4 th EIC Workshop Hampton University 19 23
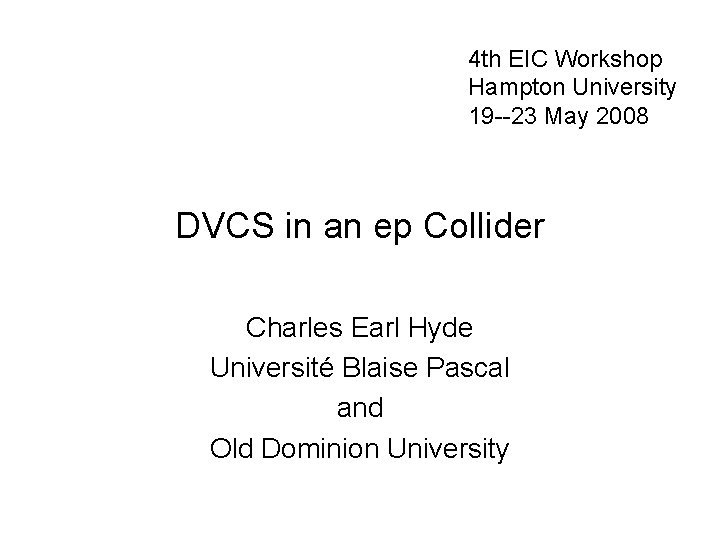
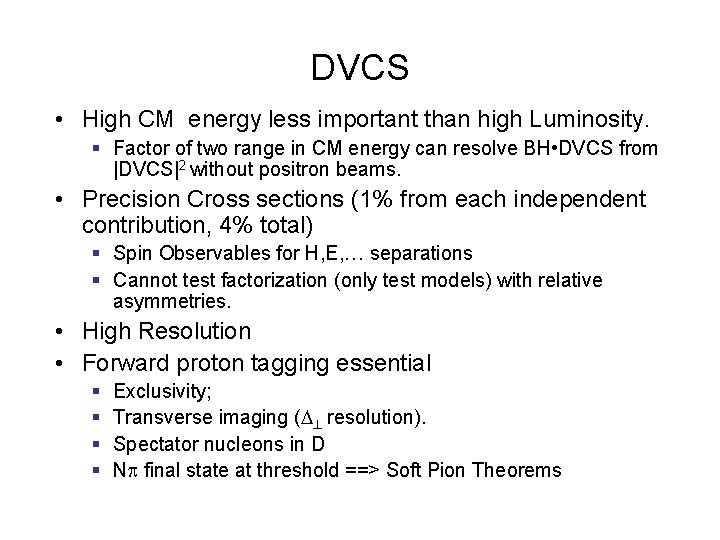
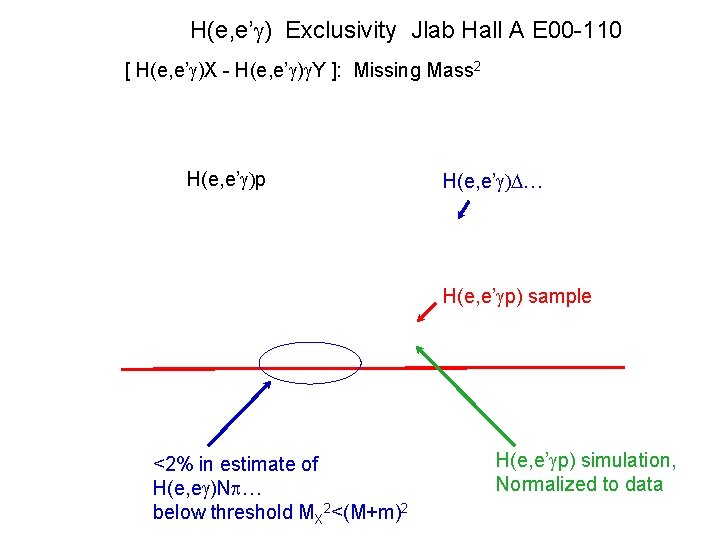
![JLab Hall A Cross Sections C. Munoz Camacho et al PRL 97 (2006) Im[BH*DVCS] JLab Hall A Cross Sections C. Munoz Camacho et al PRL 97 (2006) Im[BH*DVCS]](https://slidetodoc.com/presentation_image_h2/14c076911041f5f17a2b644a9d8c77ee/image-4.jpg)
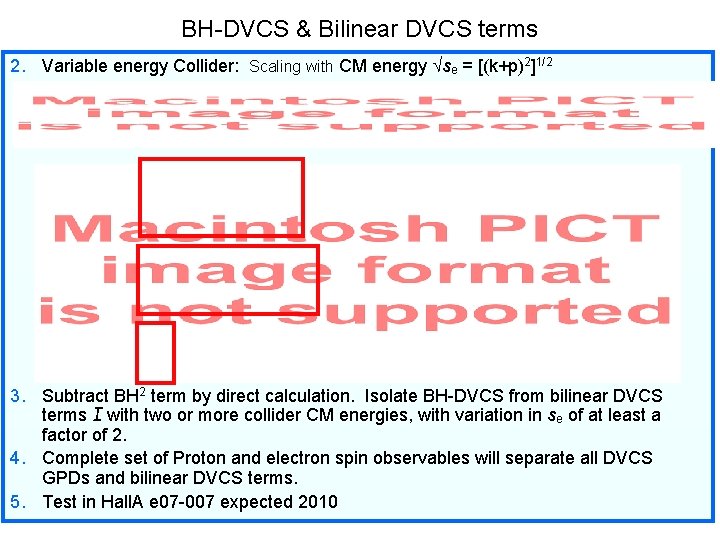
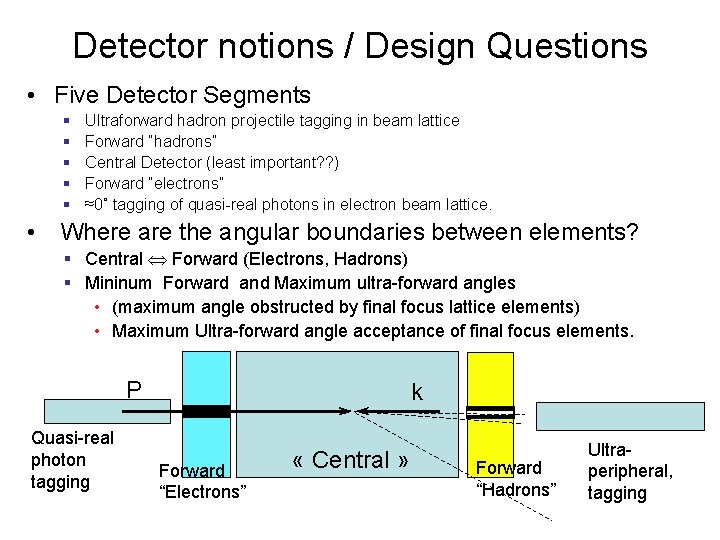
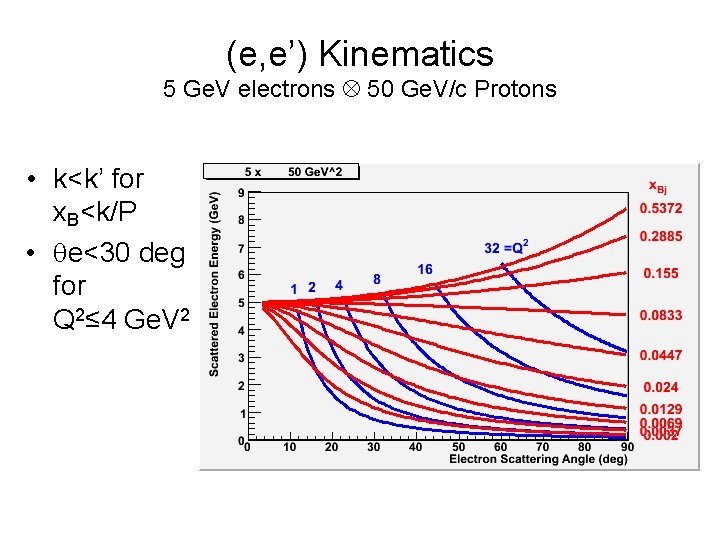
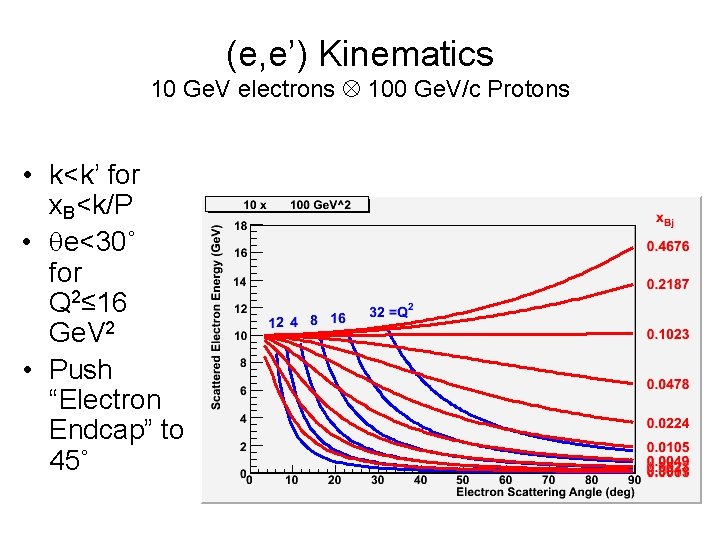
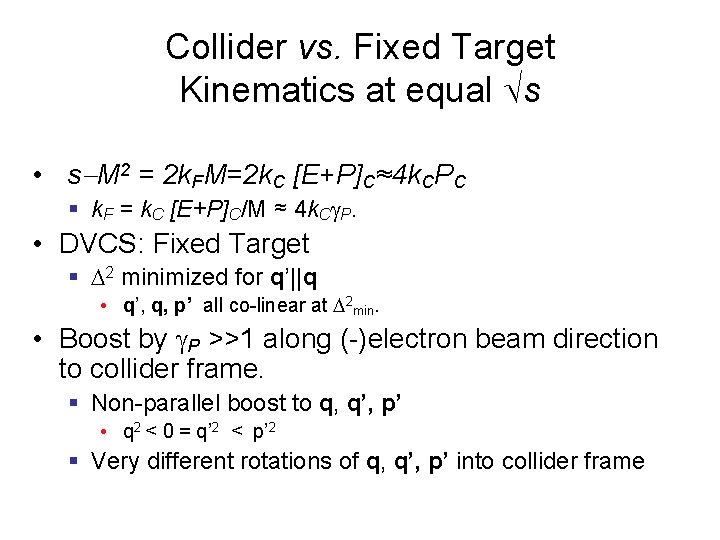
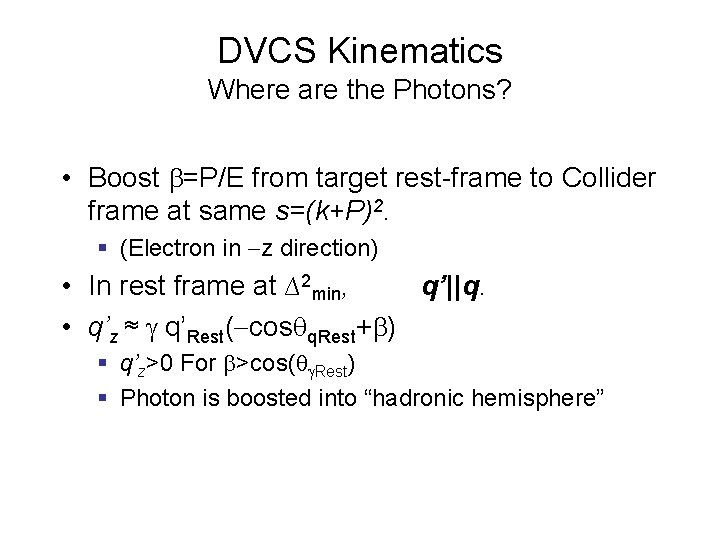
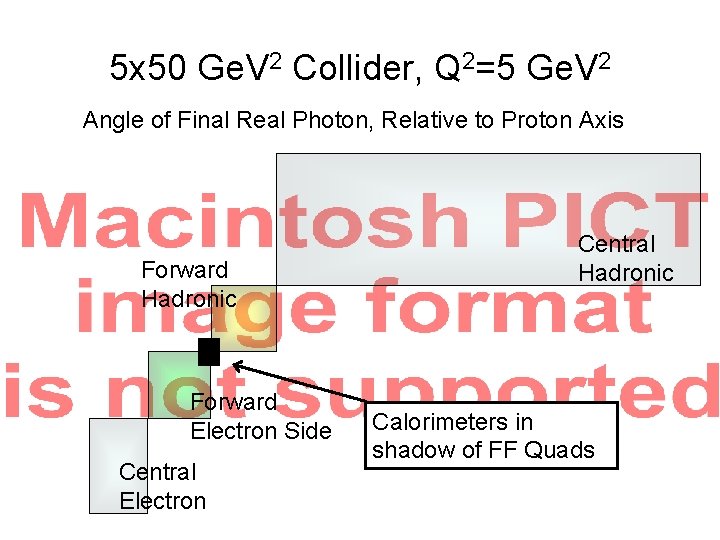
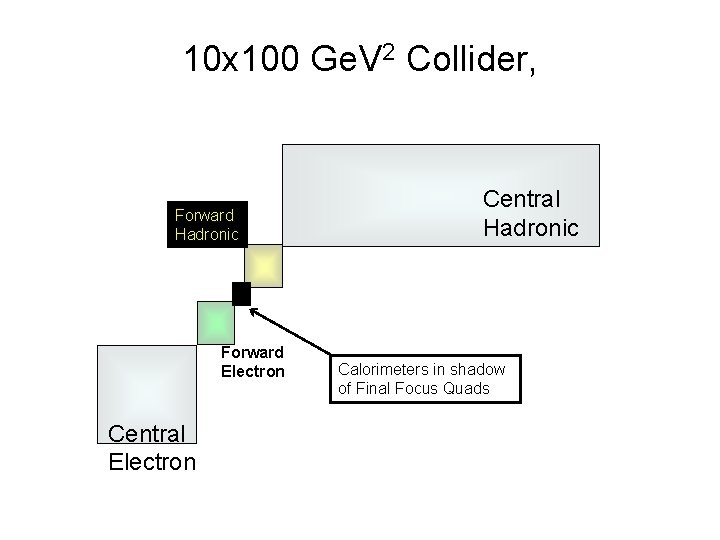
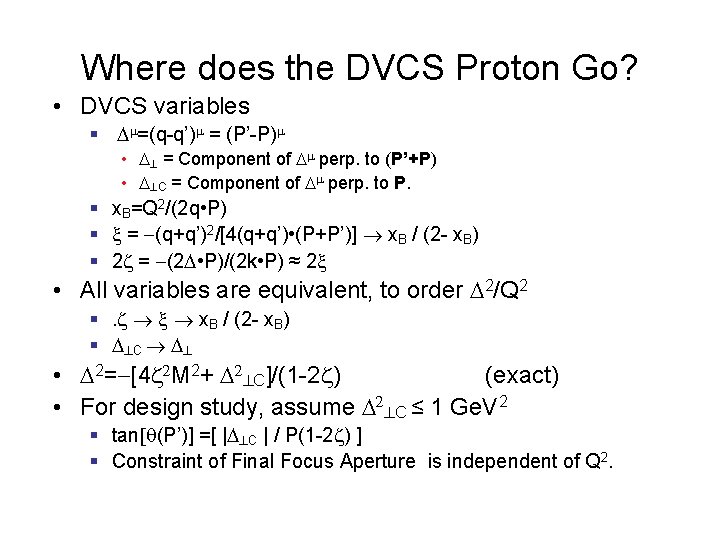
![Final Focus Aperture R at Distance L & DVCS “Recoil” Protons • Constraint [R/L] Final Focus Aperture R at Distance L & DVCS “Recoil” Protons • Constraint [R/L]](https://slidetodoc.com/presentation_image_h2/14c076911041f5f17a2b644a9d8c77ee/image-14.jpg)
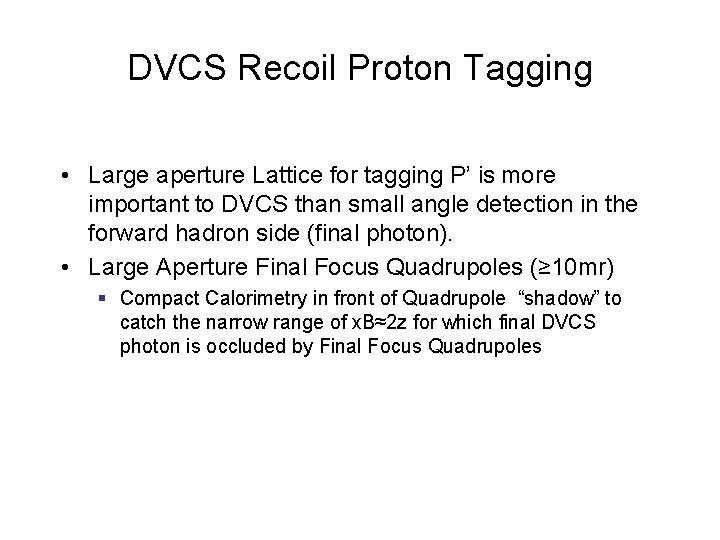
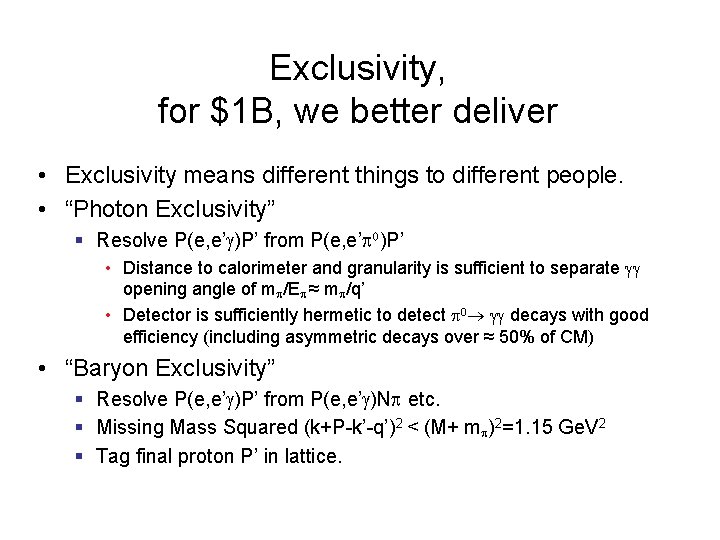
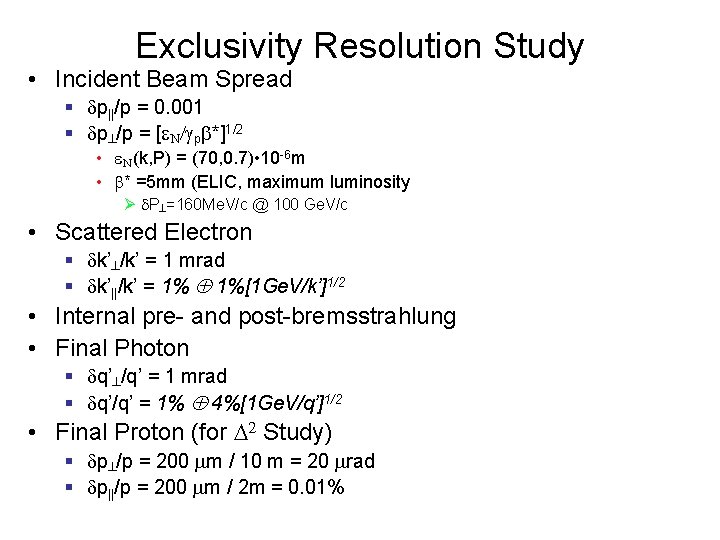
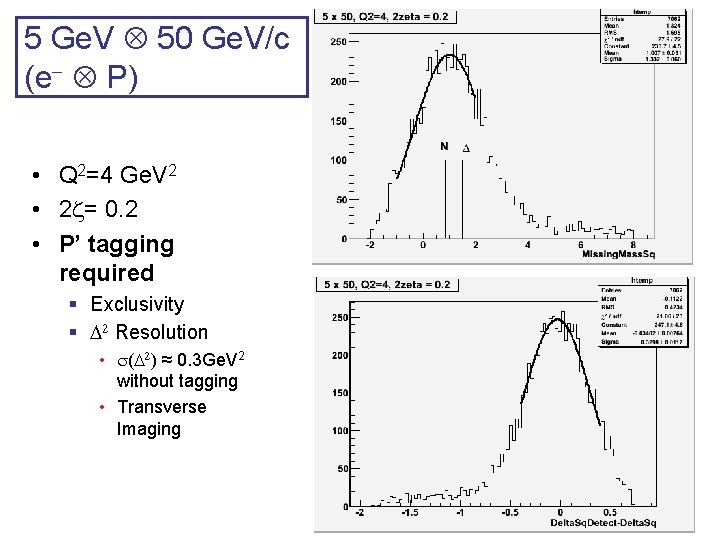
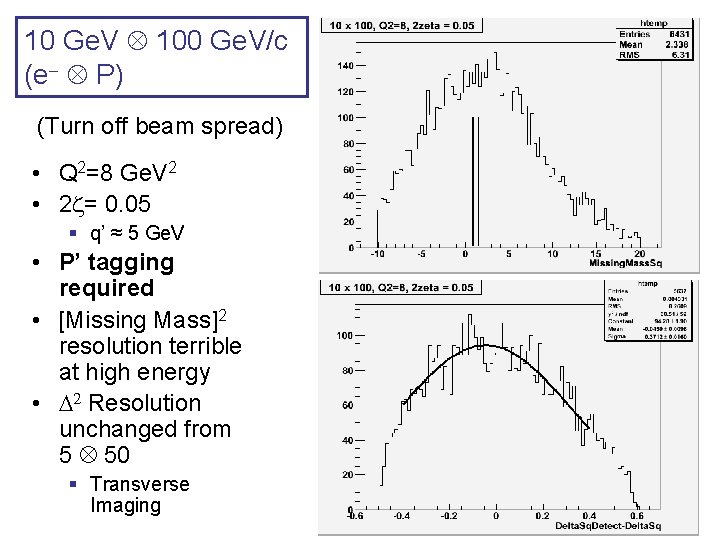
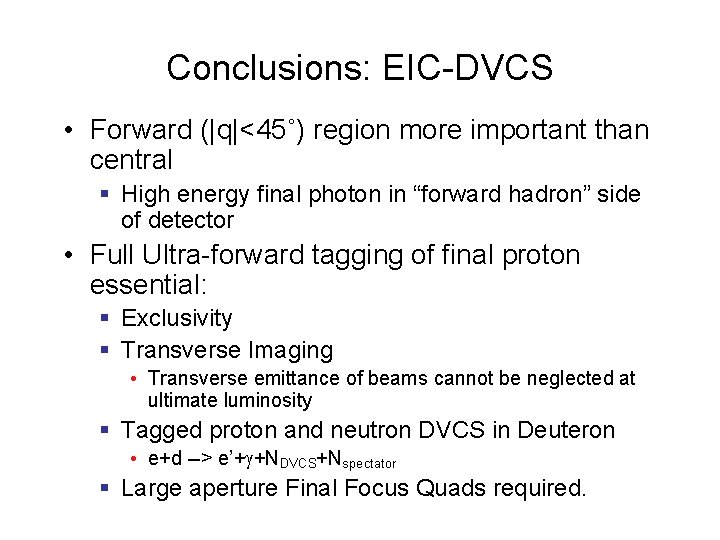
- Slides: 20
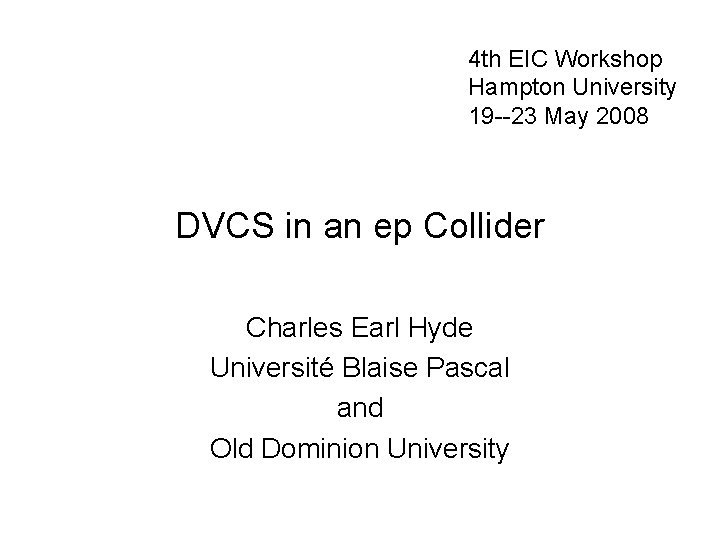
4 th EIC Workshop Hampton University 19 --23 May 2008 DVCS in an ep Collider Charles Earl Hyde Université Blaise Pascal and Old Dominion University
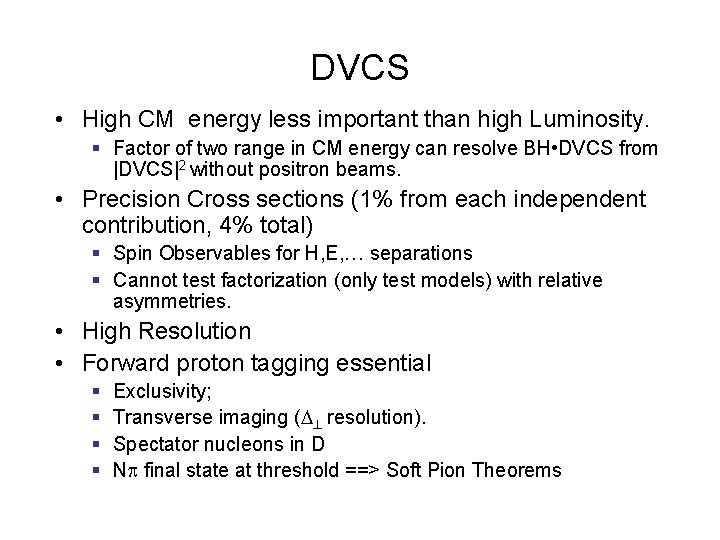
DVCS • High CM energy less important than high Luminosity. § Factor of two range in CM energy can resolve BH • DVCS from |DVCS|2 without positron beams. • Precision Cross sections (1% from each independent contribution, 4% total) § Spin Observables for H, E, … separations § Cannot test factorization (only test models) with relative asymmetries. • High Resolution • Forward proton tagging essential § § Exclusivity; Transverse imaging ( resolution). Spectator nucleons in D N final state at threshold ==> Soft Pion Theorems
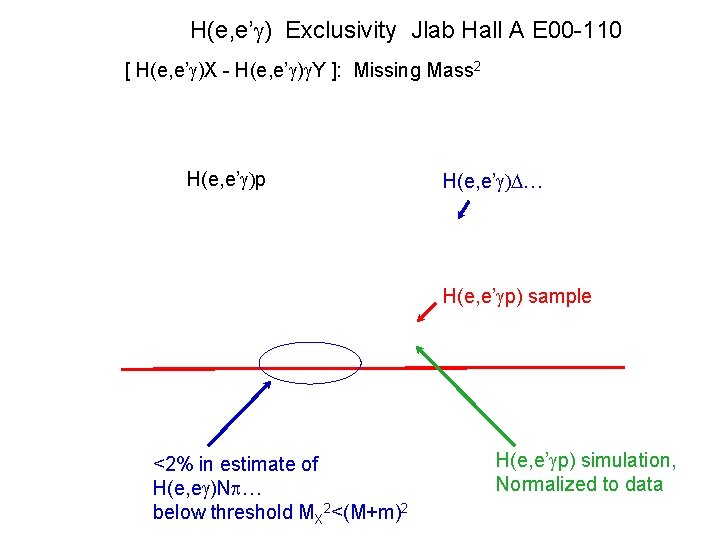
H(e, e’ ) Exclusivity Jlab Hall A E 00 -110 [ H(e, e’ )X - H(e, e’ ) Y ]: Missing Mass 2 H(e, e’ p H(e, e’ … H(e, e’ p) sample <2% in estimate of H(e, e )N … below threshold MX 2<(M+m)2 H(e, e’ p) simulation, Normalized to data
![JLab Hall A Cross Sections C Munoz Camacho et al PRL 97 2006 ImBHDVCS JLab Hall A Cross Sections C. Munoz Camacho et al PRL 97 (2006) Im[BH*DVCS]](https://slidetodoc.com/presentation_image_h2/14c076911041f5f17a2b644a9d8c77ee/image-4.jpg)
JLab Hall A Cross Sections C. Munoz Camacho et al PRL 97 (2006) Im[BH*DVCS] BH 2 Re[BH*DVCS] + |DVCS|2 • • Isolation of Re and Im parts of Twist-2 DVCS-BH interference. Kinematic weight of DVCS 2 term is ≤ 1% in Im[BH*DVCS], 4. 5% in Re. § DVCS 2 term in VGG ≈ 20.
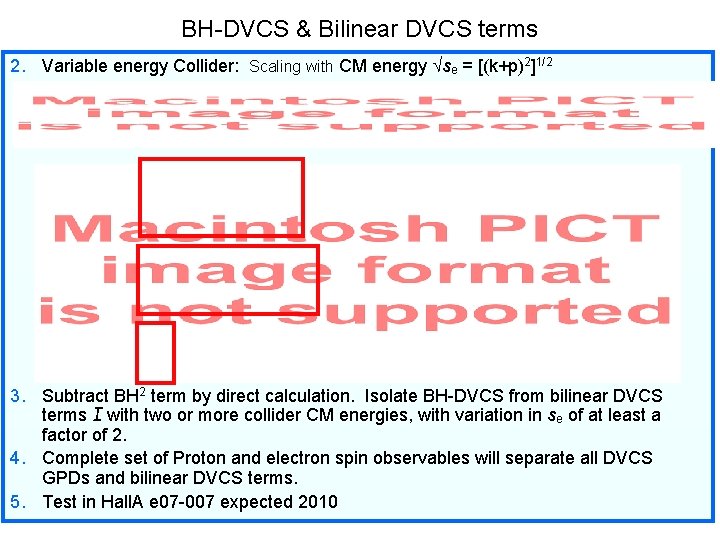
BH-DVCS & Bilinear DVCS terms 2. Variable energy Collider: Scaling with CM energy se = [(k+p)2]1/2 3. Subtract BH 2 term by direct calculation. Isolate BH-DVCS from bilinear DVCS terms I with two or more collider CM energies, with variation in se of at least a factor of 2. 4. Complete set of Proton and electron spin observables will separate all DVCS GPDs and bilinear DVCS terms. 5. Test in Hall. A e 07 -007 expected 2010
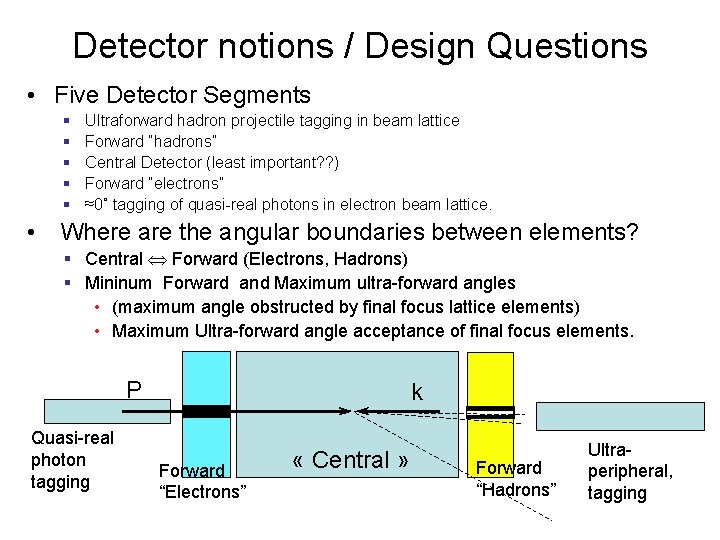
Detector notions / Design Questions • Five Detector Segments § § § • Ultraforward hadron projectile tagging in beam lattice Forward “hadrons” Central Detector (least important? ? ) Forward “electrons” ≈0˚ tagging of quasi-real photons in electron beam lattice. Where are the angular boundaries between elements? § Central Forward (Electrons, Hadrons) § Mininum Forward and Maximum ultra-forward angles • (maximum angle obstructed by final focus lattice elements) • Maximum Ultra-forward angle acceptance of final focus elements. P Quasi-real photon tagging k Forward “Electrons” « Central » Forward “Hadrons” Ultraperipheral, tagging
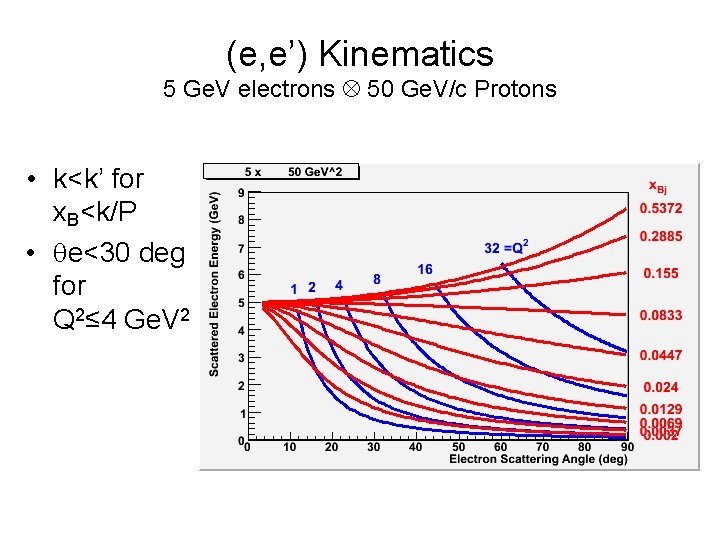
(e, e’) Kinematics 5 Ge. V electrons 50 Ge. V/c Protons • k<k’ for x. B<k/P • e<30 deg for Q 2≤ 4 Ge. V 2
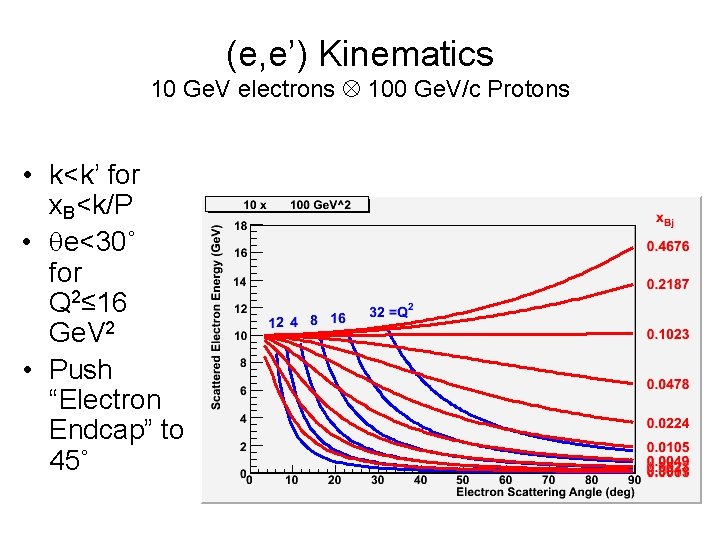
(e, e’) Kinematics 10 Ge. V electrons 100 Ge. V/c Protons • k<k’ for x. B<k/P • e<30˚ for Q 2≤ 16 Ge. V 2 • Push “Electron Endcap” to 45˚
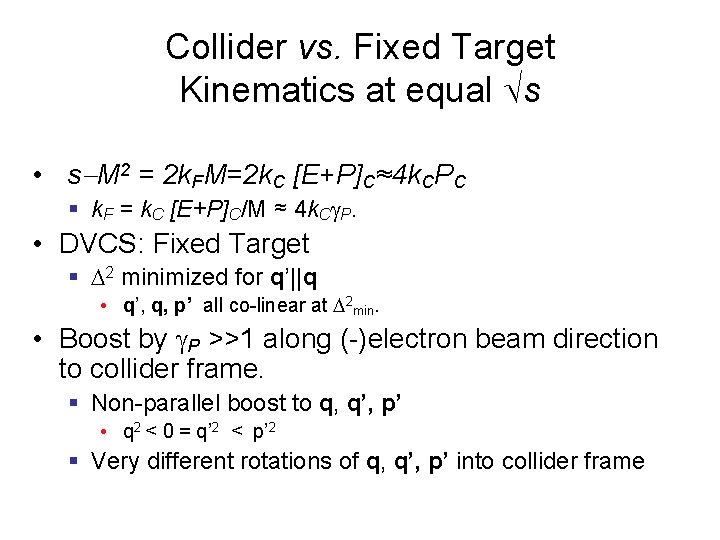
Collider vs. Fixed Target Kinematics at equal s • s M 2 = 2 k. FM=2 k. C [E+P]C≈4 k. CPC § k. F = k. C [E+P]C/M ≈ 4 k. C P. • DVCS: Fixed Target § 2 minimized for q’||q • q’, q, p’ all co-linear at 2 min. • Boost by P >>1 along (-)electron beam direction to collider frame. § Non-parallel boost to q, q’, p’ • q 2 < 0 = q’ 2 < p’ 2 § Very different rotations of q, q’, p’ into collider frame
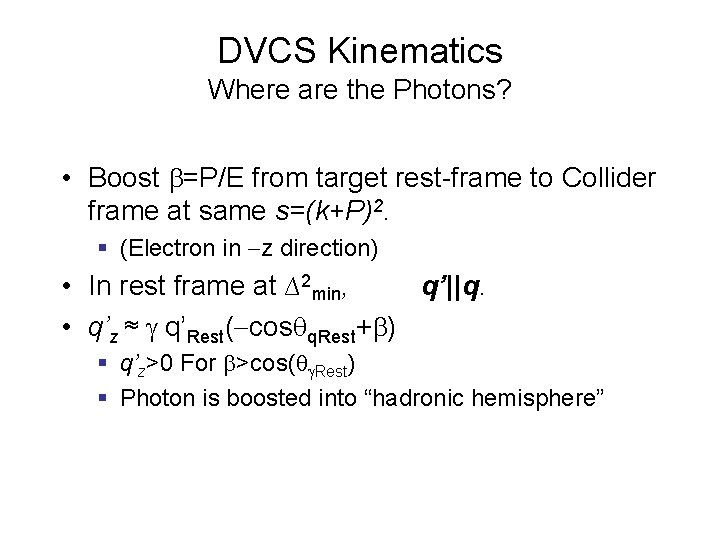
DVCS Kinematics Where are the Photons? • Boost =P/E from target rest-frame to Collider frame at same s=(k+P)2. § (Electron in z direction) • In rest frame at ∆2 min, q’||q. • q’z ≈ q’Rest( cos q. Rest+ ) § q’z>0 For >cos( Rest) § Photon is boosted into “hadronic hemisphere”
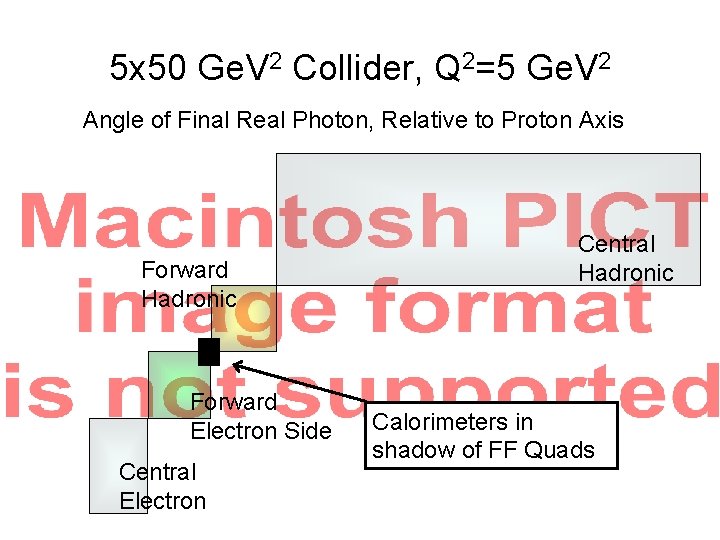
5 x 50 Ge. V 2 Collider, Q 2=5 Ge. V 2 Angle of Final Real Photon, Relative to Proton Axis Forward Hadronic Forward Electron Side Central Electron Central Hadronic Calorimeters in shadow of FF Quads
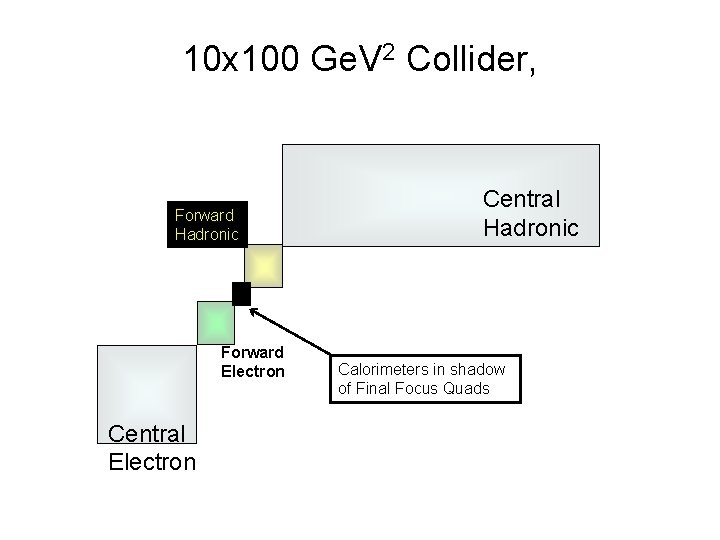
10 x 100 Ge. V 2 Collider, Forward Hadronic Forward Electron Central Hadronic Calorimeters in shadow of Final Focus Quads
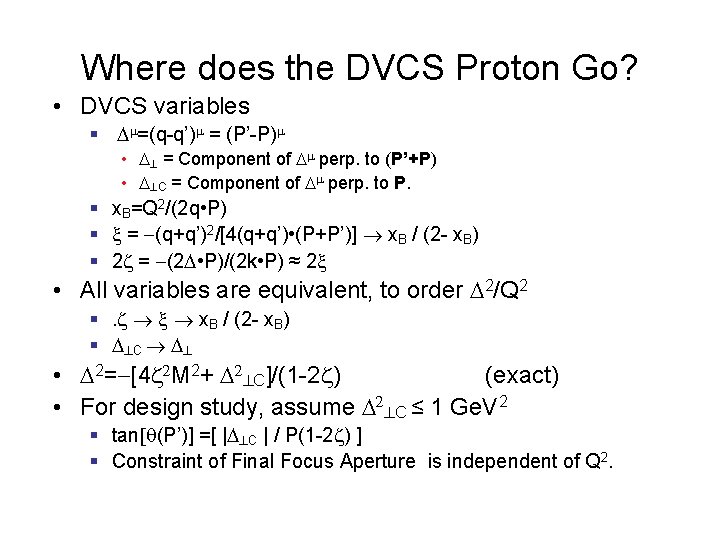
Where does the DVCS Proton Go? • DVCS variables § =(q-q’) = (P’-P) • = Component of perp. to (P’+P) • C = Component of perp. to P. § x. B=Q 2/(2 q • P) § = (q+q’)2/[4(q+q’) • (P+P’)] x. B / (2 - x. B) § 2 = (2 • P)/(2 k • P) ≈ 2 • All variables are equivalent, to order 2/Q 2 §. x. B / (2 - x. B) § C • 2= [4 M 2+ C]/(1 -2 ) (exact) • For design study, assume C ≤ 1 Ge. V 2 § tan (P’)] =[ | C | / P(1 -2 ) ] § Constraint of Final Focus Aperture is independent of Q 2.
![Final Focus Aperture R at Distance L DVCS Recoil Protons Constraint RL Final Focus Aperture R at Distance L & DVCS “Recoil” Protons • Constraint [R/L]](https://slidetodoc.com/presentation_image_h2/14c076911041f5f17a2b644a9d8c77ee/image-14.jpg)
Final Focus Aperture R at Distance L & DVCS “Recoil” Protons • Constraint [R/L] > [ | C | / P(1 -2 ) ] § 5 mr limits | C | < 500 Me. V/c at P=100 Ge. V/c § 5 mr limits | C | < 250 Me. V/c at P=50 Ge. V/c § Pion p from N final state p < 60 Me. V/c ≈ [ 5 mr] [m P/(M+m )]
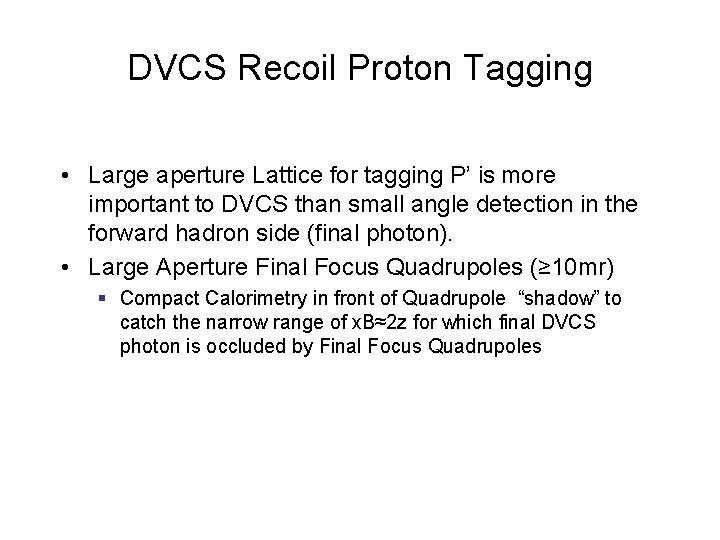
DVCS Recoil Proton Tagging • Large aperture Lattice for tagging P’ is more important to DVCS than small angle detection in the forward hadron side (final photon). • Large Aperture Final Focus Quadrupoles (≥ 10 mr) § Compact Calorimetry in front of Quadrupole “shadow” to catch the narrow range of x. B≈2 z for which final DVCS photon is occluded by Final Focus Quadrupoles
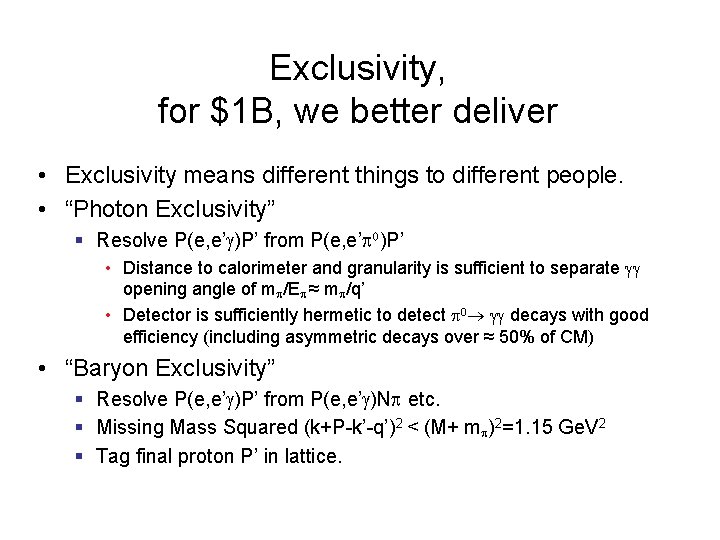
Exclusivity, for $1 B, we better deliver • Exclusivity means different things to different people. • “Photon Exclusivity” § Resolve P(e, e’ )P’ from P(e, e’ )P’ • Distance to calorimeter and granularity is sufficient to separate opening angle of m /E ≈ m /q’ • Detector is sufficiently hermetic to detect decays with good efficiency (including asymmetric decays over ≈ 50% of CM) • “Baryon Exclusivity” § Resolve P(e, e’ )P’ from P(e, e’ )N etc. § Missing Mass Squared (k+P-k’-q’)2 < (M+ m )2=1. 15 Ge. V 2 § Tag final proton P’ in lattice.
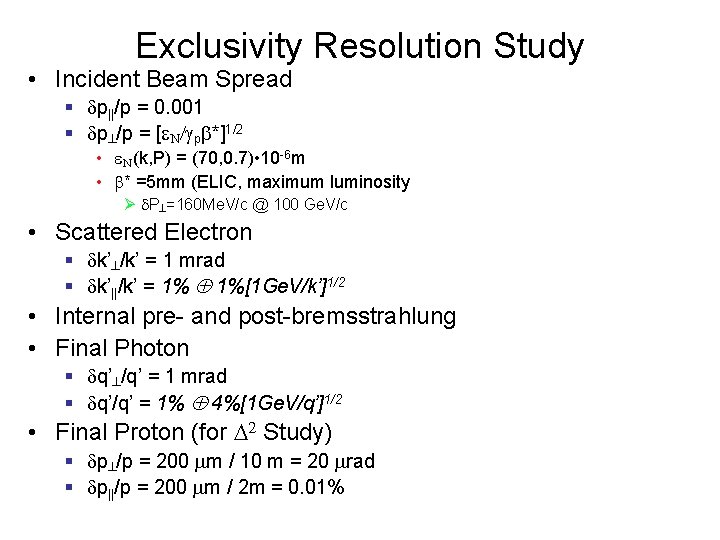
Exclusivity Resolution Study • Incident Beam Spread § p||/p = 0. 001 § p /p = [ p *]1/2 • (k, P) = (70, 0. 7) • 10 -6 m • * =5 mm (ELIC, maximum luminosity Ø P =160 Me. V/c @ 100 Ge. V/c • Scattered Electron § k’ /k’ = 1 mrad § k’||/k’ = 1% 1%[1 Ge. V/k’]1/2 • Internal pre- and post-bremsstrahlung • Final Photon § q’ /q’ = 1 mrad § q’/q’ = 1% 4%[1 Ge. V/q’]1/2 • Final Proton (for Study) § p /p = 200 m / 10 m = 20 rad § p||/p = 200 m / 2 m = 0. 01%
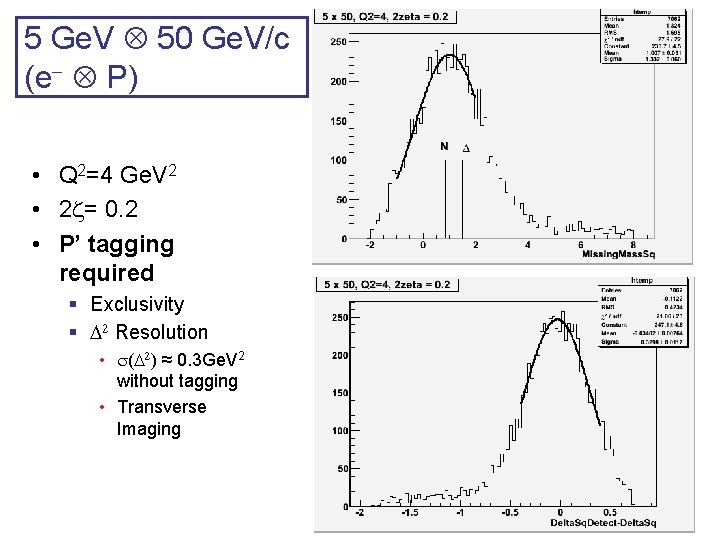
5 Ge. V 50 Ge. V/c (e P) • Q 2=4 Ge. V 2 • 2 = 0. 2 • P’ tagging required § Exclusivity § Resolution • ( ) ≈ 0. 3 Ge. V 2 without tagging • Transverse Imaging
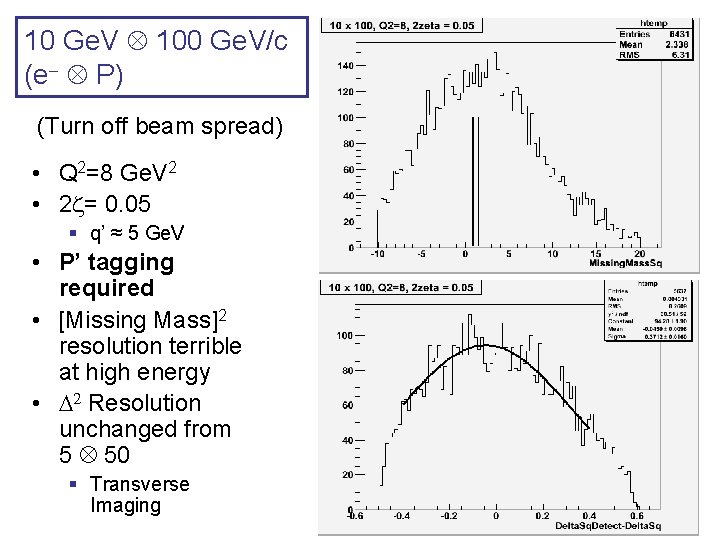
10 Ge. V 100 Ge. V/c (e P) (Turn off beam spread) • Q 2=8 Ge. V 2 • 2 = 0. 05 § q’ ≈ 5 Ge. V • P’ tagging required • [Missing Mass]2 resolution terrible at high energy • Resolution unchanged from 5 50 § Transverse Imaging
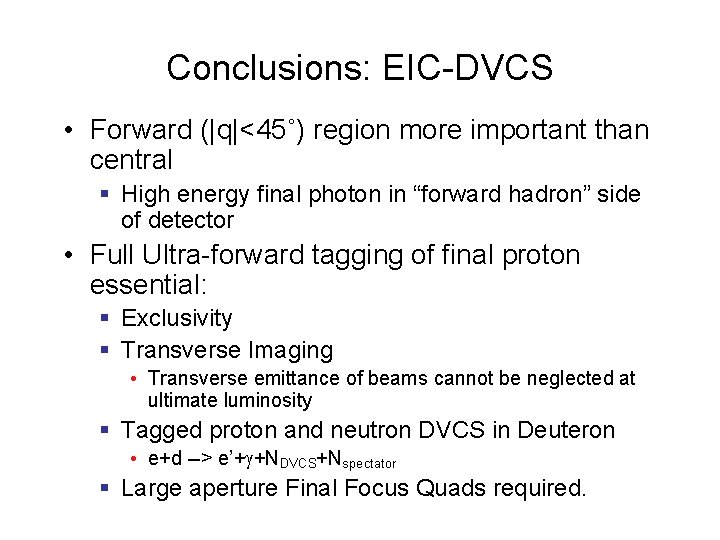
Conclusions: EIC-DVCS • Forward (|q|<45˚) region more important than central § High energy final photon in “forward hadron” side of detector • Full Ultra-forward tagging of final proton essential: § Exclusivity § Transverse Imaging • Transverse emittance of beams cannot be neglected at ultimate luminosity § Tagged proton and neutron DVCS in Deuteron • e+d --> e’+ +NDVCS+Nspectator § Large aperture Final Focus Quads required.
Student accounts hampton university
Eic approach of fundamental analysis
Fiu username
Qa eic patel the
Eic software reviews
Kickoff meting
Yellow report eic
Export inspection agency health certificate
Eic european investment centre
Diagnostico diferencial tvp
Hampton hump sign คือ
Hnncsb jobs
Ssai hampton va
Battle of hampton roads map
Segno della gobba di hampton
Enema positioning sims
Hampton hump sign คือ
Duron shearn
Aesq
Hampton pre prep school
Gobba di hampton rx