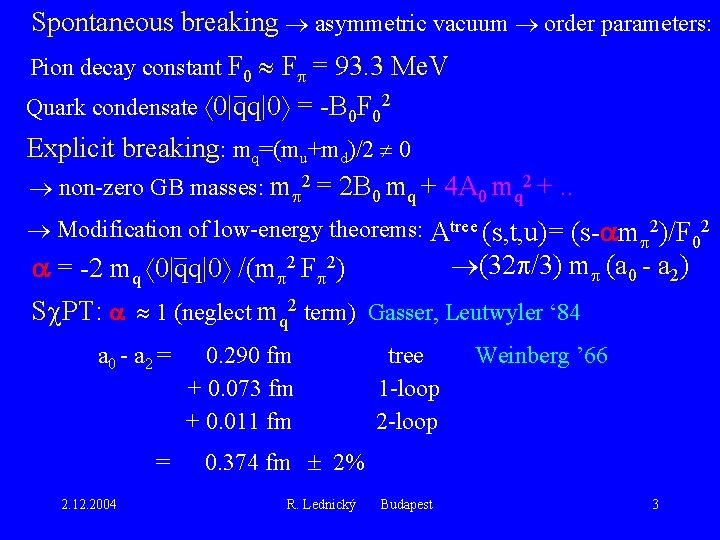
Spontaneous breaking asymmetric vacuum order parameters: Pion decay constant F 0 F = 93. 3 Me. V Quark condensate 0|qq|0 = -B 0 F 02 Explicit breaking: mq=(mu+md)/2 0 non-zero GB masses: m 2 = 2 B 0 mq + 4 A 0 mq 2 +. . Modification of low-energy theorems: Atree (s, t, u)= (s- m 2)/F 02 (32 /3) m (a 0 - a 2) = -2 mq 0|qq|0 /(m 2 F 2) S PT: 1 (neglect mq 2 term) Gasser, Leutwyler ‘ 84 a 0 - a 2 = = 2. 12. 2004 0. 290 fm + 0. 073 fm + 0. 011 fm tree 1 -loop 2 -loop Weinberg ’ 66 0. 374 fm 2% R. Lednický Budapest 3
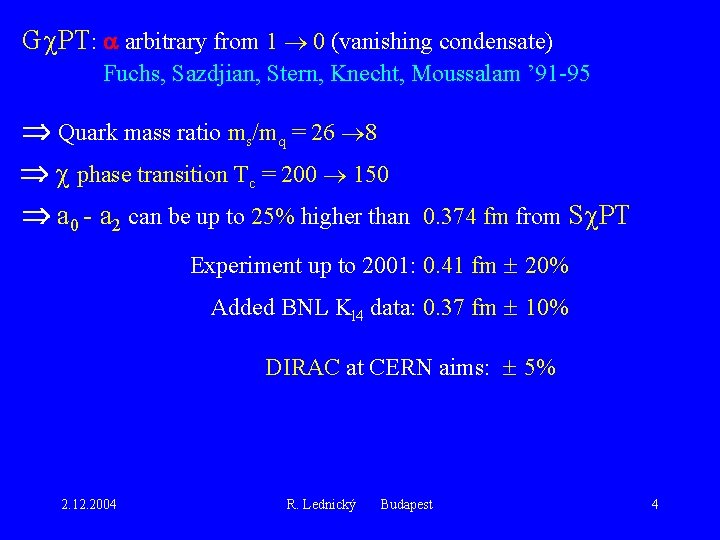
G PT: arbitrary from 1 0 (vanishing condensate) Fuchs, Sazdjian, Stern, Knecht, Moussalam ’ 91 -95 Quark mass ratio ms/mq = 26 8 phase transition Tc = 200 150 a 0 - a 2 can be up to 25% higher than 0. 374 fm from S PT Experiment up to 2001: 0. 41 fm 20% Added BNL Kl 4 data: 0. 37 fm 10% DIRAC at CERN aims: 5% 2. 12. 2004 R. Lednický Budapest 4
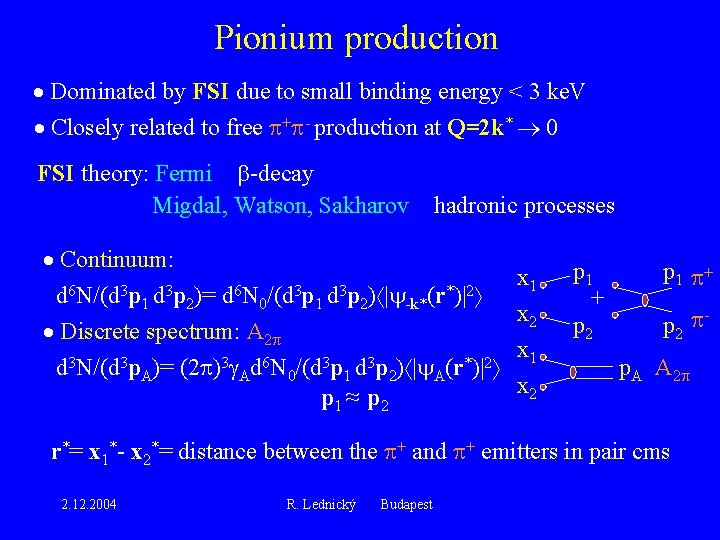
Pionium production Dominated by FSI due to small binding energy < 3 ke. V Closely related to free + - production at Q=2 k* 0 FSI theory: Fermi -decay Migdal, Watson, Sakharov hadronic processes Continuum: d 6 N/(d 3 p 1 d 3 p 2)= d 6 N 0/(d 3 p 1 d 3 p 2) | -k*(r*)|2 Discrete spectrum: A 2 d 3 N/(d 3 p. A)= (2 )3 Ad 6 N 0/(d 3 p 1 d 3 p 2) | A(r*)|2 p 1 ≈ p 2 x 1 x 2 p 1 + p 2 p. A A 2 r*= x 1*- x 2*= distance between the + and + emitters in pair cms 2. 12. 2004 R. Lednický Budapest
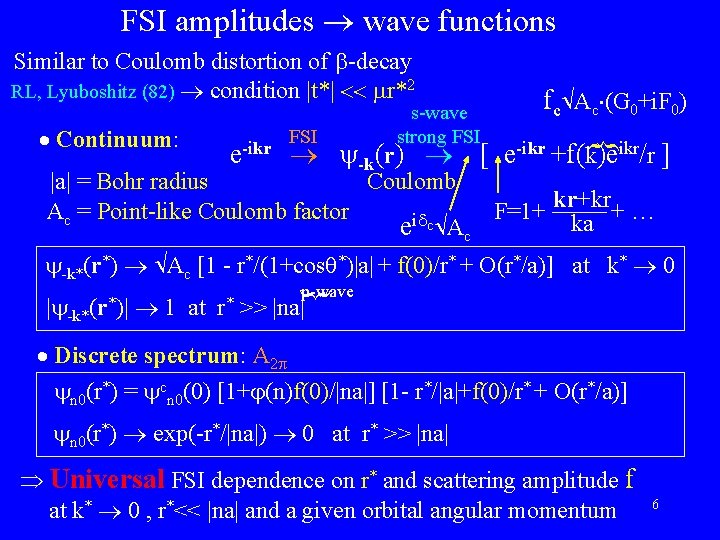
FSI amplitudes wave functions Continuum: e-ikr FSI s-wave strong FSI -k(r) fc Ac (G 0+i. F 0) } Similar to Coulomb distortion of -decay RL, Lyuboshitz (82) condition |t*| r*2 [ e-ikr +f(k)eikr/r ] |a| = Bohr radius Coulomb kr+kr+ … _______ Ac = Point-like Coulomb factor F=1+ ka ei c Ac -k*(r*) Ac [1 - r*/(1+cos *)|a| + f(0)/r* + O(r*/a)] at k* 0 1 at r* p-wave | -k*(r*)| >> |na| Discrete spectrum: A 2 n 0(r*) = cn 0(0) [1+ (n)f(0)/|na|] [1 - r*/|a|+f(0)/r* + O(r*/a)] n 0(r*) exp(-r*/|na|) 0 at r* >> |na| Universal FSI dependence on r* and scattering amplitude f at k* 0 , r*<< |na| and a given orbital angular momentum 6
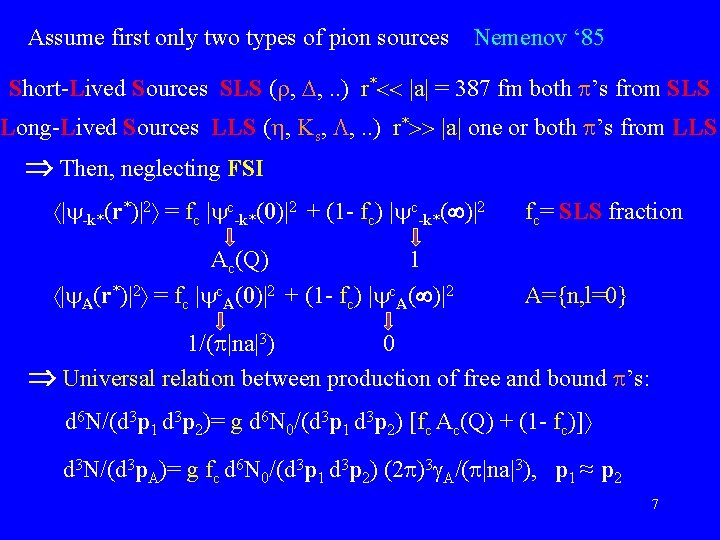
Assume first only two types of pion sources Nemenov ‘ 85 Short-Lived Sources SLS ( , , . . ) r* |a| = 387 fm both ’s from SLS Long-Lived Sources LLS ( , Ks, , . . ) r* |a| one or both ’s from LLS Then, neglecting FSI | -k*(r*)|2 = fc | c-k*(0)|2 + (1 - fc) | c-k*( )|2 fc= SLS fraction Ac(Q) 1 | A(r*)|2 = fc | c. A(0)|2 + (1 - fc) | c. A( )|2 A={n, l=0} 1/( |na|3) 0 Universal relation between production of free and bound ’s: d 6 N/(d 3 p 1 d 3 p 2)= g d 6 N 0/(d 3 p 1 d 3 p 2) [fc Ac(Q) + (1 - fc)] d 3 N/(d 3 p. A)= g fc d 6 N 0/(d 3 p 1 d 3 p 2) (2 )3 A/( |na|3), p 1 ≈ p 2 7
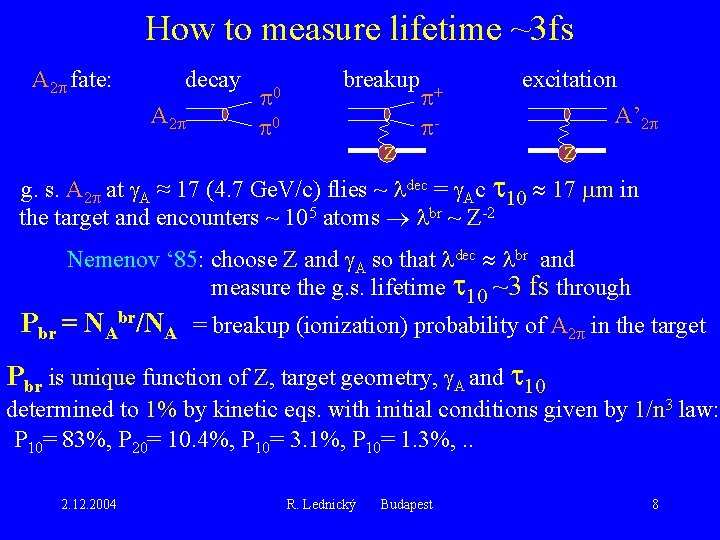
How to measure lifetime ~3 fs A 2 fate: decay A 2 0 breakup 0 + - excitation A’ 2 Z Z g. s. A 2 at A ≈ 17 (4. 7 Ge. V/c) flies ~ dec = Ac 10 17 m in the target and encounters ~ 105 atoms br ~ Z-2 Nemenov ‘ 85: choose Z and A so that dec br and measure the g. s. lifetime 10 ~3 fs through Pbr = NAbr/NA = breakup (ionization) probability of A 2 in the target Pbr is unique function of Z, target geometry, A and 10 determined to 1% by kinetic eqs. with initial conditions given by 1/n 3 law: P 10= 83%, P 20= 10. 4%, P 10= 3. 1%, P 10= 1. 3%, . . 2. 12. 2004 R. Lednický Budapest 8
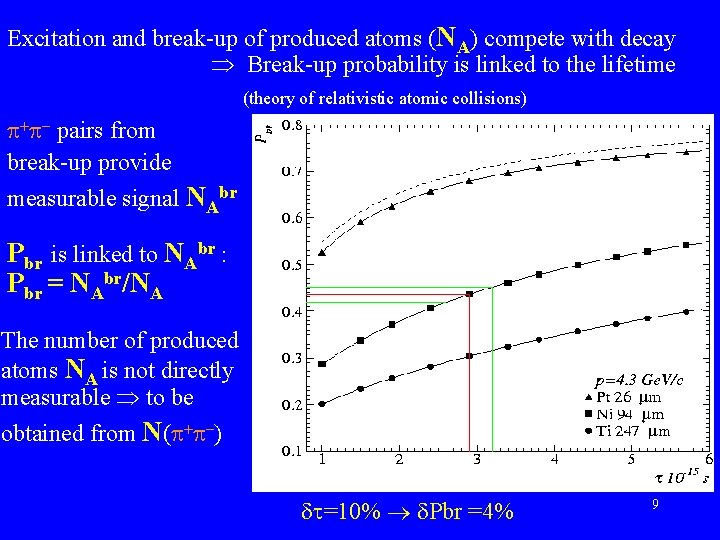
Excitation and break-up of produced atoms (NA) compete with decay Break-up probability is linked to the lifetime (theory of relativistic atomic collisions) + - pairs from break-up provide measurable signal NAbr Pbr is linked to NAbr : Pbr = NAbr/NA The number of produced atoms NA is not directly measurable to be obtained from N( + -) =10% Pbr =4% 9
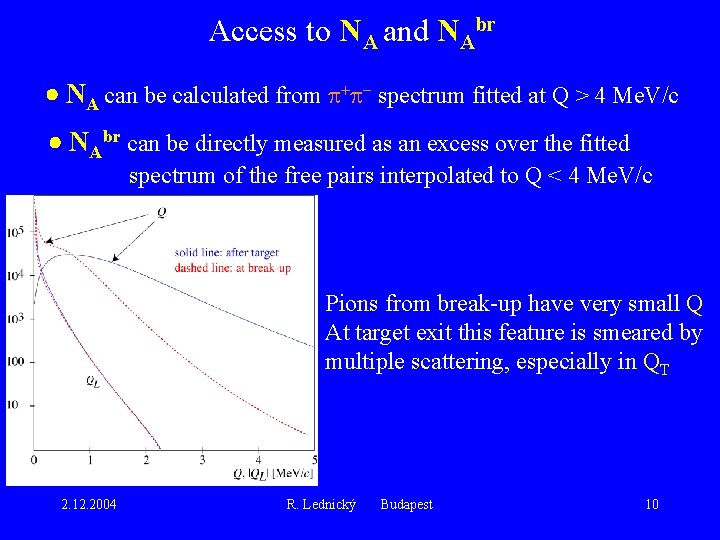
Access to NA and NAbr NA can be calculated from + - spectrum fitted at Q > 4 Me. V/c NAbr can be directly measured as an excess over the fitted spectrum of the free pairs interpolated to Q < 4 Me. V/c Pions from break-up have very small Q At target exit this feature is smeared by multiple scattering, especially in QT 2. 12. 2004 R. Lednický Budapest 10
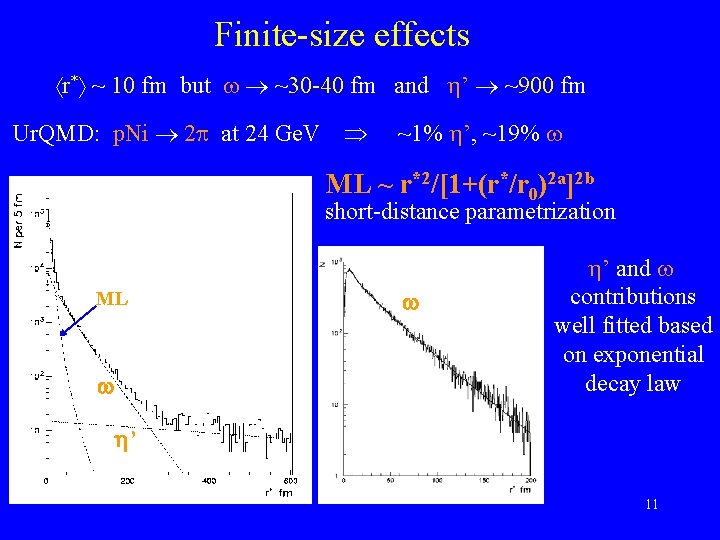
Finite-size effects r* ~ 10 fm but ~30 -40 fm and ’ ~900 fm Ur. QMD: p. Ni 2 at 24 Ge. V ~1% ’, ~19% ML ~ r*2/[1+(r*/r 0)2 a]2 b short-distance parametrization ML ’ and contributions well fitted based on exponential decay law ’ 11
![DIRAC CF CFN kr2 SLS 1 1s Q SLS determined DIRAC - - CF: CF=N{ | -k*(r*)|2 SLS +(1 - )}[1+s Q] SLS determined](https://slidetodoc.com/presentation_image_h2/4499b46858b10c5712d5eb44ca3c1e5f/image-12.jpg)
DIRAC - - CF: CF=N{ | -k*(r*)|2 SLS +(1 - )}[1+s Q] SLS determined by: N r 0 fm a b f f ’ s f = 17 6% ’, f ’ , f ML(r 0, a, b), f. ML=1 -f -f ’ G 2 fm ML G 3 fm ’ 12
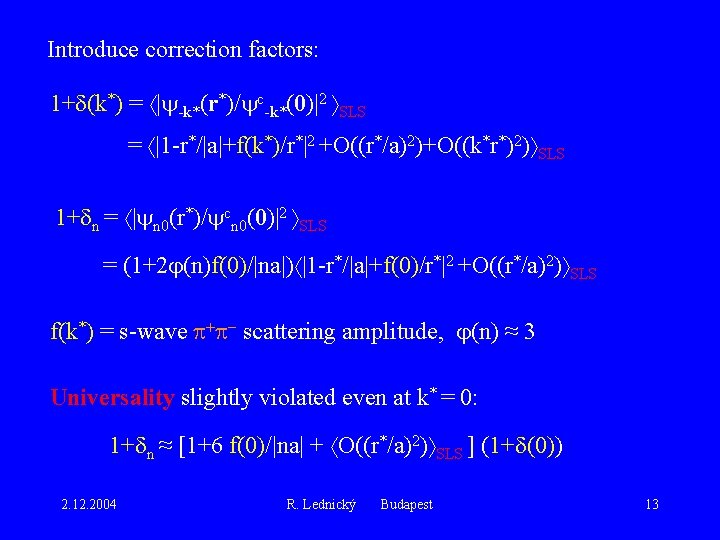
Introduce correction factors: 1+ (k*) = | -k*(r*)/ c-k*(0)|2 SLS = |1 -r*/|a|+f(k*)/r*|2 +O((r*/a)2)+O((k*r*)2) SLS 1+ n = | n 0(r*)/ cn 0(0)|2 SLS = (1+2 (n)f(0)/|na|) |1 -r*/|a|+f(0)/r*|2 +O((r*/a)2) SLS f(k*) = s-wave + - scattering amplitude, (n) ≈ 3 Universality slightly violated even at k* = 0: 1+ n ≈ [1+6 f(0)/|na| + O((r*/a)2) SLS ] (1+ (0)) 2. 12. 2004 R. Lednický Budapest 13
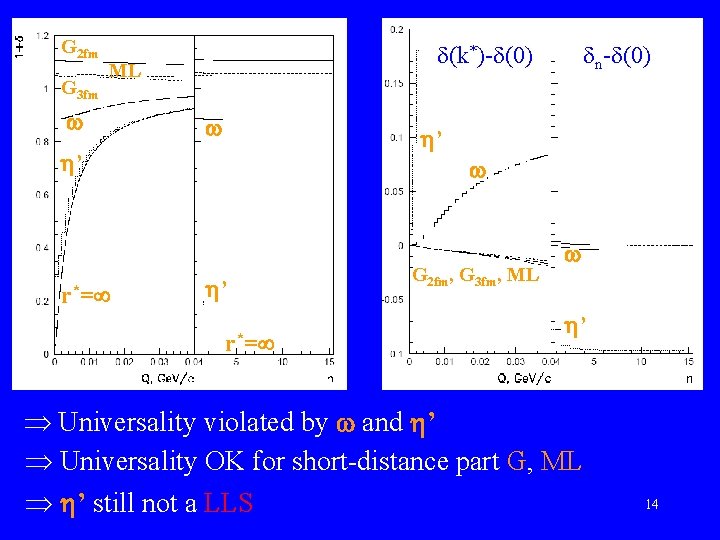
G 2 fm G 3 fm (k*)- (0) ML ’ ’ r*= n- (0) ’ r*= G 2 fm, G 3 fm, ML ’ Universality violated by and ’ Universality OK for short-distance part G, ML ’ still not a LLS 14
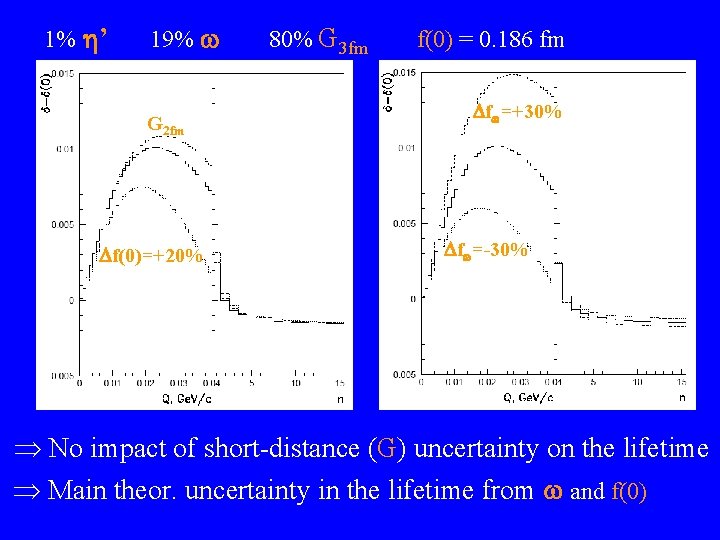
1% ’ 19% G 2 fm f(0)=+20% 80% G 3 fm f(0) = 0. 186 fm f =+30% f =-30% No impact of short-distance (G) uncertainty on the lifetime Main theor. uncertainty in the lifetime from and f(0)
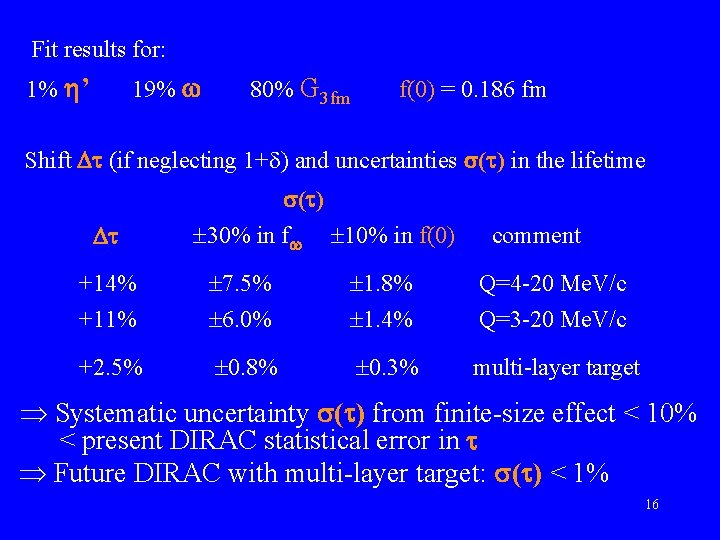
Fit results for: 1% ’ 19% 80% G 3 fm f(0) = 0. 186 fm Shift (if neglecting 1+ ) and uncertainties ( ) in the lifetime ( ) 30% in f 10% in f(0) comment +14% +11% 7. 5% 6. 0% 1. 8% 1. 4% Q=4 -20 Me. V/c Q=3 -20 Me. V/c +2. 5% 0. 8% 0. 3% multi-layer target Systematic uncertainty ( ) from finite-size effect < 10% < present DIRAC statistical error in Future DIRAC with multi-layer target: ( ) < 1% 16
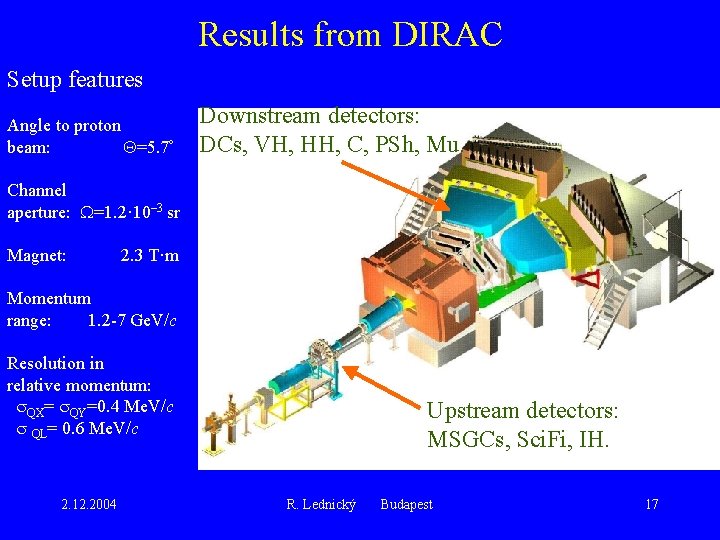
Results from DIRAC Setup features Angle to proton beam: =5. 7 Downstream detectors: DCs, VH, HH, C, PSh, Mu. Channel aperture: =1. 2· 10– 3 sr Magnet: 2. 3 T·m Momentum range: 1. 2 -7 Ge. V/c Resolution in relative momentum: QX= QY=0. 4 Me. V/c QL= 0. 6 Me. V/c 2. 12. 2004 Upstream detectors: MSGCs, Sci. Fi, IH. R. Lednický Budapest 17
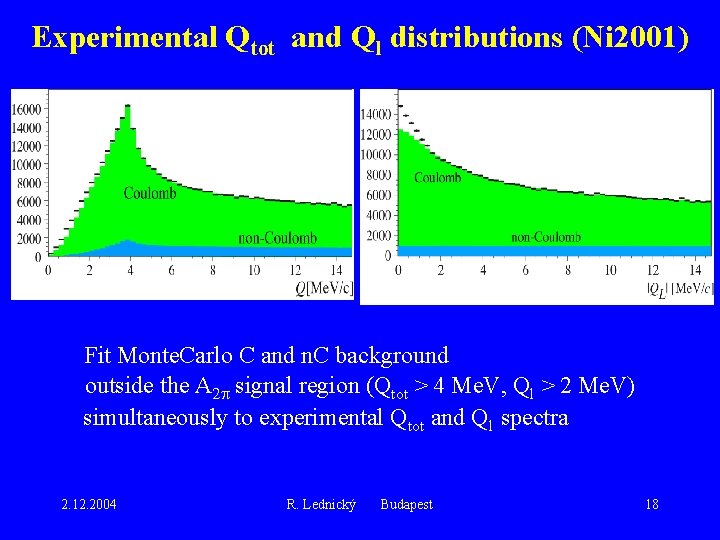
Experimental Qtot and Ql distributions (Ni 2001) Fit Monte. Carlo C and n. C background outside the A 2 signal region (Qtot > 4 Me. V, Ql > 2 Me. V) simultaneously to experimental Qtot and Ql spectra 2. 12. 2004 R. Lednický Budapest 18
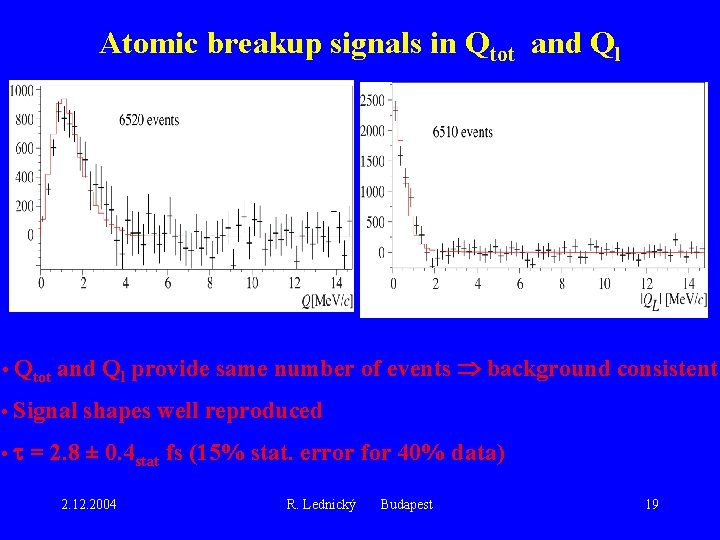
Atomic breakup signals in Qtot and Ql • Qtot and Ql provide same number of events background consistent • Signal shapes well reproduced • = 2. 8 ± 0. 4 stat fs (15% stat. error for 40% data) 2. 12. 2004 R. Lednický Budapest 19
![Correlation study of particle interaction CFNorm Purity RQMDr Scale r1 Purity scattering length Correlation study of particle interaction CF=Norm [Purity RQMD(r* Scale r*)+1 -Purity] + scattering length](https://slidetodoc.com/presentation_image_h2/4499b46858b10c5712d5eb44ca3c1e5f/image-20.jpg)
Correlation study of particle interaction CF=Norm [Purity RQMD(r* Scale r*)+1 -Purity] + scattering length f 0 from NA 49 CF + Fit CF( + ) by RQMD with SI scale: f 0 sisca f 0 input -= 0. 232 fm sisca = 0. 6 0. 1 Compare with ~0. 8 from S PT & BNL E 765 K e 20
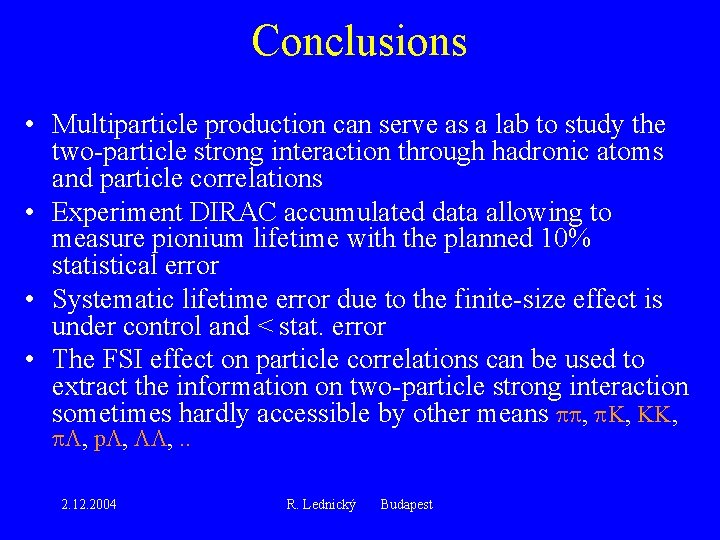
Conclusions • Multiparticle production can serve as a lab to study the two-particle strong interaction through hadronic atoms and particle correlations • Experiment DIRAC accumulated data allowing to measure pionium lifetime with the planned 10% statistical error • Systematic lifetime error due to the finite-size effect is under control and < stat. error • The FSI effect on particle correlations can be used to extract the information on two-particle strong interaction sometimes hardly accessible by other means , K, KK, , p , , . . 2. 12. 2004 R. Lednický Budapest
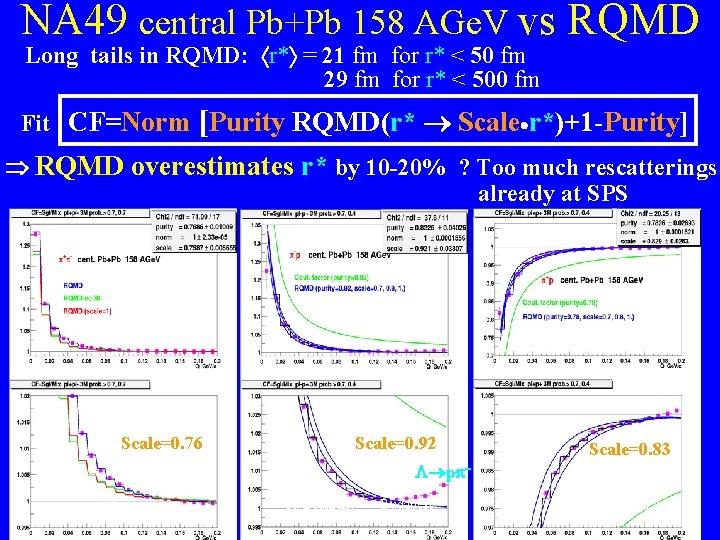
NA 49 central Pb+Pb 158 AGe. V vs RQMD Long tails in RQMD: r* = 21 fm for r* < 50 fm 29 fm for r* < 500 fm Fit CF=Norm [Purity RQMD(r* Scale r*)+1 -Purity] RQMD overestimates r* by 10 -20% ? Too much rescatterings already at SPS Scale=0. 76 Scale=0. 92 Scale=0. 83 p 2. 12. 2004 R. Lednický Budapest 22
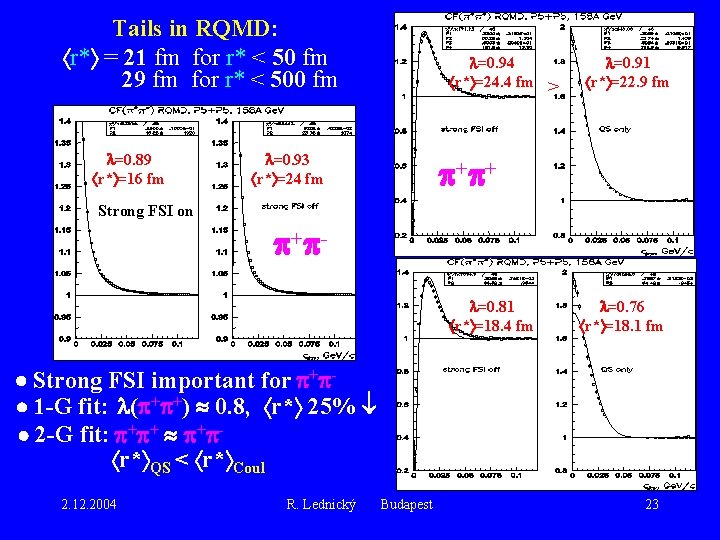
Tails in RQMD: r* = 21 fm for r* < 50 fm 29 fm for r* < 500 fm =0. 89 r* =16 fm =0. 94 r* =24. 4 fm =0. 93 r* =24 fm > =0. 91 r* =22. 9 fm + + Strong FSI on + =0. 81 r* =18. 4 fm =0. 76 r* =18. 1 fm Strong FSI important for + 1 -G fit: ( + +) 0. 8, r* 25% 2 -G fit: + + + r* QS < r* Coul 2. 12. 2004 R. Lednický Budapest 23
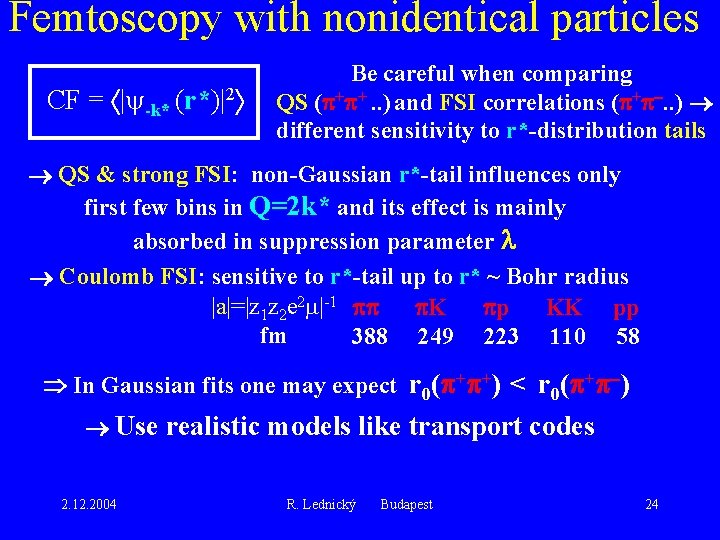
Femtoscopy with nonidentical particles CF = | -k* (r*)|2 Be careful when comparing QS ( + +. . ) and FSI correlations ( + . . ) different sensitivity to r*-distribution tails QS & strong FSI: non-Gaussian r*-tail influences only first few bins in Q=2 k* and its effect is mainly absorbed in suppression parameter Coulomb FSI: sensitive to r*-tail up to r* ~ Bohr radius |a|=|z 1 z 2 e 2 |-1 K p KK pp fm 388 249 223 110 58 In Gaussian fits one may expect r 0( + +) < r 0( + ) Use realistic models like transport codes 2. 12. 2004 R. Lednický Budapest 24
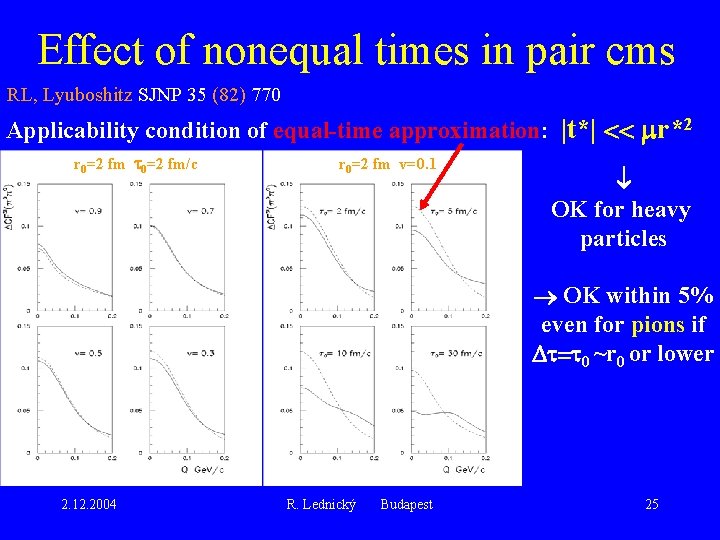
Effect of nonequal times in pair cms RL, Lyuboshitz SJNP 35 (82) 770 Applicability condition of equal-time approximation: |t*| r*2 r 0=2 fm/c r 0=2 fm v=0. 1 OK for heavy particles OK within 5% even for pions if 0 ~r 0 or lower 2. 12. 2004 R. Lednický Budapest 25
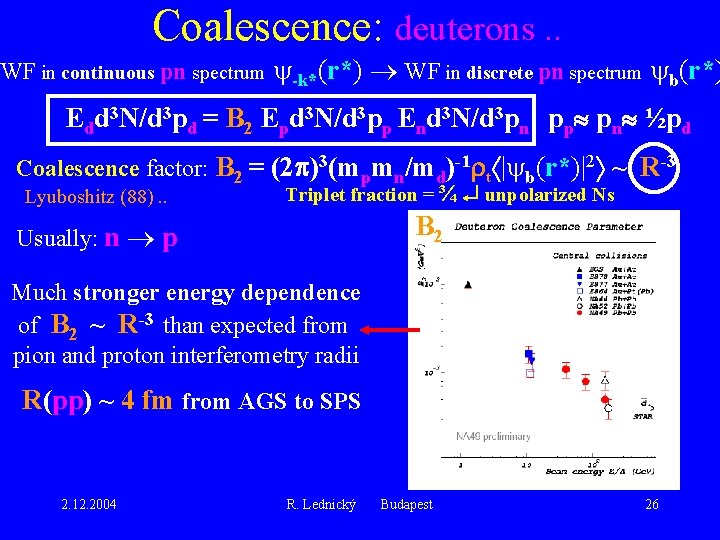
Coalescence: deuterons. . WF in continuous pn spectrum -k*(r*) WF in discrete pn spectrum b(r*) Edd 3 N/d 3 pd = B 2 Epd 3 N/d 3 pp End 3 N/d 3 pn pp pn ½pd Coalescence factor: B 2 = (2 )3(mpmn/md)-1 t | b(r*)|2 ~ R-3 Triplet fraction = ¾ unpolarized Ns Lyuboshitz (88). . B 2 Usually: n p Much stronger energy dependence of B 2 ~ R-3 than expected from pion and proton interferometry radii R(pp) ~ 4 fm from AGS to SPS 2. 12. 2004 R. Lednický Budapest 26
Alur produksi multimedia
Simple future future continuous
Past simple present simple future simple
Past continuous
Past continuous past progressive
Past simple present perfect past continuous
Future perfect by the time
Past perfect schema
Spreganje glagola biti
Past simple past continuous past perfect
Jitsmi
Continuous auditing continuous monitoring
Past simple past continuous past perfect
Future perfect future continuous future perfect continuous
Present perfect continuous auxiliary verb
Present simple past simple future simple present continuous
Causes and effects of the french and indian war
Inventory management and production planning and scheduling
Primary and secondary effects of a tectonic hazard
Pinotubo
Toe-in and toe-out effects
Causes and effects of the russian revolution
Causes and effects of french revolution
Negative effects of cotton gin
Immediate cause of french revolution
Causes and effects of french revolution
Positive and negative effects of television