Processing of ceramics Processing of ceramics Sintering or
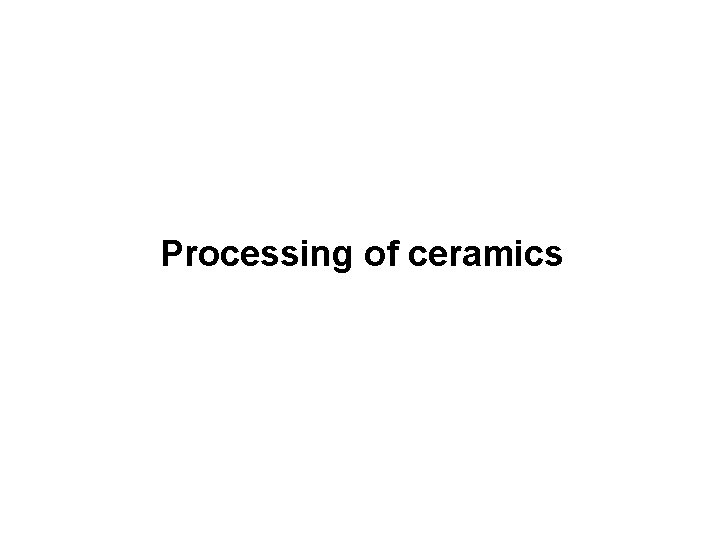
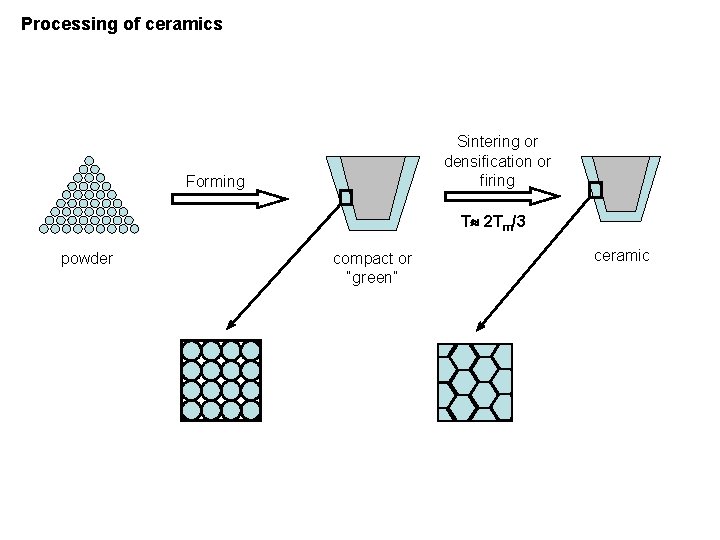
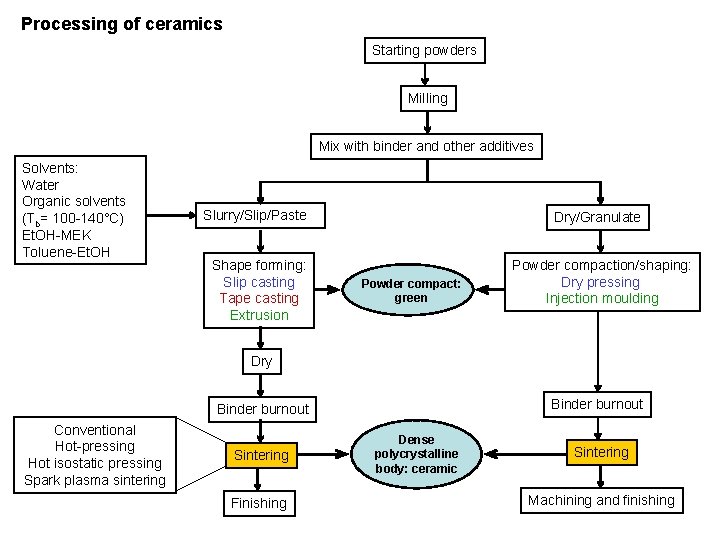
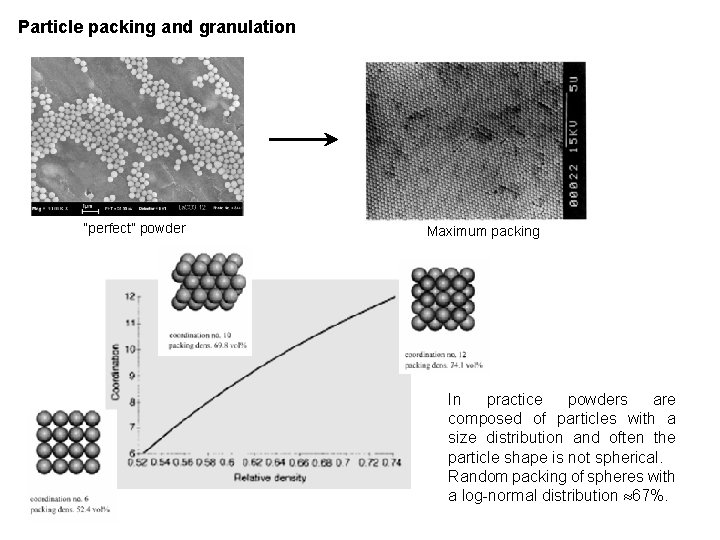
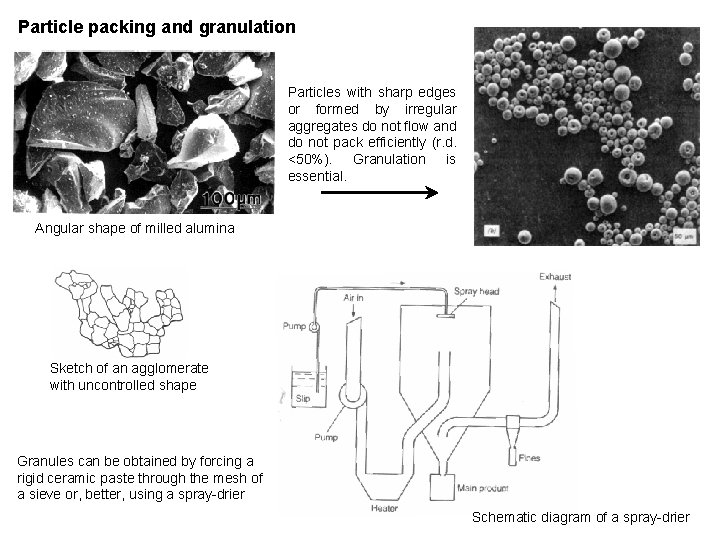
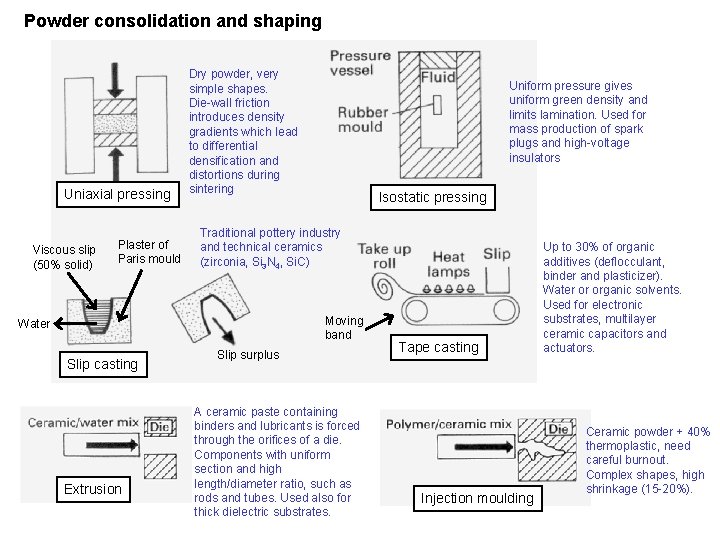
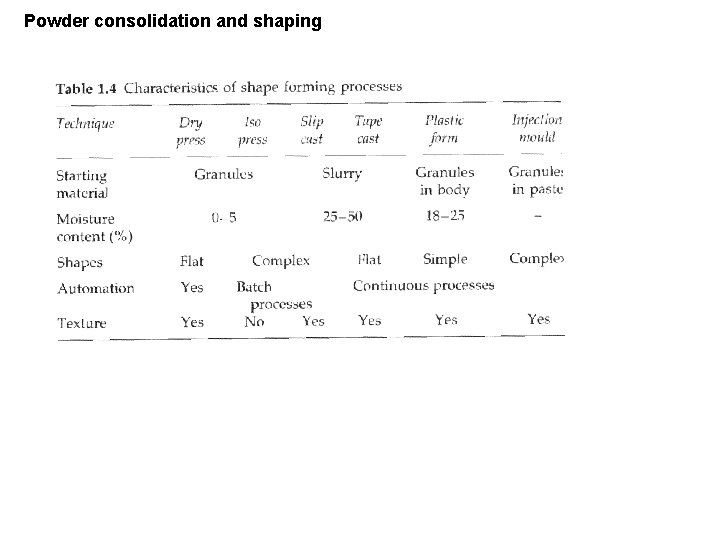
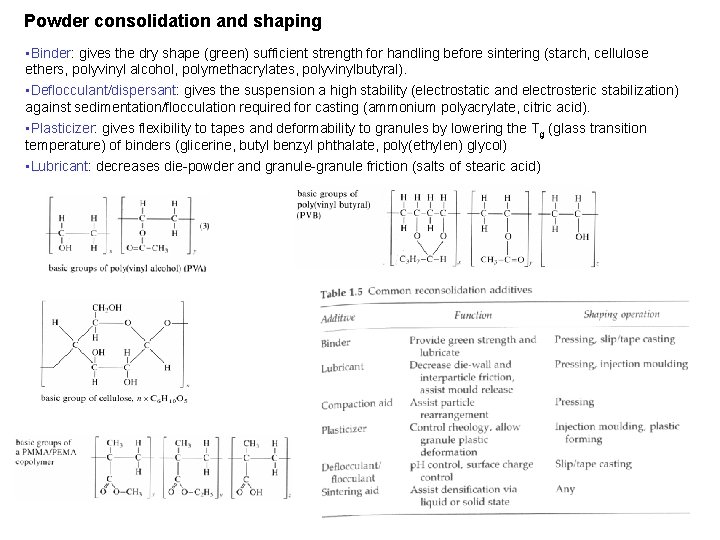
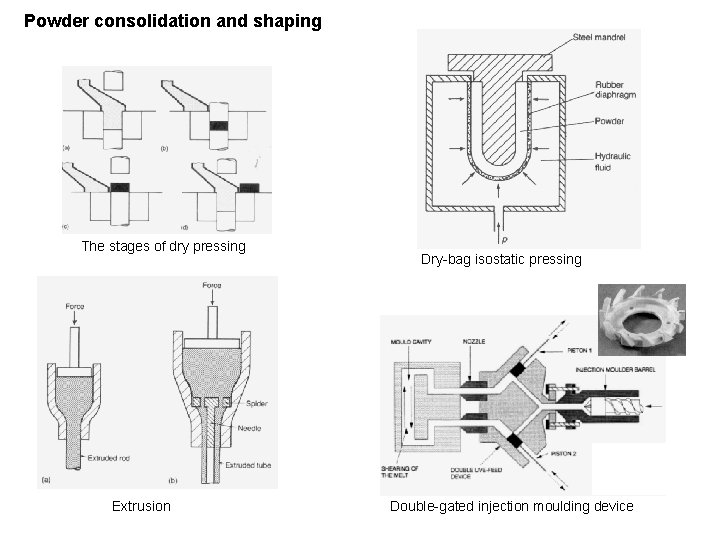
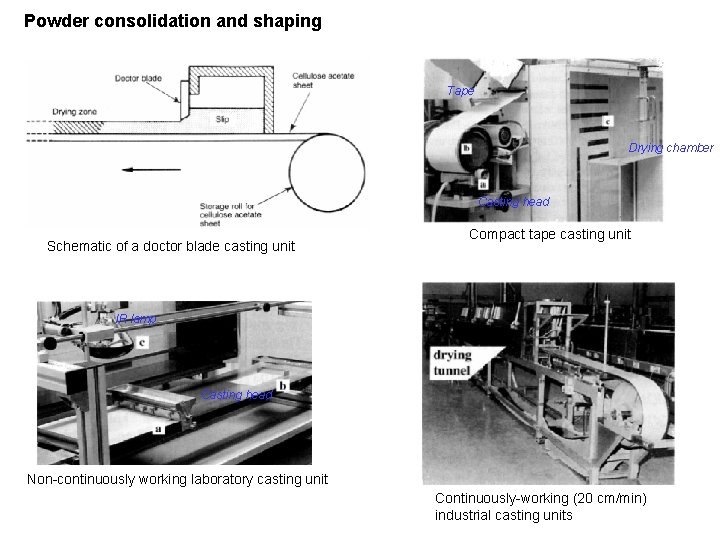
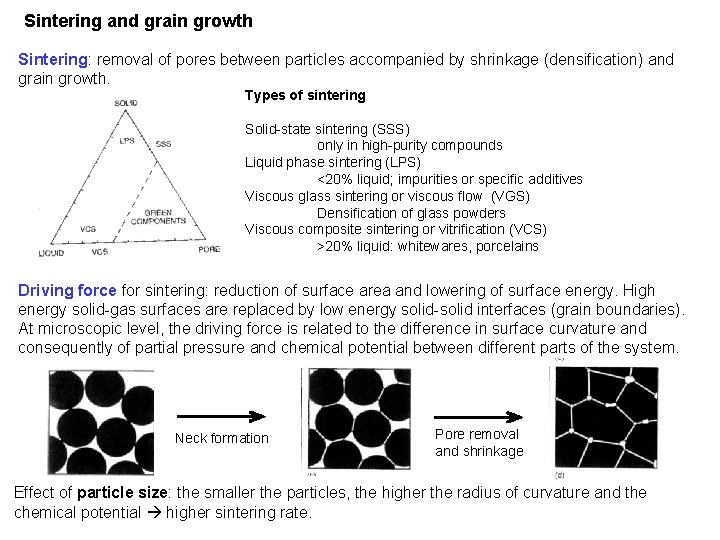
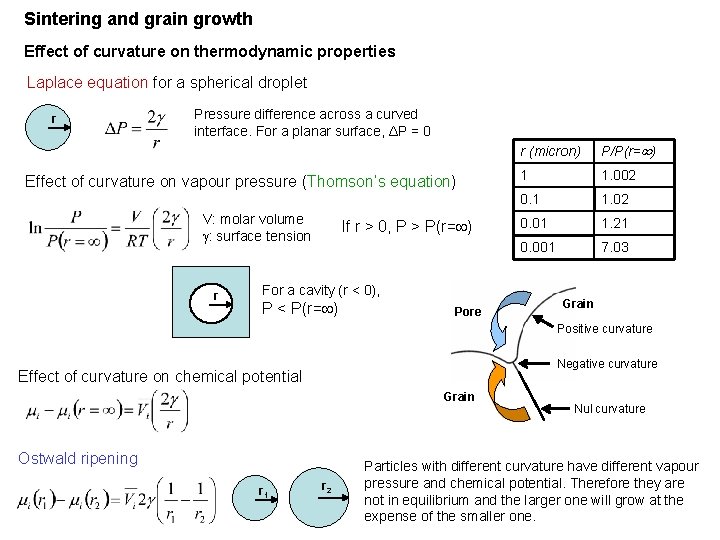
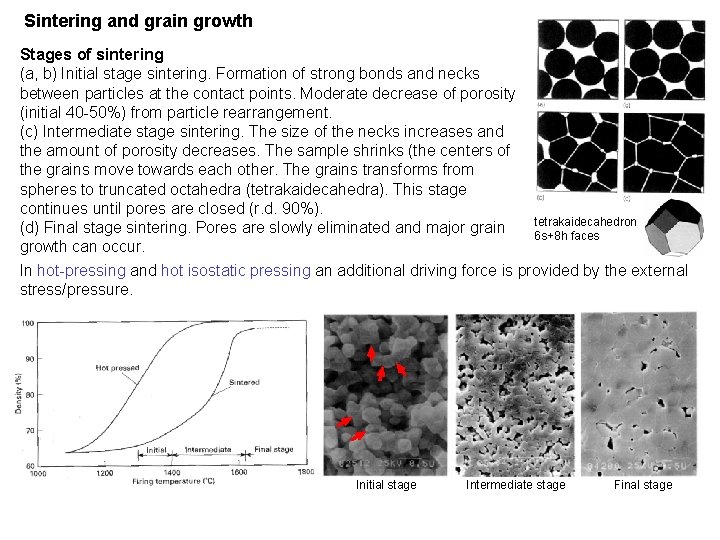
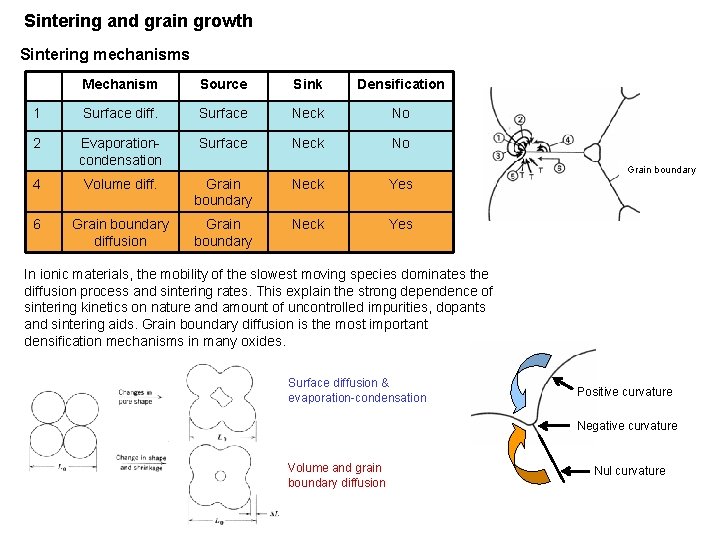
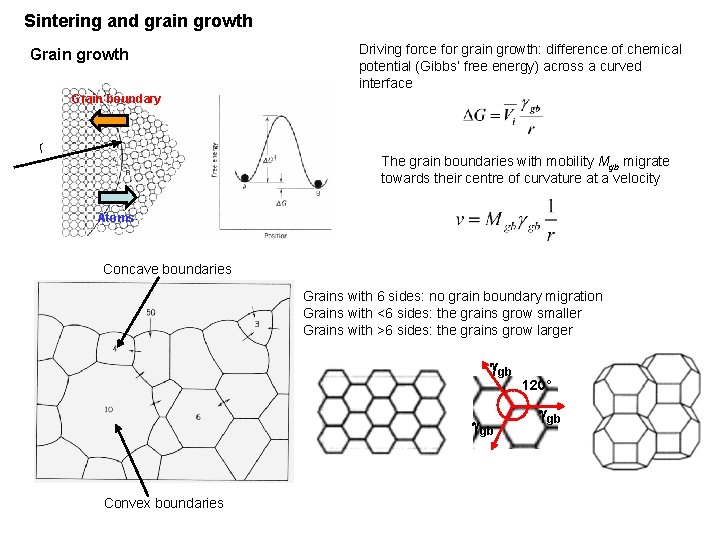
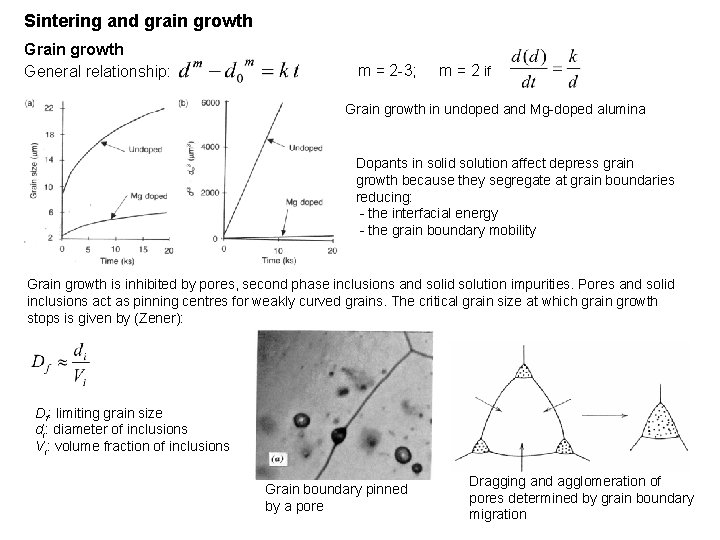
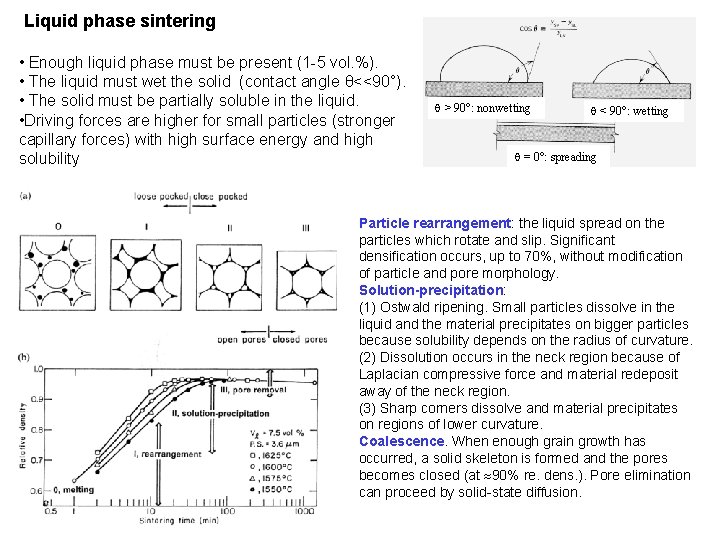
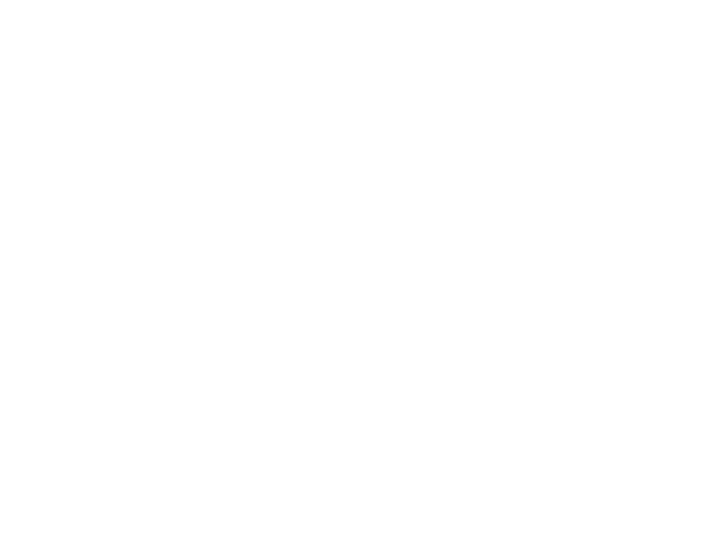
- Slides: 18
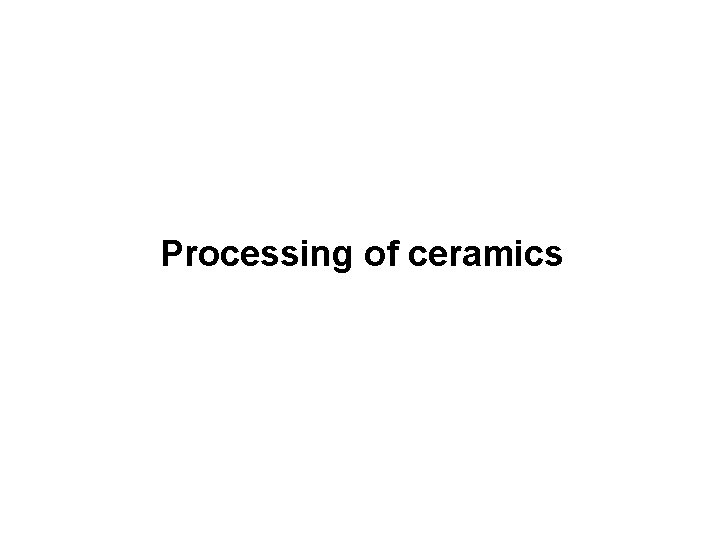
Processing of ceramics
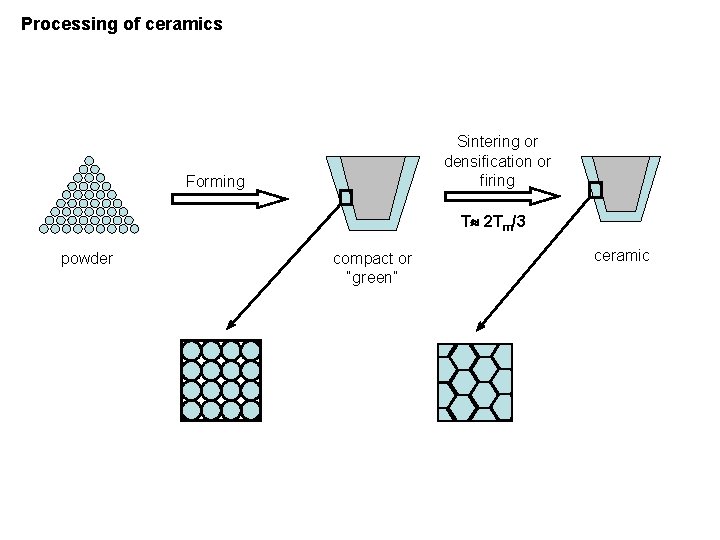
Processing of ceramics Sintering or densification or firing Forming T 2 Tm/3 powder compact or “green” ceramic
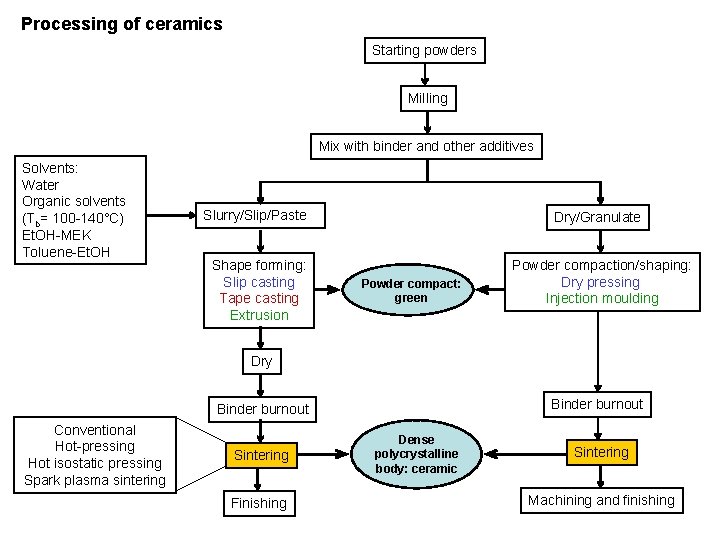
Processing of ceramics Starting powders Milling Mix with binder and other additives Solvents: Water Organic solvents (Tb= 100 -140°C) Et. OH-MEK Toluene-Et. OH Slurry/Slip/Paste Shape forming: Slip casting Tape casting Extrusion Dry/Granulate Powder compact: green Powder compaction/shaping: Dry pressing Injection moulding Dry Binder burnout Conventional Hot-pressing Hot isostatic pressing Spark plasma sintering Sintering Finishing Dense polycrystalline body: ceramic Sintering Machining and finishing
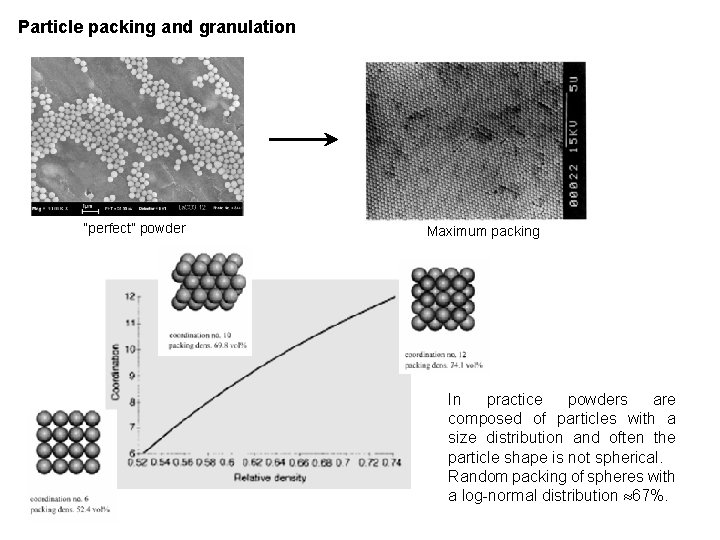
Particle packing and granulation “perfect” powder Maximum packing In practice powders are composed of particles with a size distribution and often the particle shape is not spherical. Random packing of spheres with a log-normal distribution 67%.
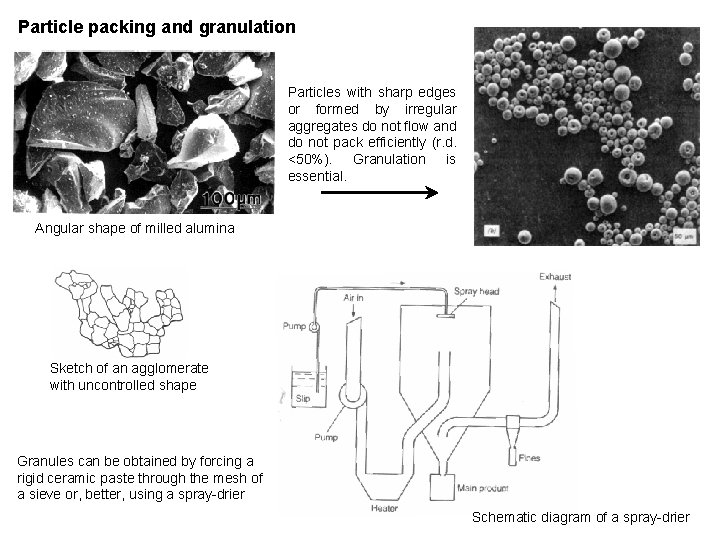
Particle packing and granulation Particles with sharp edges or formed by irregular aggregates do not flow and do not pack efficiently (r. d. <50%). Granulation is essential. Angular shape of milled alumina Sketch of an agglomerate with uncontrolled shape Granules can be obtained by forcing a rigid ceramic paste through the mesh of a sieve or, better, using a spray-drier Schematic diagram of a spray-drier
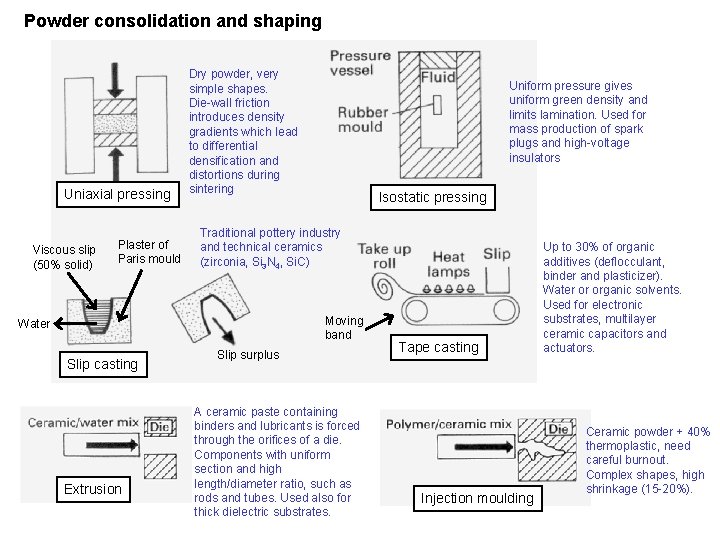
Powder consolidation and shaping Uniaxial pressing Viscous slip (50% solid) Plaster of Paris mould Dry powder, very simple shapes. Die-wall friction introduces density gradients which lead to differential densification and distortions during sintering Uniform pressure gives uniform green density and limits lamination. Used for mass production of spark plugs and high-voltage insulators Isostatic pressing Traditional pottery industry and technical ceramics (zirconia, Si 3 N 4, Si. C) Moving band Water Slip casting Extrusion Slip surplus A ceramic paste containing binders and lubricants is forced through the orifices of a die. Components with uniform section and high length/diameter ratio, such as rods and tubes. Used also for thick dielectric substrates. Tape casting Injection moulding Up to 30% of organic additives (deflocculant, binder and plasticizer). Water or organic solvents. Used for electronic substrates, multilayer ceramic capacitors and actuators. Ceramic powder + 40% thermoplastic, need careful burnout. Complex shapes, high shrinkage (15 -20%).
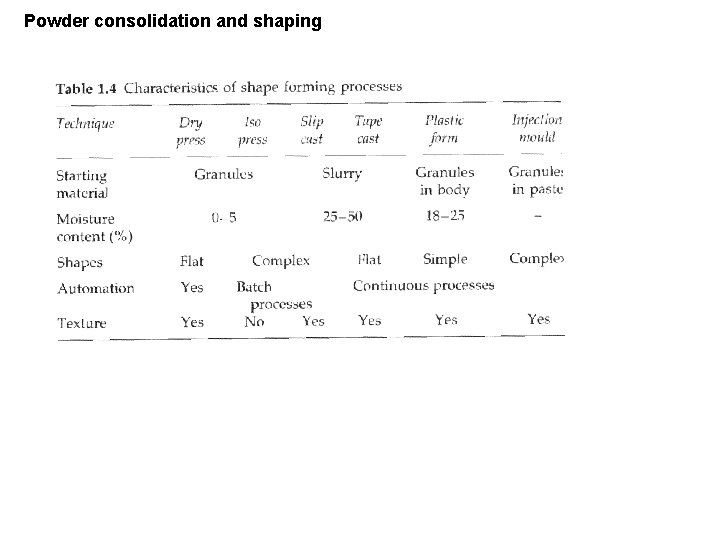
Powder consolidation and shaping
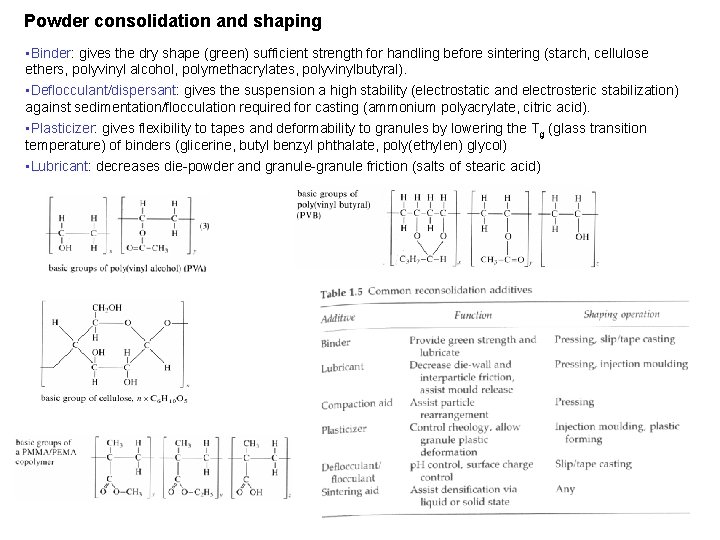
Powder consolidation and shaping • Binder: gives the dry shape (green) sufficient strength for handling before sintering (starch, cellulose ethers, polyvinyl alcohol, polymethacrylates, polyvinylbutyral). • Deflocculant/dispersant: gives the suspension a high stability (electrostatic and electrosteric stabilization) against sedimentation/flocculation required for casting (ammonium polyacrylate, citric acid). • Plasticizer: gives flexibility to tapes and deformability to granules by lowering the Tg (glass transition temperature) of binders (glicerine, butyl benzyl phthalate, poly(ethylen) glycol) • Lubricant: decreases die-powder and granule-granule friction (salts of stearic acid)
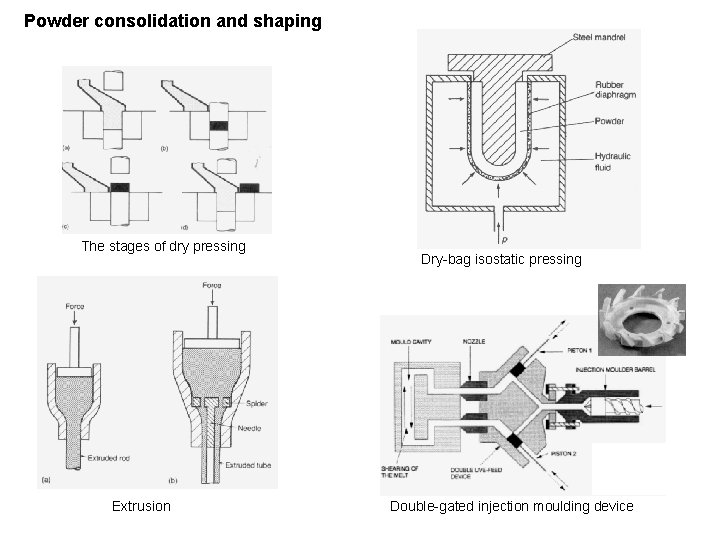
Powder consolidation and shaping The stages of dry pressing Extrusion Dry-bag isostatic pressing Double-gated injection moulding device
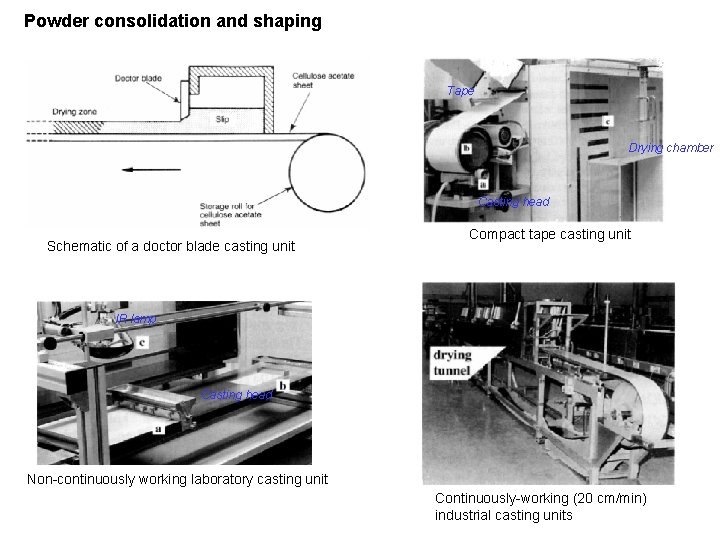
Powder consolidation and shaping Tape Drying chamber Casting head Schematic of a doctor blade casting unit Compact tape casting unit IR lamp Casting head Non-continuously working laboratory casting unit Continuously-working (20 cm/min) industrial casting units
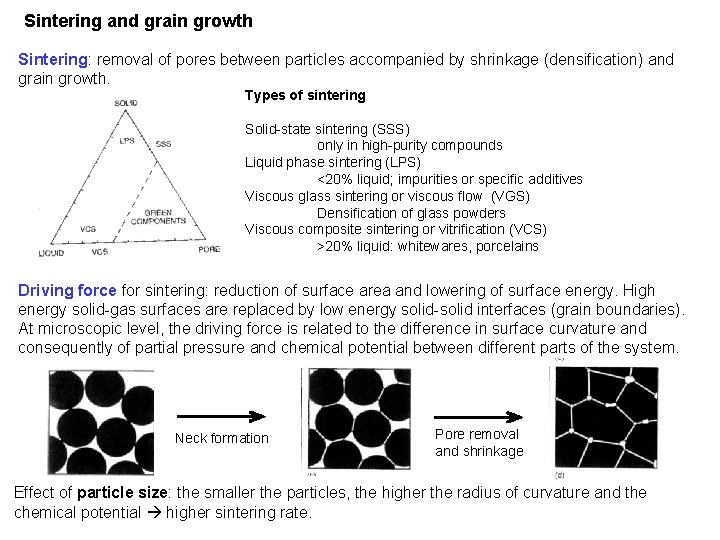
Sintering and grain growth Sintering: removal of pores between particles accompanied by shrinkage (densification) and grain growth. Types of sintering Solid-state sintering (SSS) only in high-purity compounds Liquid phase sintering (LPS) <20% liquid; impurities or specific additives Viscous glass sintering or viscous flow (VGS) Densification of glass powders Viscous composite sintering or vitrification (VCS) >20% liquid: whitewares, porcelains Driving force for sintering: reduction of surface area and lowering of surface energy. High energy solid-gas surfaces are replaced by low energy solid-solid interfaces (grain boundaries). At microscopic level, the driving force is related to the difference in surface curvature and consequently of partial pressure and chemical potential between different parts of the system. Neck formation Pore removal and shrinkage Effect of particle size: the smaller the particles, the higher the radius of curvature and the chemical potential higher sintering rate.
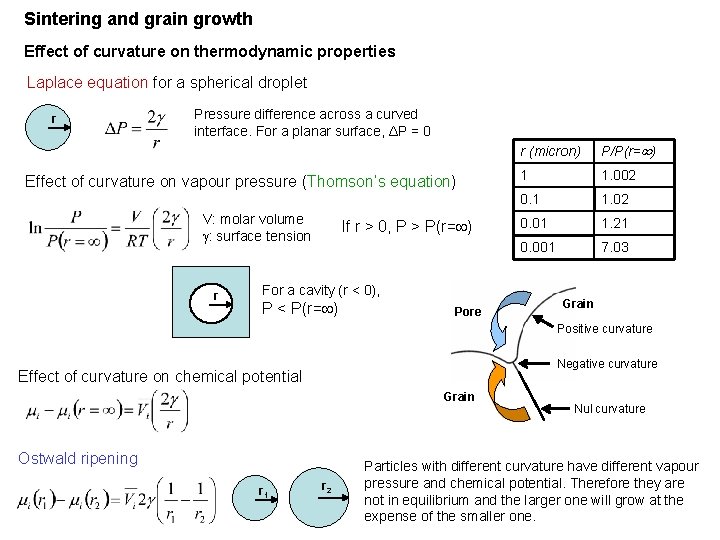
Sintering and grain growth Effect of curvature on thermodynamic properties Laplace equation for a spherical droplet r Pressure difference across a curved interface. For a planar surface, ΔP = 0 Effect of curvature on vapour pressure (Thomson’s equation) V: molar volume : surface tension r If r > 0, P > P(r= ) For a cavity (r < 0), P < P(r= ) Pore r (micron) P/P(r= ) 1 1. 002 0. 1 1. 02 0. 01 1. 21 0. 001 7. 03 Grain Positive curvature Negative curvature Effect of curvature on chemical potential Grain Ostwald ripening r 1 r 2 Nul curvature Particles with different curvature have different vapour pressure and chemical potential. Therefore they are not in equilibrium and the larger one will grow at the expense of the smaller one.
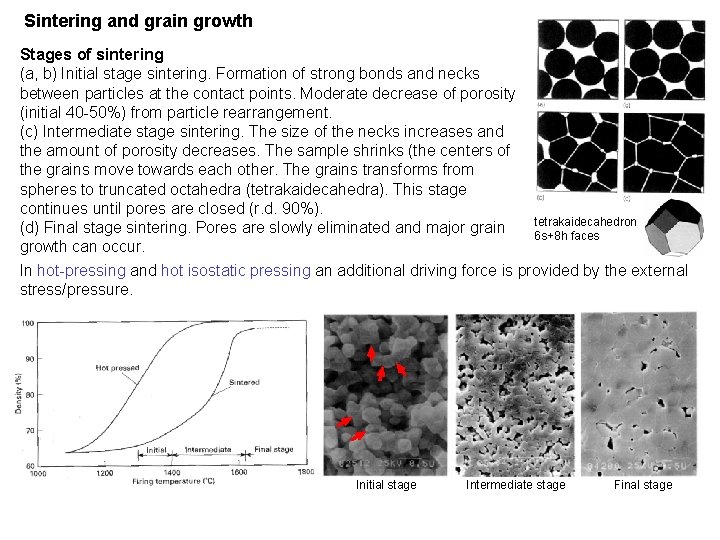
Sintering and grain growth Stages of sintering (a, b) Initial stage sintering. Formation of strong bonds and necks between particles at the contact points. Moderate decrease of porosity (initial 40 -50%) from particle rearrangement. (c) Intermediate stage sintering. The size of the necks increases and the amount of porosity decreases. The sample shrinks (the centers of the grains move towards each other. The grains transforms from spheres to truncated octahedra (tetrakaidecahedra). This stage continues until pores are closed (r. d. 90%). tetrakaidecahedron (d) Final stage sintering. Pores are slowly eliminated and major grain 6 s+8 h faces growth can occur. In hot-pressing and hot isostatic pressing an additional driving force is provided by the external stress/pressure. Initial stage Intermediate stage Final stage
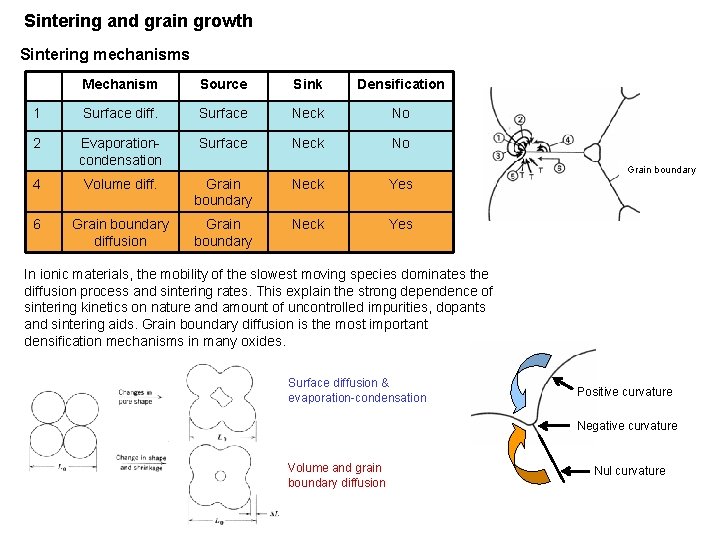
Sintering and grain growth Sintering mechanisms Mechanism Source Sink Densification 1 Surface diff. Surface Neck No 2 Evaporationcondensation Surface Neck No 4 Volume diff. Grain boundary Neck Yes 6 Grain boundary diffusion Grain boundary Neck Yes Grain boundary In ionic materials, the mobility of the slowest moving species dominates the diffusion process and sintering rates. This explain the strong dependence of sintering kinetics on nature and amount of uncontrolled impurities, dopants and sintering aids. Grain boundary diffusion is the most important densification mechanisms in many oxides. Surface diffusion & evaporation-condensation Positive curvature Negative curvature Volume and grain boundary diffusion Nul curvature
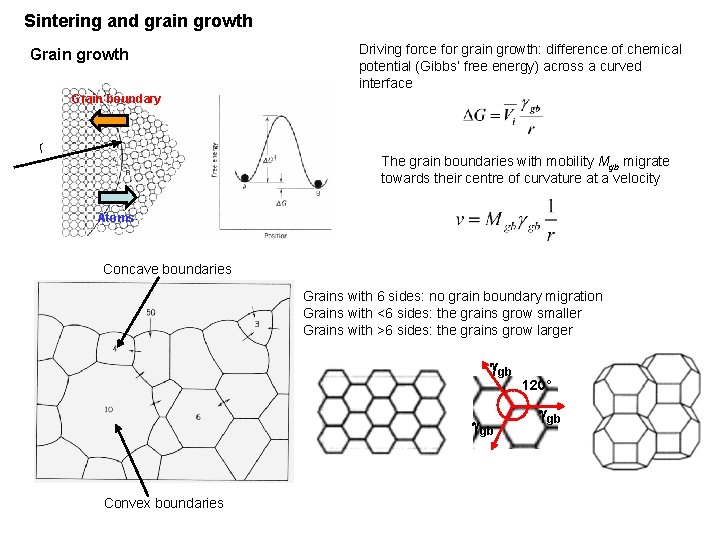
Sintering and grain growth Grain growth Driving force for grain growth: difference of chemical potential (Gibbs’ free energy) across a curved interface Grain boundary r The grain boundaries with mobility Mgb migrate towards their centre of curvature at a velocity Atoms Concave boundaries Grains with 6 sides: no grain boundary migration Grains with <6 sides: the grains grow smaller Grains with >6 sides: the grains grow larger gb gb Convex boundaries 120° gb
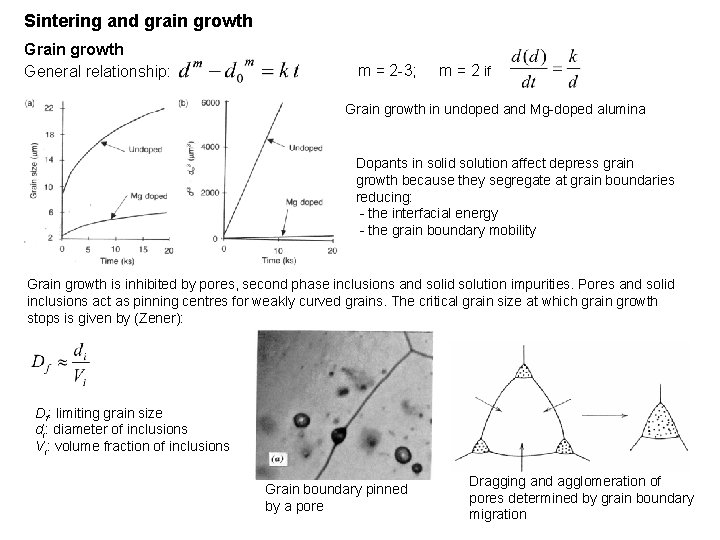
Sintering and grain growth General relationship: m = 2 -3; m = 2 if Grain growth in undoped and Mg-doped alumina Dopants in solid solution affect depress grain growth because they segregate at grain boundaries reducing: - the interfacial energy - the grain boundary mobility Grain growth is inhibited by pores, second phase inclusions and solid solution impurities. Pores and solid inclusions act as pinning centres for weakly curved grains. The critical grain size at which grain growth stops is given by (Zener): Df: limiting grain size di: diameter of inclusions Vi: volume fraction of inclusions Grain boundary pinned by a pore Dragging and agglomeration of pores determined by grain boundary migration
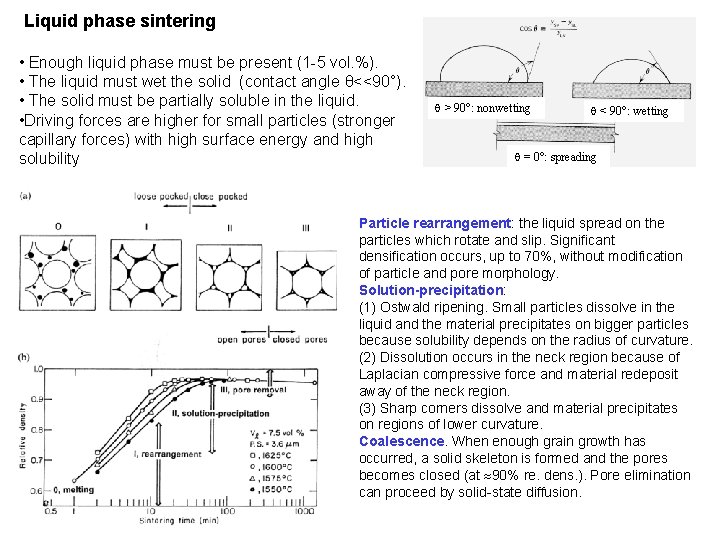
Liquid phase sintering • Enough liquid phase must be present (1 -5 vol. %). • The liquid must wet the solid (contact angle θ<<90°). • The solid must be partially soluble in the liquid. • Driving forces are higher for small particles (stronger capillary forces) with high surface energy and high solubility > 90°: nonwetting < 90°: wetting = 0°: spreading Particle rearrangement: the liquid spread on the particles which rotate and slip. Significant densification occurs, up to 70%, without modification of particle and pore morphology. Solution-precipitation: (1) Ostwald ripening. Small particles dissolve in the liquid and the material precipitates on bigger particles because solubility depends on the radius of curvature. (2) Dissolution occurs in the neck region because of Laplacian compressive force and material redeposit away of the neck region. (3) Sharp corners dissolve and material precipitates on regions of lower curvature. Coalescence. When enough grain growth has occurred, a solid skeleton is formed and the pores becomes closed (at 90% re. dens. ). Pore elimination can proceed by solid-state diffusion.
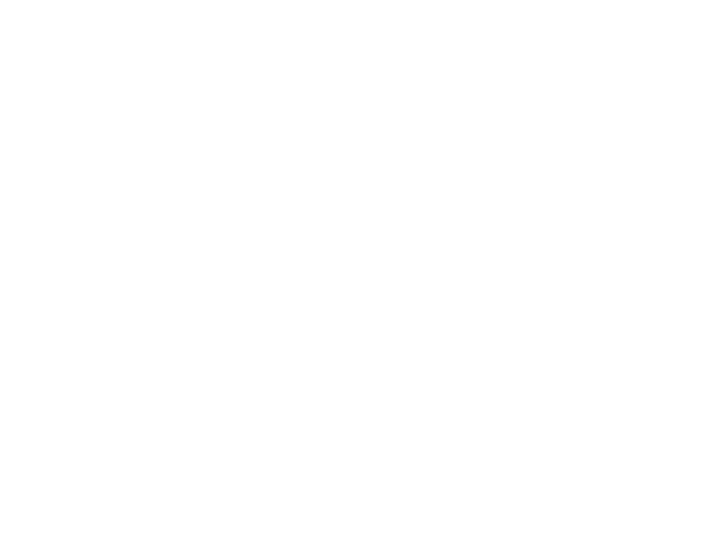
Spark plasma sintering ppt
History of selective laser sintering
Field assisted sintering technology
Gear manufacturing process
Types of sinter
Processing of ceramics
Blasch precision ceramics
Coiling clay definition
Hand building ceramics definition
Coil bowl construction
Hand building definition
Drymix plasters renders ceramics
Pinching ceramics definition
Functional ceramics application
Characteristics of pinch pot
Raku-nn
Ceramics 101
Pottery history timeline
Die blanks ceramics