Ecole Polytechnique Fdrale de Lausanne EPFL 3 D2
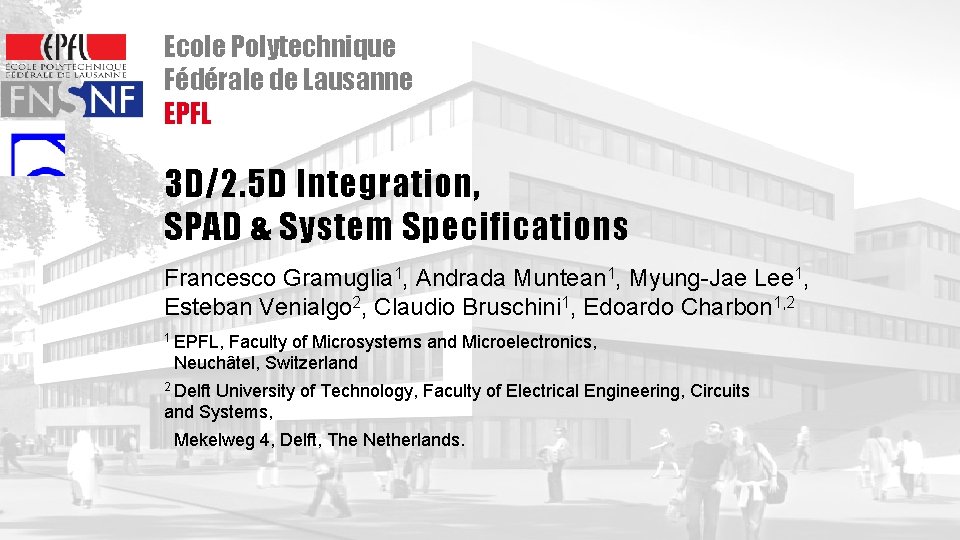
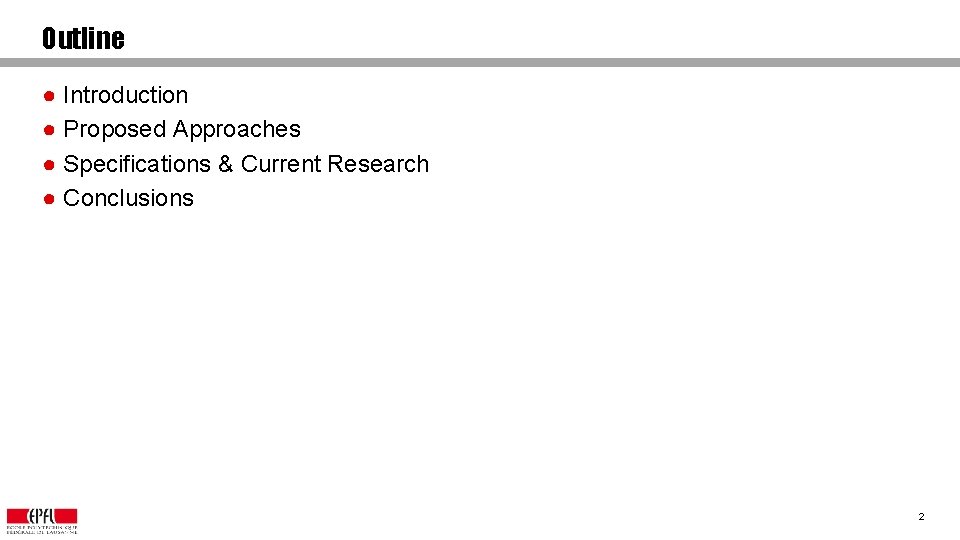
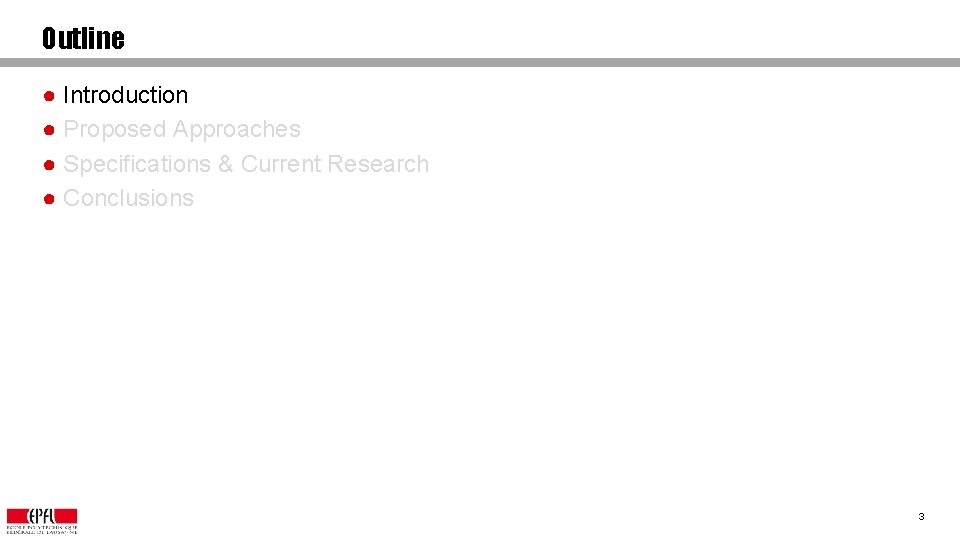
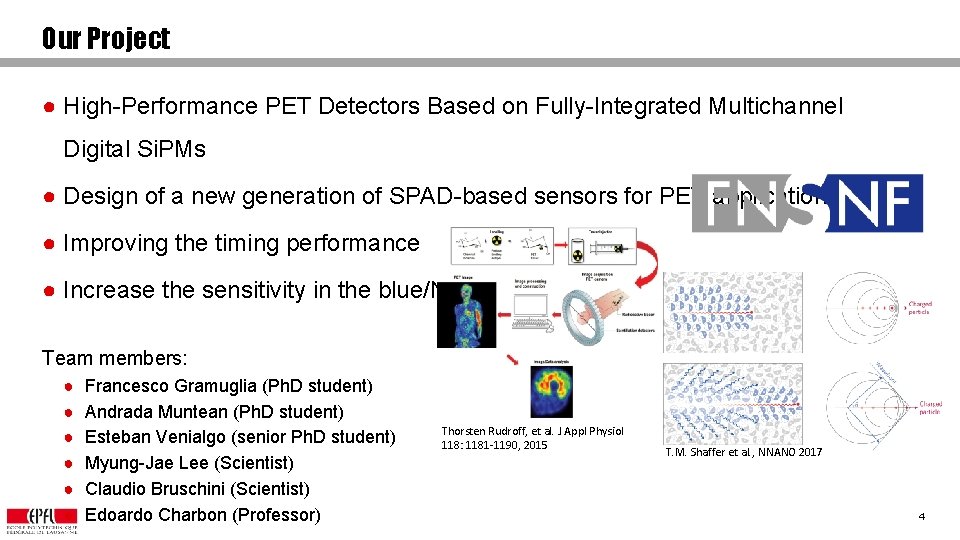
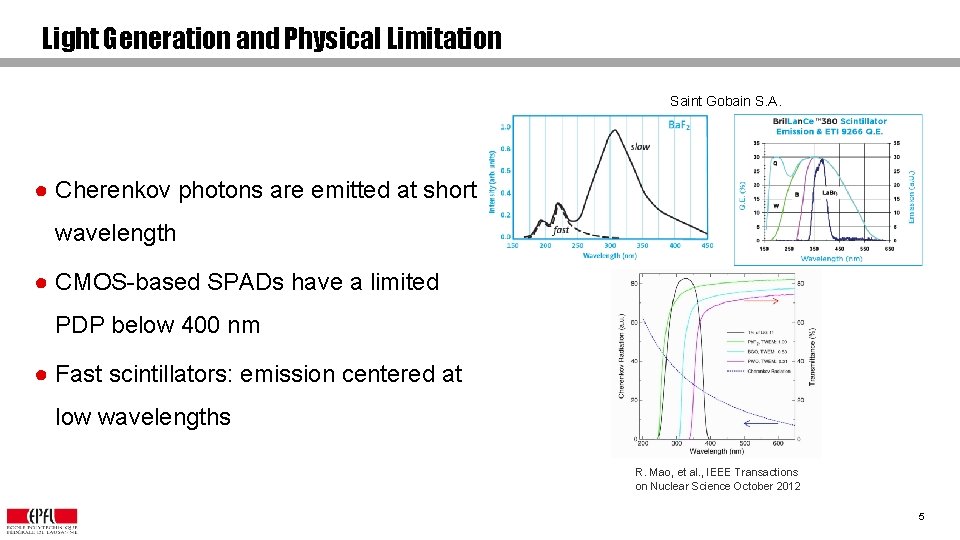
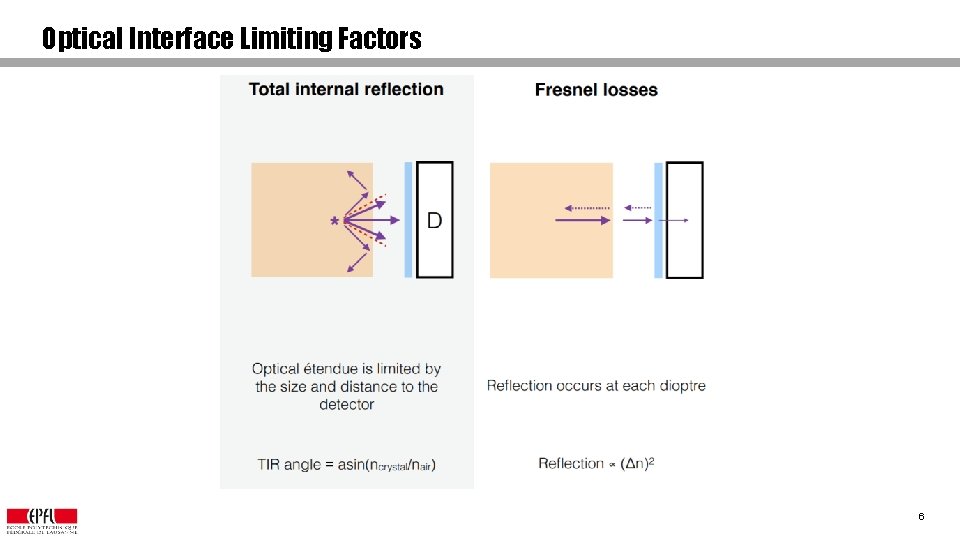
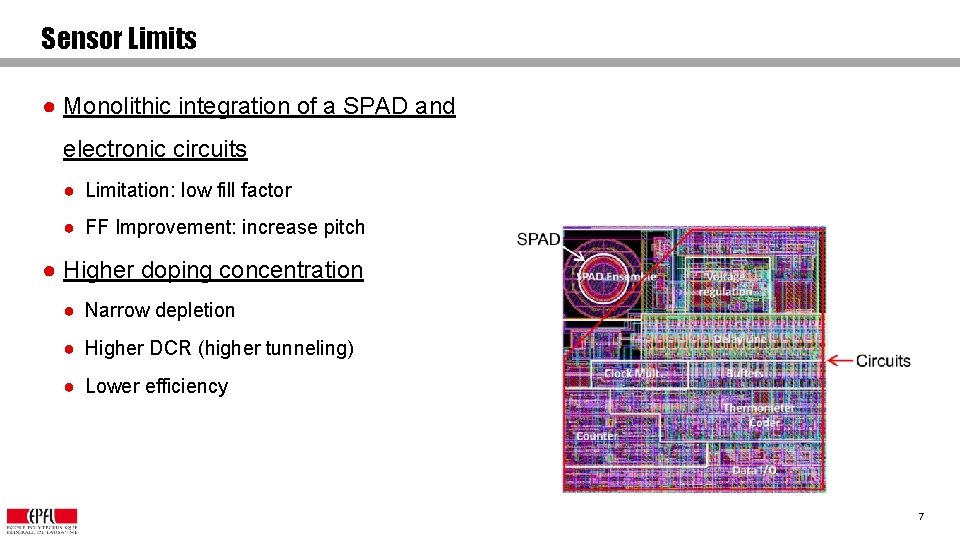
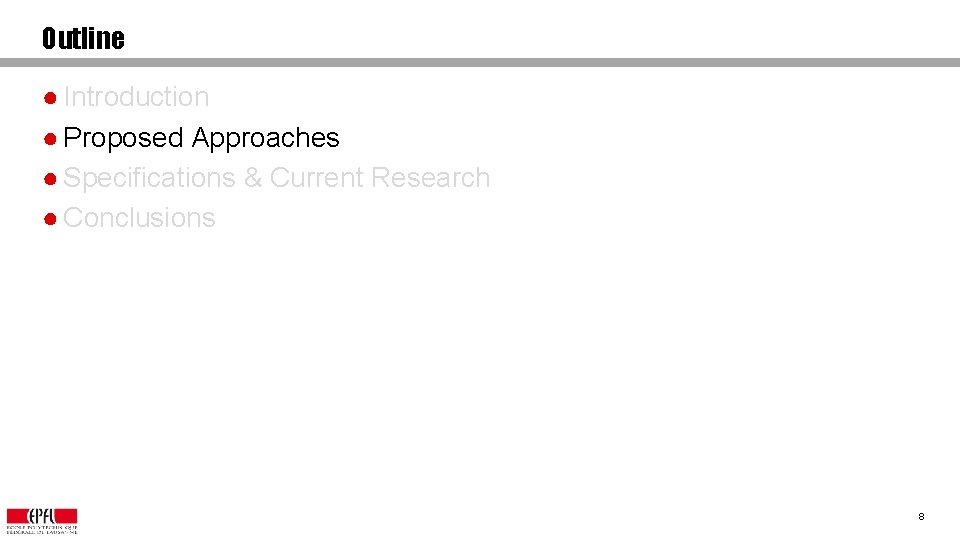
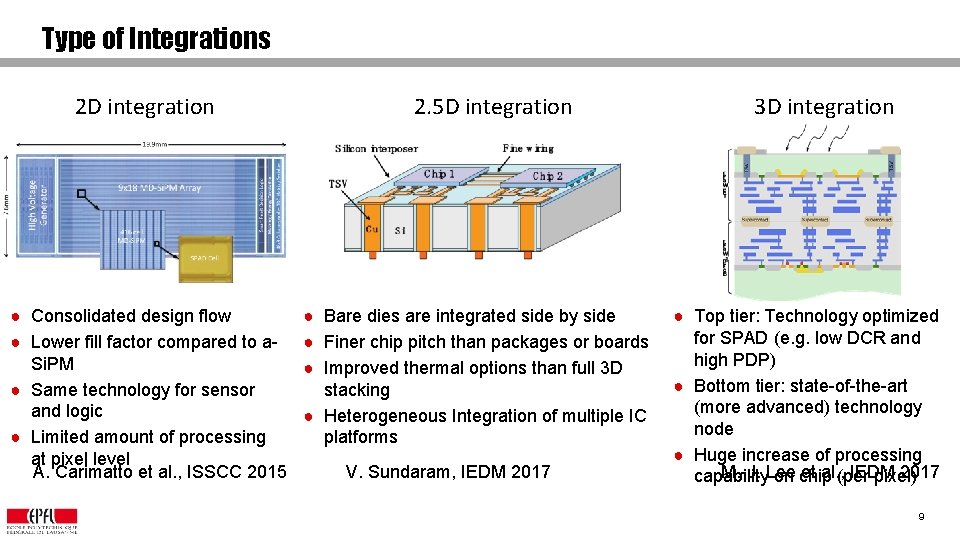
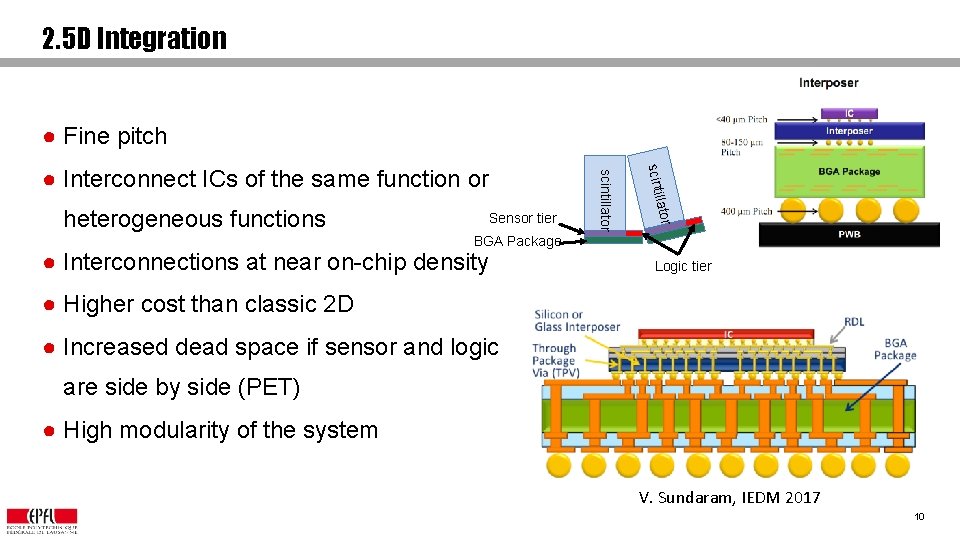
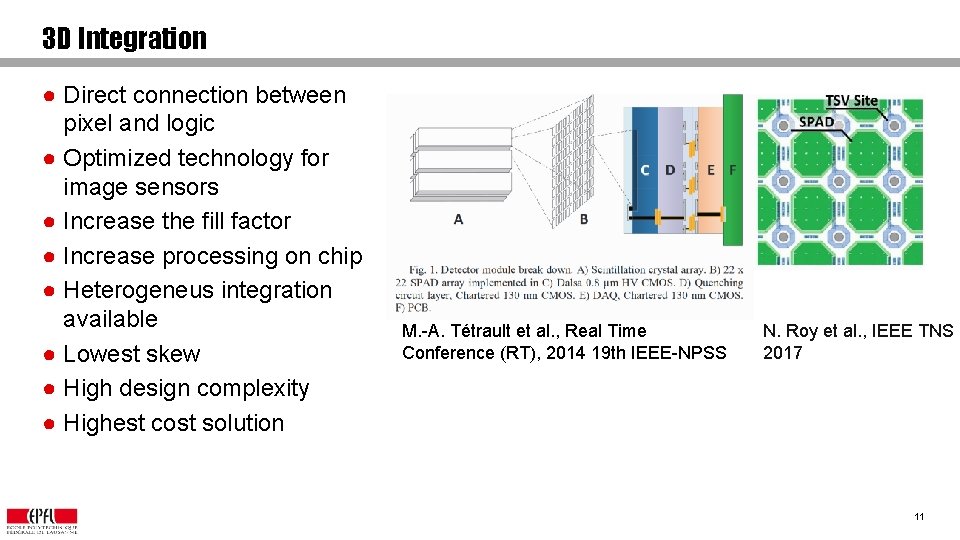
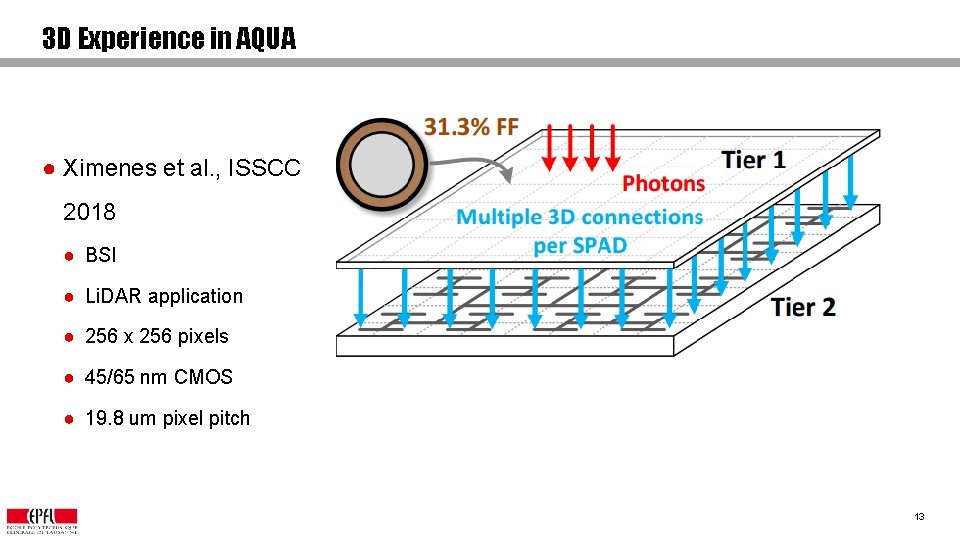
![3 D Experience in AQUA [2] – Confidential ● A. Carimatto, A. Ximenes, S. 3 D Experience in AQUA [2] – Confidential ● A. Carimatto, A. Ximenes, S.](https://slidetodoc.com/presentation_image_h2/ca0898eb05025f782a3b770e4631f507/image-13.jpg)
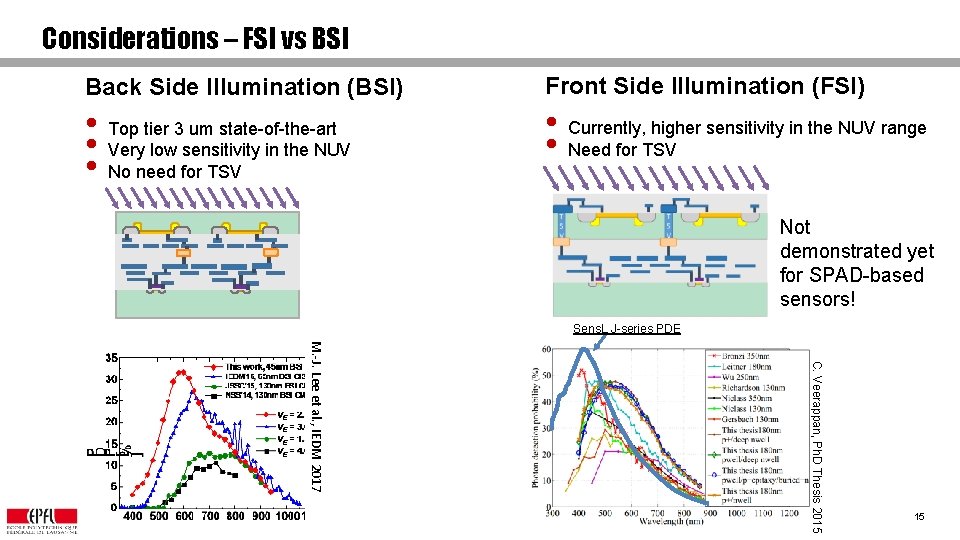
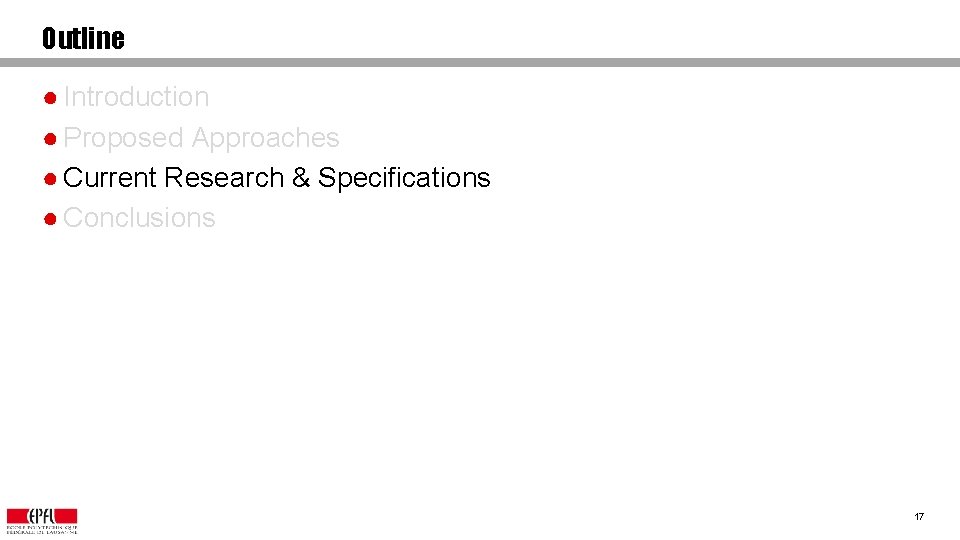
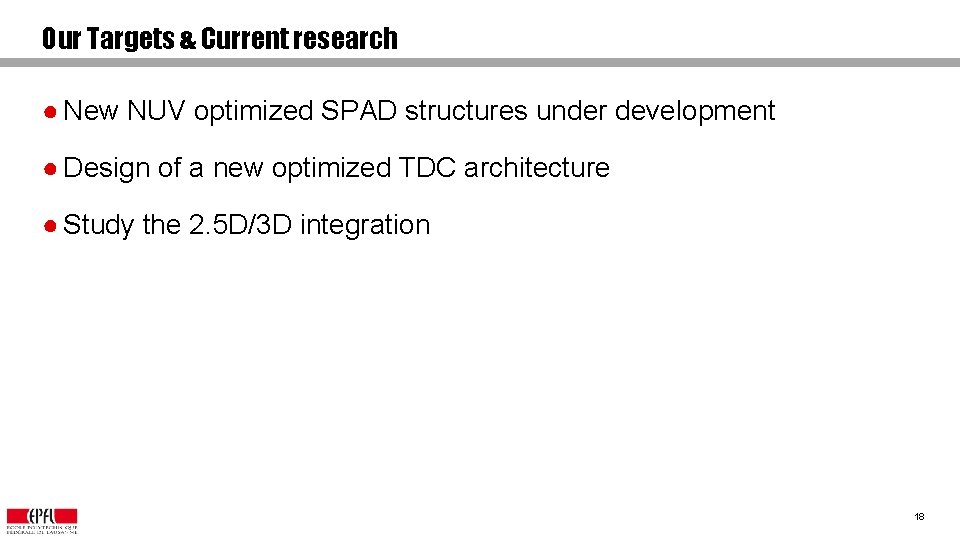
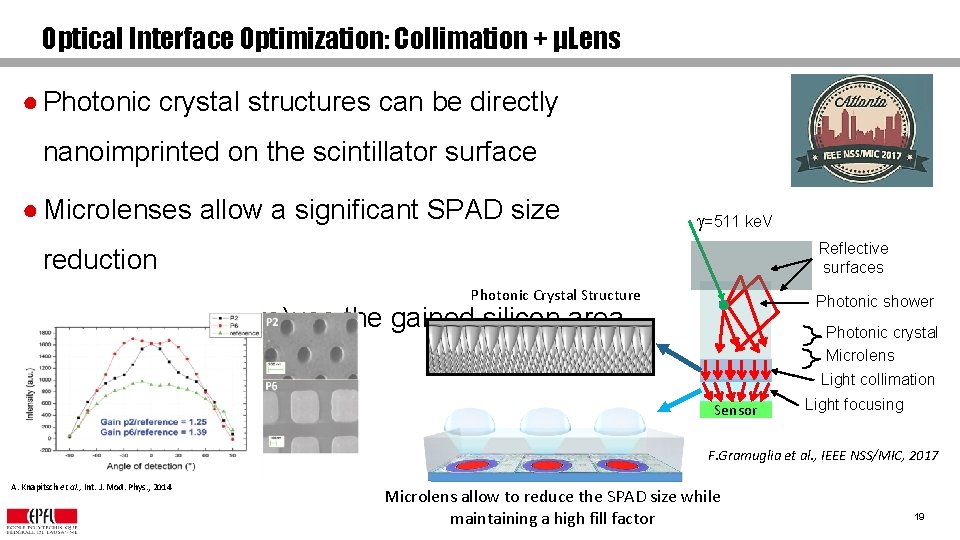
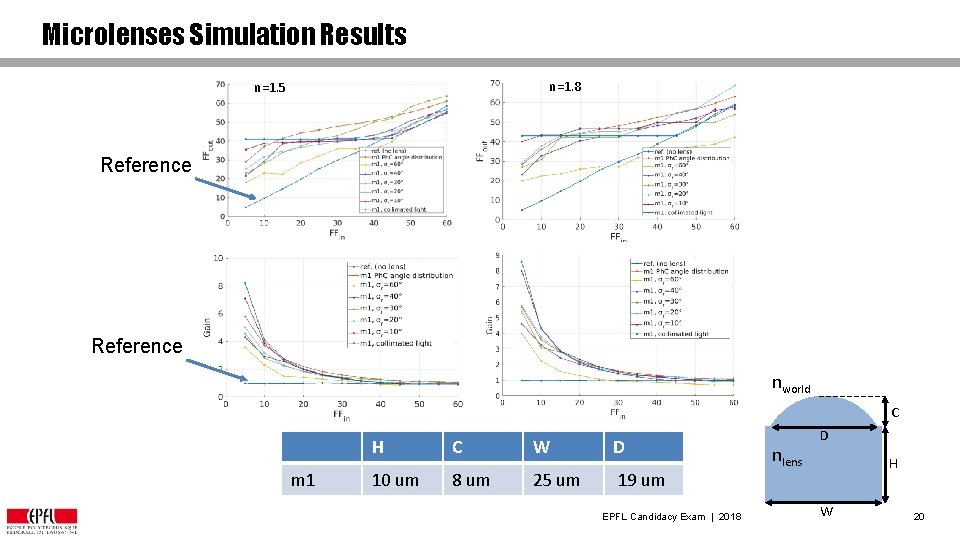
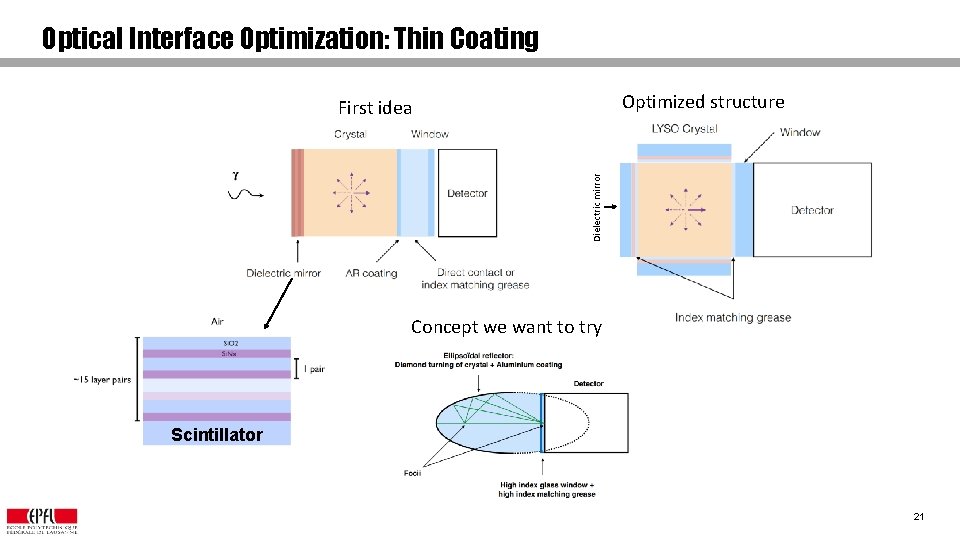
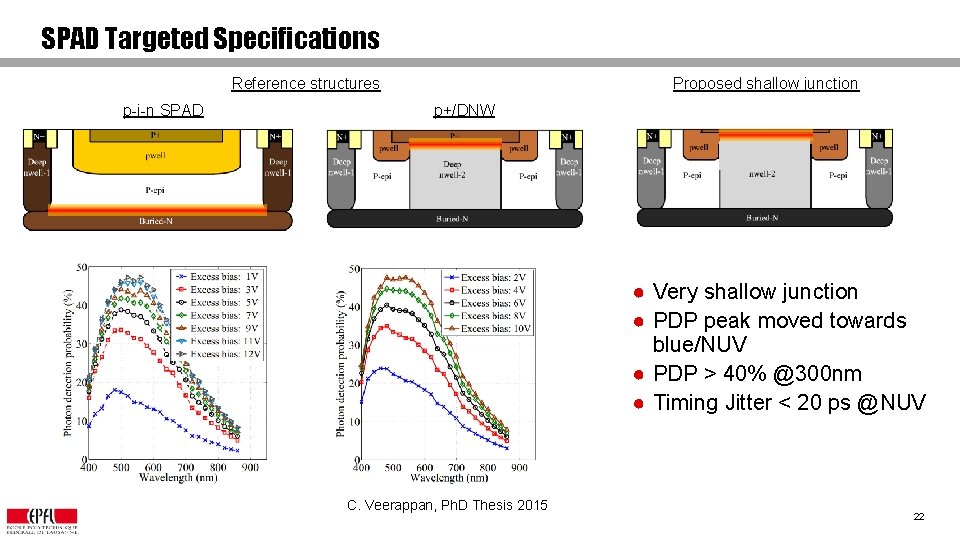
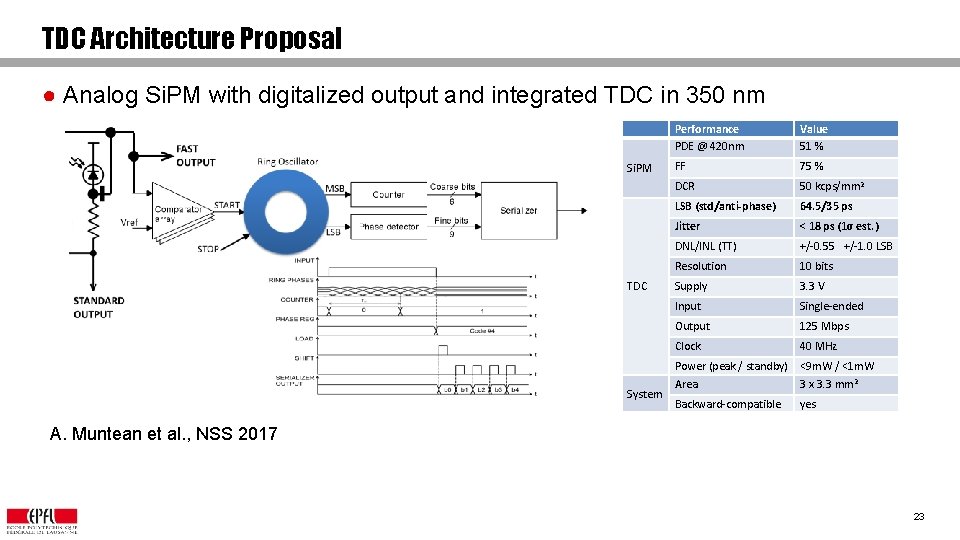
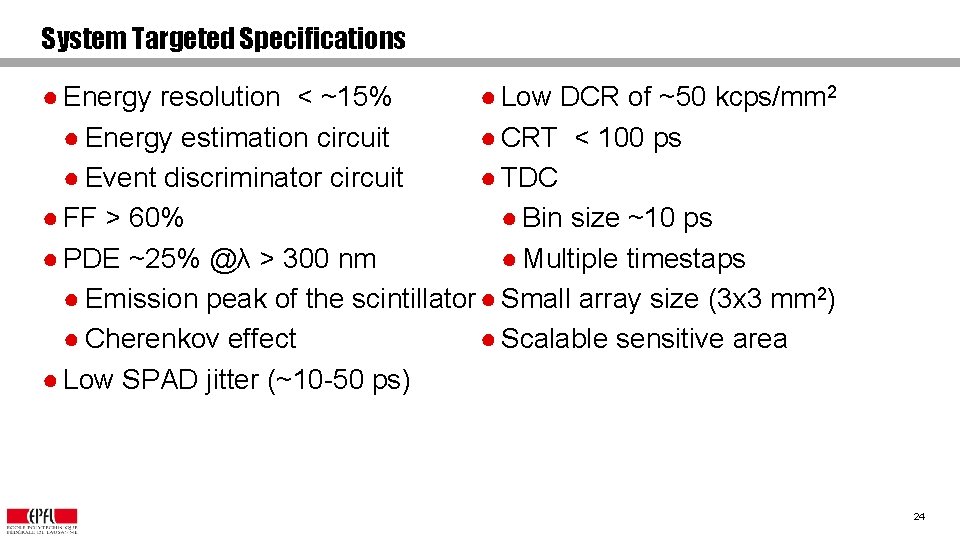
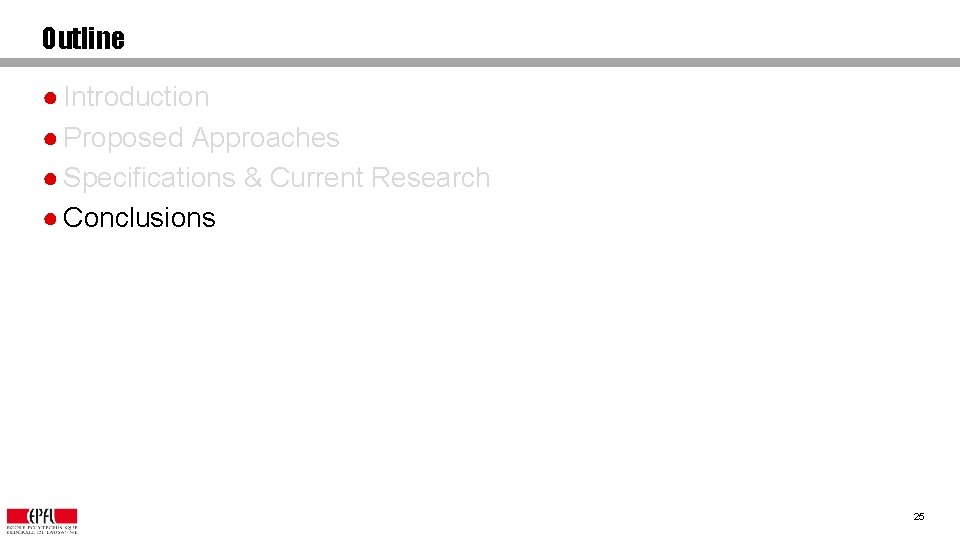
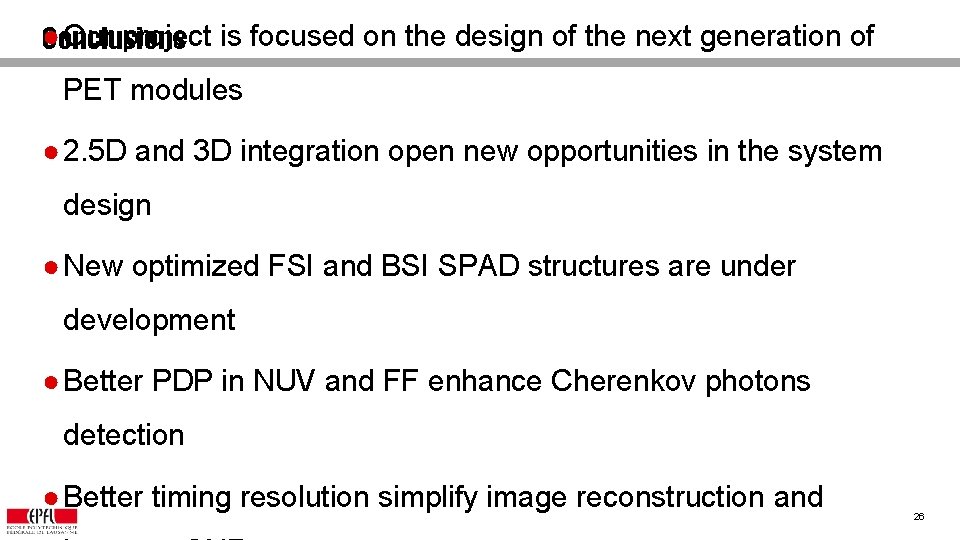
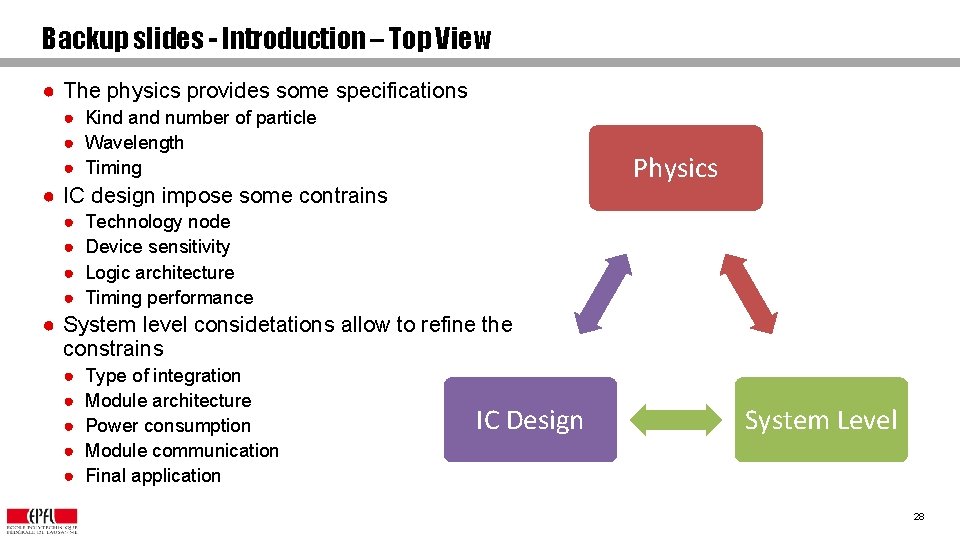
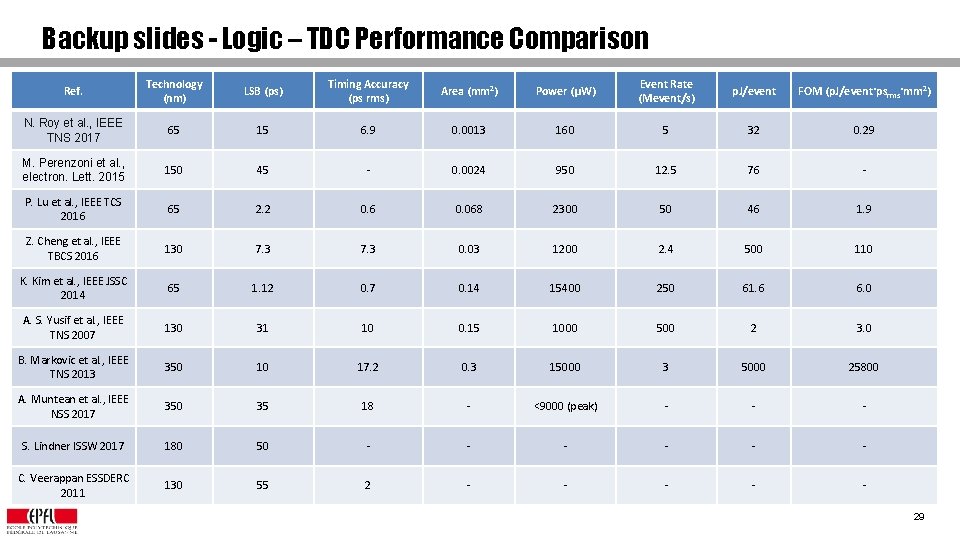
- Slides: 26
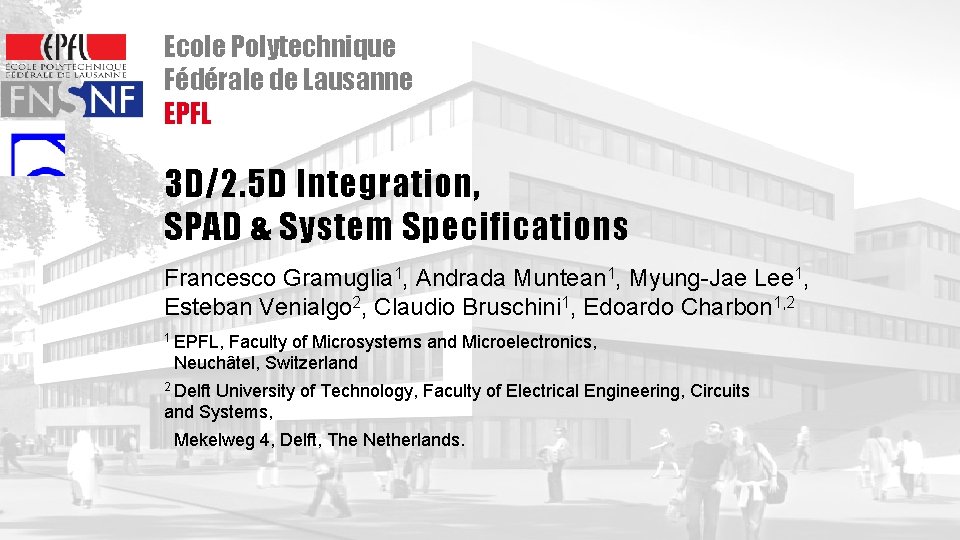
Ecole Polytechnique Fédérale de Lausanne EPFL 3 D/2. 5 D Integration, SPAD & System Specifications Francesco Gramuglia 1, Andrada Muntean 1, Myung-Jae Lee 1, Esteban Venialgo 2, Claudio Bruschini 1, Edoardo Charbon 1, 2 1 EPFL, Faculty of Microsystems and Microelectronics, Neuchâtel, Switzerland 2 Delft University of Technology, Faculty of Electrical Engineering, Circuits and Systems, Mekelweg 4, Delft, The Netherlands.
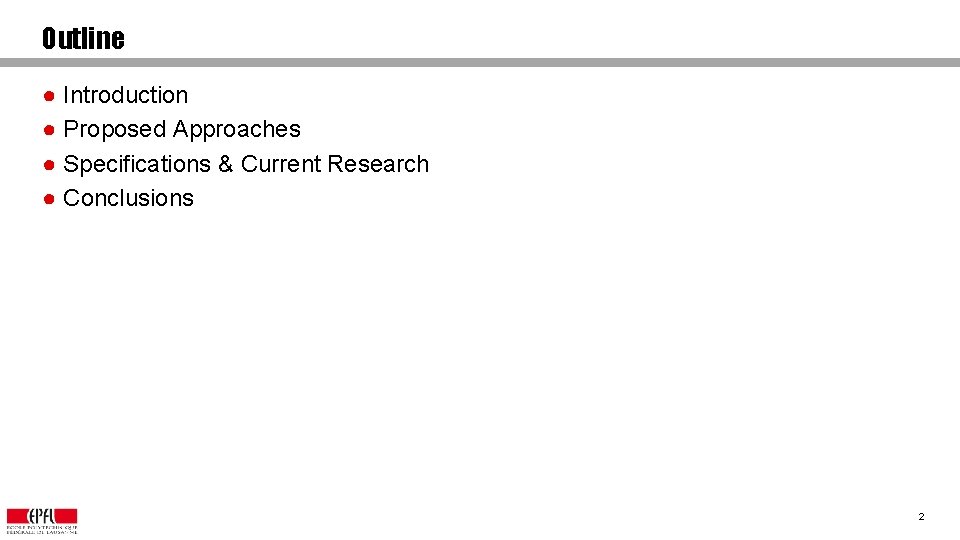
Outline ● Introduction ● Proposed Approaches ● Specifications & Current Research ● Conclusions 2
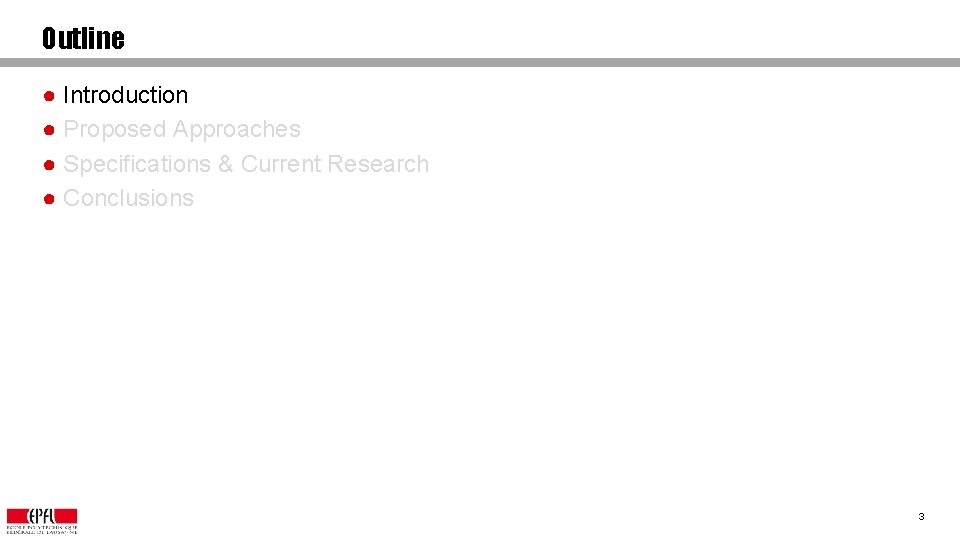
Outline ● Introduction ● Proposed Approaches ● Specifications & Current Research ● Conclusions 3
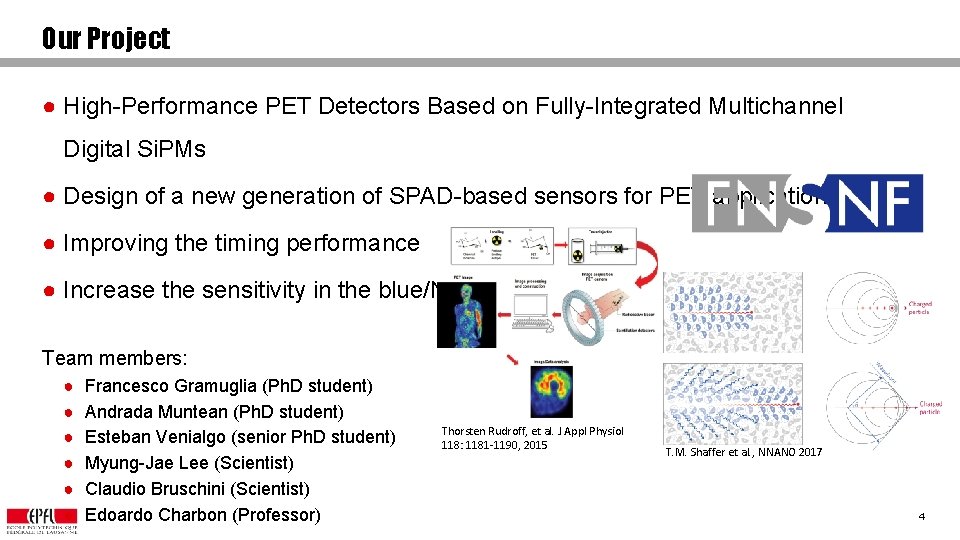
Our Project ● High-Performance PET Detectors Based on Fully-Integrated Multichannel Digital Si. PMs ● Design of a new generation of SPAD-based sensors for PET application ● Improving the timing performance ● Increase the sensitivity in the blue/NUV Team members: ● ● ● Francesco Gramuglia (Ph. D student) Andrada Muntean (Ph. D student) Esteban Venialgo (senior Ph. D student) Myung-Jae Lee (Scientist) Claudio Bruschini (Scientist) Edoardo Charbon (Professor) Thorsten Rudroff, et al. J Appl Physiol 118: 1181 -1190, 2015 T. M. Shaffer et al. , NNANO 2017 4
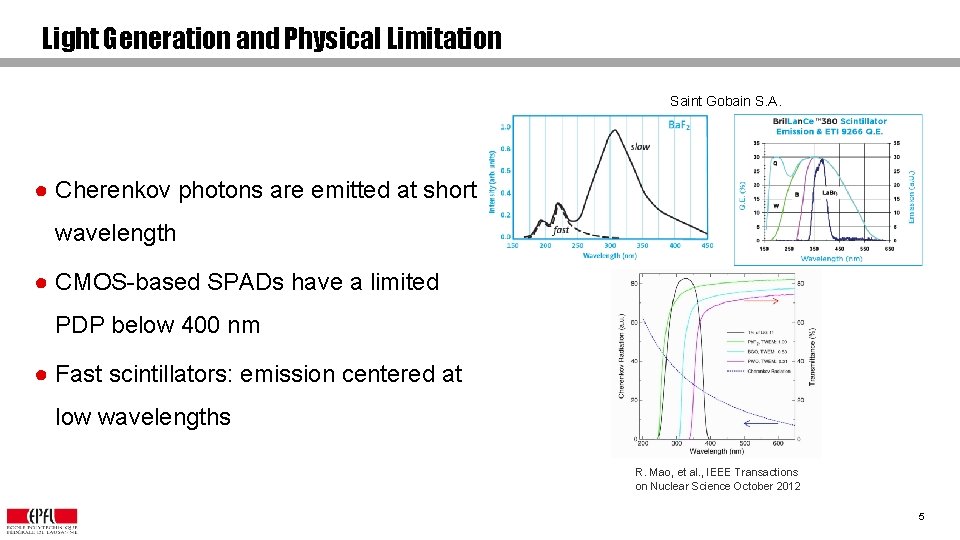
Light Generation and Physical Limitation Saint Gobain S. A. ● Cherenkov photons are emitted at short wavelength ● CMOS-based SPADs have a limited PDP below 400 nm ● Fast scintillators: emission centered at low wavelengths R. Mao, et al. , IEEE Transactions on Nuclear Science October 2012 5
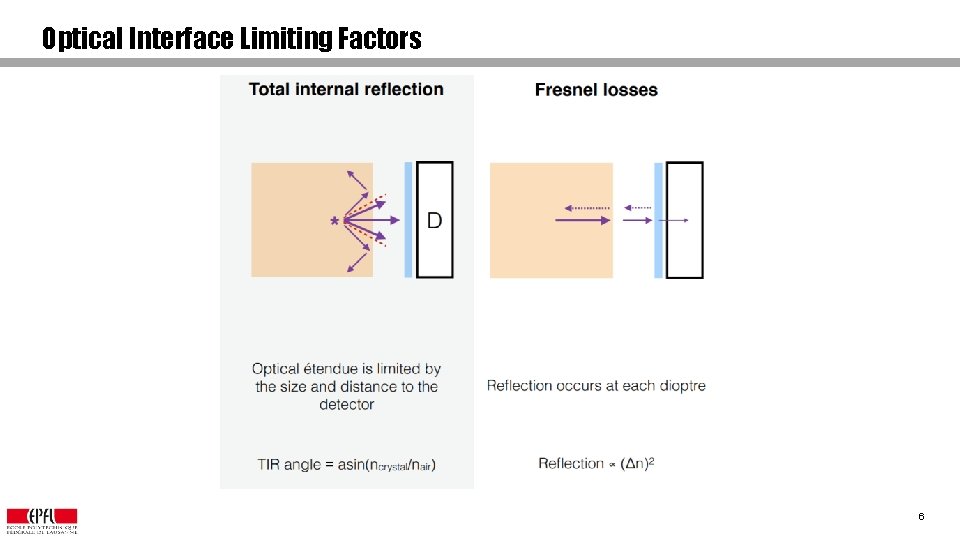
Optical Interface Limiting Factors 6
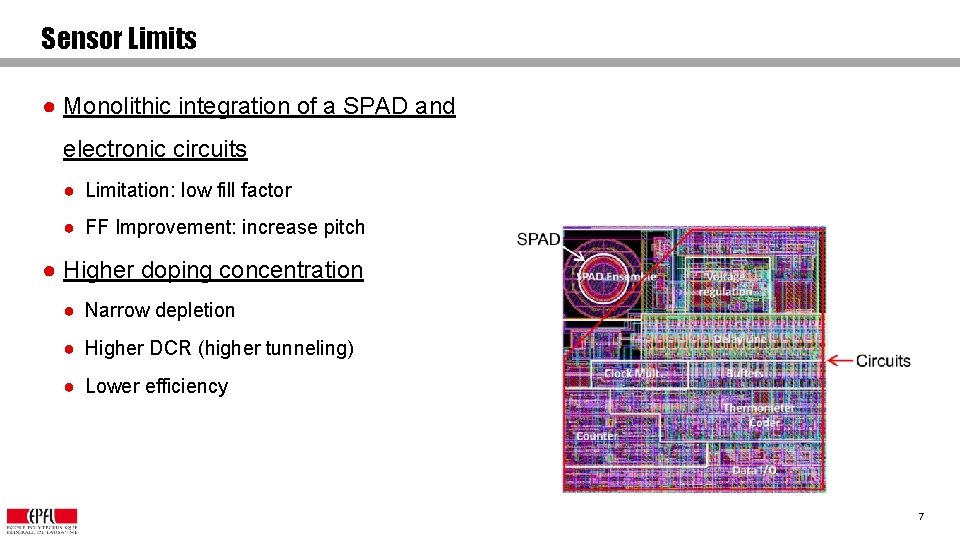
Sensor Limits ● Monolithic integration of a SPAD and electronic circuits ● Limitation: low fill factor ● FF Improvement: increase pitch ● Higher doping concentration ● Narrow depletion ● Higher DCR (higher tunneling) ● Lower efficiency 7
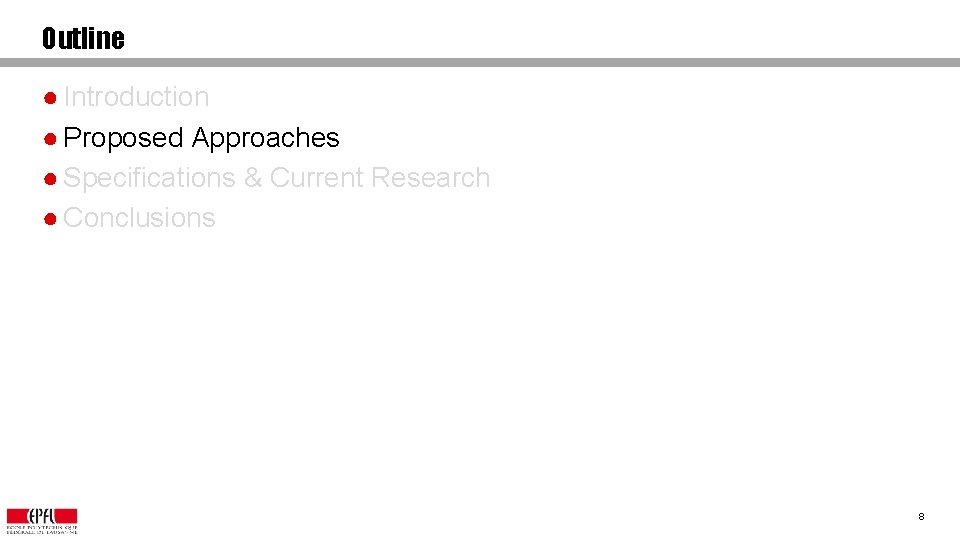
Outline ● Introduction ● Proposed Approaches ● Specifications & Current Research ● Conclusions 8
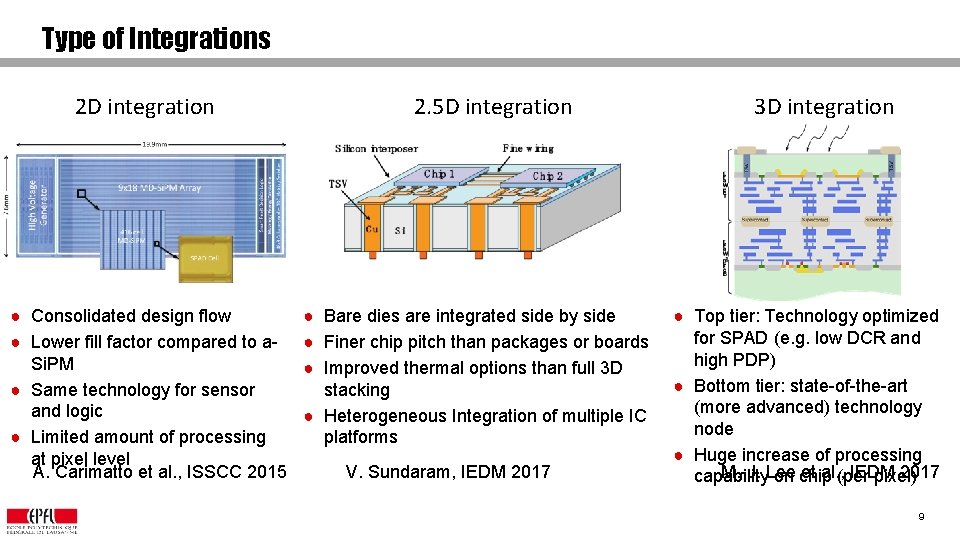
Type of Integrations 2 D integration ● Consolidated design flow ● Lower fill factor compared to a. Si. PM ● Same technology for sensor and logic ● Limited amount of processing at pixel level A. Carimatto et al. , ISSCC 2015 2. 5 D integration ● Bare dies are integrated side by side ● Finer chip pitch than packages or boards ● Improved thermal options than full 3 D stacking ● Heterogeneous Integration of multiple IC platforms V. Sundaram, IEDM 2017 3 D integration ● Top tier: Technology optimized for SPAD (e. g. low DCR and high PDP) ● Bottom tier: state-of-the-art (more advanced) technology node ● Huge increase of processing M. -J. Lee et al. , (per IEDM 2017 capability on chip pixel) 9
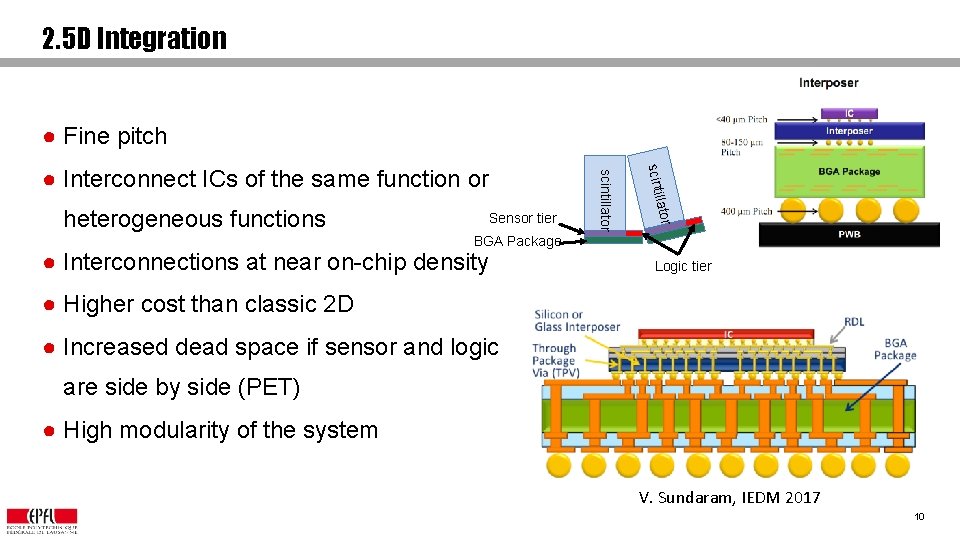
2. 5 D Integration ● Fine pitch llator Sensor tier scinti heterogeneous functions scintillator ● Interconnect ICs of the same function or BGA Package ● Interconnections at near on-chip density Logic tier ● Higher cost than classic 2 D ● Increased dead space if sensor and logic are side by side (PET) ● High modularity of the system V. Sundaram, IEDM 2017 10
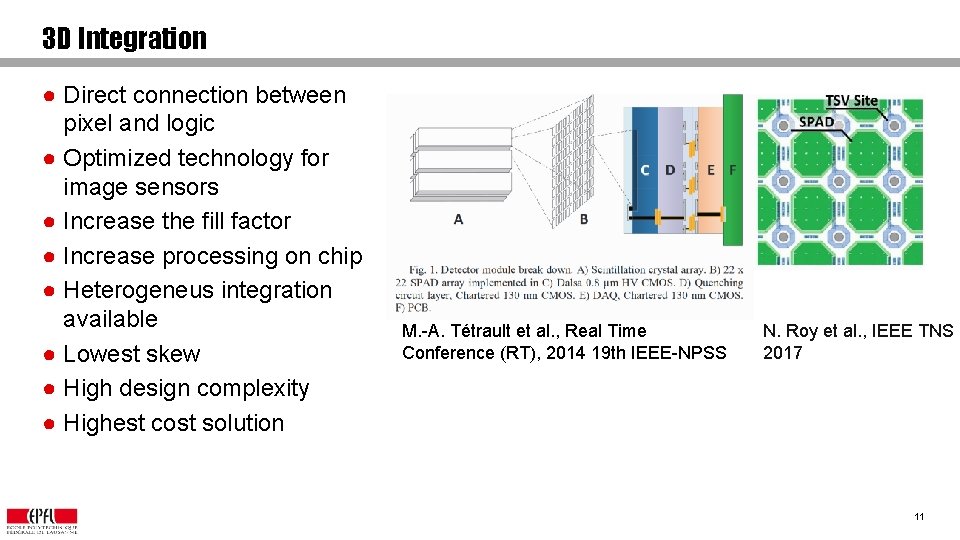
3 D Integration ● Direct connection between pixel and logic ● Optimized technology for image sensors ● Increase the fill factor ● Increase processing on chip ● Heterogeneus integration available ● Lowest skew ● High design complexity ● Highest cost solution M. -A. Tétrault et al. , Real Time Conference (RT), 2014 19 th IEEE-NPSS N. Roy et al. , IEEE TNS 2017 11
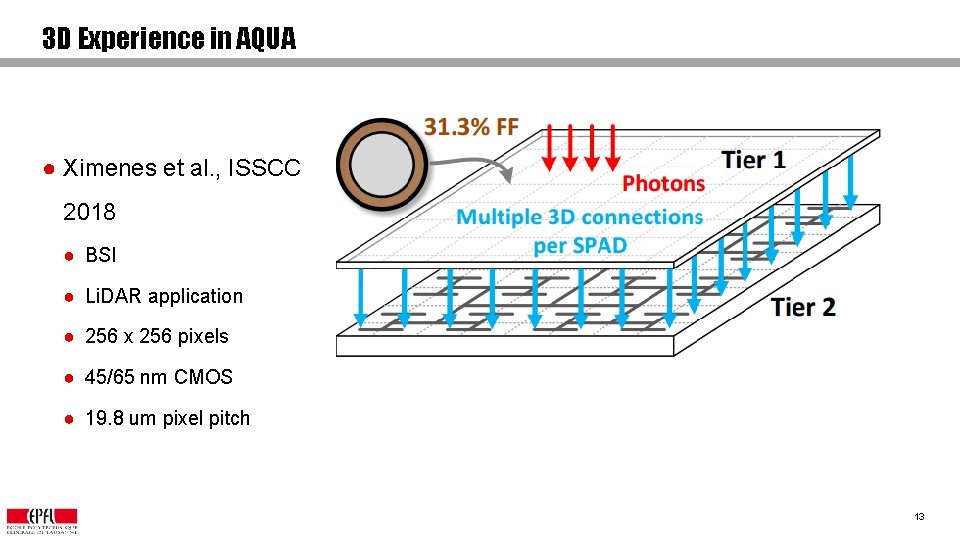
3 D Experience in AQUA ● Ximenes et al. , ISSCC 2018 ● BSI ● Li. DAR application ● 256 x 256 pixels ● 45/65 nm CMOS ● 19. 8 um pixel pitch 13
![3 D Experience in AQUA 2 Confidential A Carimatto A Ximenes S 3 D Experience in AQUA [2] – Confidential ● A. Carimatto, A. Ximenes, S.](https://slidetodoc.com/presentation_image_h2/ca0898eb05025f782a3b770e4631f507/image-13.jpg)
3 D Experience in AQUA [2] – Confidential ● A. Carimatto, A. Ximenes, S. 65 nm top tier Lidner. Intended for ISSCC 40 nm bottom Conf. registers tier Quadrant electronics 2019 L C ● BSI Quadrant electronics TDC ● Li. DAR application Quadrant electronics digital core Quadrant electronics adders/regs IO adders/regs ● Two halves: 32 x 64 and 64 x 128 SPADs ● 19. 8 um and 9. 4 um pixel ● 78% and 35% Fill Factor 1 pixel comprises 1 pitch SPAD 1 pixel comprises 4 SPADs g digital memory ● 40/65 nm CMOS electronics per quenchin pixel Timing line ● Digital read-out 14
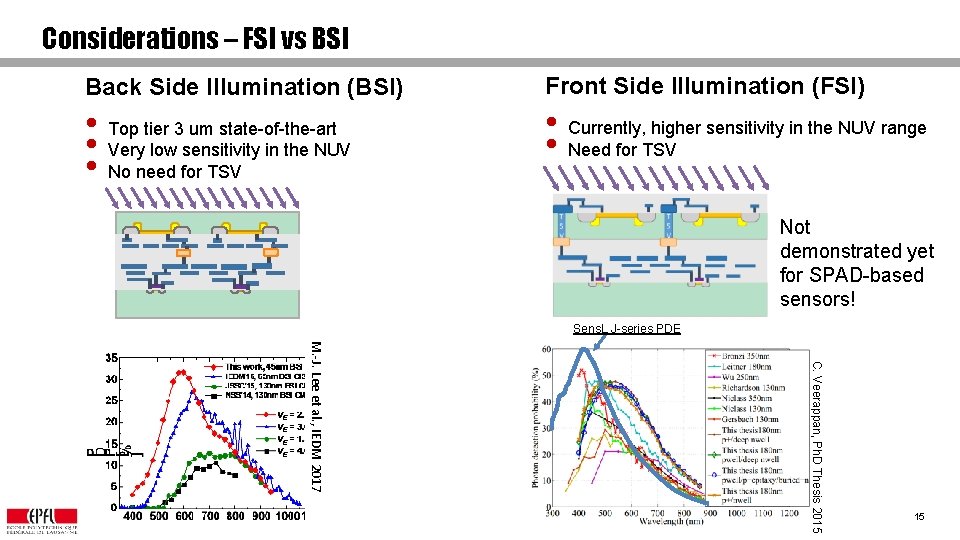
Considerations – FSI vs BSI Back Side Illumination (BSI) Front Side Illumination (FSI) • Top tier 3 um state-of-the-art • Very low sensitivity in the NUV • No need for TSV • Currently, higher sensitivity in the NUV range • Need for TSV Not demonstrated yet for SPAD-based sensors! Sens. L J-series PDE C. Veerappan, Ph. D Thesis 2015 M. -J. Lee et al. , IEDM 2017 15
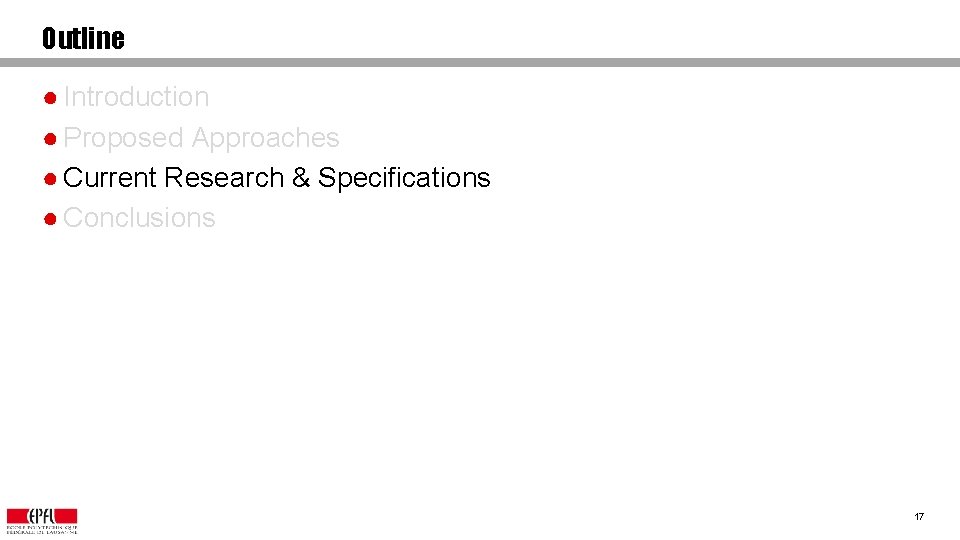
Outline ● Introduction ● Proposed Approaches ● Current Research & Specifications ● Conclusions 17
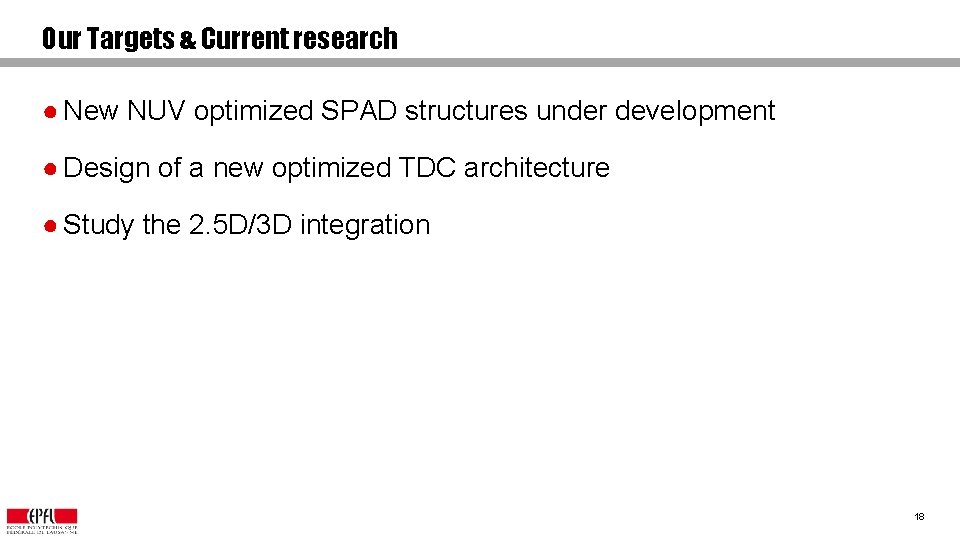
Our Targets & Current research ● New NUV optimized SPAD structures under development ● Design of a new optimized TDC architecture ● Study the 2. 5 D/3 D integration 18
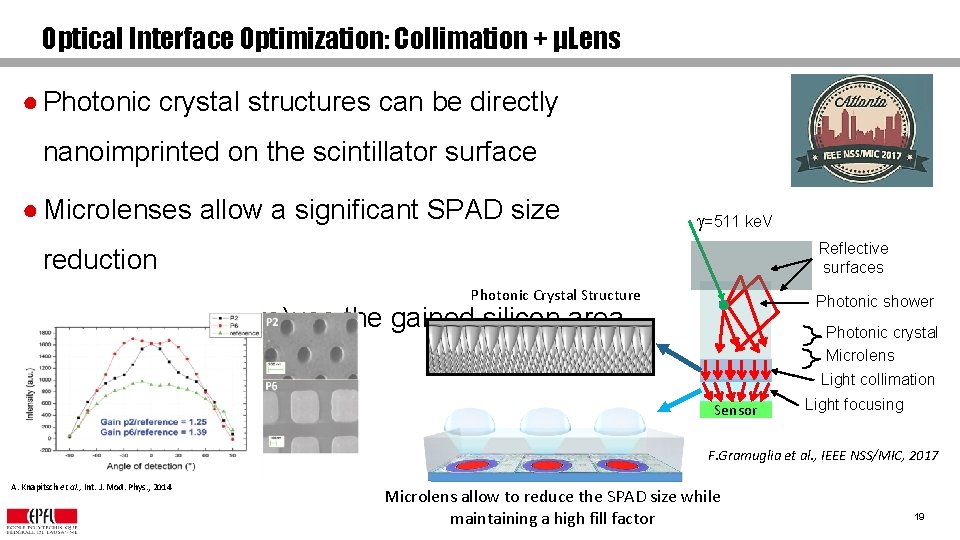
Optical Interface Optimization: Collimation + μLens ● Photonic crystal structures can be directly nanoimprinted on the scintillator surface ● Microlenses allow a significant SPAD size g=511 ke. V Reflective surfaces reduction Photonic Crystal Structure Photonic shower ● Several ways to (re)use the gained silicon area Photonic crystal Microlens Light collimation Sensor Light focusing F. Gramuglia et al. , IEEE NSS/MIC, 2017 A. Knapitsch et al. , Int. J. Mod. Phys. , 2014 Microlens allow to reduce the SPAD size while maintaining a high fill factor 19
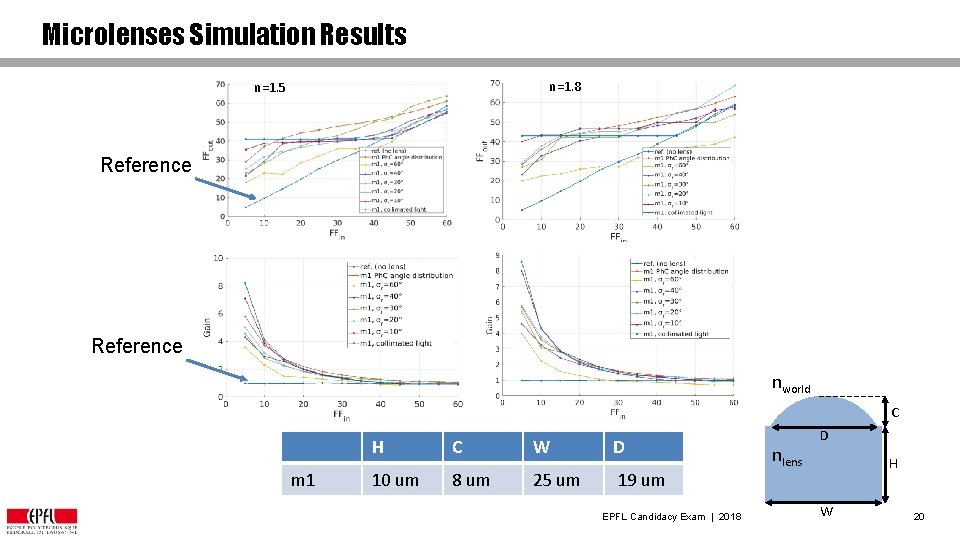
Microlenses Simulation Results n=1. 8 n=1. 5 Reference nworld C m 1 H C W D 10 um 8 um 25 um 19 um EPFL Candidacy Exam | 2018 nlens D H W 20
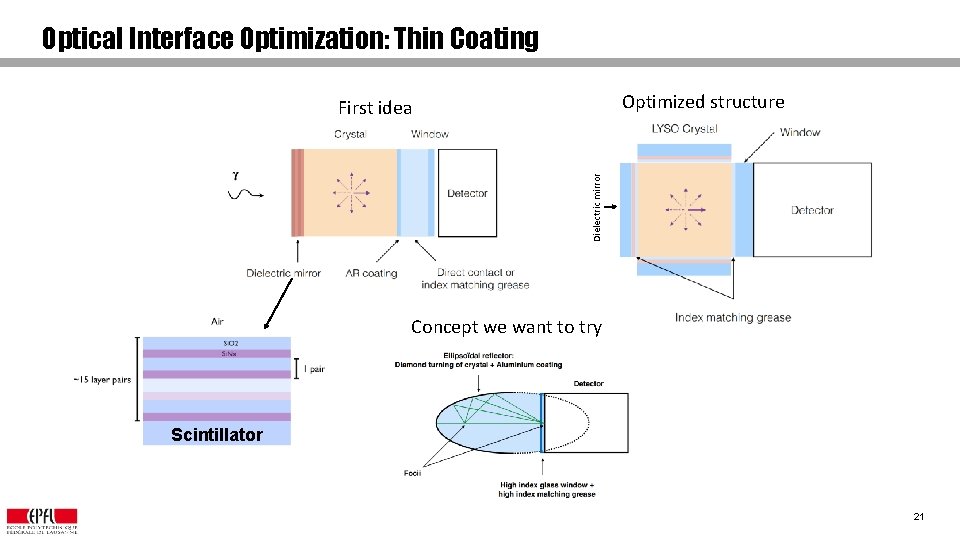
Optical Interface Optimization: Thin Coating Optimized structure Dielectric mirror First idea Concept we want to try Scintillator 21
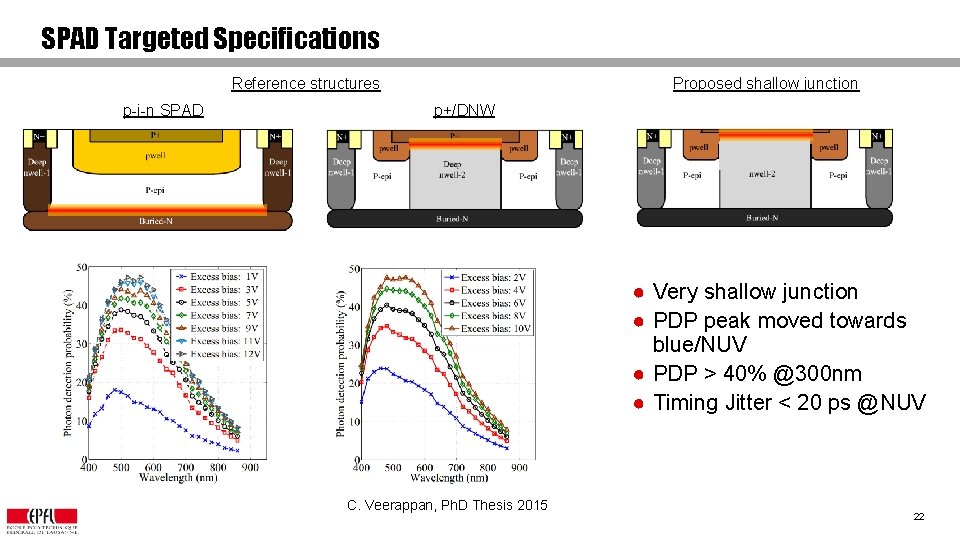
SPAD Targeted Specifications Proposed shallow junction Reference structures p-i-n SPAD p+/DNW ● Very shallow junction ● PDP peak moved towards blue/NUV ● PDP > 40% @300 nm ● Timing Jitter < 20 ps @NUV C. Veerappan, Ph. D Thesis 2015 22
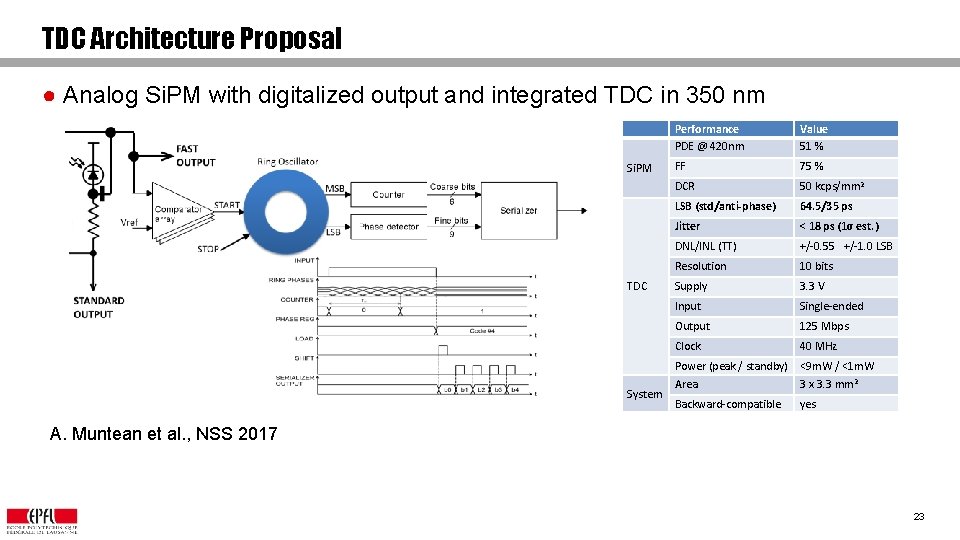
TDC Architecture Proposal ● Analog Si. PM with digitalized output and integrated TDC in 350 nm Si. PM TDC System Performance PDE @ 420 nm Value 51 % FF 75 % DCR 50 kcps/mm 2 LSB (std/anti-phase) 64. 5/35 ps Jitter < 18 ps (1σ est. ) DNL/INL (TT) +/-0. 55 +/-1. 0 LSB Resolution 10 bits Supply 3. 3 V Input Single-ended Output 125 Mbps Clock 40 MHz Power (peak / standby) <9 m. W / <1 m. W Area 3 x 3. 3 mm 2 Backward-compatible yes A. Muntean et al. , NSS 2017 23
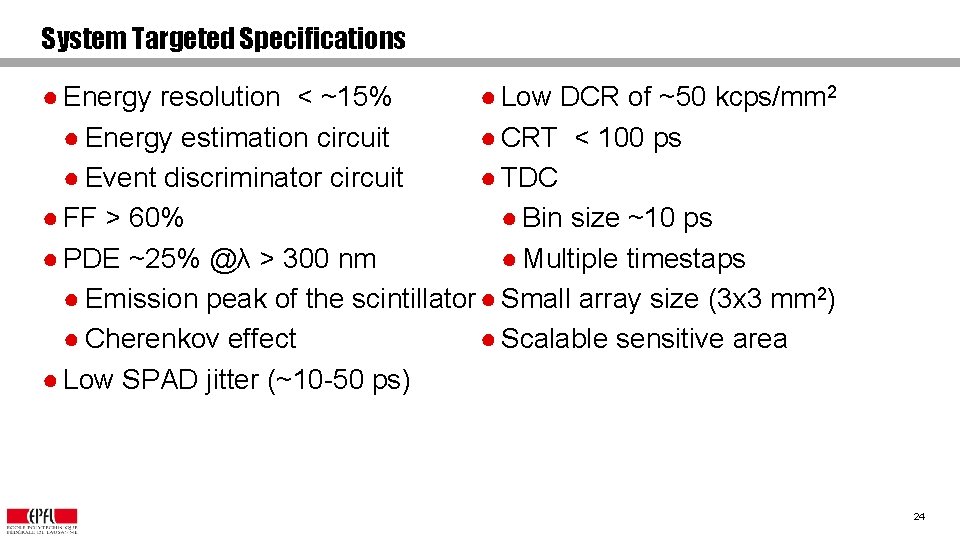
System Targeted Specifications ● Low DCR of ~50 kcps/mm 2 ● Energy resolution < ~15% ● CRT < 100 ps ● Energy estimation circuit ● TDC ● Event discriminator circuit ● Bin size ~10 ps ● FF > 60% ● Multiple timestaps ● PDE ~25% @λ > 300 nm ● Emission peak of the scintillator ● Small array size (3 x 3 mm 2) ● Scalable sensitive area ● Cherenkov effect ● Low SPAD jitter (~10 -50 ps) 24
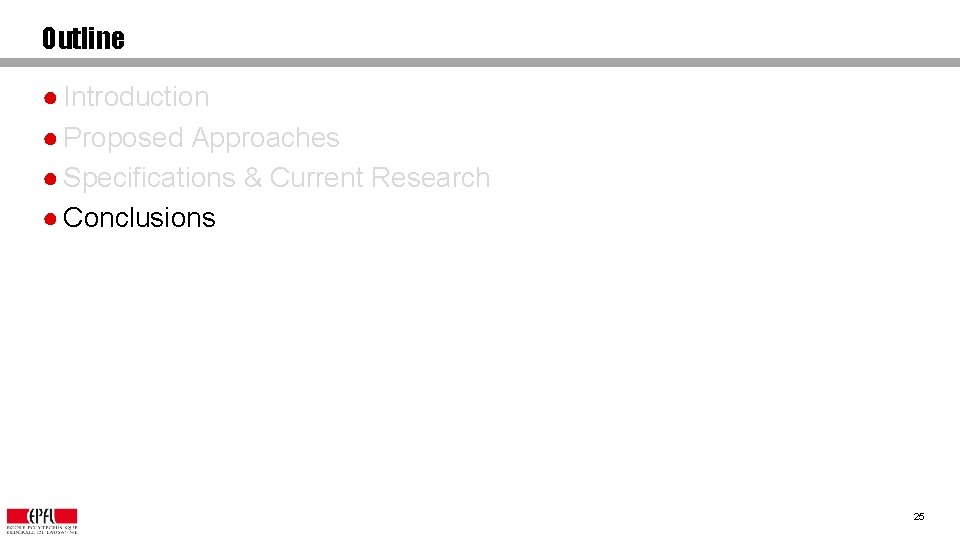
Outline ● Introduction ● Proposed Approaches ● Specifications & Current Research ● Conclusions 25
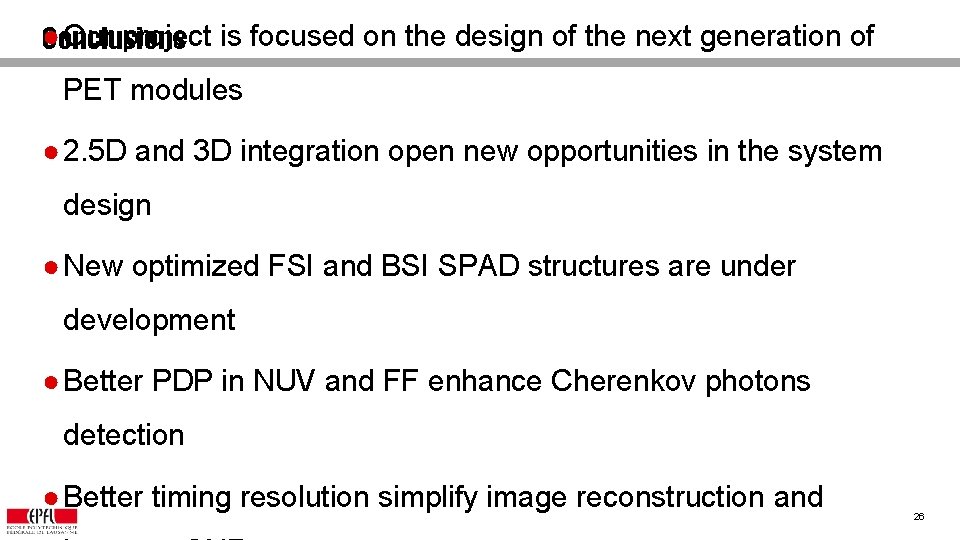
● Our project is focused on the design of the next generation of Conclusions PET modules ● 2. 5 D and 3 D integration open new opportunities in the system design ● New optimized FSI and BSI SPAD structures are under development ● Better PDP in NUV and FF enhance Cherenkov photons detection ● Better timing resolution simplify image reconstruction and 26
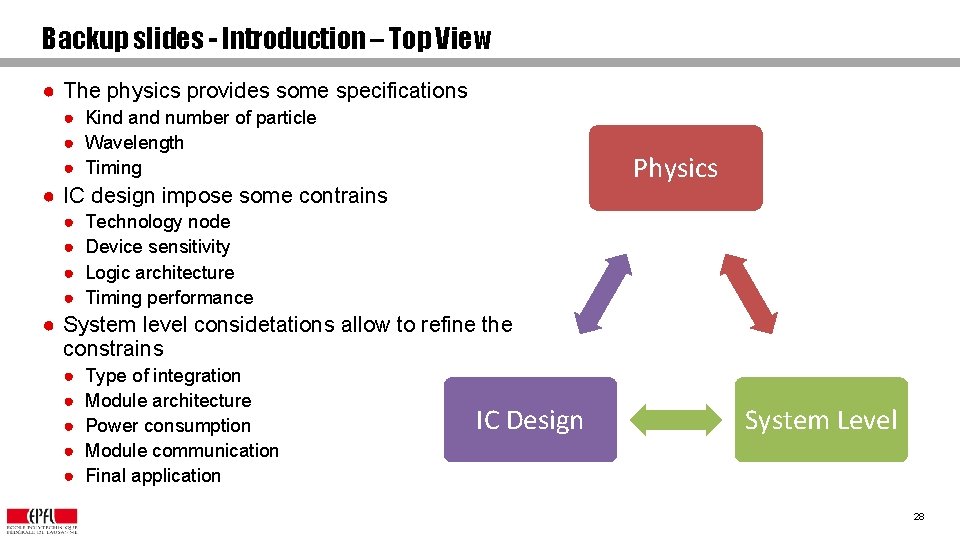
Backup slides - Introduction – Top View ● The physics provides some specifications ● Kind and number of particle ● Wavelength ● Timing Physics ● IC design impose some contrains ● ● Technology node Device sensitivity Logic architecture Timing performance ● System level considetations allow to refine the constrains ● ● ● Type of integration Module architecture Power consumption Module communication Final application IC Design System Level 28
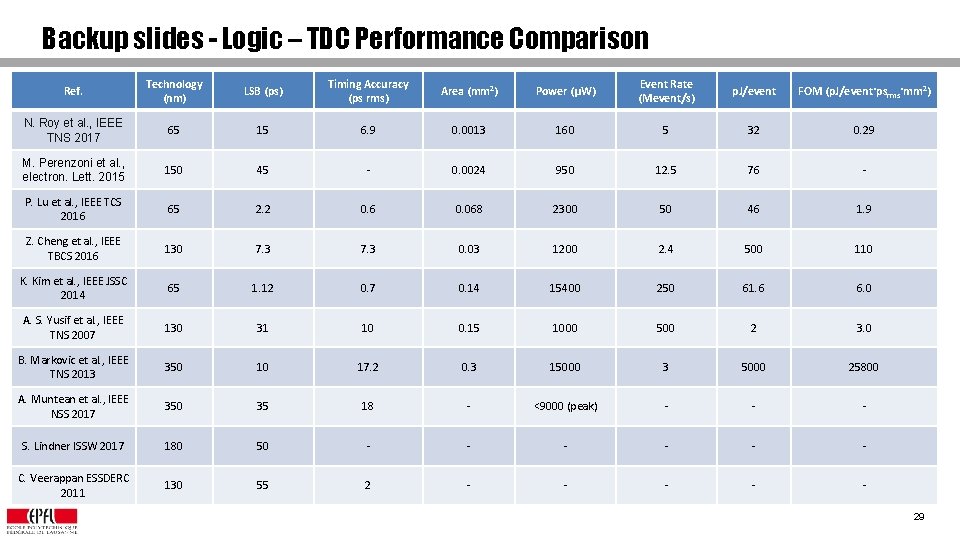
Backup slides - Logic – TDC Performance Comparison Ref. Technology (nm) LSB (ps) Timing Accuracy (ps rms) Area (mm 2) Power (μW) Event Rate (Mevent/s) p. J/event FOM (p. J/event·psrms·mm 2) N. Roy et al. , IEEE TNS 2017 65 15 6. 9 0. 0013 160 5 32 0. 29 M. Perenzoni et al. , electron. Lett. 2015 150 45 - 0. 0024 950 12. 5 76 - P. Lu et al. , IEEE TCS 2016 65 2. 2 0. 6 0. 068 2300 50 46 1. 9 Z. Cheng et al. , IEEE TBCS 2016 130 7. 3 0. 03 1200 2. 4 500 110 K. Kim et al. , IEEE JSSC 2014 65 1. 12 0. 7 0. 14 15400 250 61. 6 6. 0 A. S. Yusif et al. , IEEE TNS 2007 130 31 10 0. 15 1000 500 2 3. 0 B. Markovic et al. , IEEE TNS 2013 350 10 17. 2 0. 3 15000 3 5000 25800 A. Muntean et al. , IEEE NSS 2017 350 35 18 - <9000 (peak) - - - S. Lindner ISSW 2017 180 50 - - - C. Veerappan ESSDERC 2011 130 55 2 - - 29
Master in economics polytechnique
Polytechnique formation continue
Université polytechnique hauts-de-france
Bcx polytechnique
Polytechnique vontovorona
Bobar polytechnique
Scala lausanne
Mazhab lausanne
Pharmacie gamma lausanne laboratoire
Einkaufsstrasse lausanne
Storage lausanne
Sovereignty is not given it is taken
Intelligent agents epfl
Epfl internship
Tasos kyrillidis
Ine epfl
Game theory epfl
Moodle epfl
Ic epfl
Epfl library
Industrial automation epfl
Epfl map
Mobile networks epfl
Epfl cmi
Guido haefeli
Point velo epfl
Epfl infoscience