Peter J Russell A molecular Approach 2 nd
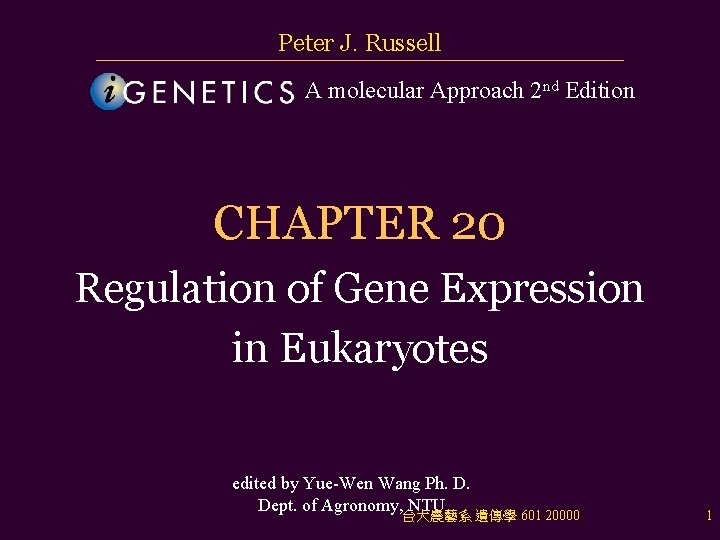
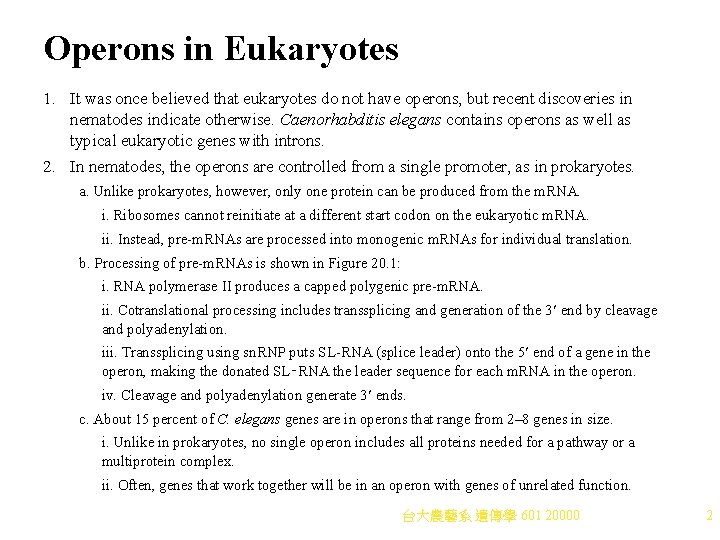
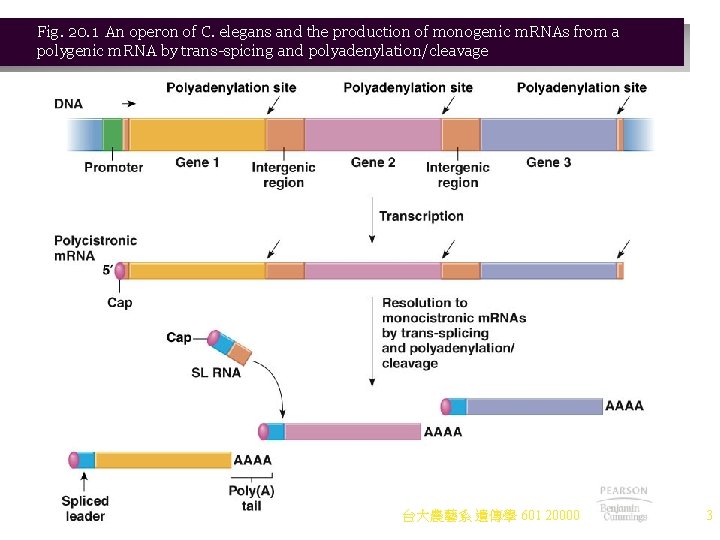
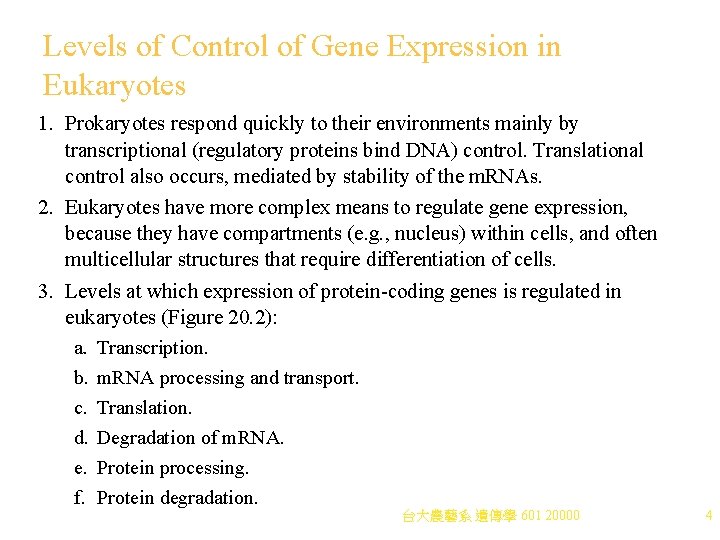
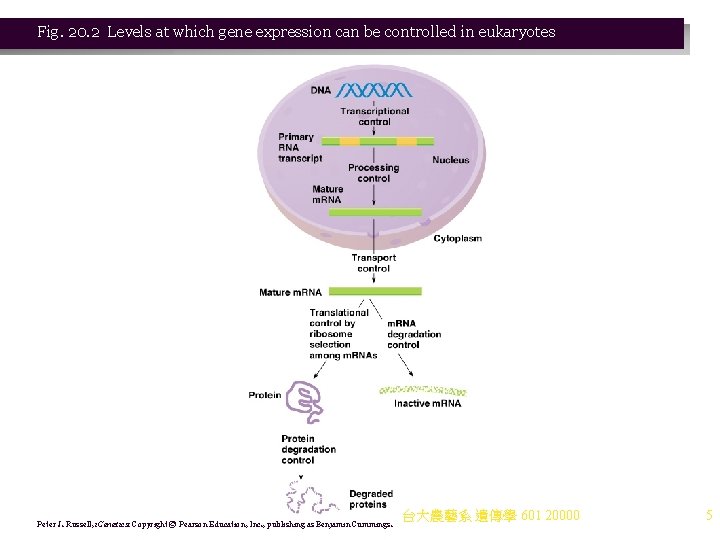
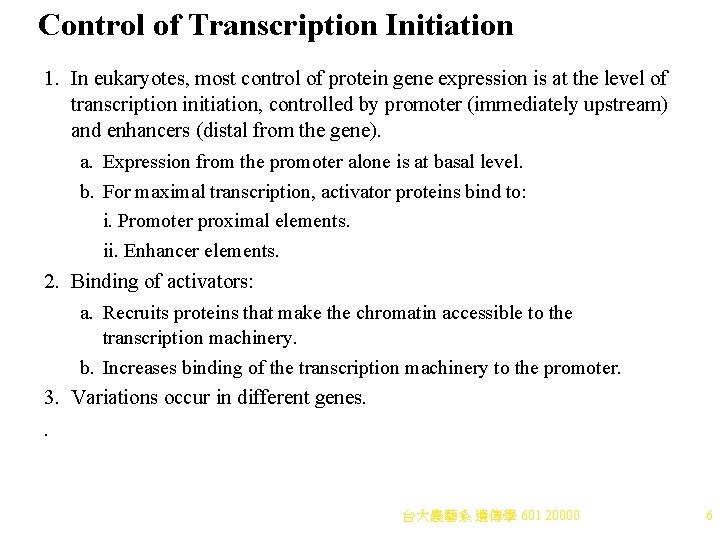
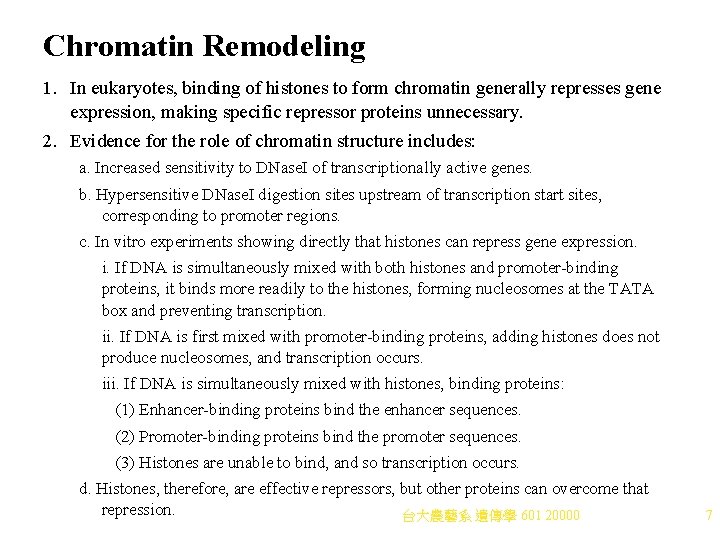
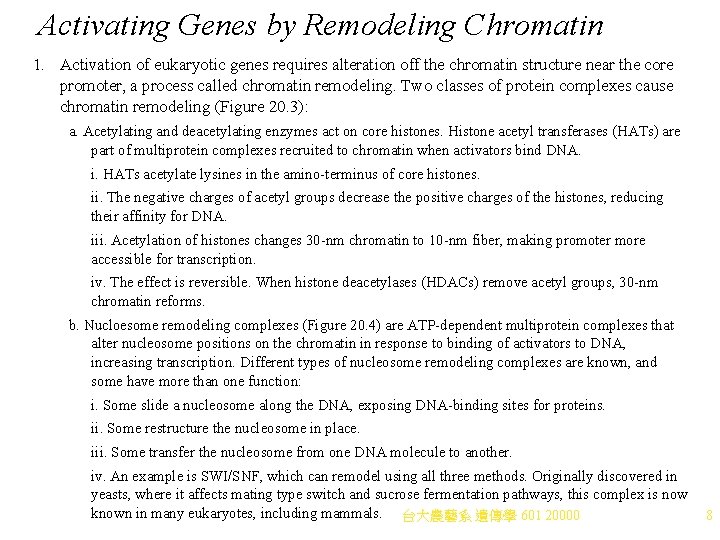
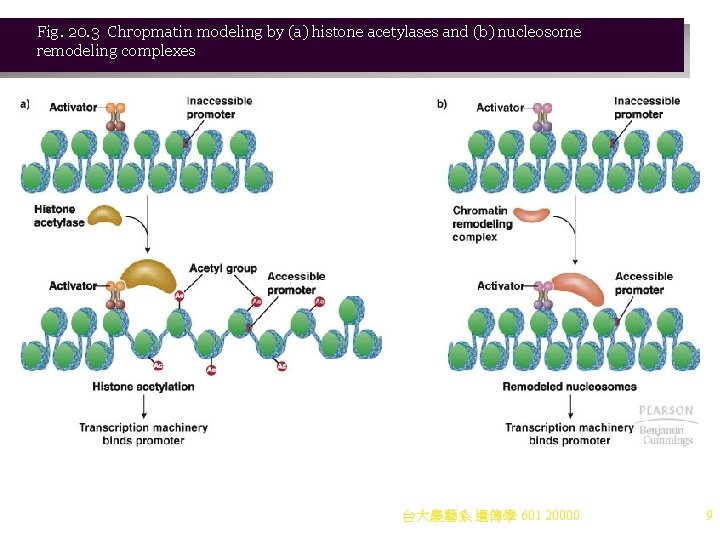
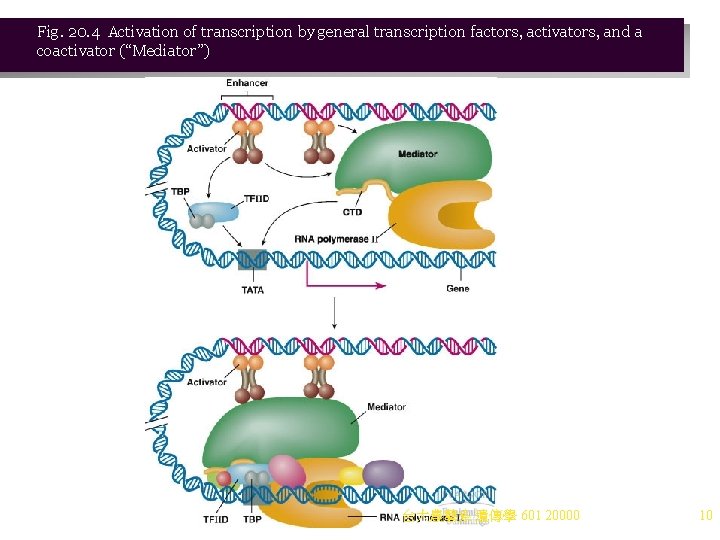
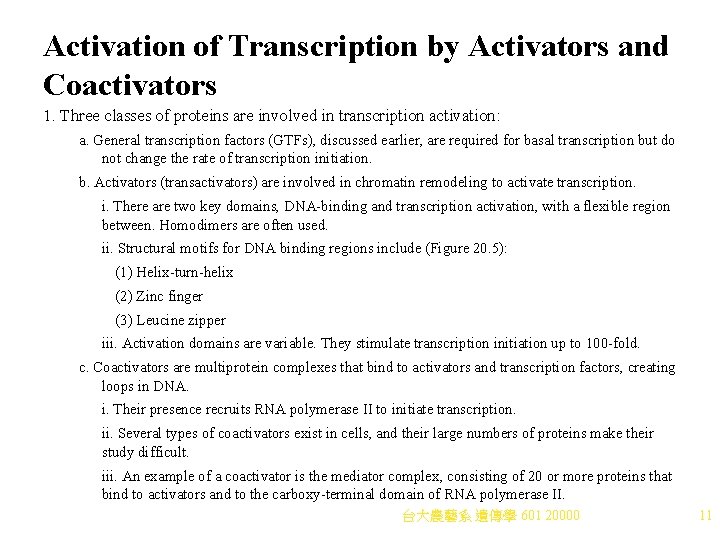
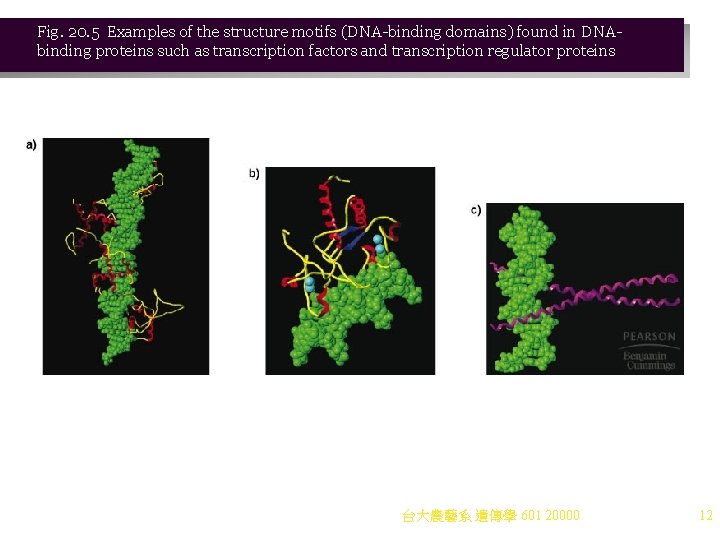
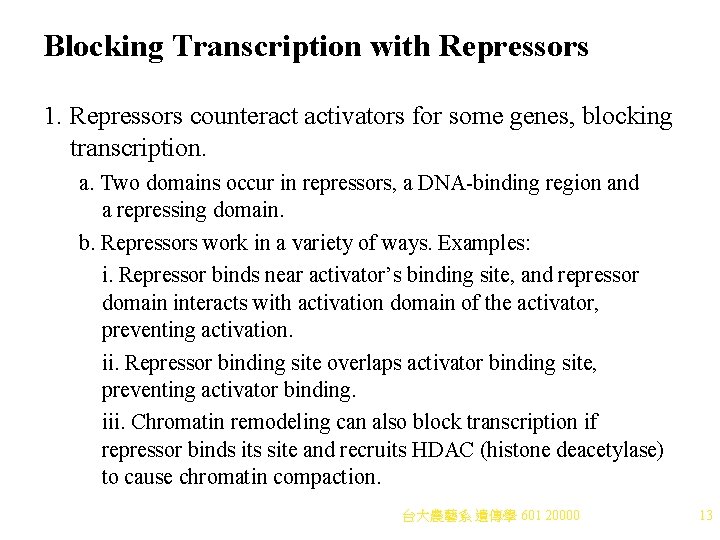
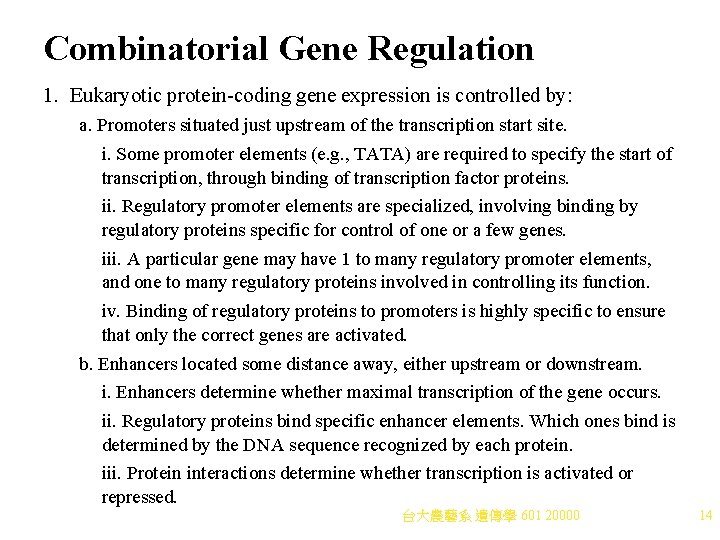
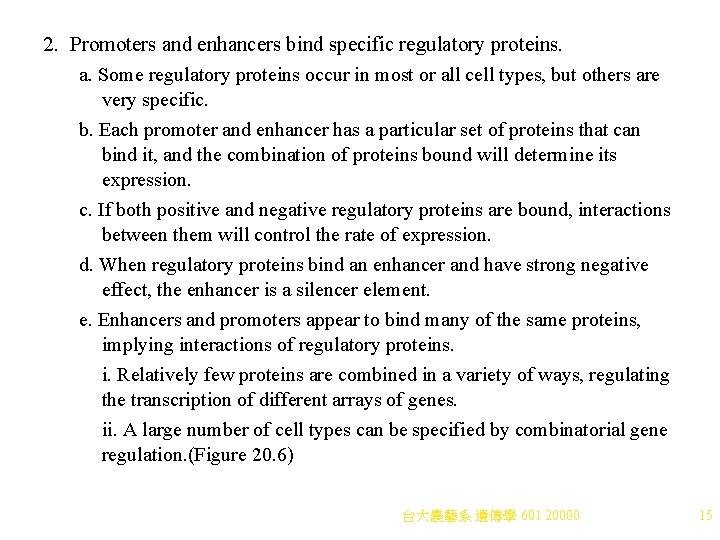
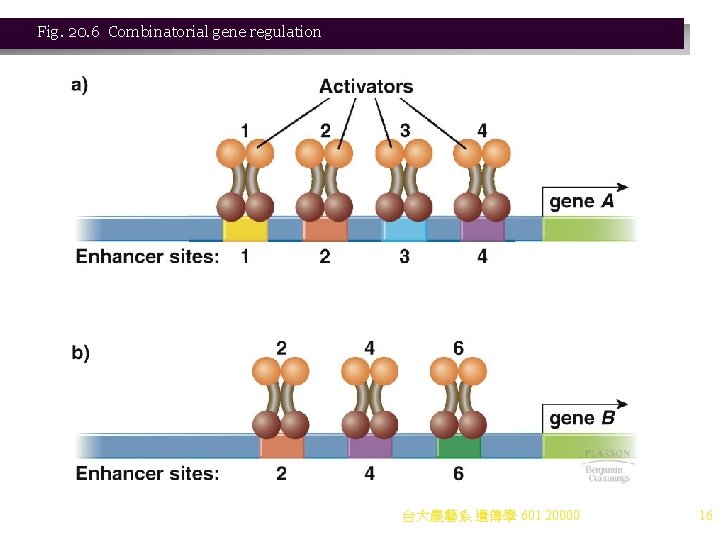
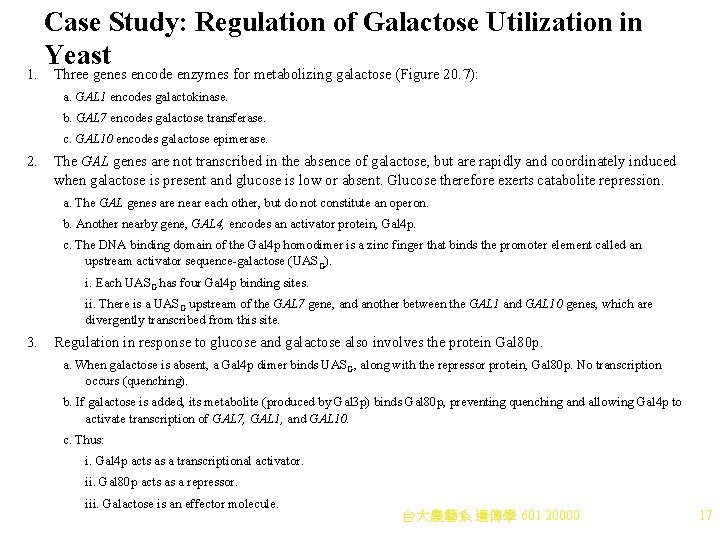
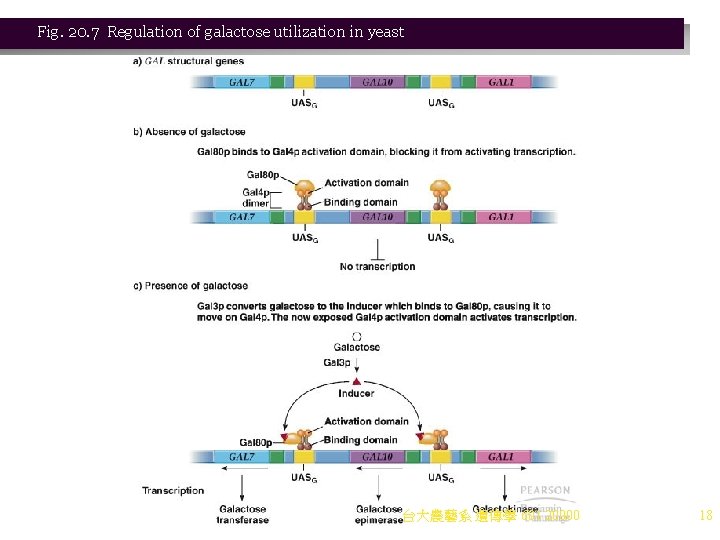
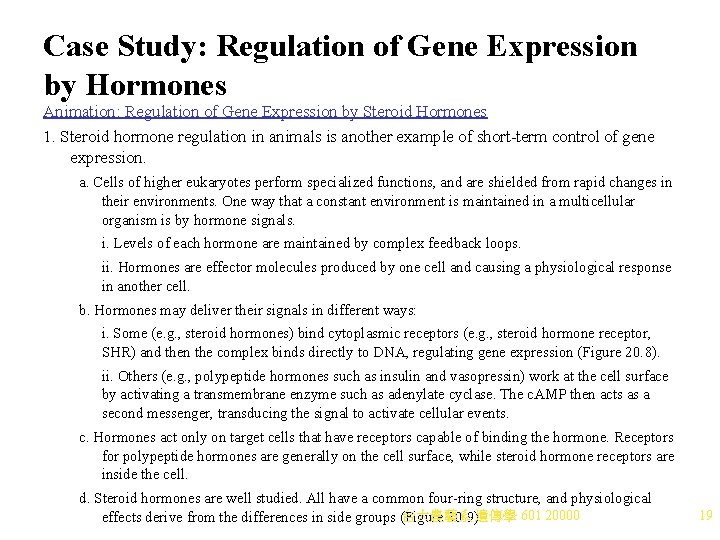
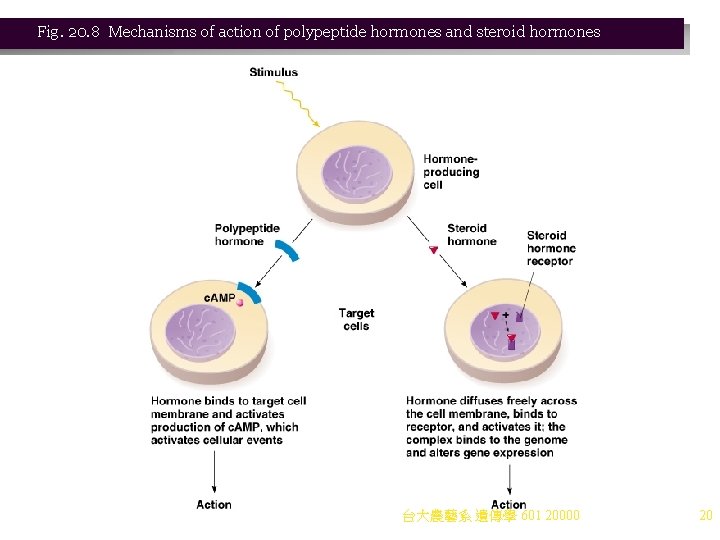
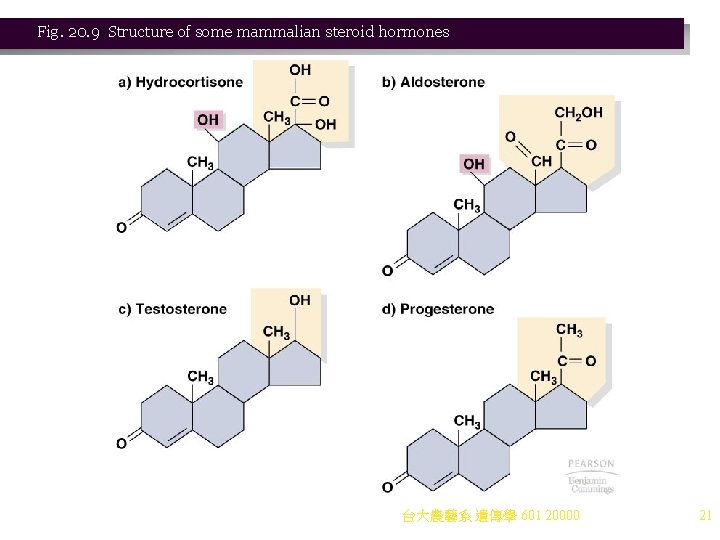
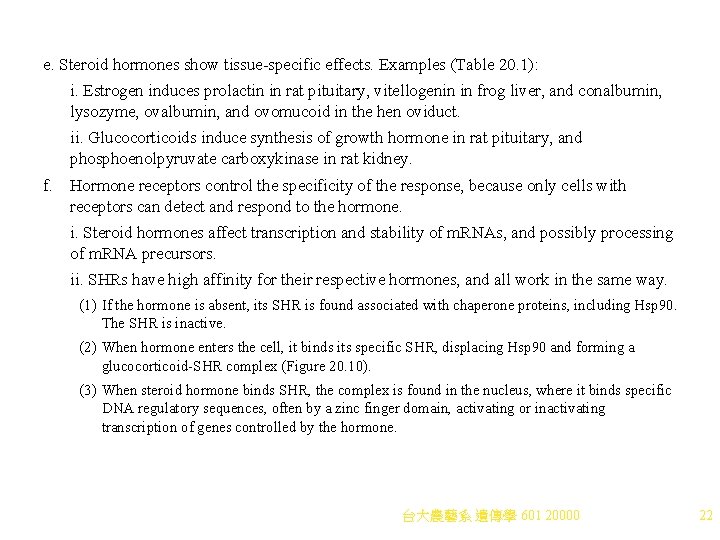
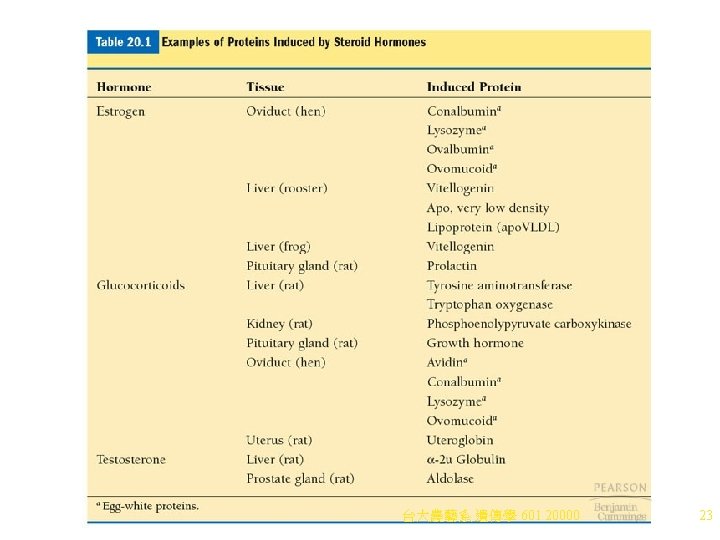
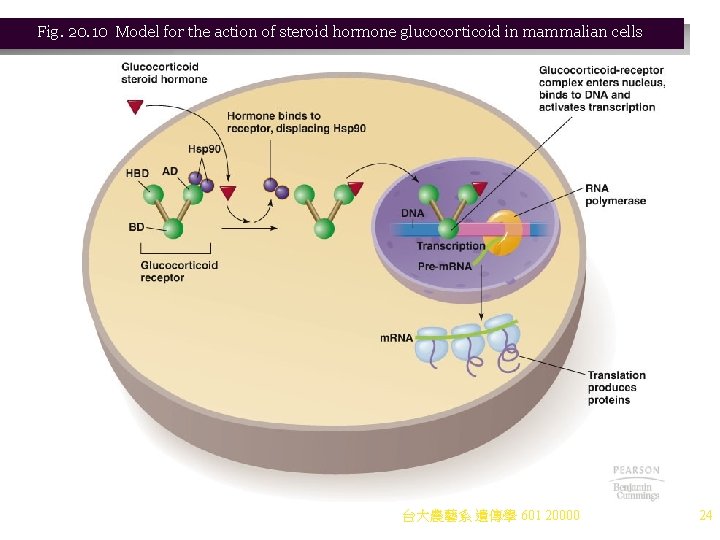
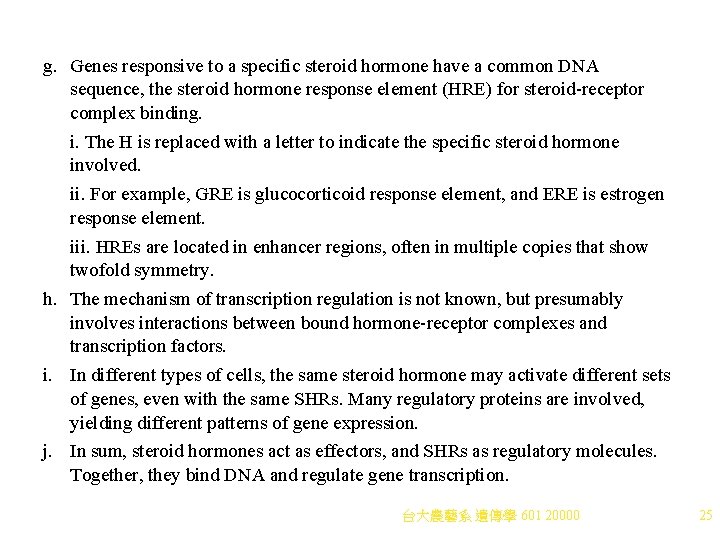
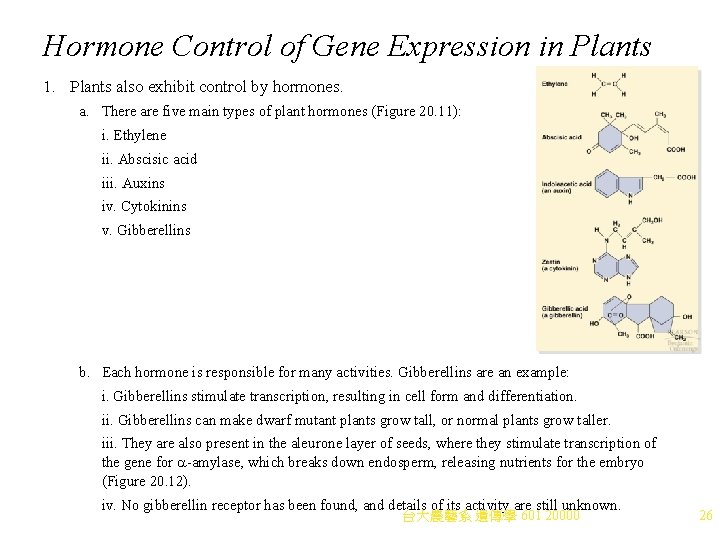
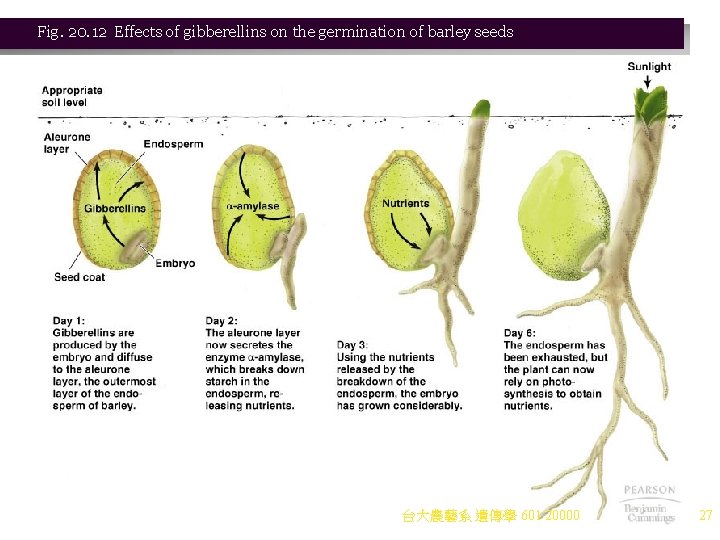
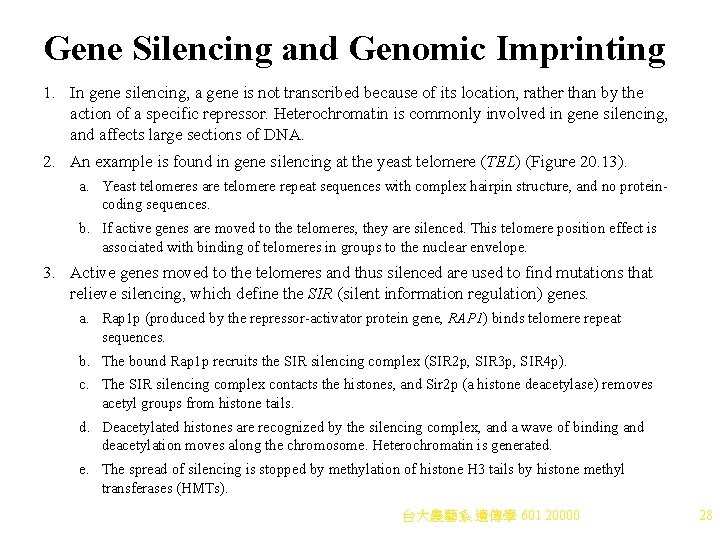
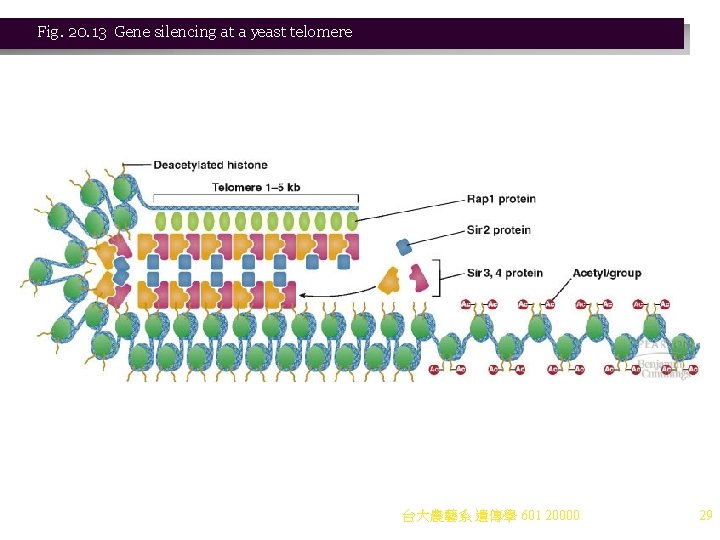
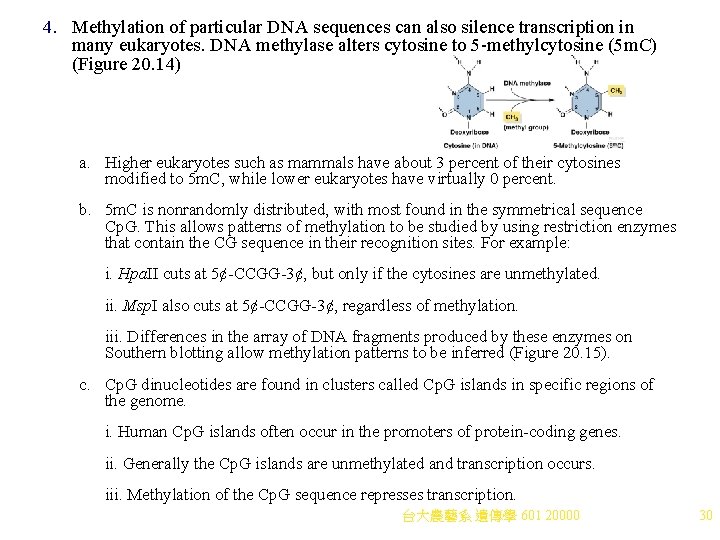
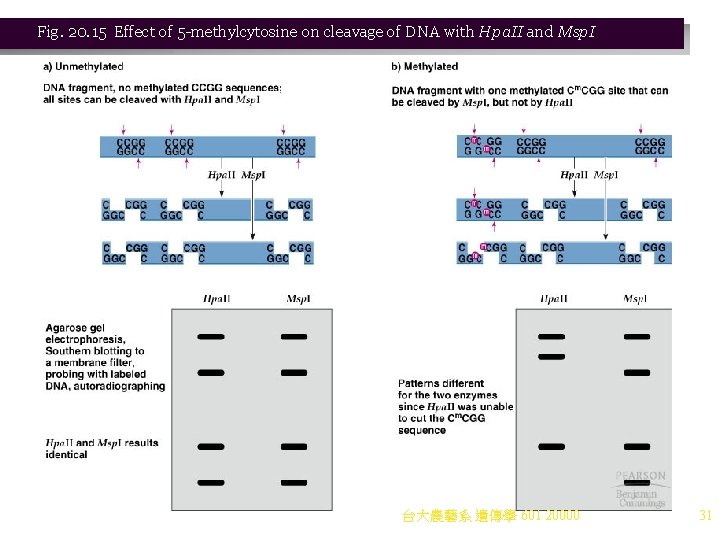
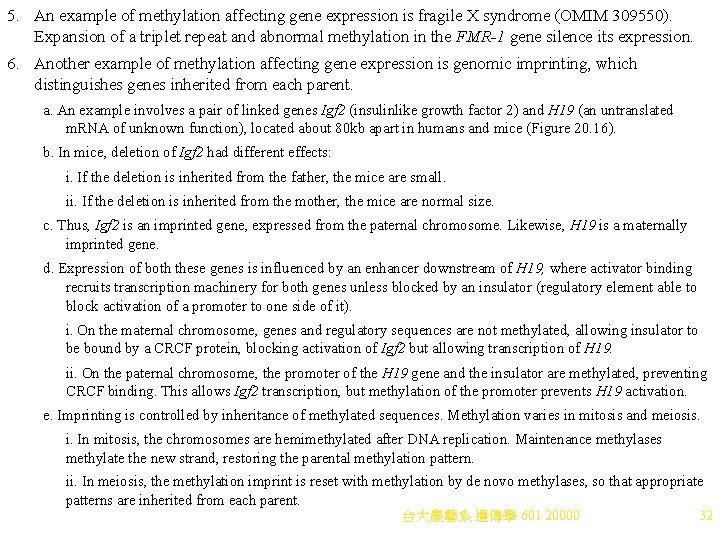
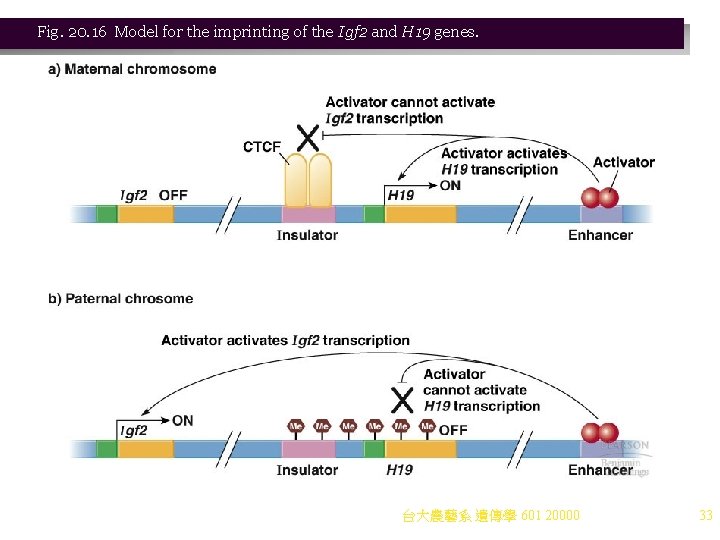
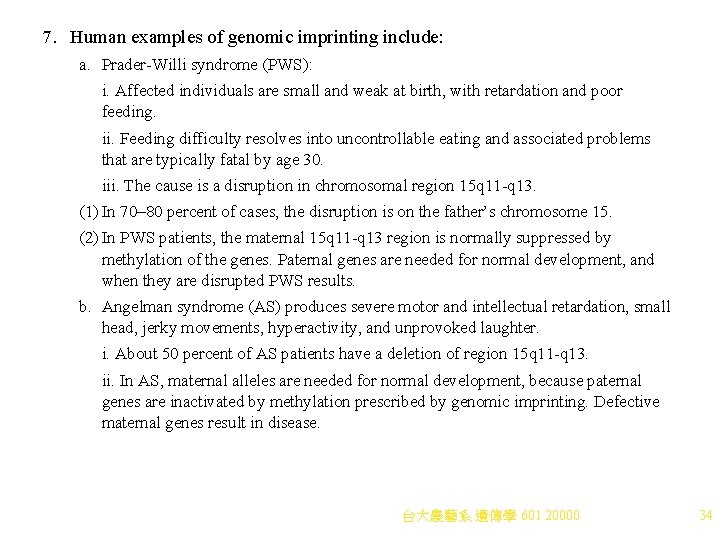
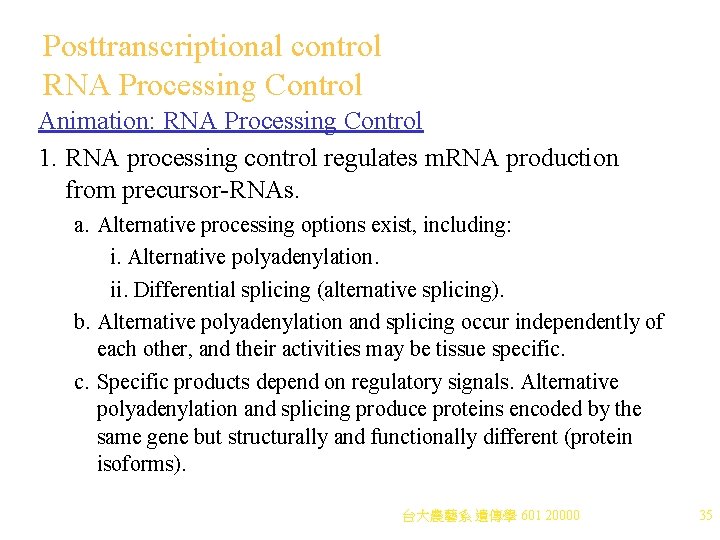
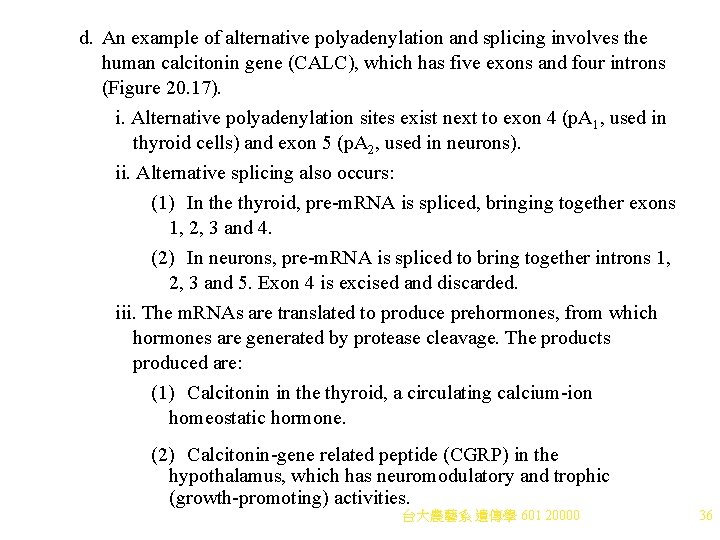
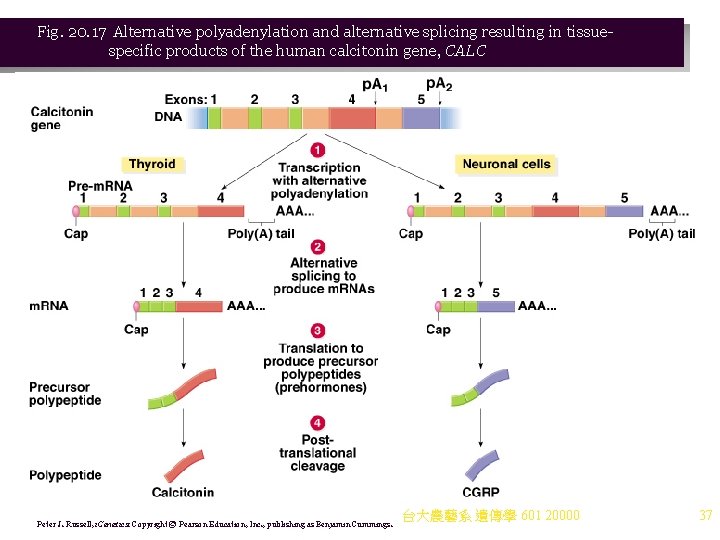
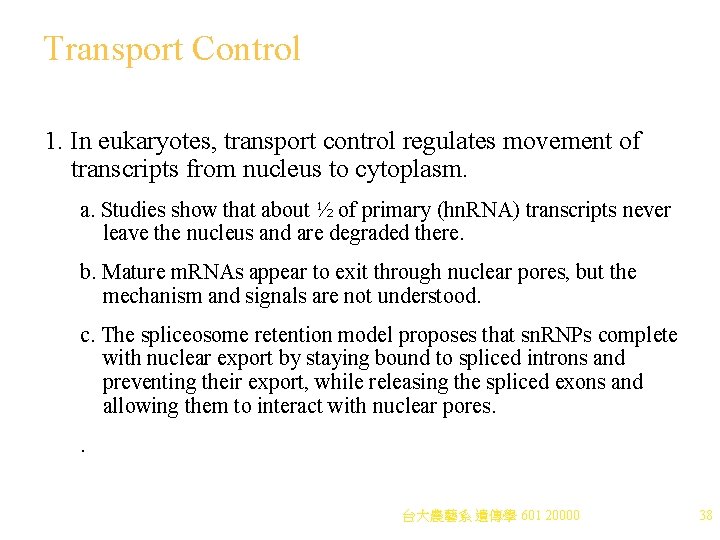
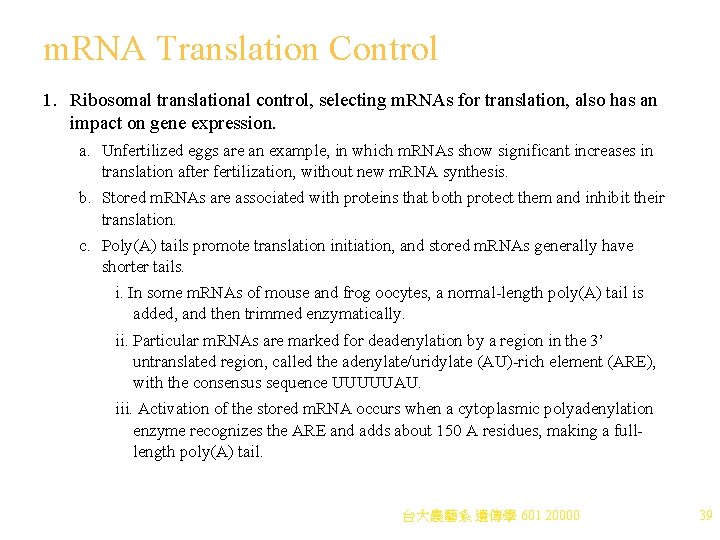
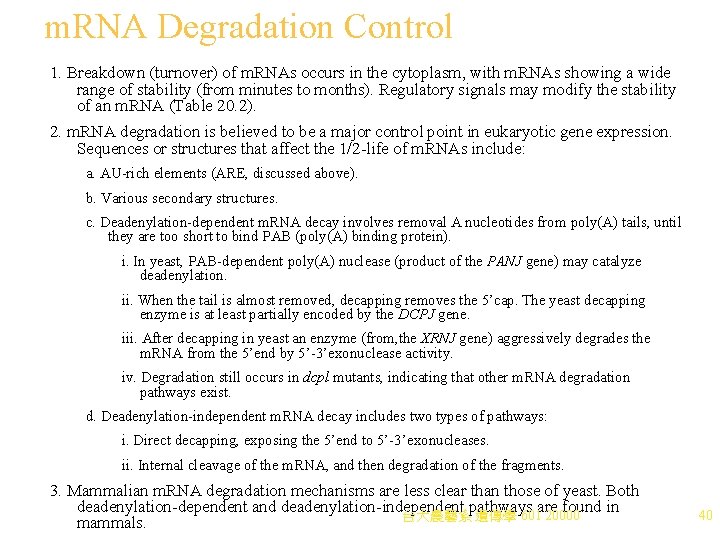
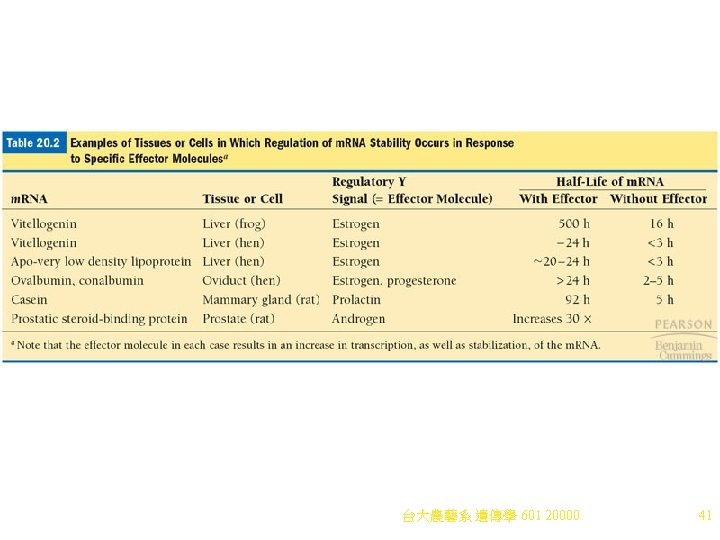
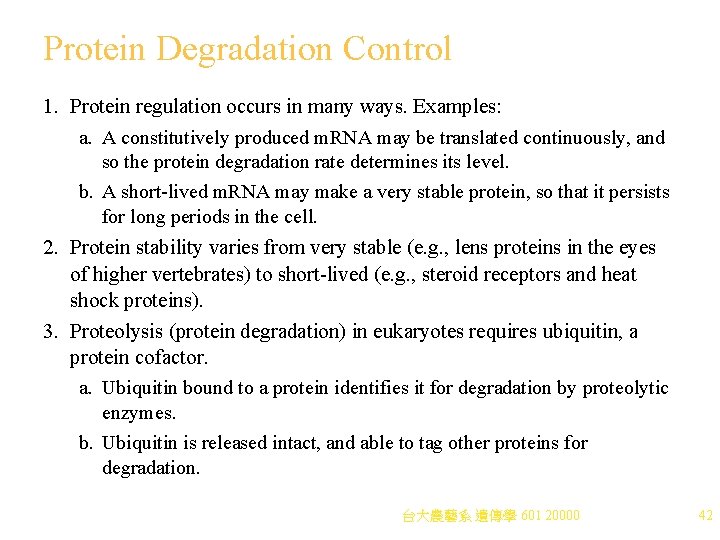
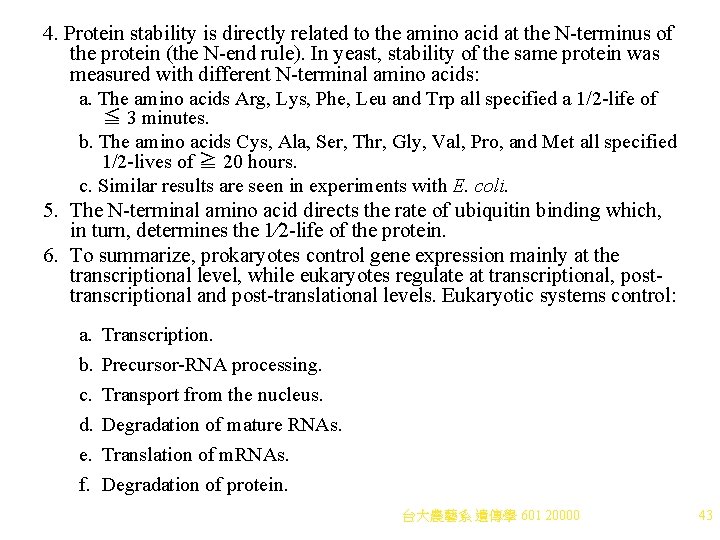
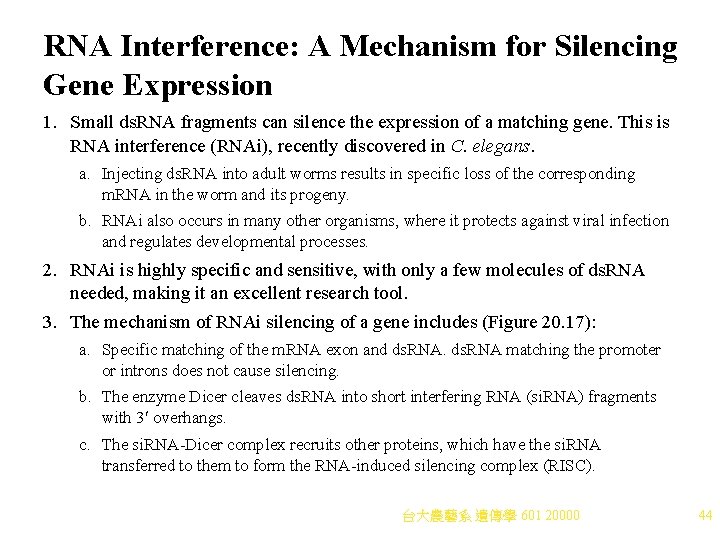
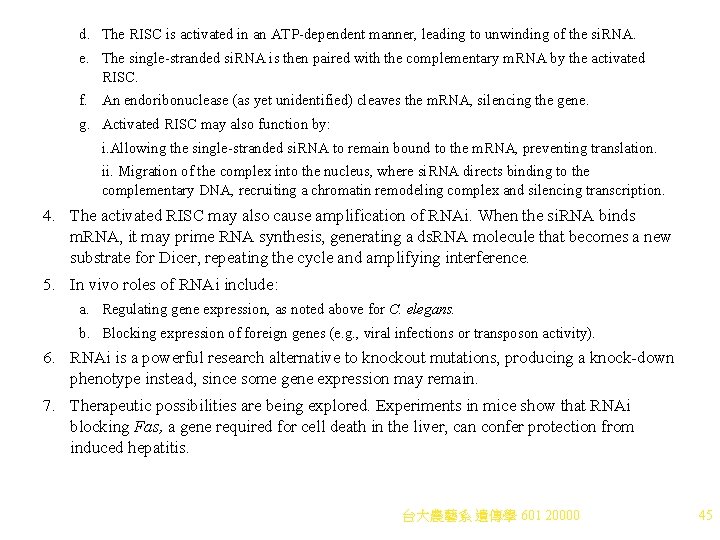
- Slides: 45
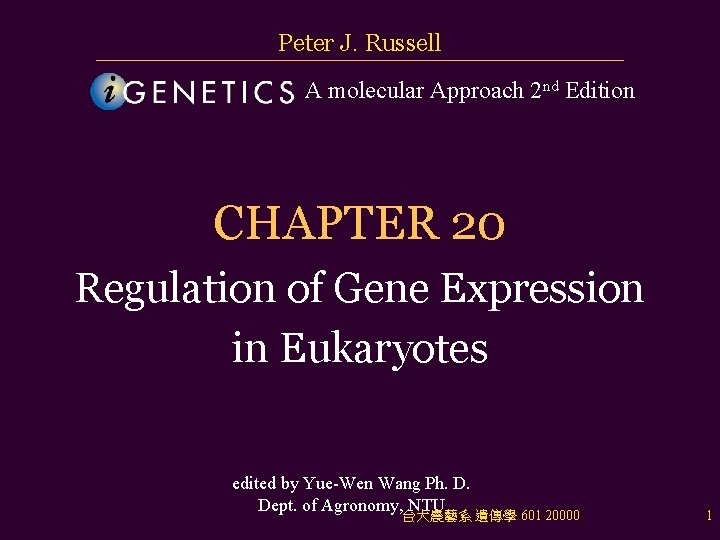
Peter J. Russell A molecular Approach 2 nd Edition CHAPTER 20 Regulation of Gene Expression in Eukaryotes edited by Yue-Wen Wang Ph. D. Dept. of Agronomy, NTU 台大農藝系 遺傳學 601 20000 1
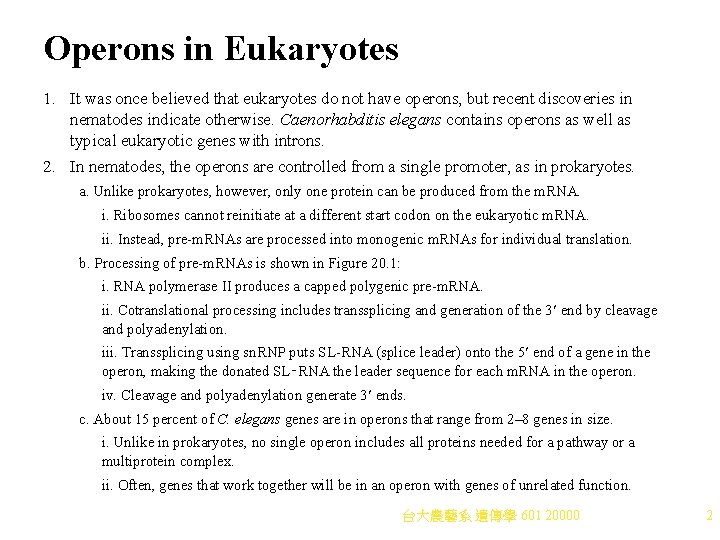
Operons in Eukaryotes 1. It was once believed that eukaryotes do not have operons, but recent discoveries in nematodes indicate otherwise. Caenorhabditis elegans contains operons as well as typical eukaryotic genes with introns. 2. In nematodes, the operons are controlled from a single promoter, as in prokaryotes. a. Unlike prokaryotes, however, only one protein can be produced from the m. RNA. i. Ribosomes cannot reinitiate at a different start codon on the eukaryotic m. RNA. ii. Instead, pre-m. RNAs are processed into monogenic m. RNAs for individual translation. b. Processing of pre-m. RNAs is shown in Figure 20. 1: i. RNA polymerase II produces a capped polygenic pre-m. RNA. ii. Cotranslational processing includes transsplicing and generation of the 3¢ end by cleavage and polyadenylation. iii. Transsplicing using sn. RNP puts SL-RNA (splice leader) onto the 5¢ end of a gene in the operon, making the donated SL‑RNA the leader sequence for each m. RNA in the operon. iv. Cleavage and polyadenylation generate 3¢ ends. c. About 15 percent of C. elegans genes are in operons that range from 2– 8 genes in size. i. Unlike in prokaryotes, no single operon includes all proteins needed for a pathway or a multiprotein complex. ii. Often, genes that work together will be in an operon with genes of unrelated function. 台大農藝系 遺傳學 601 20000 2
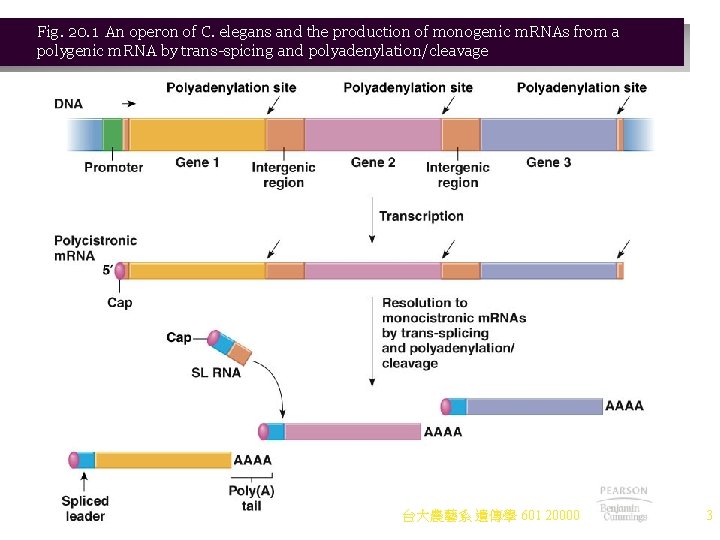
Fig. 20. 1 An operon of C. elegans and the production of monogenic m. RNAs from a polygenic m. RNA by trans-spicing and polyadenylation/cleavage 台大農藝系 遺傳學 601 20000 3
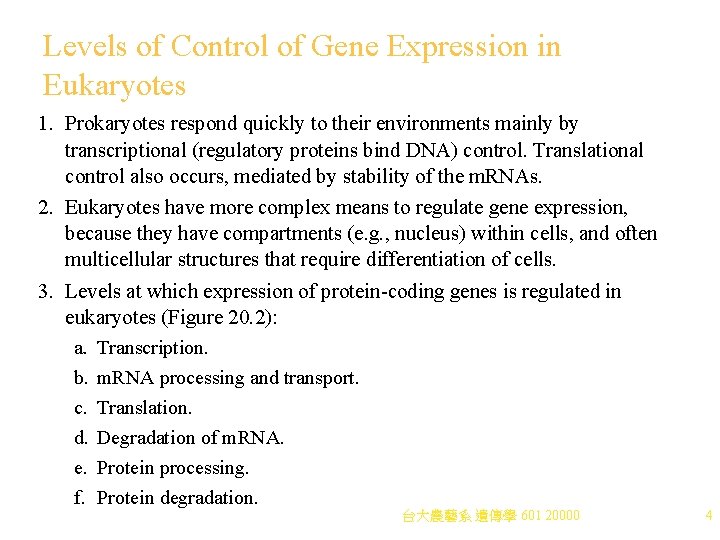
Levels of Control of Gene Expression in Eukaryotes 1. Prokaryotes respond quickly to their environments mainly by transcriptional (regulatory proteins bind DNA) control. Translational control also occurs, mediated by stability of the m. RNAs. 2. Eukaryotes have more complex means to regulate gene expression, because they have compartments (e. g. , nucleus) within cells, and often multicellular structures that require differentiation of cells. 3. Levels at which expression of protein-coding genes is regulated in eukaryotes (Figure 20. 2): a. Transcription. b. m. RNA processing and transport. c. Translation. d. Degradation of m. RNA. e. Protein processing. f. Protein degradation. 台大農藝系 遺傳學 601 20000 4
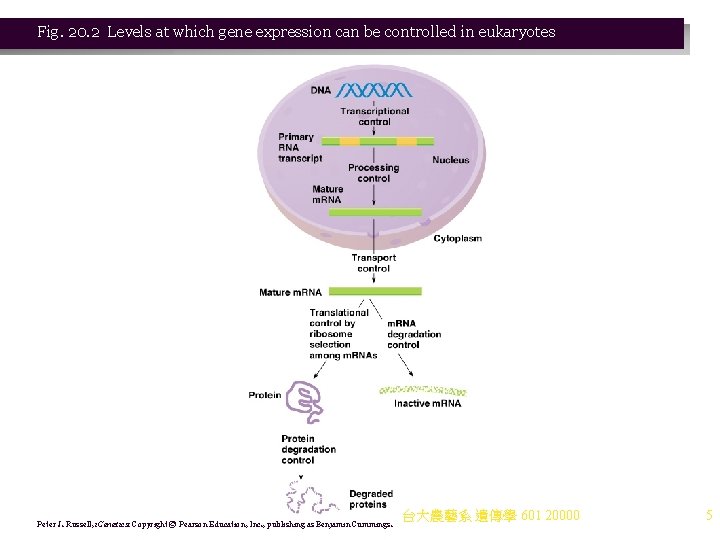
Fig. 20. 2 Levels at which gene expression can be controlled in eukaryotes Peter J. Russell, i. Genetics: Copyright © Pearson Education, Inc. , publishing as Benjamin Cummings. 台大農藝系 遺傳學 601 20000 5
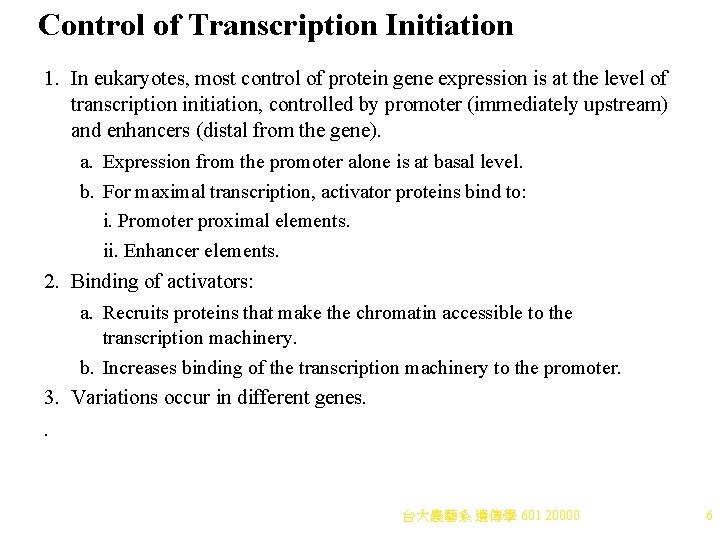
Control of Transcription Initiation 1. In eukaryotes, most control of protein gene expression is at the level of transcription initiation, controlled by promoter (immediately upstream) and enhancers (distal from the gene). a. Expression from the promoter alone is at basal level. b. For maximal transcription, activator proteins bind to: i. Promoter proximal elements. ii. Enhancer elements. 2. Binding of activators: a. Recruits proteins that make the chromatin accessible to the transcription machinery. b. Increases binding of the transcription machinery to the promoter. 3. Variations occur in different genes. . 台大農藝系 遺傳學 601 20000 6
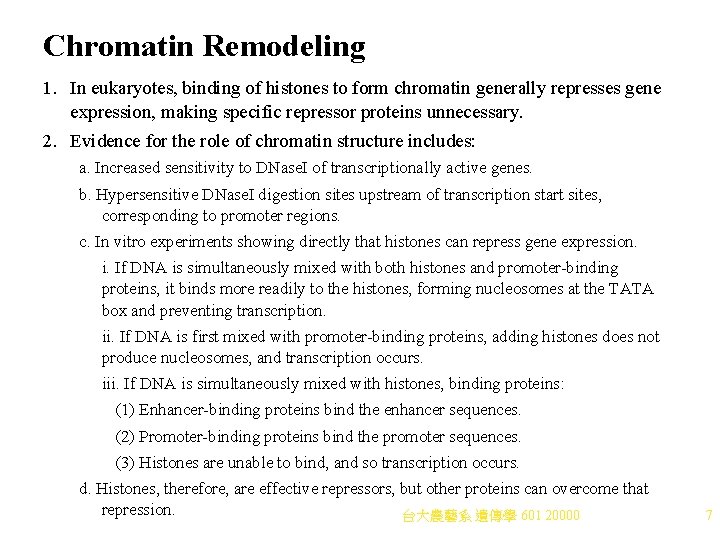
Chromatin Remodeling 1. In eukaryotes, binding of histones to form chromatin generally represses gene expression, making specific repressor proteins unnecessary. 2. Evidence for the role of chromatin structure includes: a. Increased sensitivity to DNase. I of transcriptionally active genes. b. Hypersensitive DNase. I digestion sites upstream of transcription start sites, corresponding to promoter regions. c. In vitro experiments showing directly that histones can repress gene expression. i. If DNA is simultaneously mixed with both histones and promoter-binding proteins, it binds more readily to the histones, forming nucleosomes at the TATA box and preventing transcription. ii. If DNA is first mixed with promoter-binding proteins, adding histones does not produce nucleosomes, and transcription occurs. iii. If DNA is simultaneously mixed with histones, binding proteins: (1) Enhancer-binding proteins bind the enhancer sequences. (2) Promoter-binding proteins bind the promoter sequences. (3) Histones are unable to bind, and so transcription occurs. d. Histones, therefore, are effective repressors, but other proteins can overcome that repression. 台大農藝系 遺傳學 601 20000 7
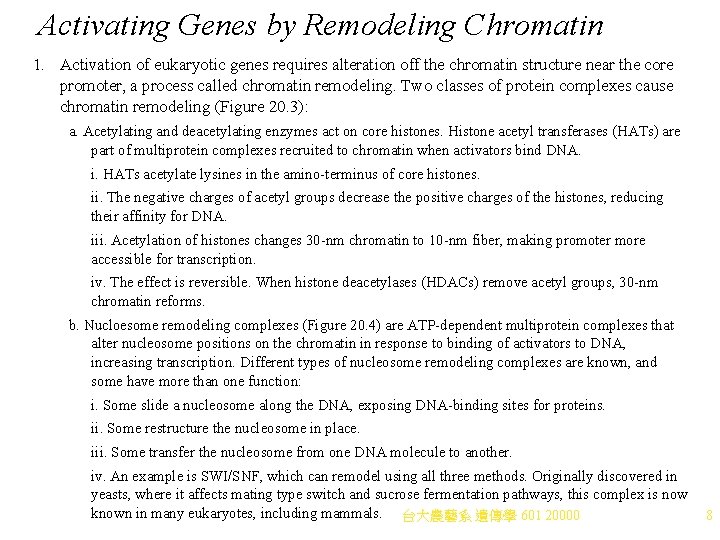
Activating Genes by Remodeling Chromatin 1. Activation of eukaryotic genes requires alteration off the chromatin structure near the core promoter, a process called chromatin remodeling. Two classes of protein complexes cause chromatin remodeling (Figure 20. 3): a. Acetylating and deacetylating enzymes act on core histones. Histone acetyl transferases (HATs) are part of multiprotein complexes recruited to chromatin when activators bind DNA. i. HATs acetylate lysines in the amino-terminus of core histones. ii. The negative charges of acetyl groups decrease the positive charges of the histones, reducing their affinity for DNA. iii. Acetylation of histones changes 30 -nm chromatin to 10 -nm fiber, making promoter more accessible for transcription. iv. The effect is reversible. When histone deacetylases (HDACs) remove acetyl groups, 30 -nm chromatin reforms. b. Nucloesome remodeling complexes (Figure 20. 4) are ATP-dependent multiprotein complexes that alter nucleosome positions on the chromatin in response to binding of activators to DNA, increasing transcription. Different types of nucleosome remodeling complexes are known, and some have more than one function: i. Some slide a nucleosome along the DNA, exposing DNA-binding sites for proteins. ii. Some restructure the nucleosome in place. iii. Some transfer the nucleosome from one DNA molecule to another. iv. An example is SWI/SNF, which can remodel using all three methods. Originally discovered in yeasts, where it affects mating type switch and sucrose fermentation pathways, this complex is now known in many eukaryotes, including mammals. 台大農藝系 遺傳學 601 20000 8
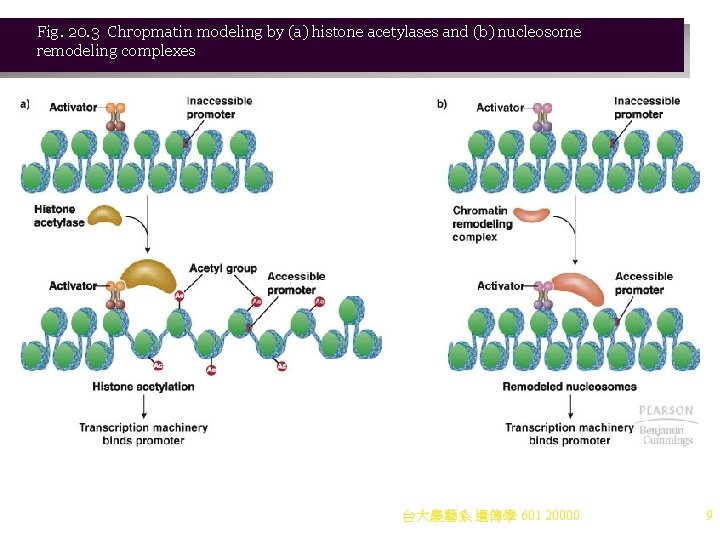
Fig. 20. 3 Chropmatin modeling by (a) histone acetylases and (b) nucleosome remodeling complexes 台大農藝系 遺傳學 601 20000 9
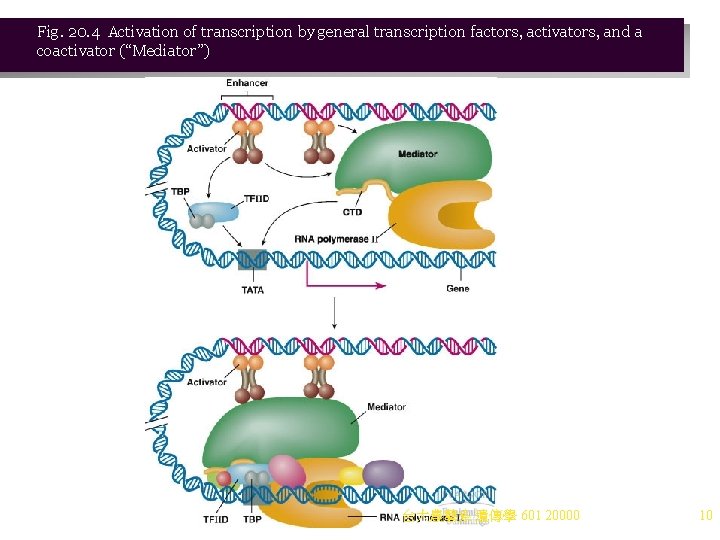
Fig. 20. 4 Activation of transcription by general transcription factors, activators, and a coactivator (“Mediator”) 台大農藝系 遺傳學 601 20000 10
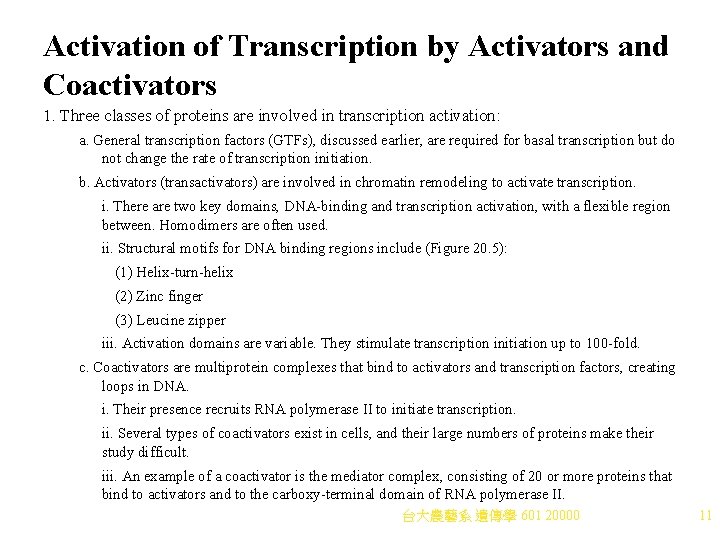
Activation of Transcription by Activators and Coactivators 1. Three classes of proteins are involved in transcription activation: a. General transcription factors (GTFs), discussed earlier, are required for basal transcription but do not change the rate of transcription initiation. b. Activators (transactivators) are involved in chromatin remodeling to activate transcription. i. There are two key domains, DNA-binding and transcription activation, with a flexible region between. Homodimers are often used. ii. Structural motifs for DNA binding regions include (Figure 20. 5): (1) Helix-turn-helix (2) Zinc finger (3) Leucine zipper iii. Activation domains are variable. They stimulate transcription initiation up to 100 -fold. c. Coactivators are multiprotein complexes that bind to activators and transcription factors, creating loops in DNA. i. Their presence recruits RNA polymerase II to initiate transcription. ii. Several types of coactivators exist in cells, and their large numbers of proteins make their study difficult. iii. An example of a coactivator is the mediator complex, consisting of 20 or more proteins that bind to activators and to the carboxy-terminal domain of RNA polymerase II. 台大農藝系 遺傳學 601 20000 11
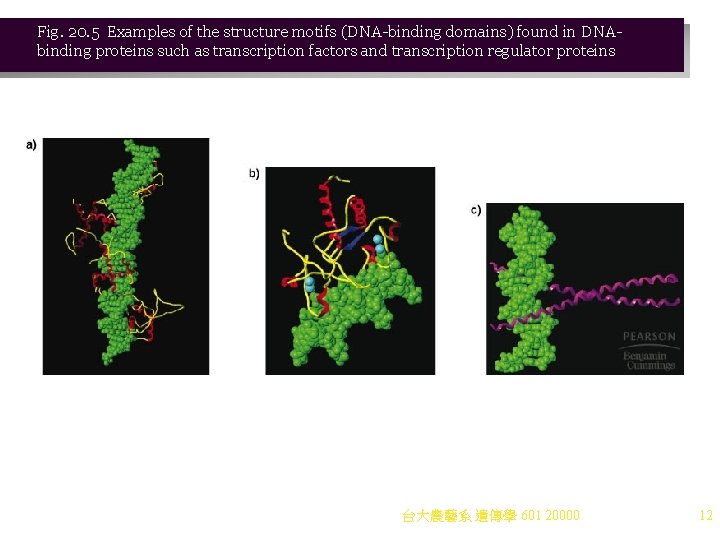
Fig. 20. 5 Examples of the structure motifs (DNA-binding domains) found in DNAbinding proteins such as transcription factors and transcription regulator proteins 台大農藝系 遺傳學 601 20000 12
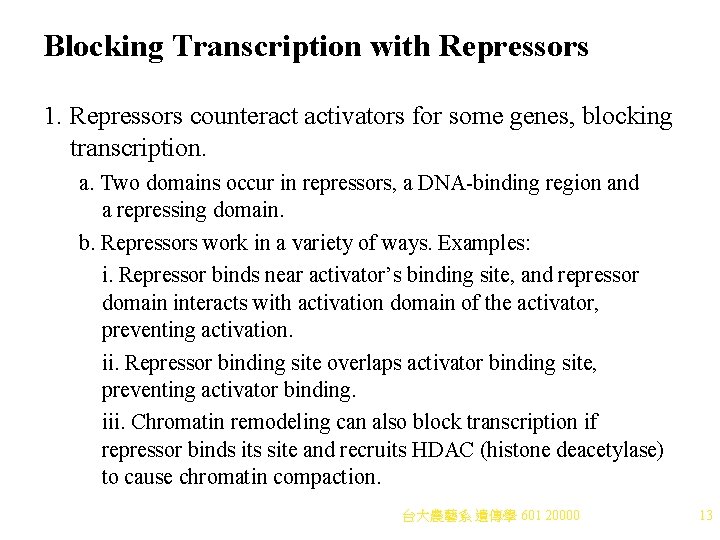
Blocking Transcription with Repressors 1. Repressors counteract activators for some genes, blocking transcription. a. Two domains occur in repressors, a DNA-binding region and a repressing domain. b. Repressors work in a variety of ways. Examples: i. Repressor binds near activator’s binding site, and repressor domain interacts with activation domain of the activator, preventing activation. ii. Repressor binding site overlaps activator binding site, preventing activator binding. iii. Chromatin remodeling can also block transcription if repressor binds its site and recruits HDAC (histone deacetylase) to cause chromatin compaction. 台大農藝系 遺傳學 601 20000 13
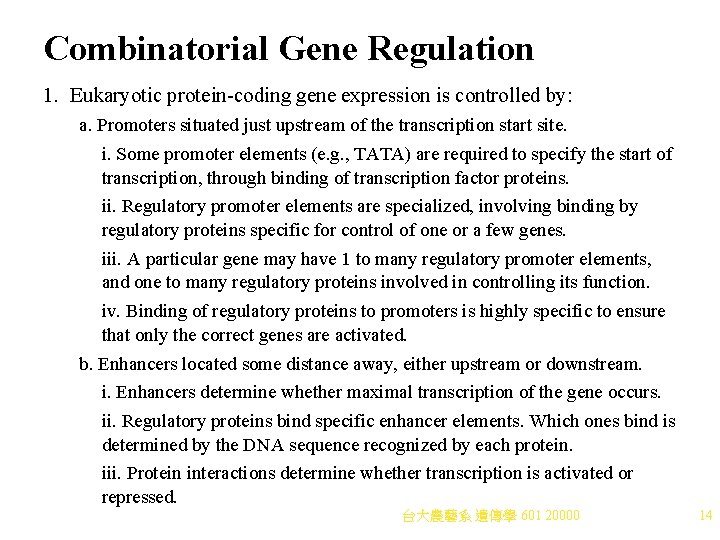
Combinatorial Gene Regulation 1. Eukaryotic protein-coding gene expression is controlled by: a. Promoters situated just upstream of the transcription start site. i. Some promoter elements (e. g. , TATA) are required to specify the start of transcription, through binding of transcription factor proteins. ii. Regulatory promoter elements are specialized, involving binding by regulatory proteins specific for control of one or a few genes. iii. A particular gene may have 1 to many regulatory promoter elements, and one to many regulatory proteins involved in controlling its function. iv. Binding of regulatory proteins to promoters is highly specific to ensure that only the correct genes are activated. b. Enhancers located some distance away, either upstream or downstream. i. Enhancers determine whether maximal transcription of the gene occurs. ii. Regulatory proteins bind specific enhancer elements. Which ones bind is determined by the DNA sequence recognized by each protein. iii. Protein interactions determine whether transcription is activated or repressed. 台大農藝系 遺傳學 601 20000 14
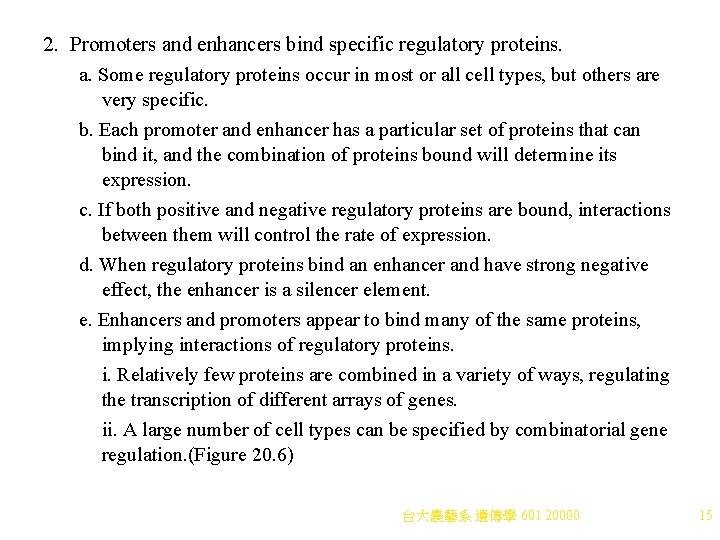
2. Promoters and enhancers bind specific regulatory proteins. a. Some regulatory proteins occur in most or all cell types, but others are very specific. b. Each promoter and enhancer has a particular set of proteins that can bind it, and the combination of proteins bound will determine its expression. c. If both positive and negative regulatory proteins are bound, interactions between them will control the rate of expression. d. When regulatory proteins bind an enhancer and have strong negative effect, the enhancer is a silencer element. e. Enhancers and promoters appear to bind many of the same proteins, implying interactions of regulatory proteins. i. Relatively few proteins are combined in a variety of ways, regulating the transcription of different arrays of genes. ii. A large number of cell types can be specified by combinatorial gene regulation. (Figure 20. 6) 台大農藝系 遺傳學 601 20000 15
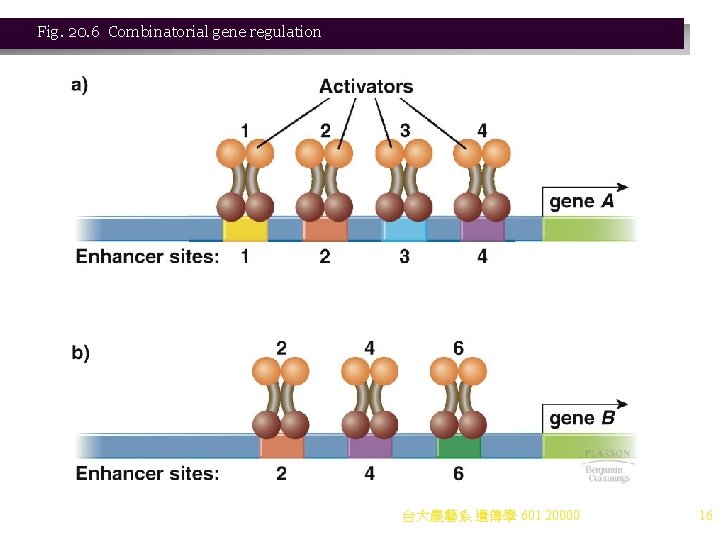
Fig. 20. 6 Combinatorial gene regulation 台大農藝系 遺傳學 601 20000 16
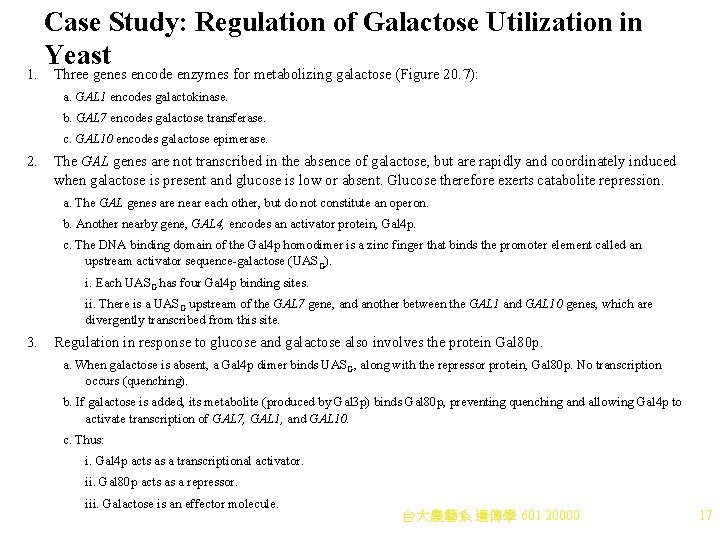
1. Case Study: Regulation of Galactose Utilization in Yeast Three genes encode enzymes for metabolizing galactose (Figure 20. 7): a. GAL 1 encodes galactokinase. b. GAL 7 encodes galactose transferase. c. GAL 10 encodes galactose epimerase. 2. The GAL genes are not transcribed in the absence of galactose, but are rapidly and coordinately induced when galactose is present and glucose is low or absent. Glucose therefore exerts catabolite repression. a. The GAL genes are near each other, but do not constitute an operon. b. Another nearby gene, GAL 4, encodes an activator protein, Gal 4 p. c. The DNA binding domain of the Gal 4 p homodimer is a zinc finger that binds the promoter element called an upstream activator sequence-galactose (UASG). i. Each UASG has four Gal 4 p binding sites. ii. There is a UASG upstream of the GAL 7 gene, and another between the GAL 1 and GAL 10 genes, which are divergently transcribed from this site. 3. Regulation in response to glucose and galactose also involves the protein Gal 80 p. a. When galactose is absent, a Gal 4 p dimer binds UASG, along with the repressor protein, Gal 80 p. No transcription occurs (quenching). b. If galactose is added, its metabolite (produced by Gal 3 p) binds Gal 80 p, preventing quenching and allowing Gal 4 p to activate transcription of GAL 7, GAL 1, and GAL 10. c. Thus: i. Gal 4 p acts as a transcriptional activator. ii. Gal 80 p acts as a repressor. iii. Galactose is an effector molecule. 台大農藝系 遺傳學 601 20000 17
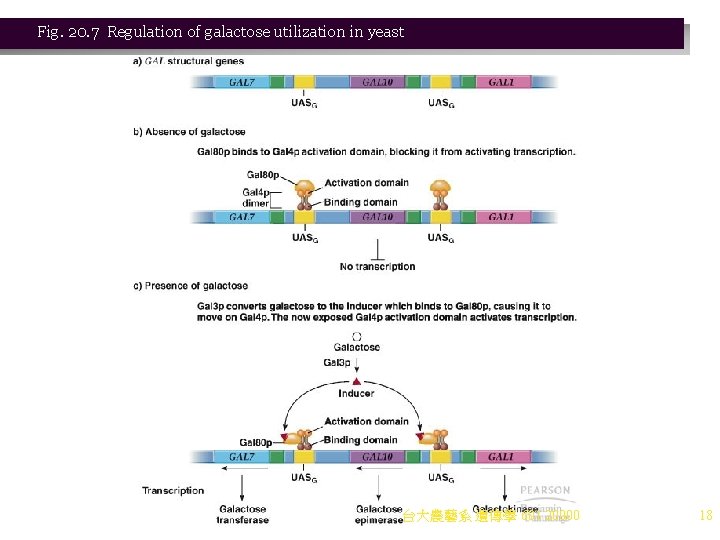
Fig. 20. 7 Regulation of galactose utilization in yeast 台大農藝系 遺傳學 601 20000 18
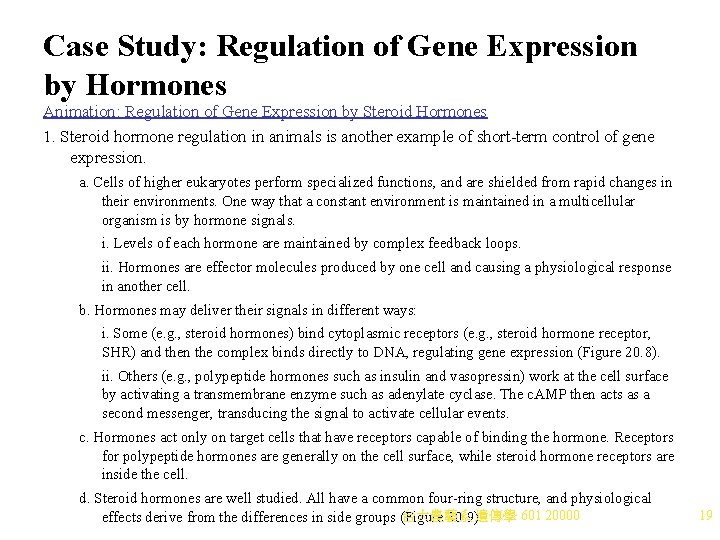
Case Study: Regulation of Gene Expression by Hormones Animation: Regulation of Gene Expression by Steroid Hormones 1. Steroid hormone regulation in animals is another example of short-term control of gene expression. a. Cells of higher eukaryotes perform specialized functions, and are shielded from rapid changes in their environments. One way that a constant environment is maintained in a multicellular organism is by hormone signals. i. Levels of each hormone are maintained by complex feedback loops. ii. Hormones are effector molecules produced by one cell and causing a physiological response in another cell. b. Hormones may deliver their signals in different ways: i. Some (e. g. , steroid hormones) bind cytoplasmic receptors (e. g. , steroid hormone receptor, SHR) and then the complex binds directly to DNA, regulating gene expression (Figure 20. 8). ii. Others (e. g. , polypeptide hormones such as insulin and vasopressin) work at the cell surface by activating a transmembrane enzyme such as adenylate cyclase. The c. AMP then acts as a second messenger, transducing the signal to activate cellular events. c. Hormones act only on target cells that have receptors capable of binding the hormone. Receptors for polypeptide hormones are generally on the cell surface, while steroid hormone receptors are inside the cell. d. Steroid hormones are well studied. All have a common four-ring structure, and physiological 台大農藝系 遺傳學 601 20000 effects derive from the differences in side groups (Figure 20. 9). 19
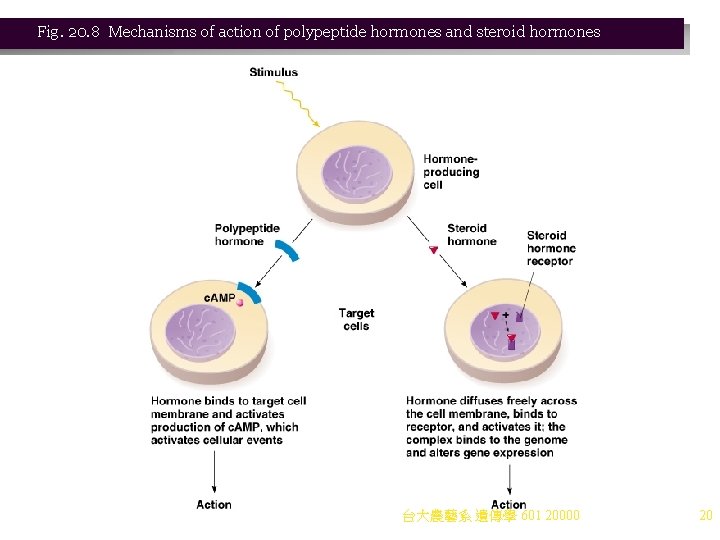
Fig. 20. 8 Mechanisms of action of polypeptide hormones and steroid hormones 台大農藝系 遺傳學 601 20000 20
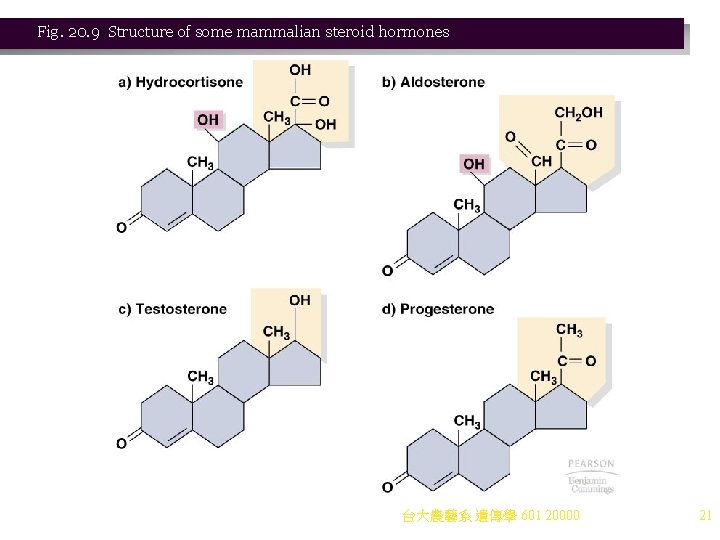
Fig. 20. 9 Structure of some mammalian steroid hormones 台大農藝系 遺傳學 601 20000 21
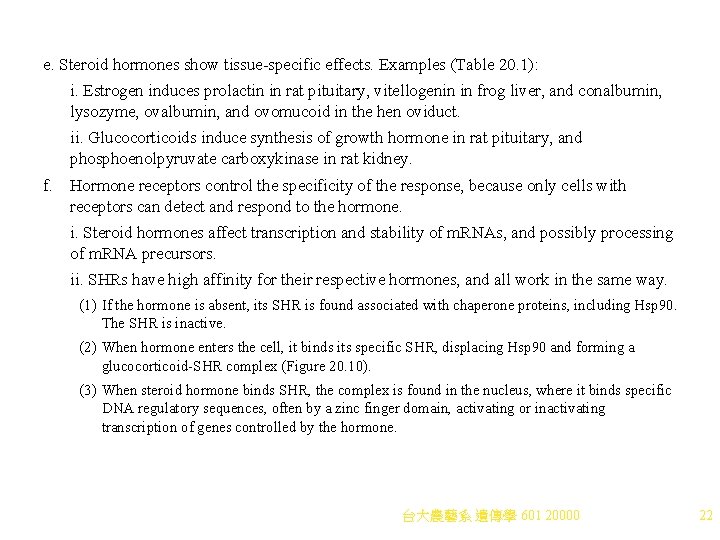
e. Steroid hormones show tissue-specific effects. Examples (Table 20. 1): i. Estrogen induces prolactin in rat pituitary, vitellogenin in frog liver, and conalbumin, lysozyme, ovalbumin, and ovomucoid in the hen oviduct. ii. Glucocorticoids induce synthesis of growth hormone in rat pituitary, and phosphoenolpyruvate carboxykinase in rat kidney. f. Hormone receptors control the specificity of the response, because only cells with receptors can detect and respond to the hormone. i. Steroid hormones affect transcription and stability of m. RNAs, and possibly processing of m. RNA precursors. ii. SHRs have high affinity for their respective hormones, and all work in the same way. (1) If the hormone is absent, its SHR is found associated with chaperone proteins, including Hsp 90. The SHR is inactive. (2) When hormone enters the cell, it binds its specific SHR, displacing Hsp 90 and forming a glucocorticoid-SHR complex (Figure 20. 10). (3) When steroid hormone binds SHR, the complex is found in the nucleus, where it binds specific DNA regulatory sequences, often by a zinc finger domain, activating or inactivating transcription of genes controlled by the hormone. 台大農藝系 遺傳學 601 20000 22
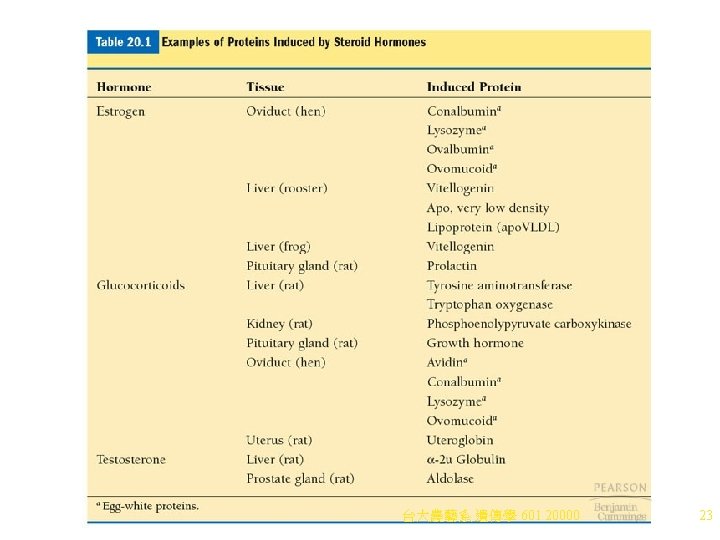
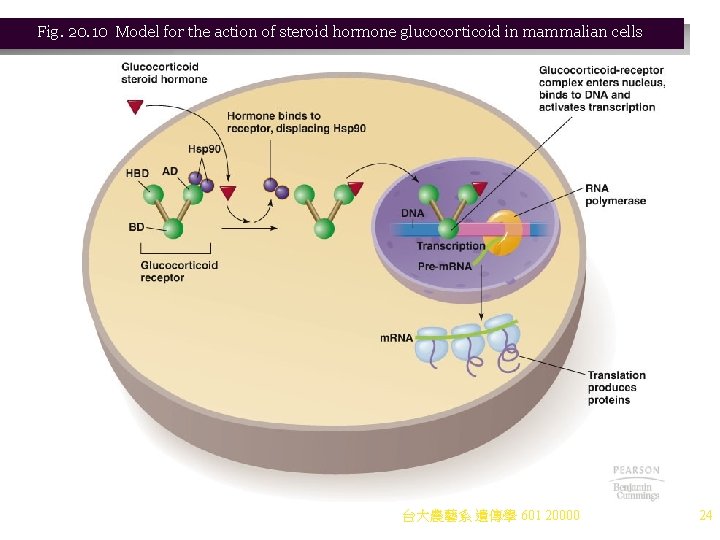
Fig. 20. 10 Model for the action of steroid hormone glucocorticoid in mammalian cells 台大農藝系 遺傳學 601 20000 24
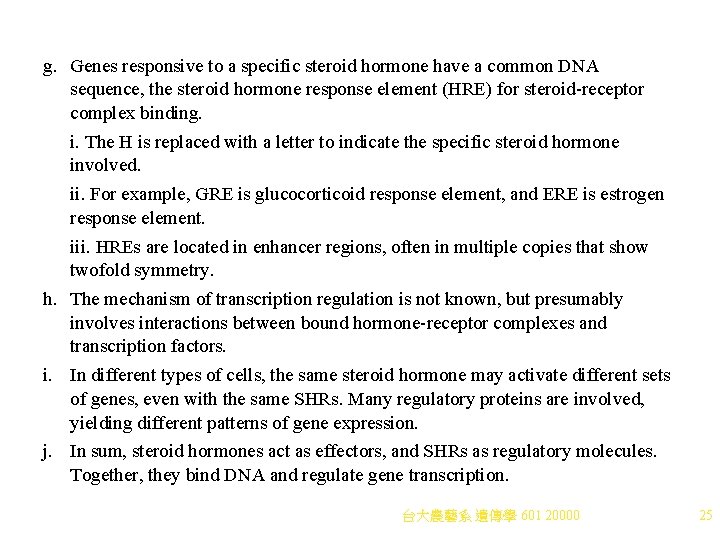
g. Genes responsive to a specific steroid hormone have a common DNA sequence, the steroid hormone response element (HRE) for steroid-receptor complex binding. i. The H is replaced with a letter to indicate the specific steroid hormone involved. ii. For example, GRE is glucocorticoid response element, and ERE is estrogen response element. iii. HREs are located in enhancer regions, often in multiple copies that show twofold symmetry. h. The mechanism of transcription regulation is not known, but presumably involves interactions between bound hormone-receptor complexes and transcription factors. i. In different types of cells, the same steroid hormone may activate different sets of genes, even with the same SHRs. Many regulatory proteins are involved, yielding different patterns of gene expression. j. In sum, steroid hormones act as effectors, and SHRs as regulatory molecules. Together, they bind DNA and regulate gene transcription. 台大農藝系 遺傳學 601 20000 25
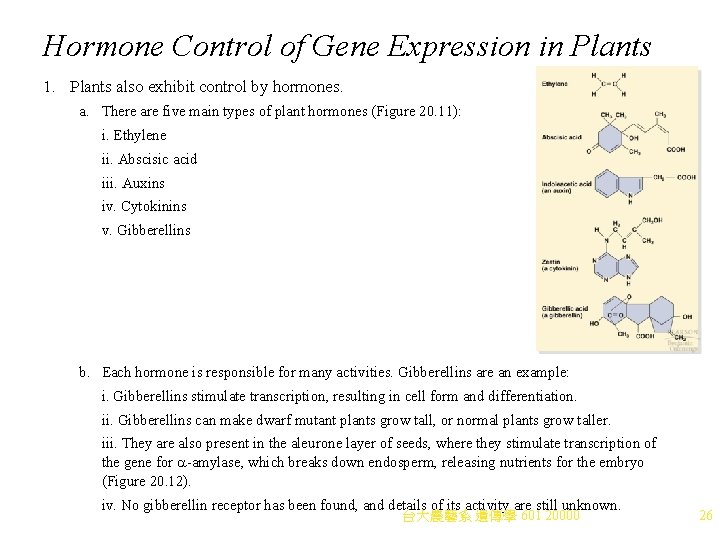
Hormone Control of Gene Expression in Plants 1. Plants also exhibit control by hormones. a. There are five main types of plant hormones (Figure 20. 11): i. Ethylene ii. Abscisic acid iii. Auxins iv. Cytokinins v. Gibberellins b. Each hormone is responsible for many activities. Gibberellins are an example: i. Gibberellins stimulate transcription, resulting in cell form and differentiation. ii. Gibberellins can make dwarf mutant plants grow tall, or normal plants grow taller. iii. They are also present in the aleurone layer of seeds, where they stimulate transcription of the gene for a-amylase, which breaks down endosperm, releasing nutrients for the embryo (Figure 20. 12). iv. No gibberellin receptor has been found, and details of its activity are still unknown. 台大農藝系 遺傳學 601 20000 26
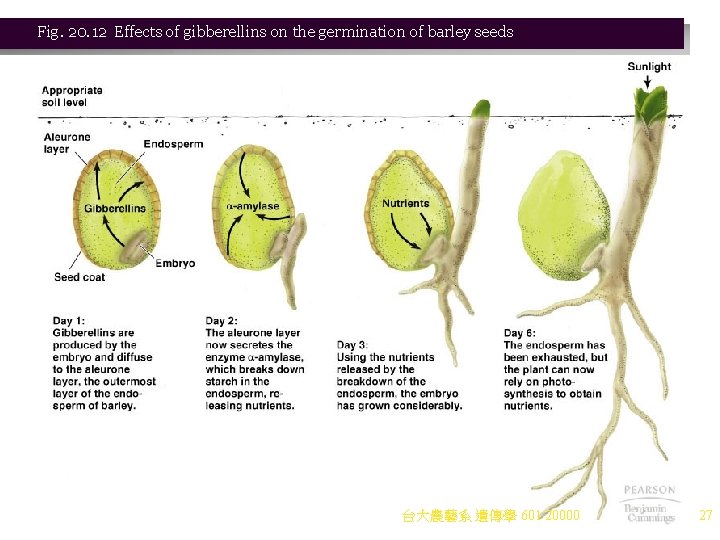
Fig. 20. 12 Effects of gibberellins on the germination of barley seeds 台大農藝系 遺傳學 601 20000 27
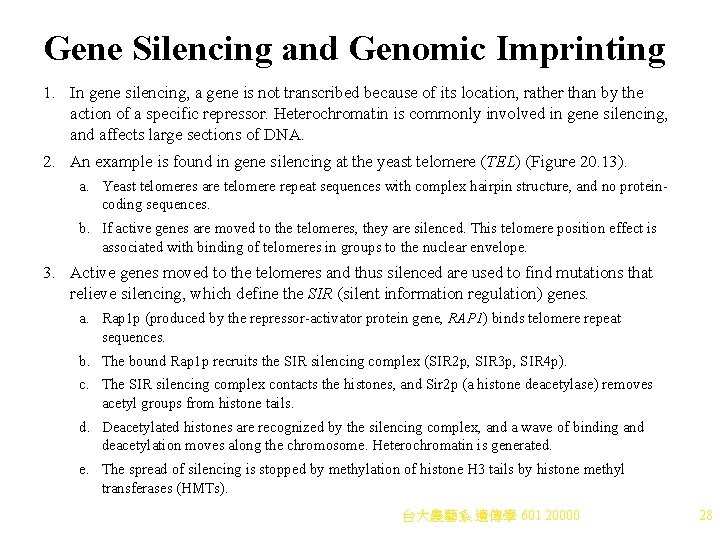
Gene Silencing and Genomic Imprinting 1. In gene silencing, a gene is not transcribed because of its location, rather than by the action of a specific repressor. Heterochromatin is commonly involved in gene silencing, and affects large sections of DNA. 2. An example is found in gene silencing at the yeast telomere (TEL) (Figure 20. 13). a. Yeast telomeres are telomere repeat sequences with complex hairpin structure, and no proteincoding sequences. b. If active genes are moved to the telomeres, they are silenced. This telomere position effect is associated with binding of telomeres in groups to the nuclear envelope. 3. Active genes moved to the telomeres and thus silenced are used to find mutations that relieve silencing, which define the SIR (silent information regulation) genes. a. Rap 1 p (produced by the repressor-activator protein gene, RAP 1) binds telomere repeat sequences. b. The bound Rap 1 p recruits the SIR silencing complex (SIR 2 p, SIR 3 p, SIR 4 p). c. The SIR silencing complex contacts the histones, and Sir 2 p (a histone deacetylase) removes acetyl groups from histone tails. d. Deacetylated histones are recognized by the silencing complex, and a wave of binding and deacetylation moves along the chromosome. Heterochromatin is generated. e. The spread of silencing is stopped by methylation of histone H 3 tails by histone methyl transferases (HMTs). 台大農藝系 遺傳學 601 20000 28
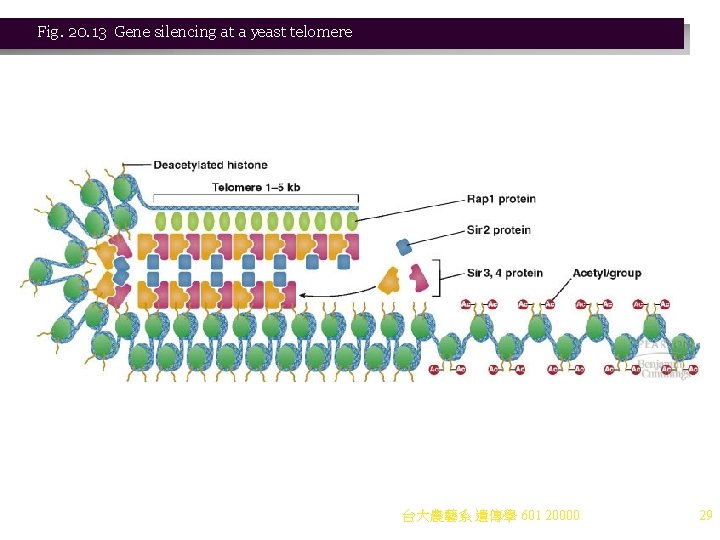
Fig. 20. 13 Gene silencing at a yeast telomere 台大農藝系 遺傳學 601 20000 29
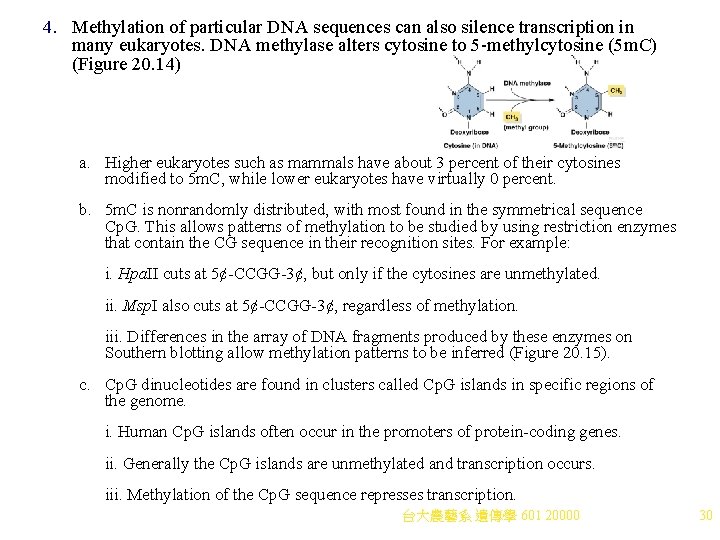
4. Methylation of particular DNA sequences can also silence transcription in many eukaryotes. DNA methylase alters cytosine to 5 -methylcytosine (5 m. C) (Figure 20. 14) a. Higher eukaryotes such as mammals have about 3 percent of their cytosines modified to 5 m. C, while lower eukaryotes have virtually 0 percent. b. 5 m. C is nonrandomly distributed, with most found in the symmetrical sequence Cp. G. This allows patterns of methylation to be studied by using restriction enzymes that contain the CG sequence in their recognition sites. For example: i. Hpa. II cuts at 5¢-CCGG-3¢, but only if the cytosines are unmethylated. ii. Msp. I also cuts at 5¢-CCGG-3¢, regardless of methylation. iii. Differences in the array of DNA fragments produced by these enzymes on Southern blotting allow methylation patterns to be inferred (Figure 20. 15). c. Cp. G dinucleotides are found in clusters called Cp. G islands in specific regions of the genome. i. Human Cp. G islands often occur in the promoters of protein-coding genes. ii. Generally the Cp. G islands are unmethylated and transcription occurs. iii. Methylation of the Cp. G sequence represses transcription. 台大農藝系 遺傳學 601 20000 30
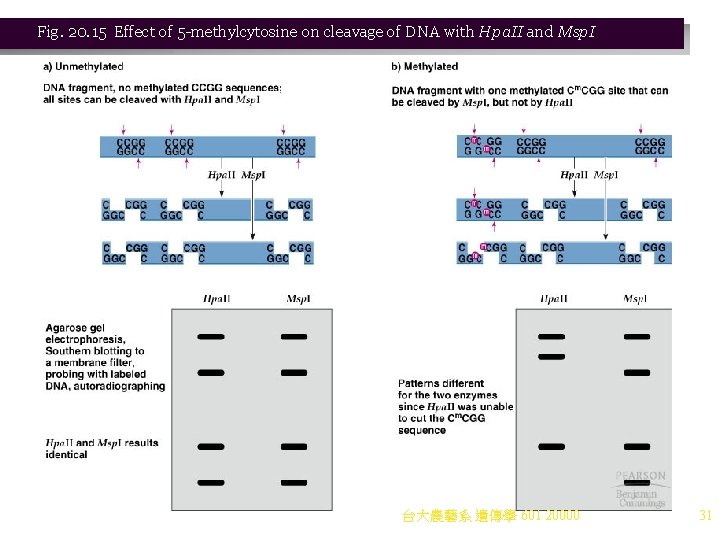
Fig. 20. 15 Effect of 5 -methylcytosine on cleavage of DNA with Hpa. II and Msp. I 台大農藝系 遺傳學 601 20000 31
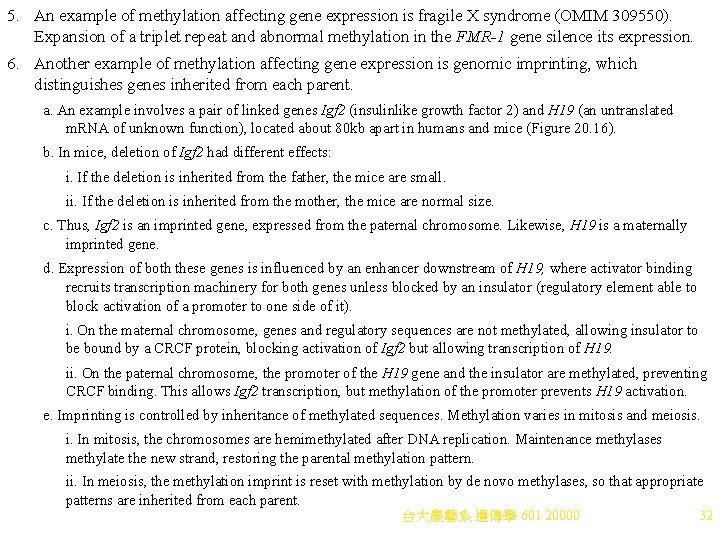
5. An example of methylation affecting gene expression is fragile X syndrome (OMIM 309550). Expansion of a triplet repeat and abnormal methylation in the FMR-1 gene silence its expression. 6. Another example of methylation affecting gene expression is genomic imprinting, which distinguishes genes inherited from each parent. a. An example involves a pair of linked genes Igf 2 (insulinlike growth factor 2) and H 19 (an untranslated m. RNA of unknown function), located about 80 kb apart in humans and mice (Figure 20. 16). b. In mice, deletion of Igf 2 had different effects: i. If the deletion is inherited from the father, the mice are small. ii. If the deletion is inherited from the mother, the mice are normal size. c. Thus, Igf 2 is an imprinted gene, expressed from the paternal chromosome. Likewise, H 19 is a maternally imprinted gene. d. Expression of both these genes is influenced by an enhancer downstream of H 19, where activator binding recruits transcription machinery for both genes unless blocked by an insulator (regulatory element able to block activation of a promoter to one side of it). i. On the maternal chromosome, genes and regulatory sequences are not methylated, allowing insulator to be bound by a CRCF protein, blocking activation of Igf 2 but allowing transcription of H 19. ii. On the paternal chromosome, the promoter of the H 19 gene and the insulator are methylated, preventing CRCF binding. This allows Igf 2 transcription, but methylation of the promoter prevents H 19 activation. e. Imprinting is controlled by inheritance of methylated sequences. Methylation varies in mitosis and meiosis. i. In mitosis, the chromosomes are hemimethylated after DNA replication. Maintenance methylases methylate the new strand, restoring the parental methylation pattern. ii. In meiosis, the methylation imprint is reset with methylation by de novo methylases, so that appropriate patterns are inherited from each parent. 台大農藝系 遺傳學 601 20000 32
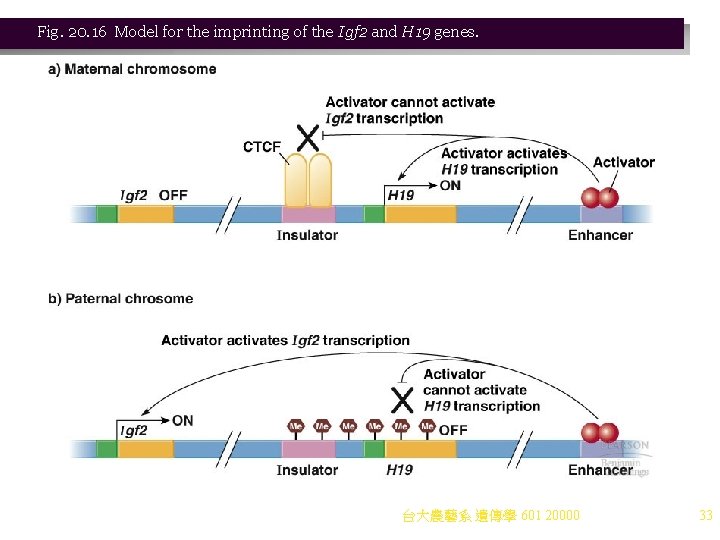
Fig. 20. 16 Model for the imprinting of the Igf 2 and H 19 genes. 台大農藝系 遺傳學 601 20000 33
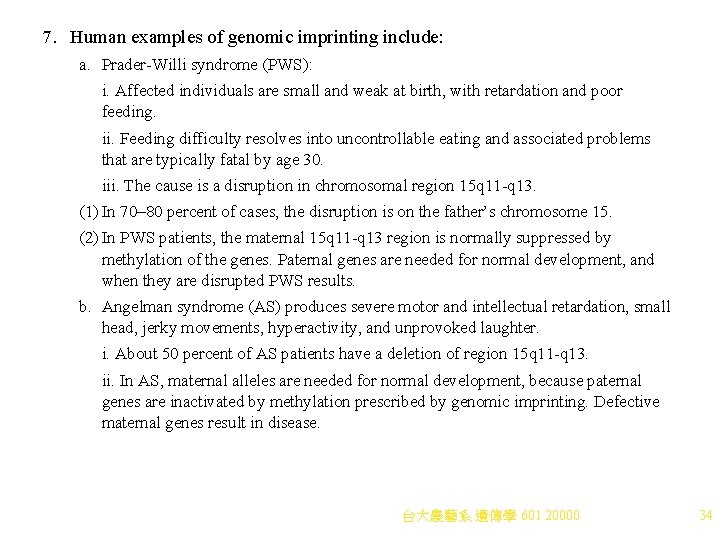
7. Human examples of genomic imprinting include: a. Prader-Willi syndrome (PWS): i. Affected individuals are small and weak at birth, with retardation and poor feeding. ii. Feeding difficulty resolves into uncontrollable eating and associated problems that are typically fatal by age 30. iii. The cause is a disruption in chromosomal region 15 q 11 -q 13. (1) In 70– 80 percent of cases, the disruption is on the father’s chromosome 15. (2) In PWS patients, the maternal 15 q 11 -q 13 region is normally suppressed by methylation of the genes. Paternal genes are needed for normal development, and when they are disrupted PWS results. b. Angelman syndrome (AS) produces severe motor and intellectual retardation, small head, jerky movements, hyperactivity, and unprovoked laughter. i. About 50 percent of AS patients have a deletion of region 15 q 11 -q 13. ii. In AS, maternal alleles are needed for normal development, because paternal genes are inactivated by methylation prescribed by genomic imprinting. Defective maternal genes result in disease. 台大農藝系 遺傳學 601 20000 34
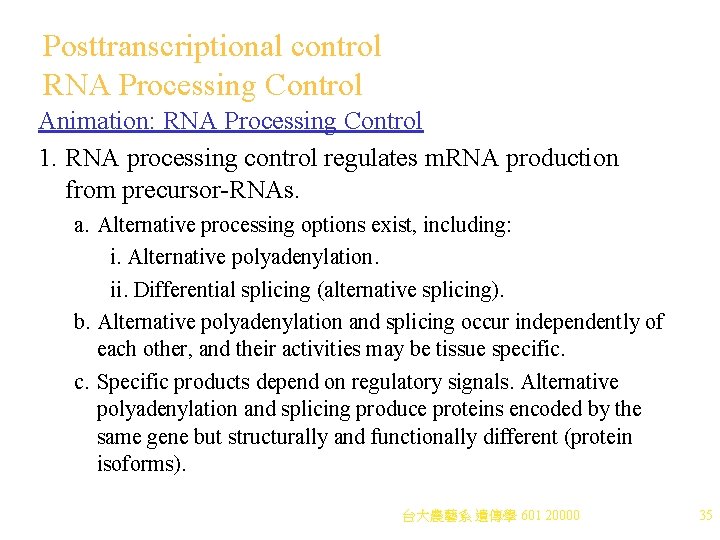
Posttranscriptional control RNA Processing Control Animation: RNA Processing Control 1. RNA processing control regulates m. RNA production from precursor-RNAs. a. Alternative processing options exist, including: i. Alternative polyadenylation. ii. Differential splicing (alternative splicing). b. Alternative polyadenylation and splicing occur independently of each other, and their activities may be tissue specific. c. Specific products depend on regulatory signals. Alternative polyadenylation and splicing produce proteins encoded by the same gene but structurally and functionally different (protein isoforms). 台大農藝系 遺傳學 601 20000 35
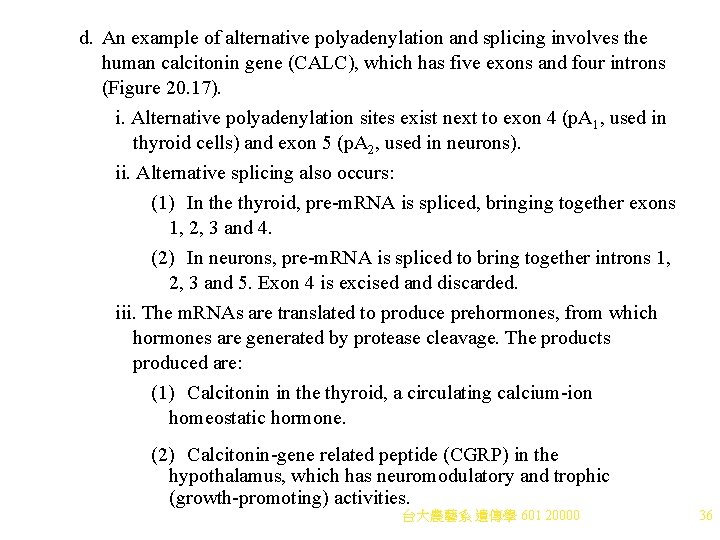
d. An example of alternative polyadenylation and splicing involves the human calcitonin gene (CALC), which has five exons and four introns (Figure 20. 17). i. Alternative polyadenylation sites exist next to exon 4 (p. A 1, used in thyroid cells) and exon 5 (p. A 2, used in neurons). ii. Alternative splicing also occurs: (1) In the thyroid, pre-m. RNA is spliced, bringing together exons 1, 2, 3 and 4. (2) In neurons, pre-m. RNA is spliced to bring together introns 1, 2, 3 and 5. Exon 4 is excised and discarded. iii. The m. RNAs are translated to produce prehormones, from which hormones are generated by protease cleavage. The products produced are: (1) Calcitonin in the thyroid, a circulating calcium-ion homeostatic hormone. (2) Calcitonin-gene related peptide (CGRP) in the hypothalamus, which has neuromodulatory and trophic (growth-promoting) activities. 台大農藝系 遺傳學 601 20000 36
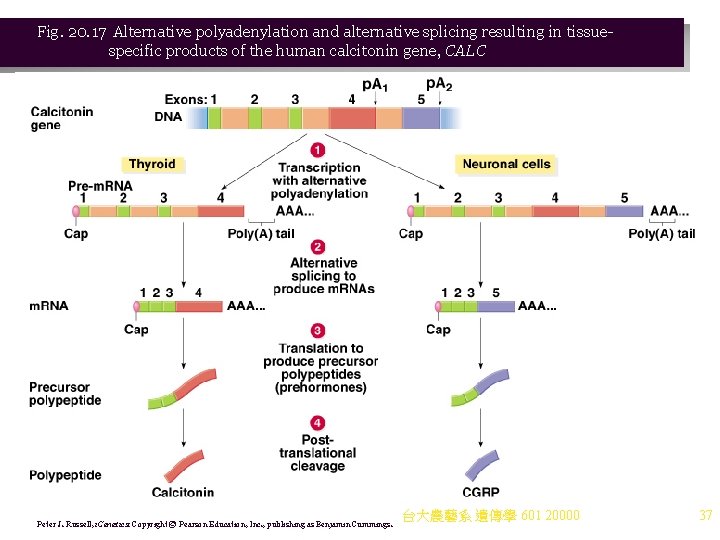
Fig. 20. 17 Alternative polyadenylation and alternative splicing resulting in tissuespecific products of the human calcitonin gene, CALC Peter J. Russell, i. Genetics: Copyright © Pearson Education, Inc. , publishing as Benjamin Cummings. 台大農藝系 遺傳學 601 20000 37
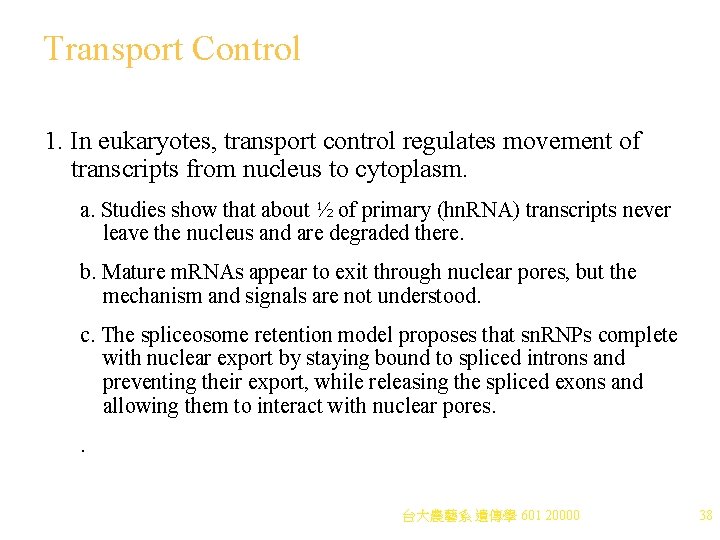
Transport Control 1. In eukaryotes, transport control regulates movement of transcripts from nucleus to cytoplasm. a. Studies show that about ½ of primary (hn. RNA) transcripts never leave the nucleus and are degraded there. b. Mature m. RNAs appear to exit through nuclear pores, but the mechanism and signals are not understood. c. The spliceosome retention model proposes that sn. RNPs complete with nuclear export by staying bound to spliced introns and preventing their export, while releasing the spliced exons and allowing them to interact with nuclear pores. . 台大農藝系 遺傳學 601 20000 38
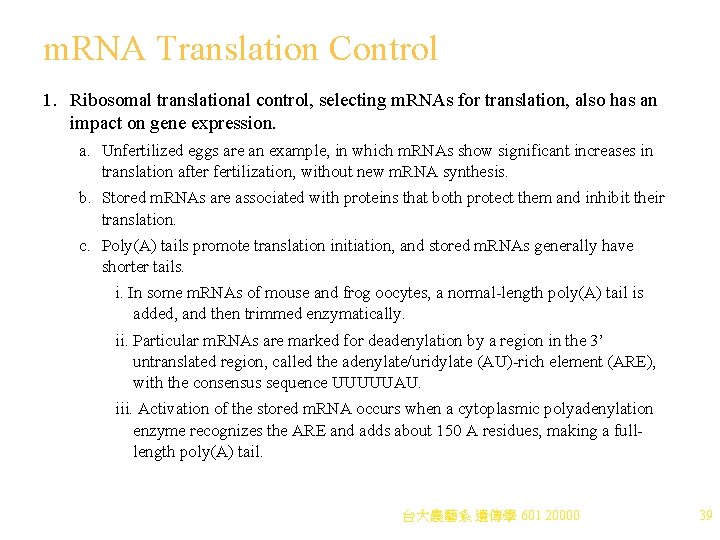
m. RNA Translation Control 1. Ribosomal translational control, selecting m. RNAs for translation, also has an impact on gene expression. a. Unfertilized eggs are an example, in which m. RNAs show significant increases in translation after fertilization, without new m. RNA synthesis. b. Stored m. RNAs are associated with proteins that both protect them and inhibit their translation. c. Poly(A) tails promote translation initiation, and stored m. RNAs generally have shorter tails. i. In some m. RNAs of mouse and frog oocytes, a normal-length poly(A) tail is added, and then trimmed enzymatically. ii. Particular m. RNAs are marked for deadenylation by a region in the 3’ untranslated region, called the adenylate/uridylate (AU)-rich element (ARE), with the consensus sequence UUUUUAU. iii. Activation of the stored m. RNA occurs when a cytoplasmic polyadenylation enzyme recognizes the ARE and adds about 150 A residues, making a fulllength poly(A) tail. 台大農藝系 遺傳學 601 20000 39
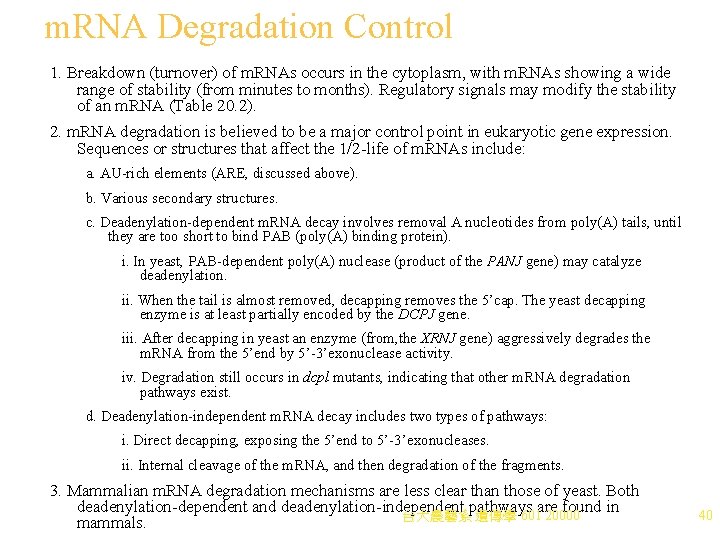
m. RNA Degradation Control 1. Breakdown (turnover) of m. RNAs occurs in the cytoplasm, with m. RNAs showing a wide range of stability (from minutes to months). Regulatory signals may modify the stability of an m. RNA (Table 20. 2). 2. m. RNA degradation is believed to be a major control point in eukaryotic gene expression. Sequences or structures that affect the 1/2 -life of m. RNAs include: a. AU-rich elements (ARE, discussed above). b. Various secondary structures. c. Deadenylation-dependent m. RNA decay involves removal A nucleotides from poly(A) tails, until they are too short to bind PAB (poly(A) binding protein). i. In yeast, PAB-dependent poly(A) nuclease (product of the PANJ gene) may catalyze deadenylation. ii. When the tail is almost removed, decapping removes the 5’cap. The yeast decapping enzyme is at least partially encoded by the DCPJ gene. iii. After decapping in yeast an enzyme (from, the XRNJ gene) aggressively degrades the m. RNA from the 5’end by 5’-3’exonuclease activity. iv. Degradation still occurs in dcpl mutants, indicating that other m. RNA degradation pathways exist. d. Deadenylation-independent m. RNA decay includes two types of pathways: i. Direct decapping, exposing the 5’end to 5’-3’exonucleases. ii. Internal cleavage of the m. RNA, and then degradation of the fragments. 3. Mammalian m. RNA degradation mechanisms are less clear than those of yeast. Both deadenylation-dependent and deadenylation-independent pathways are found in 台大農藝系 遺傳學 601 20000 mammals. 40
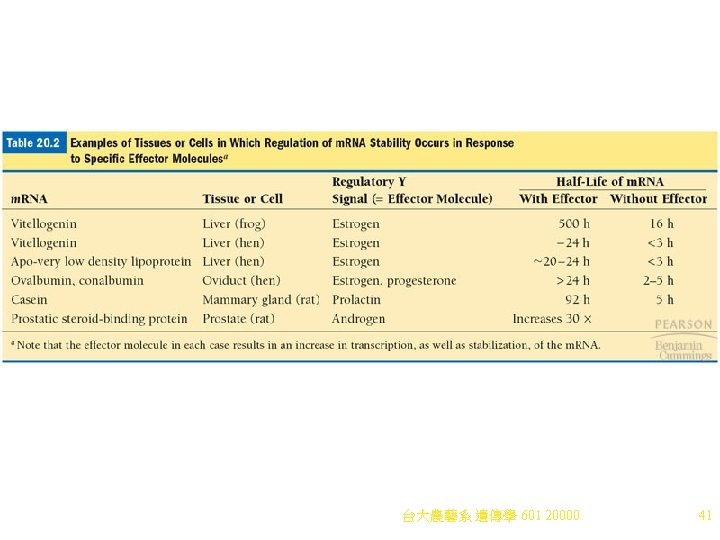
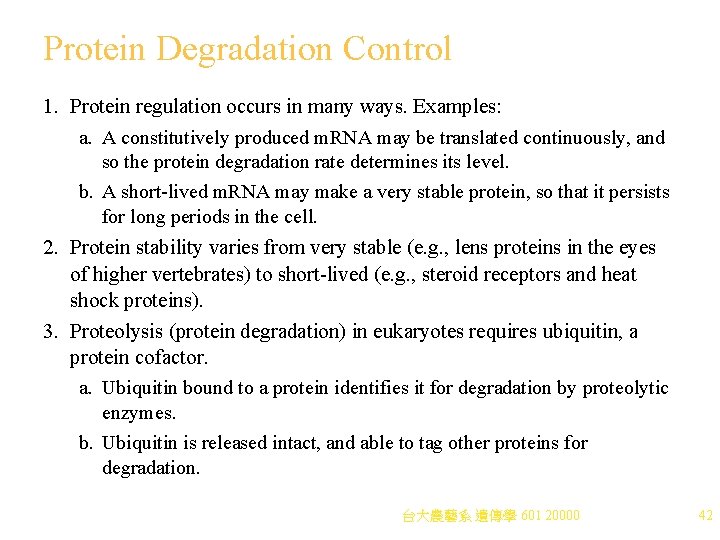
Protein Degradation Control 1. Protein regulation occurs in many ways. Examples: a. A constitutively produced m. RNA may be translated continuously, and so the protein degradation rate determines its level. b. A short-lived m. RNA may make a very stable protein, so that it persists for long periods in the cell. 2. Protein stability varies from very stable (e. g. , lens proteins in the eyes of higher vertebrates) to short-lived (e. g. , steroid receptors and heat shock proteins). 3. Proteolysis (protein degradation) in eukaryotes requires ubiquitin, a protein cofactor. a. Ubiquitin bound to a protein identifies it for degradation by proteolytic enzymes. b. Ubiquitin is released intact, and able to tag other proteins for degradation. 台大農藝系 遺傳學 601 20000 42
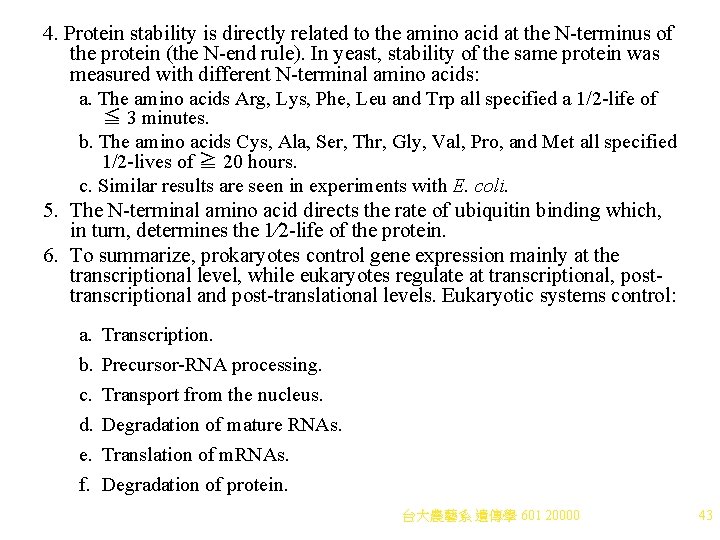
4. Protein stability is directly related to the amino acid at the N-terminus of the protein (the N-end rule). In yeast, stability of the same protein was measured with different N-terminal amino acids: a. The amino acids Arg, Lys, Phe, Leu and Trp all specified a 1/2 -life of ≦ 3 minutes. b. The amino acids Cys, Ala, Ser, Thr, Gly, Val, Pro, and Met all specified 1/2 -lives of ≧ 20 hours. c. Similar results are seen in experiments with E. coli. 5. The N-terminal amino acid directs the rate of ubiquitin binding which, in turn, determines the 1⁄2 -life of the protein. 6. To summarize, prokaryotes control gene expression mainly at the transcriptional level, while eukaryotes regulate at transcriptional, posttranscriptional and post-translational levels. Eukaryotic systems control: a. b. c. d. e. f. Transcription. Precursor-RNA processing. Transport from the nucleus. Degradation of mature RNAs. Translation of m. RNAs. Degradation of protein. 台大農藝系 遺傳學 601 20000 43
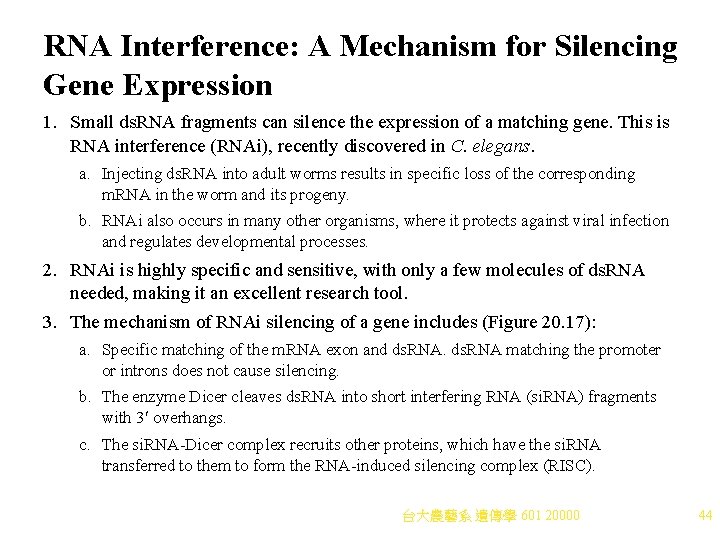
RNA Interference: A Mechanism for Silencing Gene Expression 1. Small ds. RNA fragments can silence the expression of a matching gene. This is RNA interference (RNAi), recently discovered in C. elegans. a. Injecting ds. RNA into adult worms results in specific loss of the corresponding m. RNA in the worm and its progeny. b. RNAi also occurs in many other organisms, where it protects against viral infection and regulates developmental processes. 2. RNAi is highly specific and sensitive, with only a few molecules of ds. RNA needed, making it an excellent research tool. 3. The mechanism of RNAi silencing of a gene includes (Figure 20. 17): a. Specific matching of the m. RNA exon and ds. RNA matching the promoter or introns does not cause silencing. b. The enzyme Dicer cleaves ds. RNA into short interfering RNA (si. RNA) fragments with 3¢ overhangs. c. The si. RNA-Dicer complex recruits other proteins, which have the si. RNA transferred to them to form the RNA-induced silencing complex (RISC). 台大農藝系 遺傳學 601 20000 44
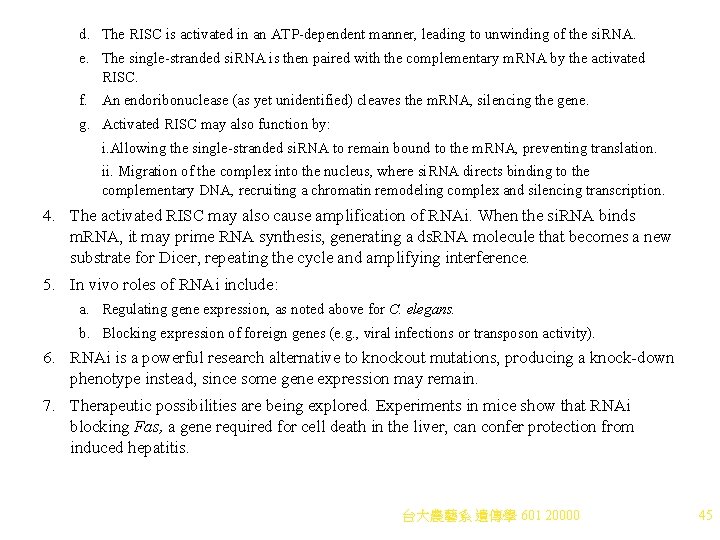
d. The RISC is activated in an ATP-dependent manner, leading to unwinding of the si. RNA. e. The single-stranded si. RNA is then paired with the complementary m. RNA by the activated RISC. f. An endoribonuclease (as yet unidentified) cleaves the m. RNA, silencing the gene. g. Activated RISC may also function by: i. Allowing the single-stranded si. RNA to remain bound to the m. RNA, preventing translation. ii. Migration of the complex into the nucleus, where si. RNA directs binding to the complementary DNA, recruiting a chromatin remodeling complex and silencing transcription. 4. The activated RISC may also cause amplification of RNAi. When the si. RNA binds m. RNA, it may prime RNA synthesis, generating a ds. RNA molecule that becomes a new substrate for Dicer, repeating the cycle and amplifying interference. 5. In vivo roles of RNAi include: a. Regulating gene expression, as noted above for C. elegans. b. Blocking expression of foreign genes (e. g. , viral infections or transposon activity). 6. RNAi is a powerful research alternative to knockout mutations, producing a knock-down phenotype instead, since some gene expression may remain. 7. Therapeutic possibilities are being explored. Experiments in mice show that RNAi blocking Fas, a gene required for cell death in the liver, can confer protection from induced hepatitis. 台大農藝系 遺傳學 601 20000 45
What is a covalent bond simple definition
Giant molecular structure vs simple molecular structure
Giant molecular structure vs simple molecular structure
Democritus atomic model diagram
Virtual circuit tables
Cognitive approach vs behavioral approach
Shower approach marketing
Approach approach conflict
Cognitive approach vs behavioral approach
What is research
Traditional approach to system development
Tony wagner's seven survival skills
What is the horror genre analyzing the text answers
Bryant's traction
Iso 9001 vs cmmi
Russell etymology
The plot against people
Russell chuderewicz
On the hr diagram red supergiants like betelgeuse lie
Fph e portfolio
What is ai?
The hertzsprung russell diagram shows
Fundador de los testigos de jehova charles taze russell
Barber paradox
Russell's paradox
Bertrand russell on denoting
Celia russell
Paradosso di russell soluzione
How to avoid foolish opinions short summary
H-r diagram worksheet
Russell wallace day
Adeline russell
Plaque index scoring criteria
The trailside killer
James clayton lawson
Russell vs copleston
Copleston and russell debate summary
Bulimia deutsch
Taylor russel tables
John russell houston
What is the horror genre by sharon russell
Snowfall james
Russel and norvig
Richard russell rick riordan jr
Lynne russell
One line prayer quotes