A Management and Control System MCS for District
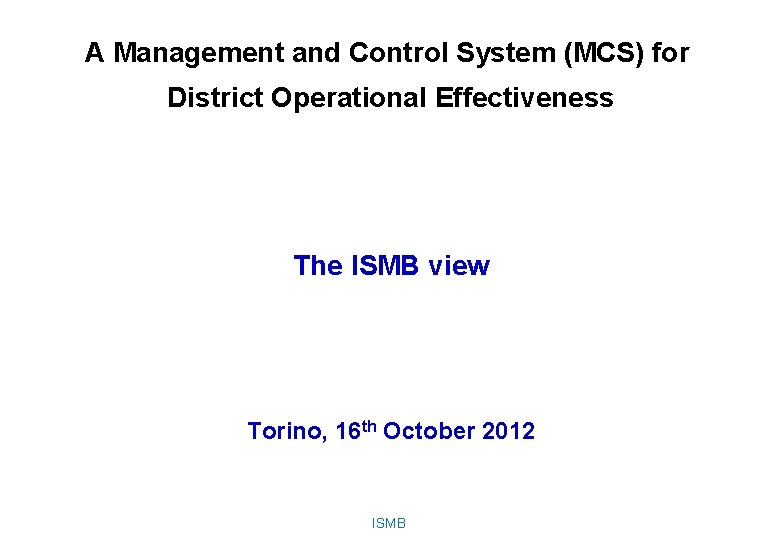
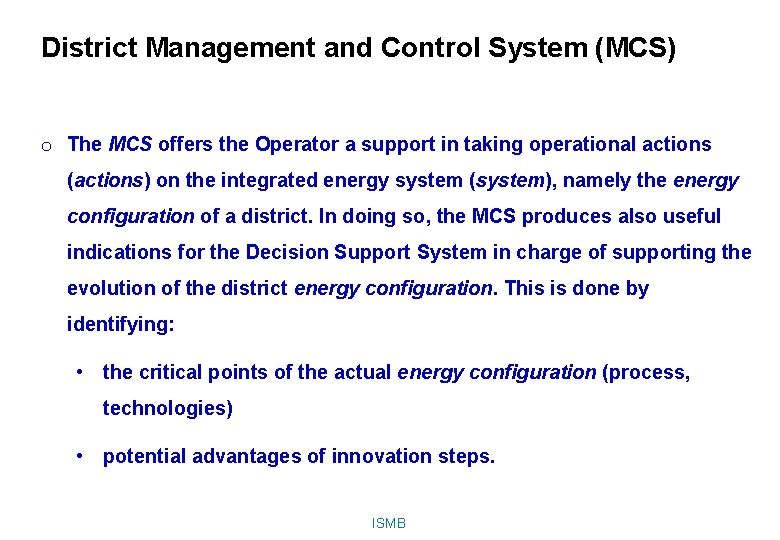
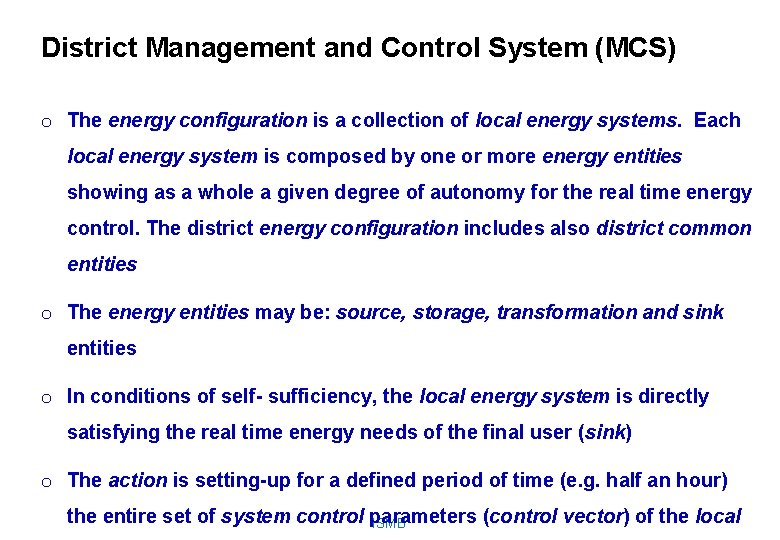
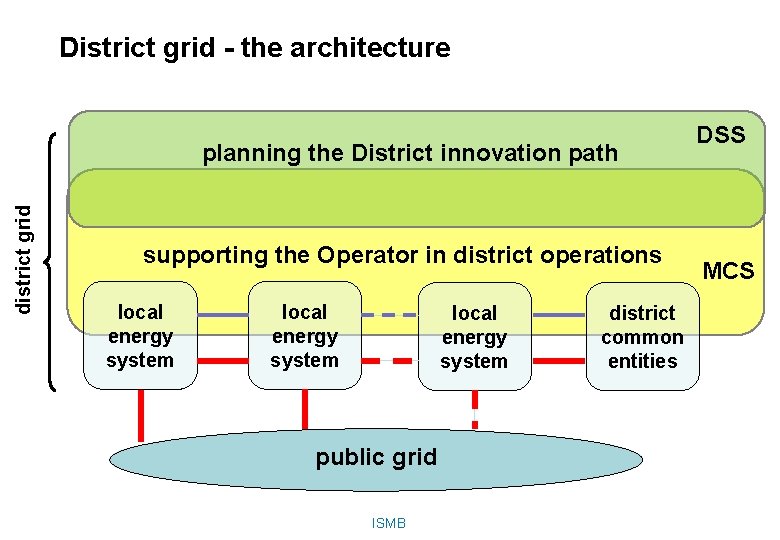
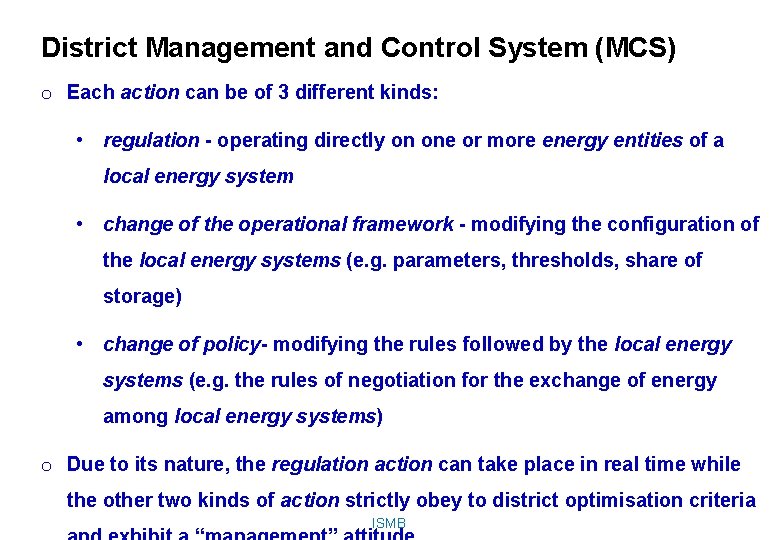
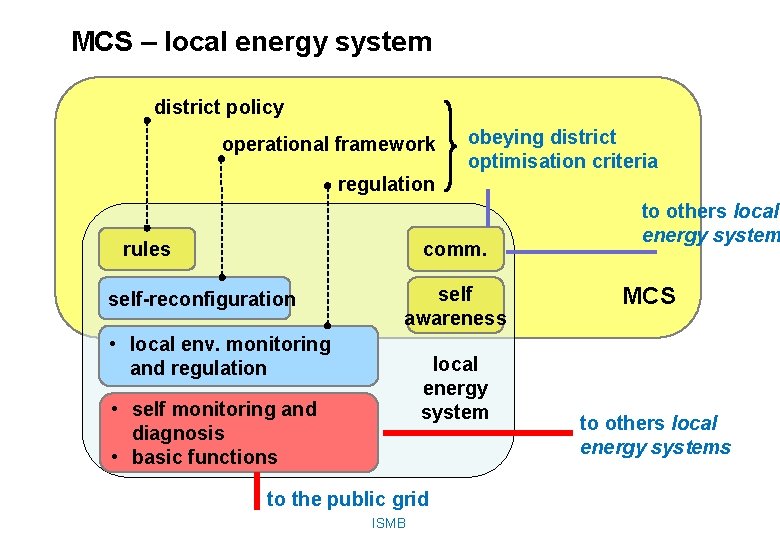
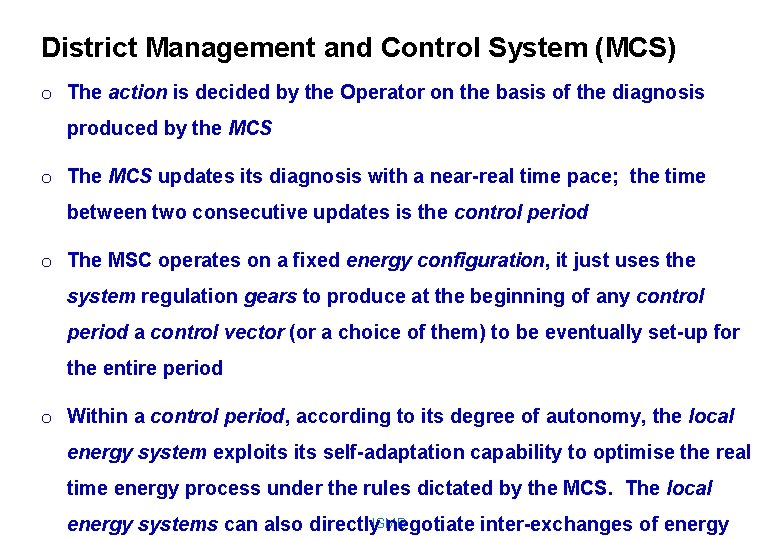
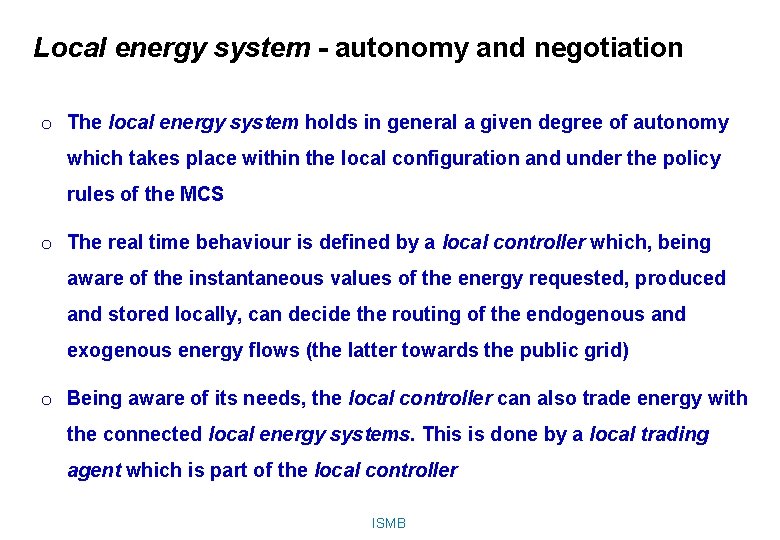
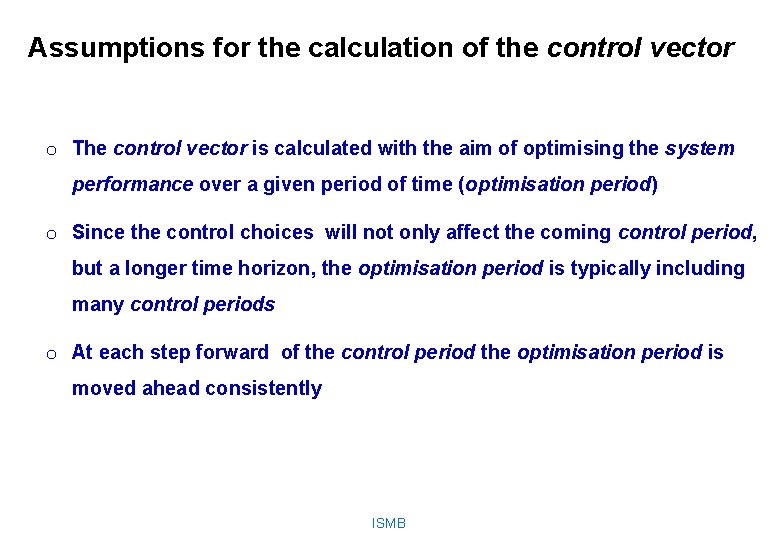
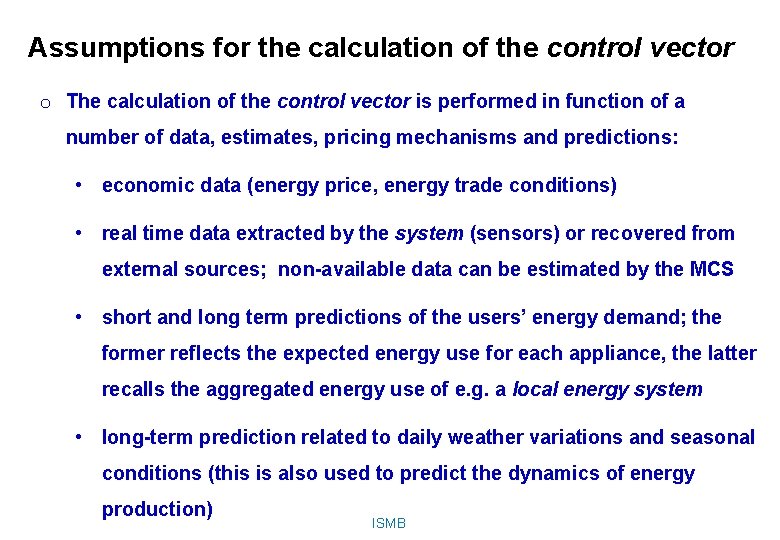
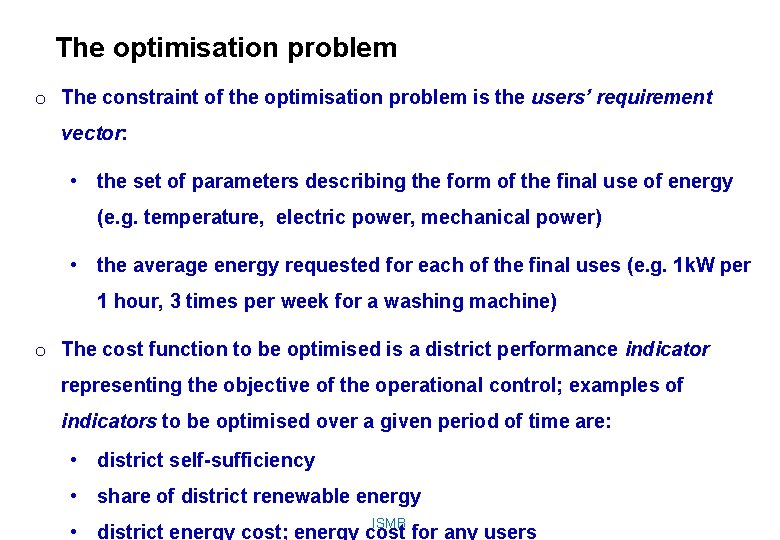
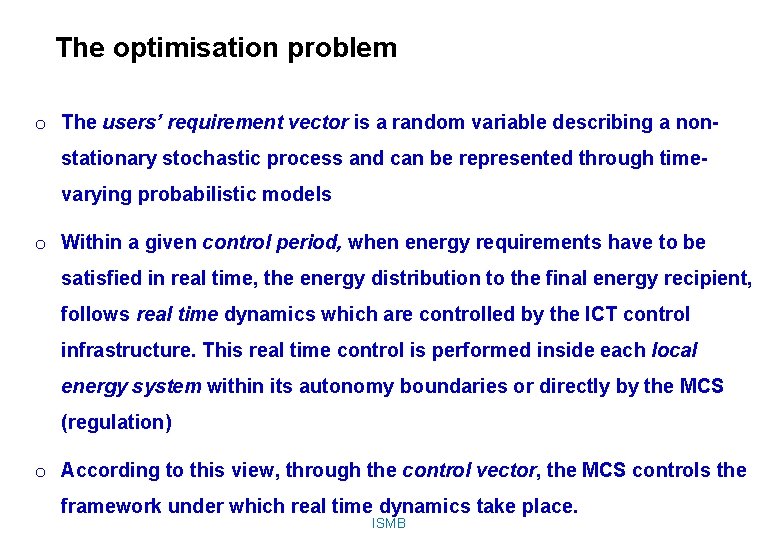
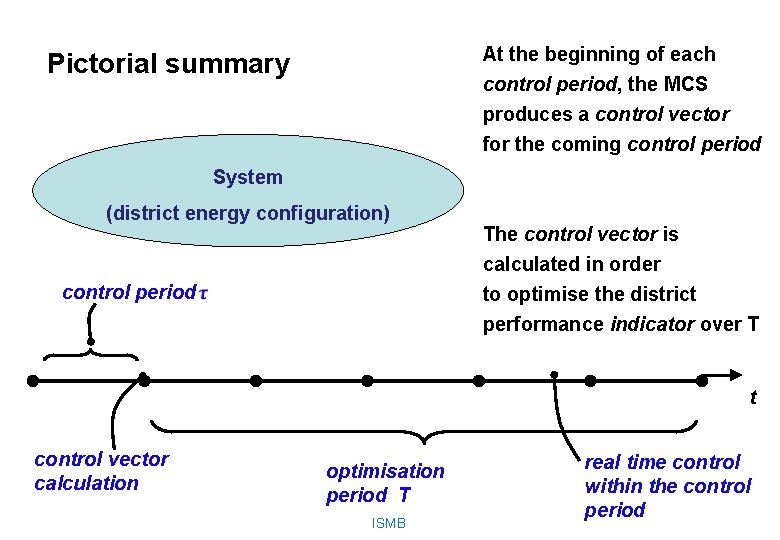
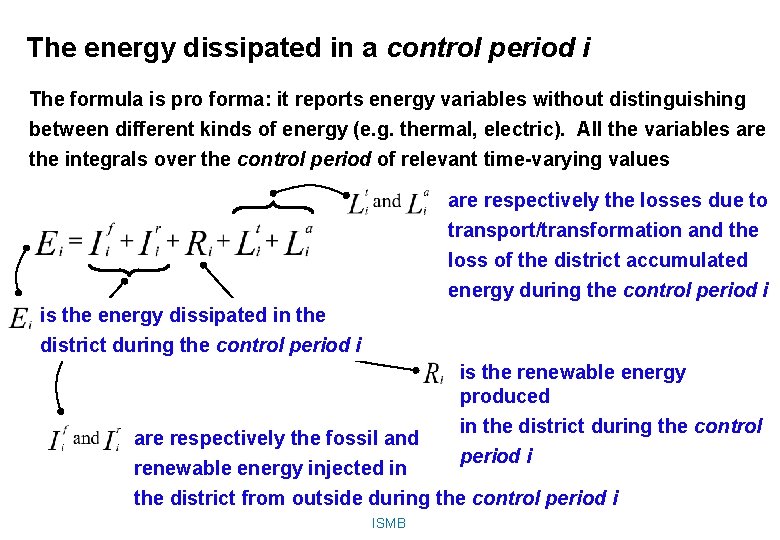
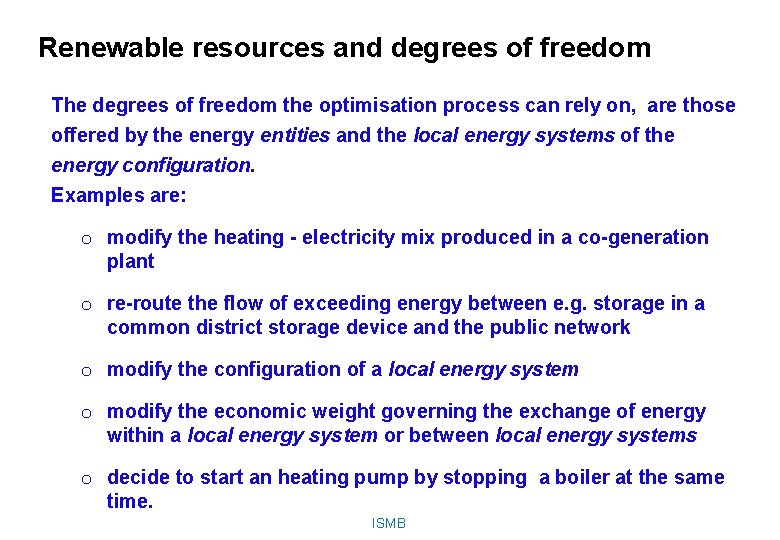
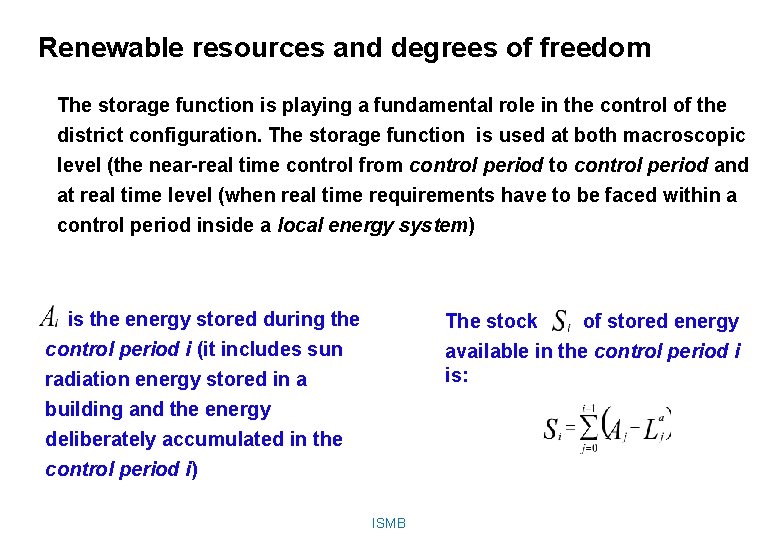
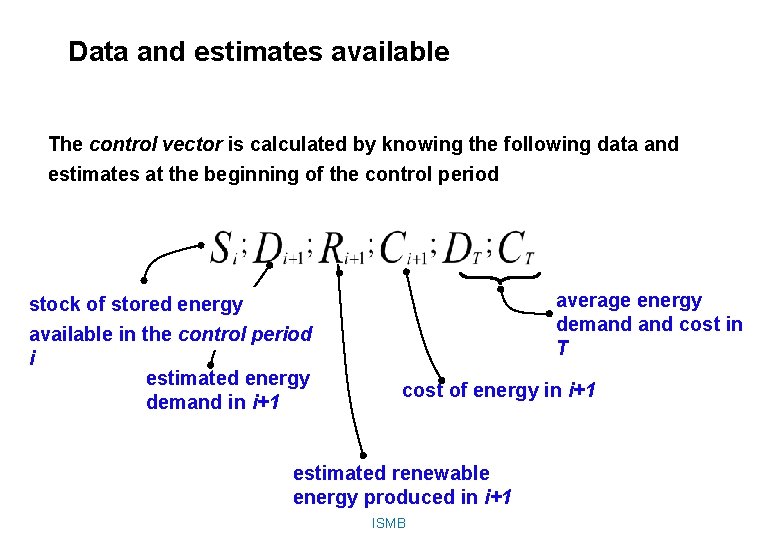
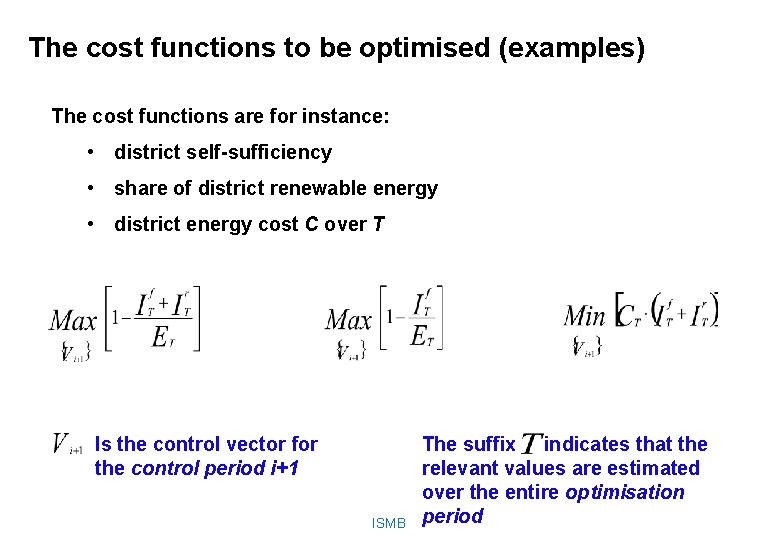
- Slides: 18
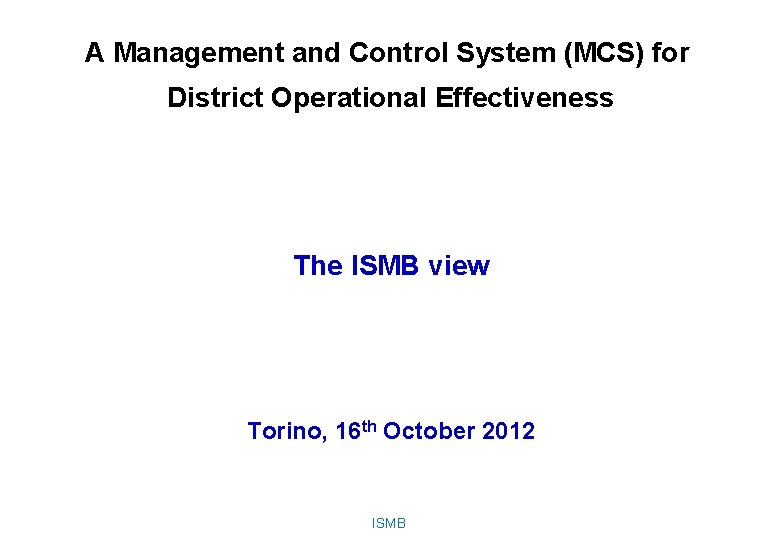
A Management and Control System (MCS) for District Operational Effectiveness The ISMB view Torino, 16 th October 2012 ISMB
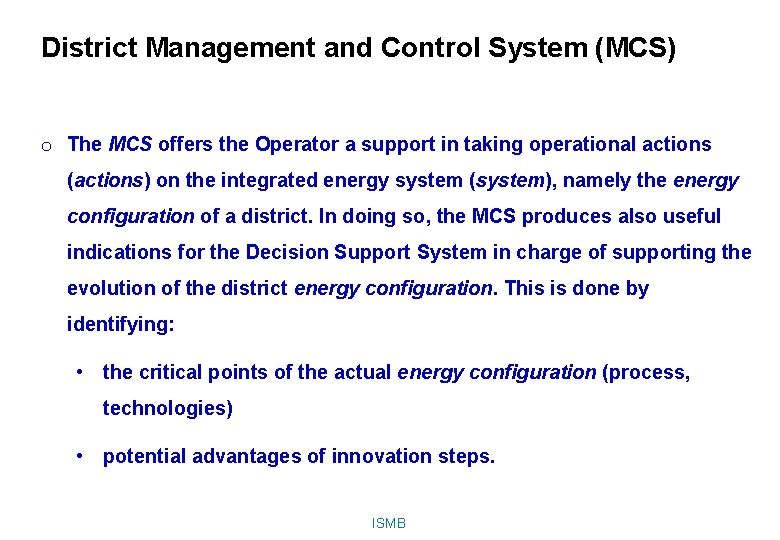
District Management and Control System (MCS) o The MCS offers the Operator a support in taking operational actions (actions) on the integrated energy system (system), namely the energy configuration of a district. In doing so, the MCS produces also useful indications for the Decision Support System in charge of supporting the evolution of the district energy configuration. This is done by identifying: • the critical points of the actual energy configuration (process, technologies) • potential advantages of innovation steps. ISMB
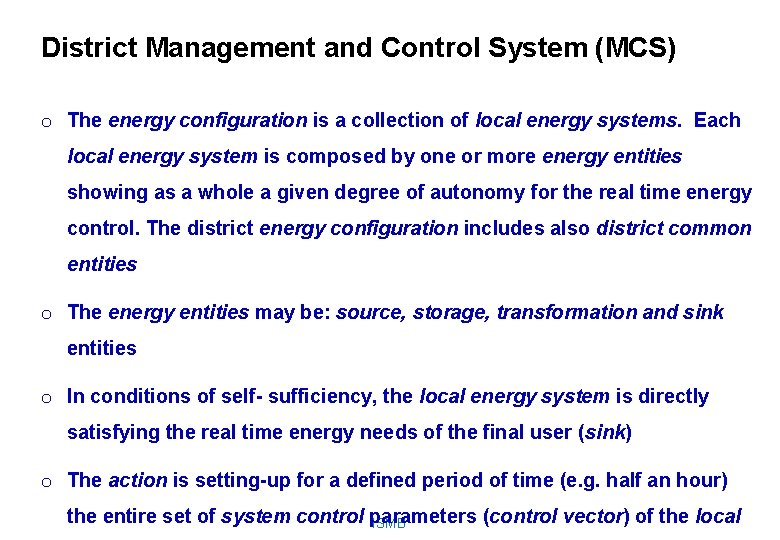
District Management and Control System (MCS) o The energy configuration is a collection of local energy systems. Each local energy system is composed by one or more energy entities showing as a whole a given degree of autonomy for the real time energy control. The district energy configuration includes also district common entities o The energy entities may be: source, storage, transformation and sink entities o In conditions of self- sufficiency, the local energy system is directly satisfying the real time energy needs of the final user (sink) o The action is setting-up for a defined period of time (e. g. half an hour) the entire set of system control parameters (control vector) of the local ISMB
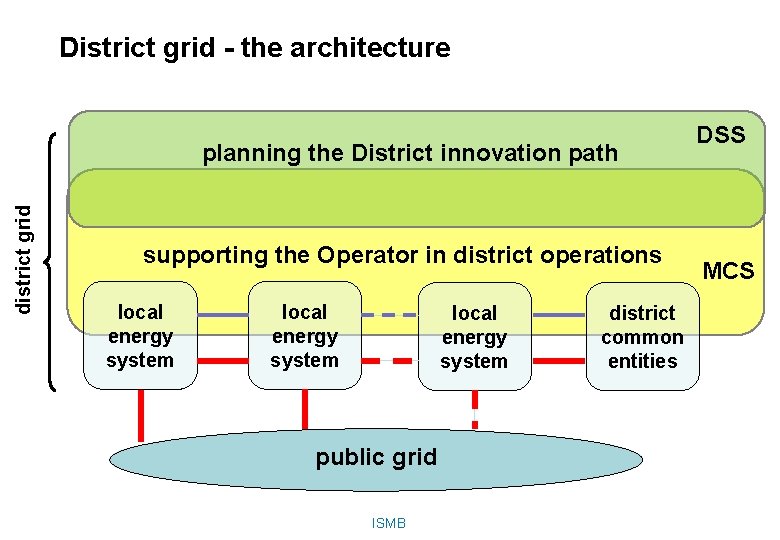
District grid - the architecture district grid planning the District innovation path supporting the Operator in district operations local energy system public grid ISMB district common entities DSS MCS
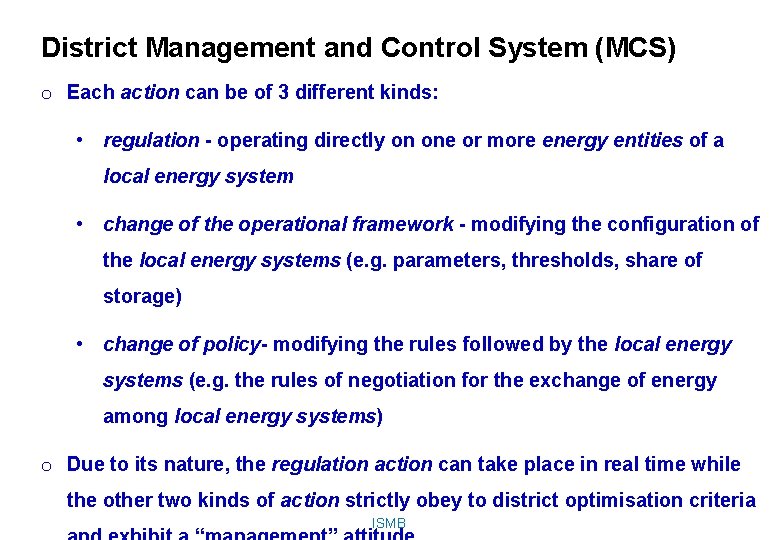
District Management and Control System (MCS) o Each action can be of 3 different kinds: • regulation - operating directly on one or more energy entities of a local energy system • change of the operational framework - modifying the configuration of the local energy systems (e. g. parameters, thresholds, share of storage) • change of policy- modifying the rules followed by the local energy systems (e. g. the rules of negotiation for the exchange of energy among local energy systems) o Due to its nature, the regulation action can take place in real time while the other two kinds of action strictly obey to district optimisation criteria ISMB
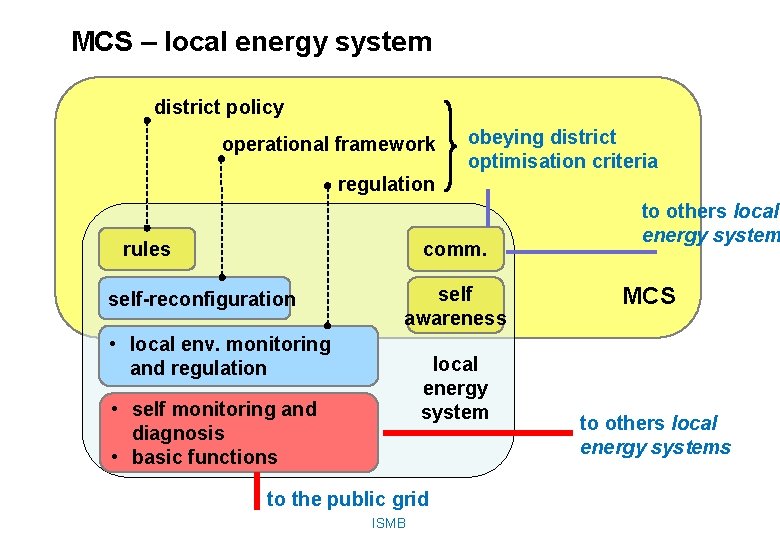
MCS – local energy system district policy operational framework obeying district optimisation criteria regulation comm. rules self-reconfiguration self awareness • local env. monitoring and regulation local energy system • self monitoring and diagnosis • basic functions to the public grid ISMB to others local energy system MCS to others local energy systems
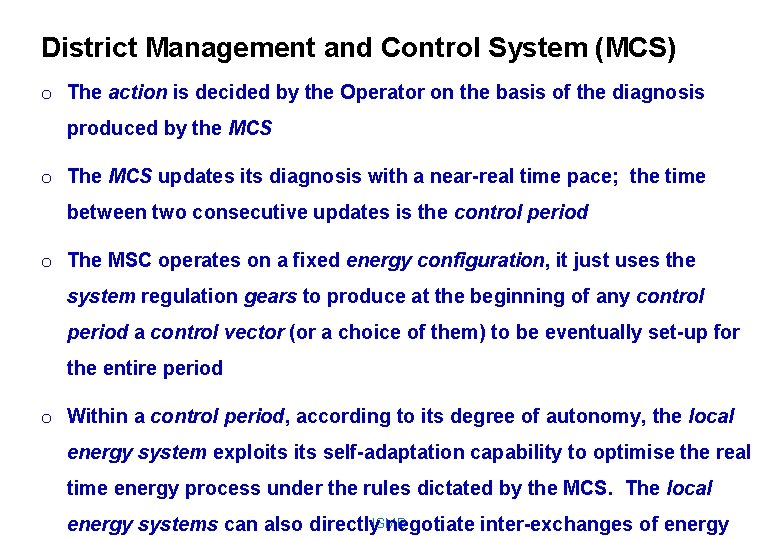
District Management and Control System (MCS) o The action is decided by the Operator on the basis of the diagnosis produced by the MCS o The MCS updates its diagnosis with a near-real time pace; the time between two consecutive updates is the control period o The MSC operates on a fixed energy configuration, it just uses the system regulation gears to produce at the beginning of any control period a control vector (or a choice of them) to be eventually set-up for the entire period o Within a control period, according to its degree of autonomy, the local energy system exploits self-adaptation capability to optimise the real time energy process under the rules dictated by the MCS. The local energy systems can also directly. ISMB negotiate inter-exchanges of energy
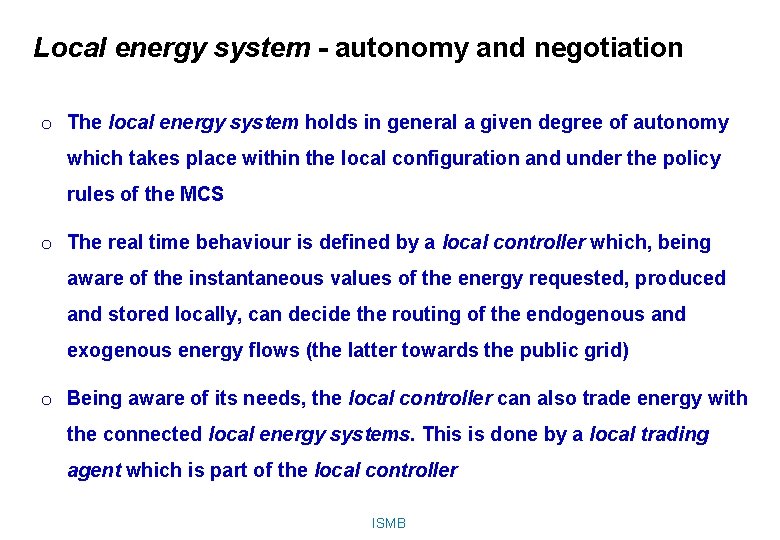
Local energy system - autonomy and negotiation o The local energy system holds in general a given degree of autonomy which takes place within the local configuration and under the policy rules of the MCS o The real time behaviour is defined by a local controller which, being aware of the instantaneous values of the energy requested, produced and stored locally, can decide the routing of the endogenous and exogenous energy flows (the latter towards the public grid) o Being aware of its needs, the local controller can also trade energy with the connected local energy systems. This is done by a local trading agent which is part of the local controller ISMB
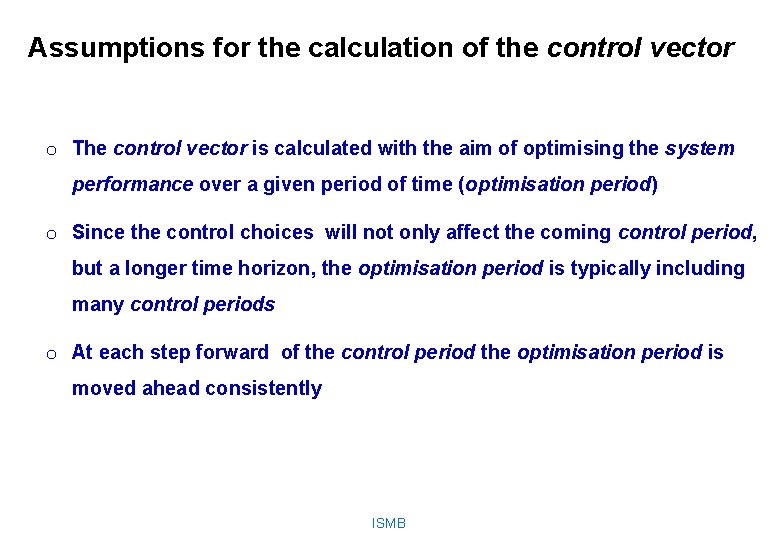
Assumptions for the calculation of the control vector o The control vector is calculated with the aim of optimising the system performance over a given period of time (optimisation period) o Since the control choices will not only affect the coming control period, but a longer time horizon, the optimisation period is typically including many control periods o At each step forward of the control period the optimisation period is moved ahead consistently ISMB
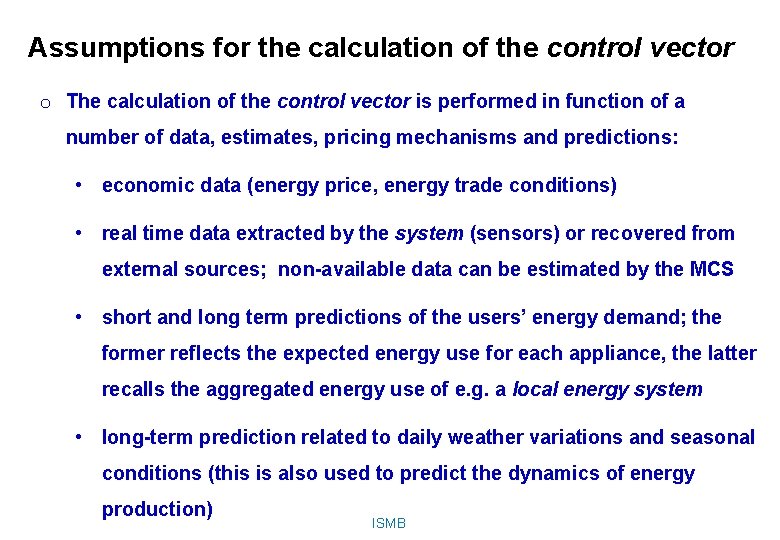
Assumptions for the calculation of the control vector o The calculation of the control vector is performed in function of a number of data, estimates, pricing mechanisms and predictions: • economic data (energy price, energy trade conditions) • real time data extracted by the system (sensors) or recovered from external sources; non-available data can be estimated by the MCS • short and long term predictions of the users’ energy demand; the former reflects the expected energy use for each appliance, the latter recalls the aggregated energy use of e. g. a local energy system • long-term prediction related to daily weather variations and seasonal conditions (this is also used to predict the dynamics of energy production) ISMB
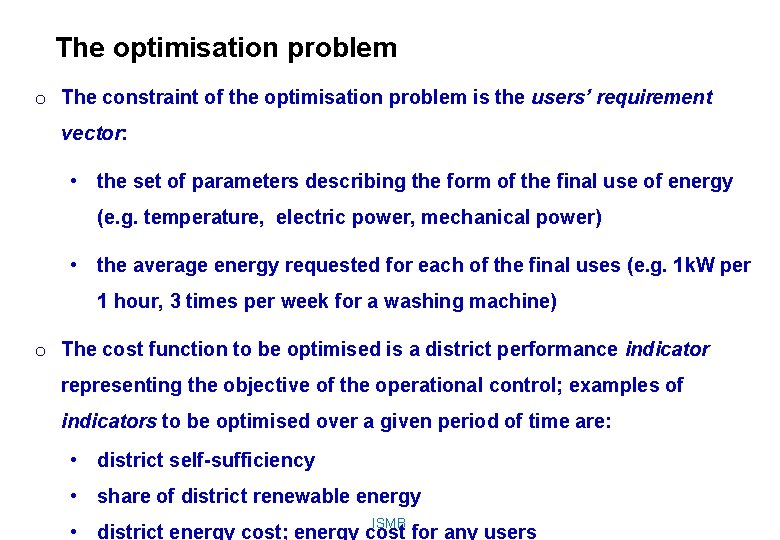
The optimisation problem o The constraint of the optimisation problem is the users’ requirement vector: • the set of parameters describing the form of the final use of energy (e. g. temperature, electric power, mechanical power) • the average energy requested for each of the final uses (e. g. 1 k. W per 1 hour, 3 times per week for a washing machine) o The cost function to be optimised is a district performance indicator representing the objective of the operational control; examples of indicators to be optimised over a given period of time are: • district self-sufficiency • share of district renewable energy ISMB • district energy cost; energy cost for any users
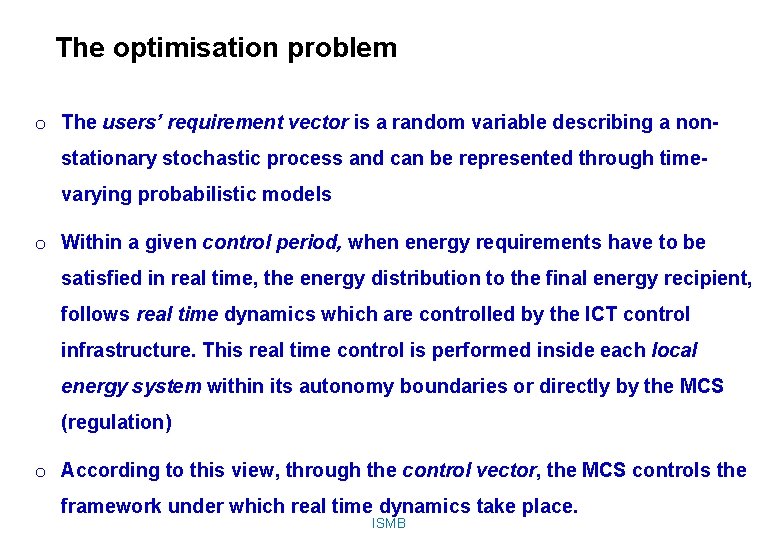
The optimisation problem o The users’ requirement vector is a random variable describing a nonstationary stochastic process and can be represented through timevarying probabilistic models o Within a given control period, when energy requirements have to be satisfied in real time, the energy distribution to the final energy recipient, follows real time dynamics which are controlled by the ICT control infrastructure. This real time control is performed inside each local energy system within its autonomy boundaries or directly by the MCS (regulation) o According to this view, through the control vector, the MCS controls the framework under which real time dynamics take place. ISMB
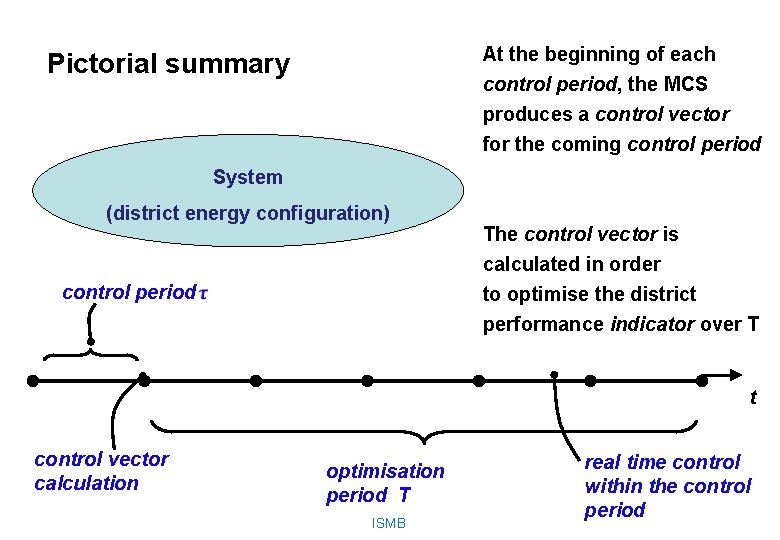
At the beginning of each control period, the MCS Pictorial summary produces a control vector for the coming control period System (district energy configuration) control period The control vector is calculated in order to optimise the district performance indicator over T t control vector calculation optimisation period T ISMB real time control within the control period
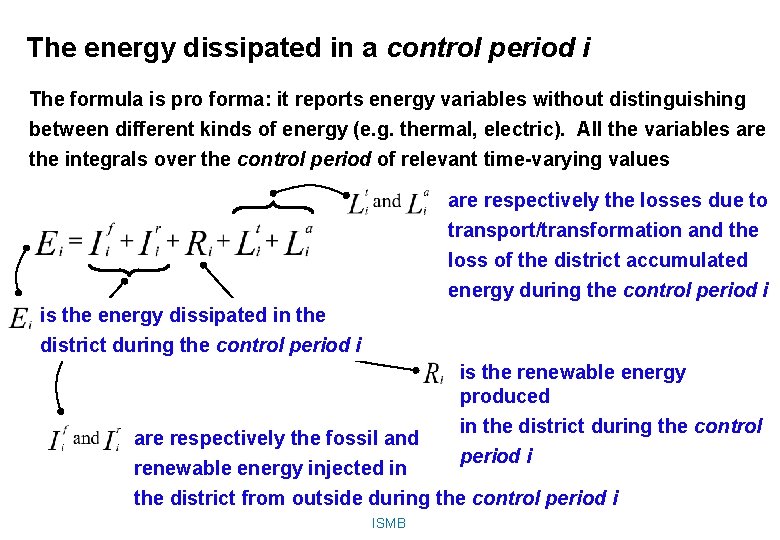
The energy dissipated in a control period i The formula is pro forma: it reports energy variables without distinguishing between different kinds of energy (e. g. thermal, electric). All the variables are the integrals over the control period of relevant time-varying values are respectively the losses due to transport/transformation and the loss of the district accumulated energy during the control period i is the energy dissipated in the district during the control period i is the renewable energy produced are respectively the fossil and renewable energy injected in in the district during the control period i the district from outside during the control period i ISMB
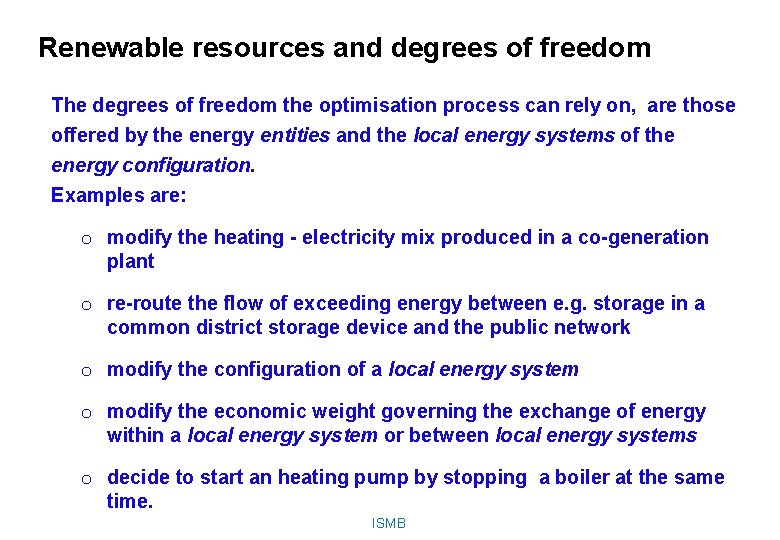
Renewable resources and degrees of freedom The degrees of freedom the optimisation process can rely on, are those offered by the energy entities and the local energy systems of the energy configuration. Examples are: o modify the heating - electricity mix produced in a co-generation plant o re-route the flow of exceeding energy between e. g. storage in a common district storage device and the public network o modify the configuration of a local energy system o modify the economic weight governing the exchange of energy within a local energy system or between local energy systems o decide to start an heating pump by stopping a boiler at the same time. ISMB
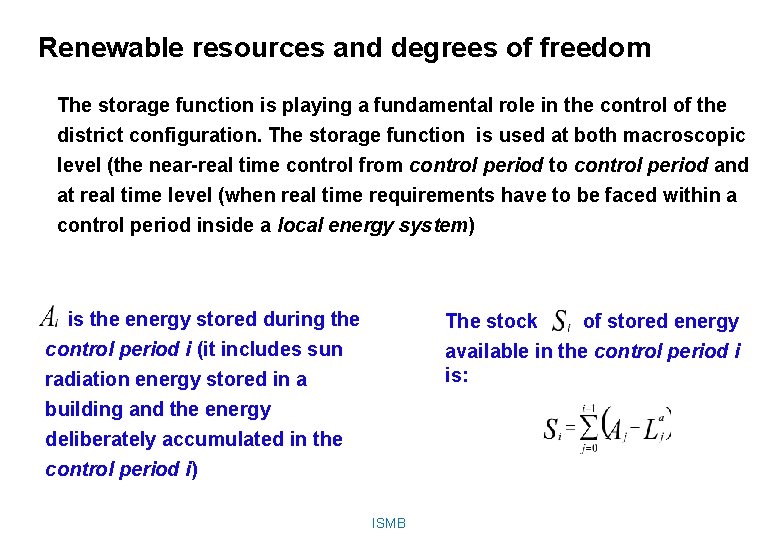
Renewable resources and degrees of freedom The storage function is playing a fundamental role in the control of the district configuration. The storage function is used at both macroscopic level (the near-real time control from control period to control period and at real time level (when real time requirements have to be faced within a control period inside a local energy system) is the energy stored during the control period i (it includes sun The stock of stored energy available in the control period i is: radiation energy stored in a building and the energy deliberately accumulated in the control period i) ISMB
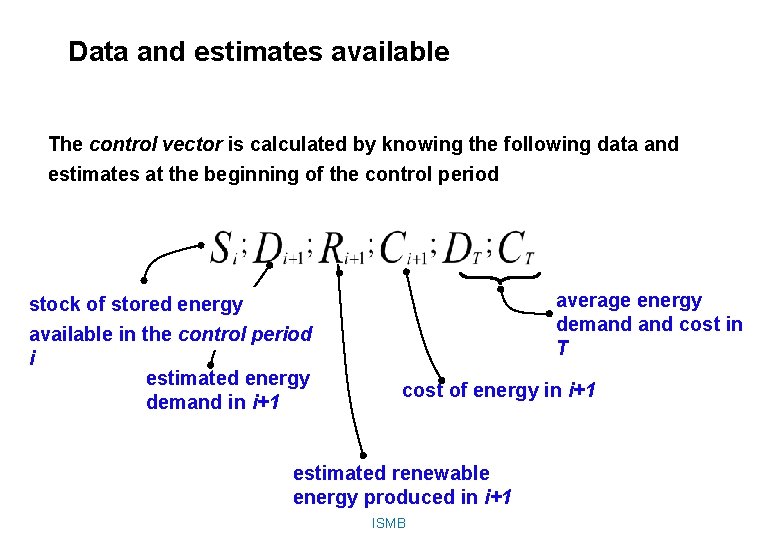
Data and estimates available The control vector is calculated by knowing the following data and estimates at the beginning of the control period stock of stored energy available in the control period i estimated energy demand in i+1 average energy demand cost in T cost of energy in i+1 estimated renewable energy produced in i+1 ISMB
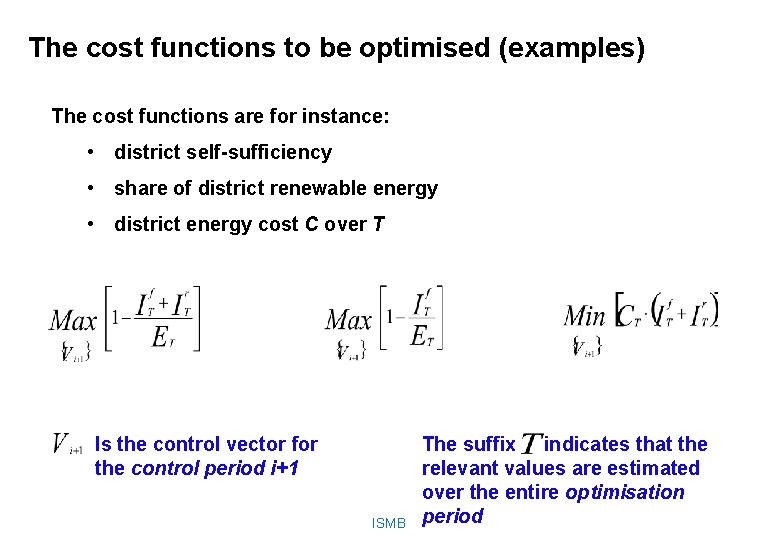
The cost functions to be optimised (examples) The cost functions are for instance: • district self-sufficiency • share of district renewable energy • district energy cost C over T Is the control vector for the control period i+1 ISMB The suffix indicates that the relevant values are estimated over the entire optimisation period