Frequency Chirp measurement Technique using chromatic dispersion David
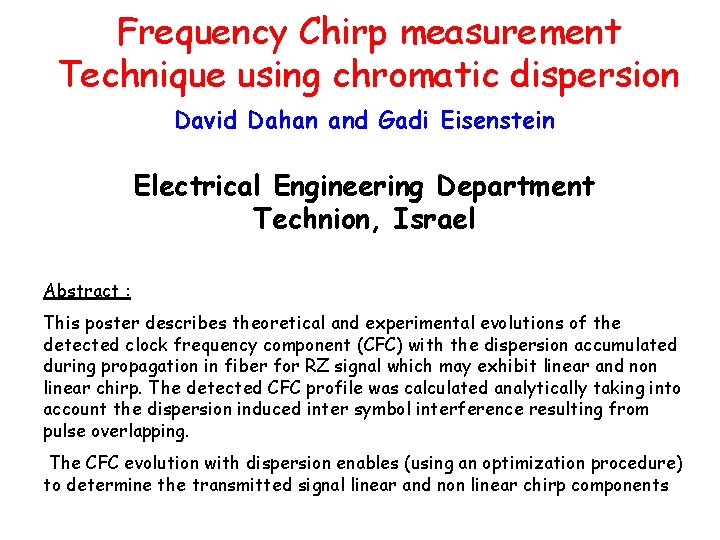
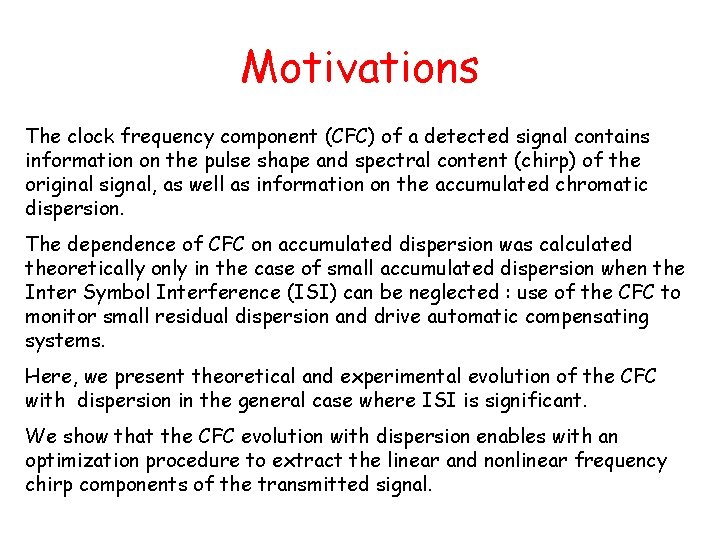
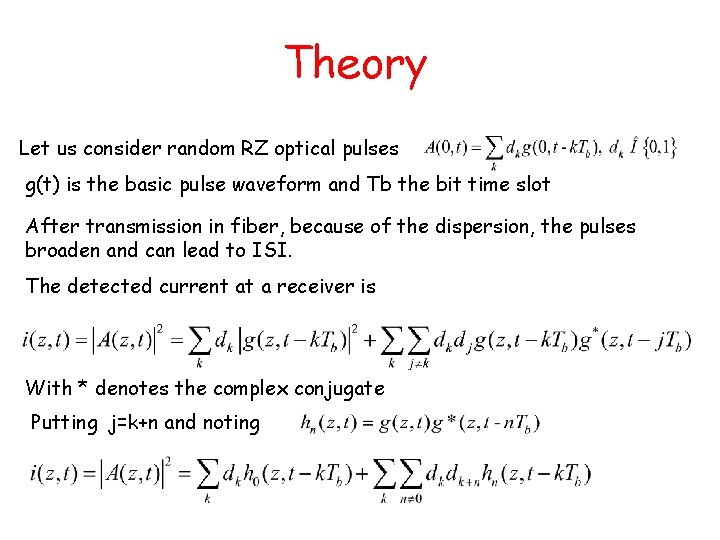
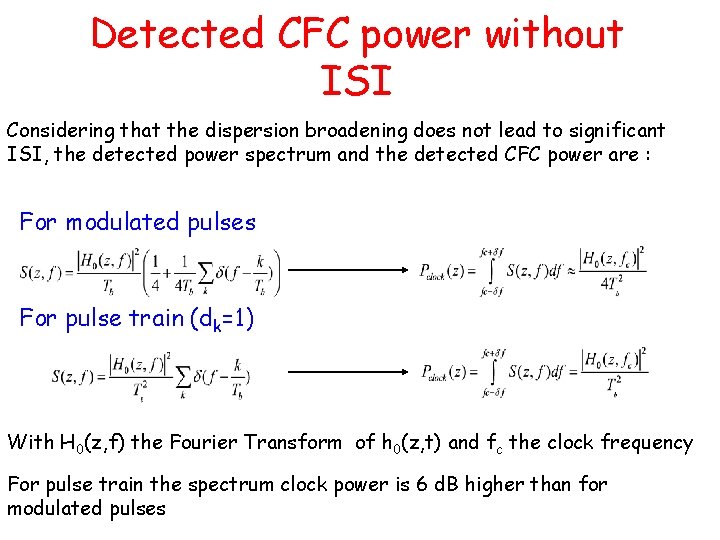
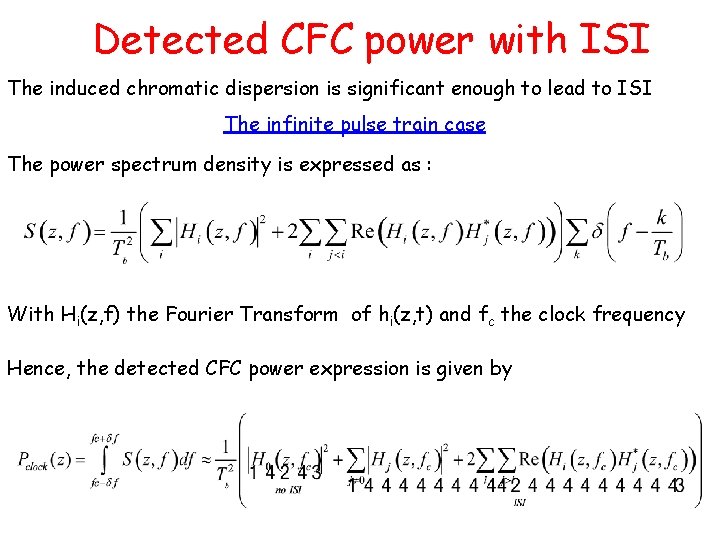
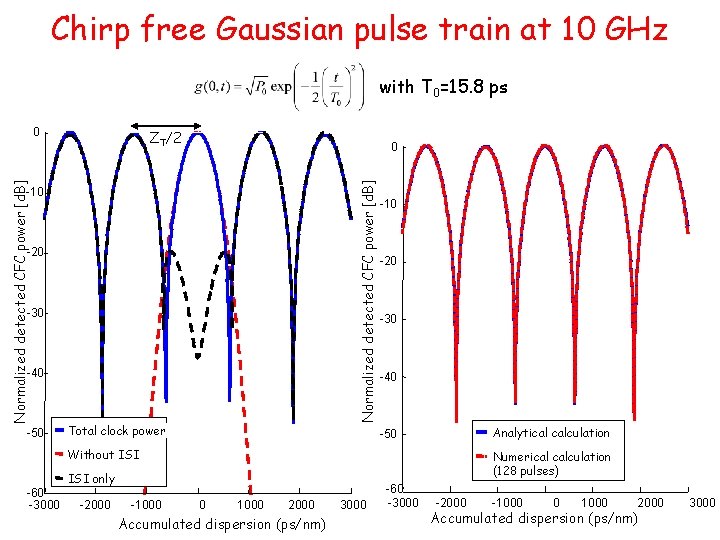
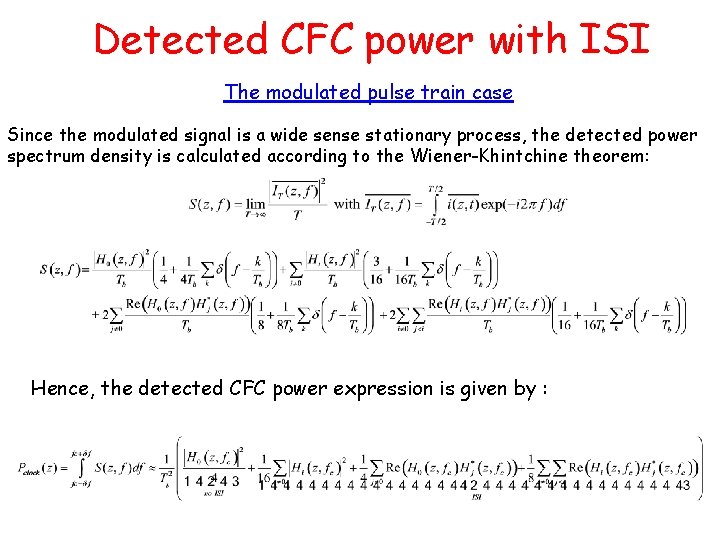
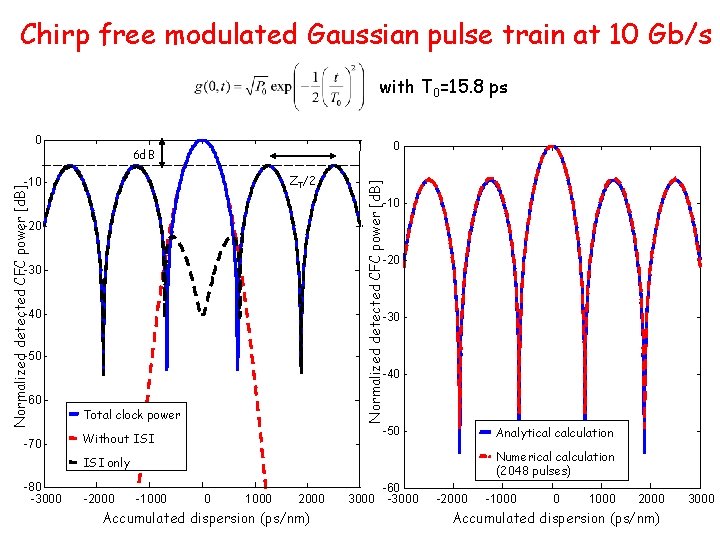
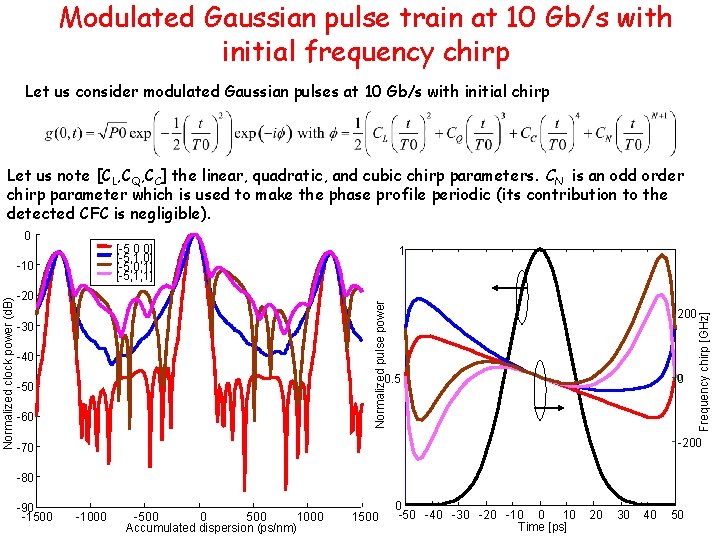
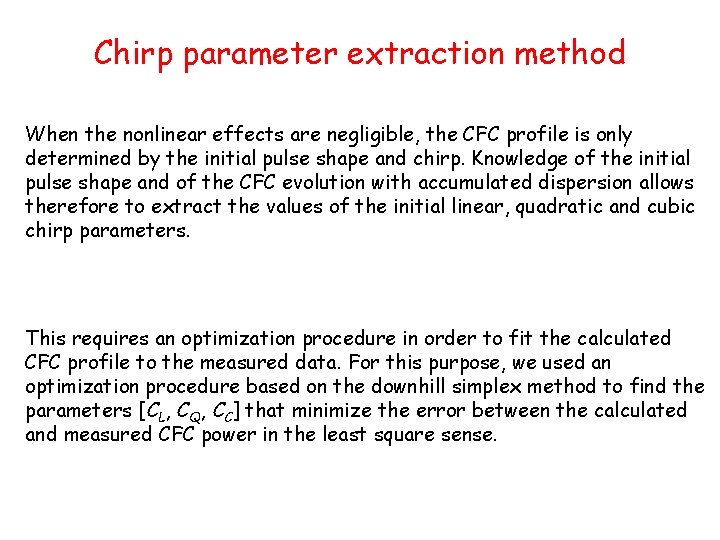
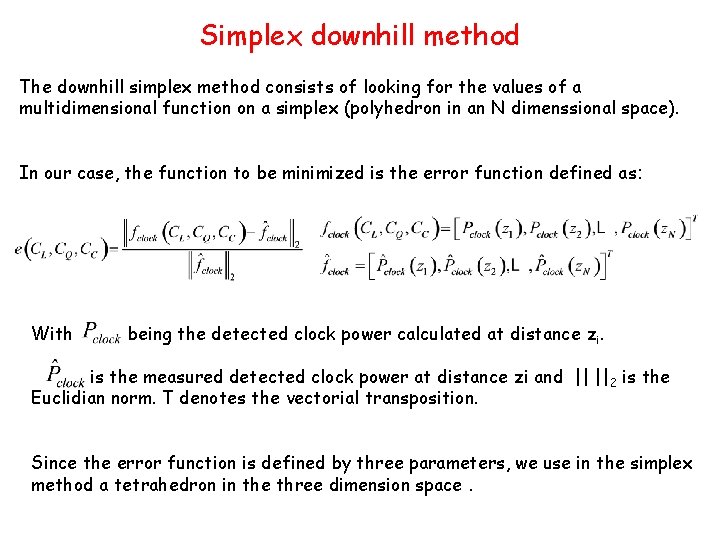
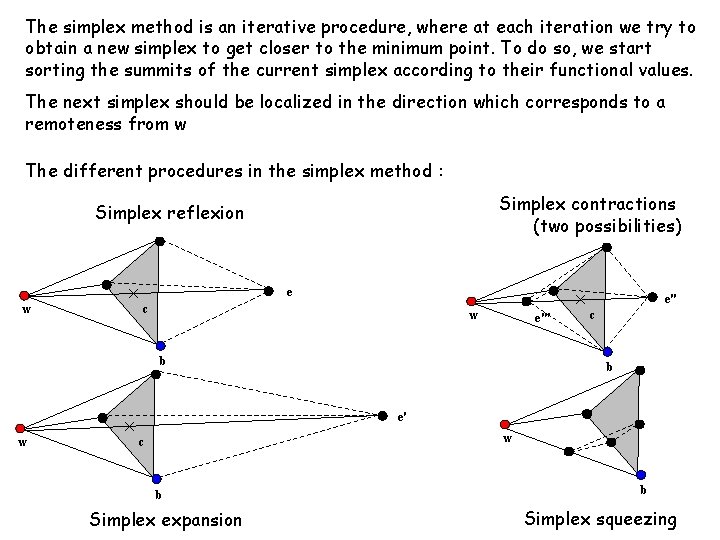
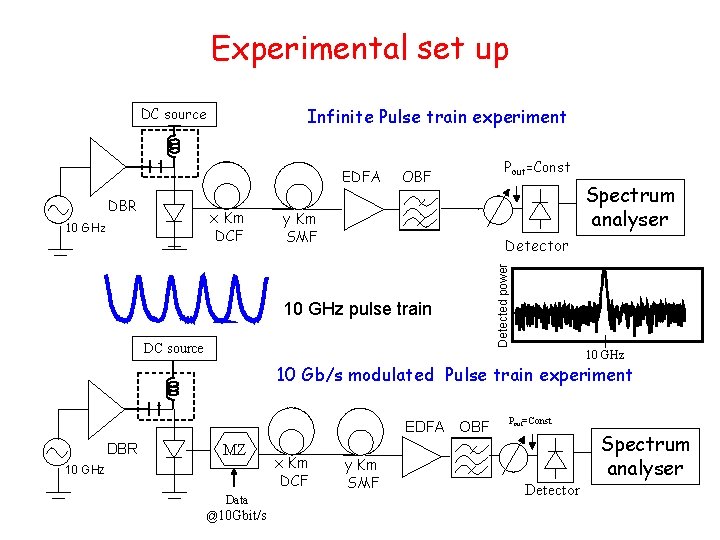
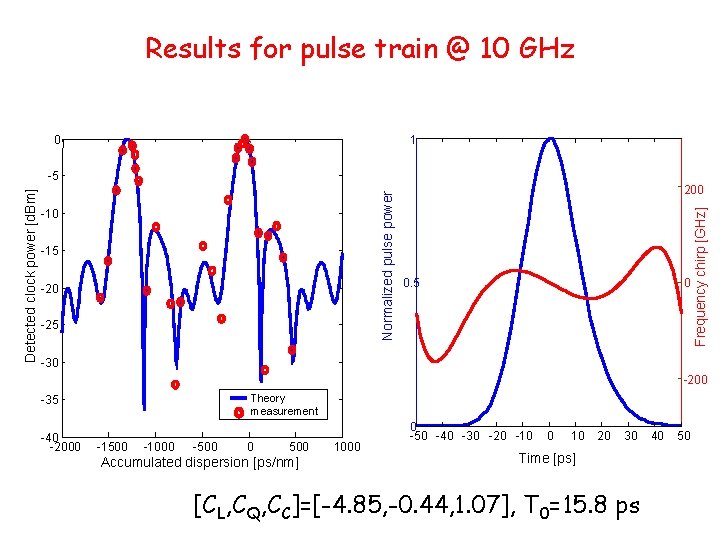
![Results for Modulated pulses @ 10 Gbit/s 200 -50 -60 Frequency chirp [GHz] -40 Results for Modulated pulses @ 10 Gbit/s 200 -50 -60 Frequency chirp [GHz] -40](https://slidetodoc.com/presentation_image/31be3493e9723a92d669c61c863eea23/image-15.jpg)
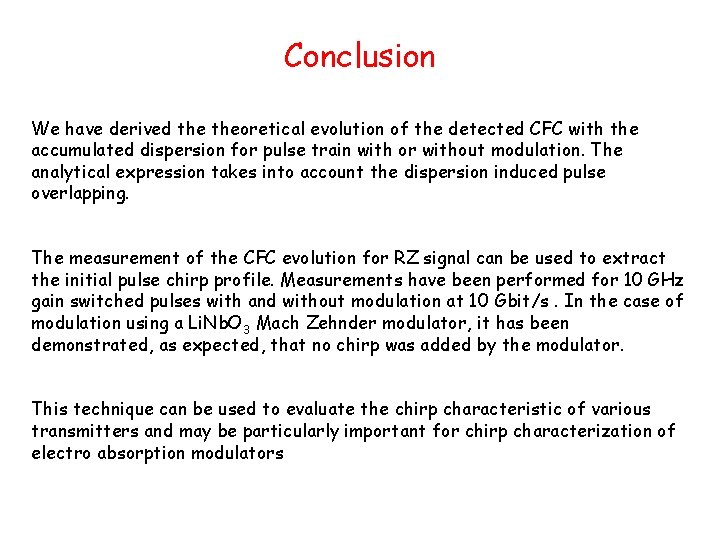
- Slides: 16
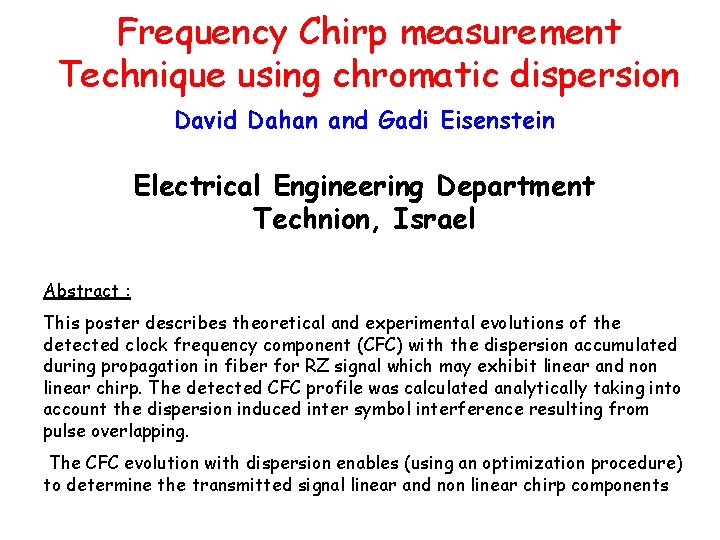
Frequency Chirp measurement Technique using chromatic dispersion David Dahan and Gadi Eisenstein Electrical Engineering Department Technion, Israel Abstract : This poster describes theoretical and experimental evolutions of the detected clock frequency component (CFC) with the dispersion accumulated during propagation in fiber for RZ signal which may exhibit linear and non linear chirp. The detected CFC profile was calculated analytically taking into account the dispersion induced inter symbol interference resulting from pulse overlapping. The CFC evolution with dispersion enables (using an optimization procedure) to determine the transmitted signal linear and non linear chirp components
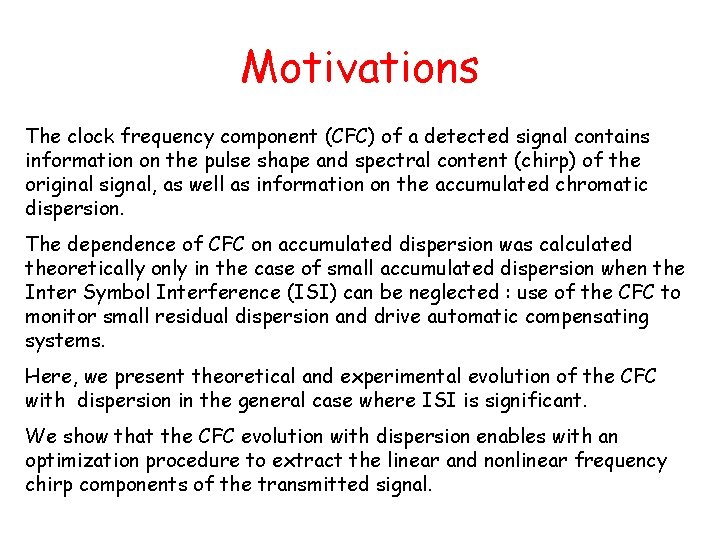
Motivations The clock frequency component (CFC) of a detected signal contains information on the pulse shape and spectral content (chirp) of the original signal, as well as information on the accumulated chromatic dispersion. The dependence of CFC on accumulated dispersion was calculated theoretically only in the case of small accumulated dispersion when the Inter Symbol Interference (ISI) can be neglected : use of the CFC to monitor small residual dispersion and drive automatic compensating systems. Here, we present theoretical and experimental evolution of the CFC with dispersion in the general case where ISI is significant. We show that the CFC evolution with dispersion enables with an optimization procedure to extract the linear and nonlinear frequency chirp components of the transmitted signal.
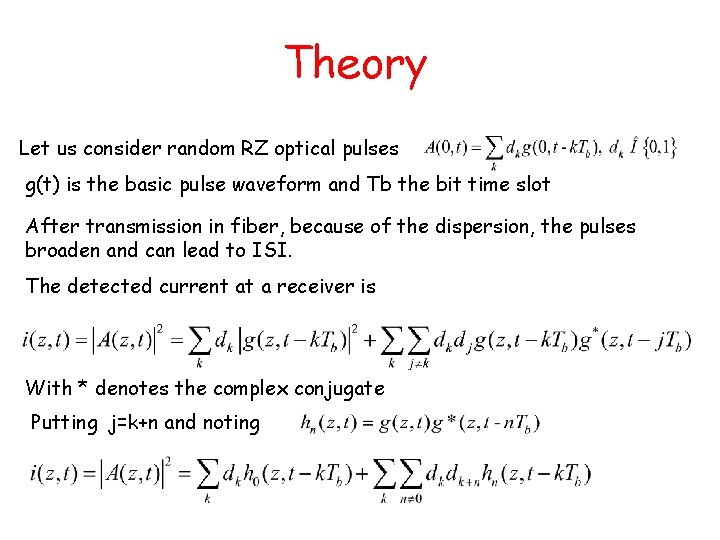
Theory Let us consider random RZ optical pulses g(t) is the basic pulse waveform and Tb the bit time slot After transmission in fiber, because of the dispersion, the pulses broaden and can lead to ISI. The detected current at a receiver is With * denotes the complex conjugate Putting j=k+n and noting
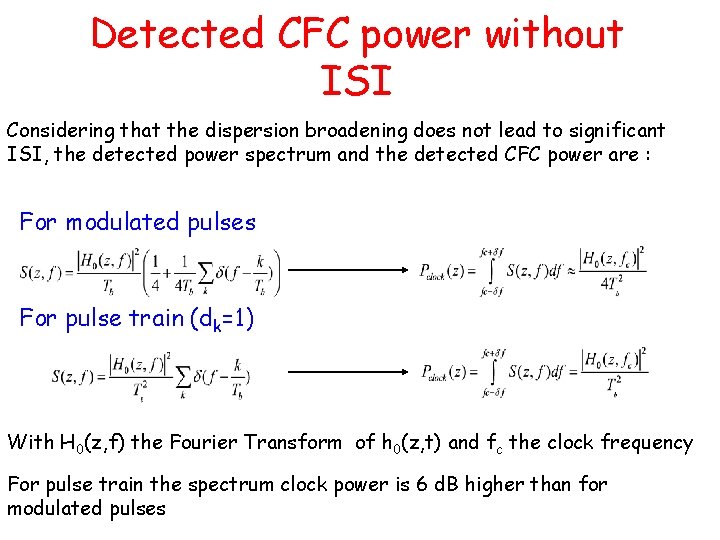
Detected CFC power without ISI Considering that the dispersion broadening does not lead to significant ISI, the detected power spectrum and the detected CFC power are : For modulated pulses For pulse train (dk=1) With H 0(z, f) the Fourier Transform of h 0(z, t) and fc the clock frequency For pulse train the spectrum clock power is 6 d. B higher than for modulated pulses
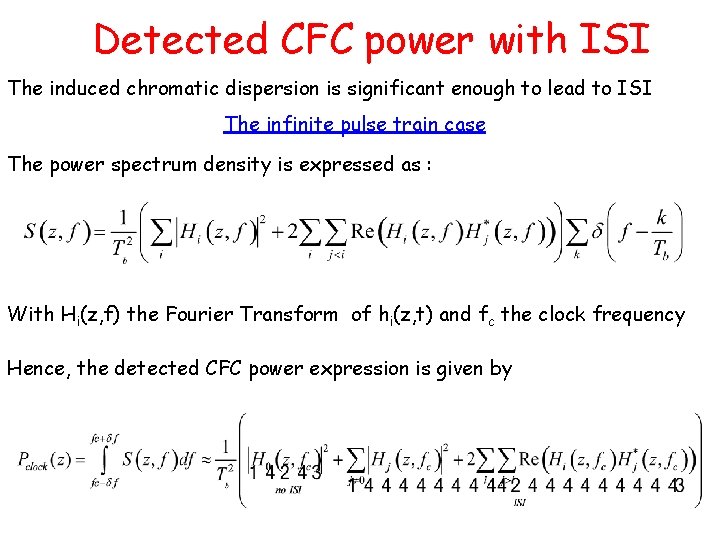
Detected CFC power with ISI The induced chromatic dispersion is significant enough to lead to ISI The infinite pulse train case The power spectrum density is expressed as : With Hi(z, f) the Fourier Transform of hi(z, t) and fc the clock frequency Hence, the detected CFC power expression is given by
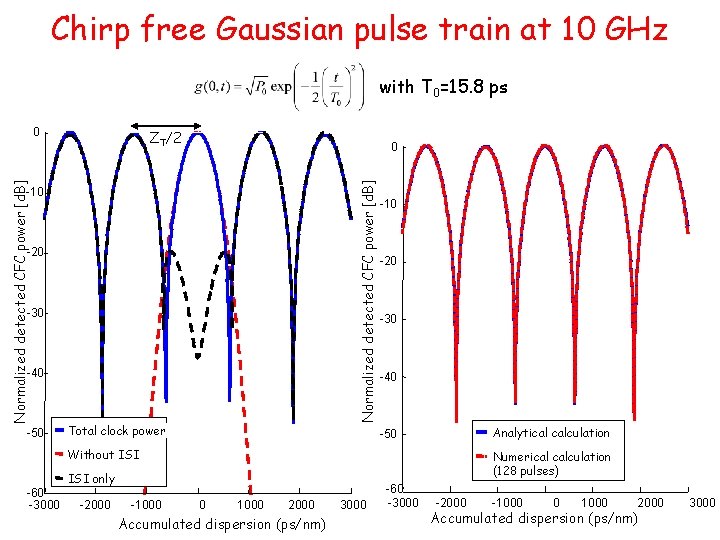
Chirp free Gaussian pulse train at 10 GHz with T 0=15. 8 ps ZT/2 0 Normalized detected CFC power [d. B] 0 -10 -20 -30 -40 -50 Total clock power -10 -20 -30 -40 Analytical calculation -50 Without ISI Numerical calculation (128 pulses) ISI only -60 -3000 -2000 -1000 0 1000 2000 Accumulated dispersion (ps/nm) 3000
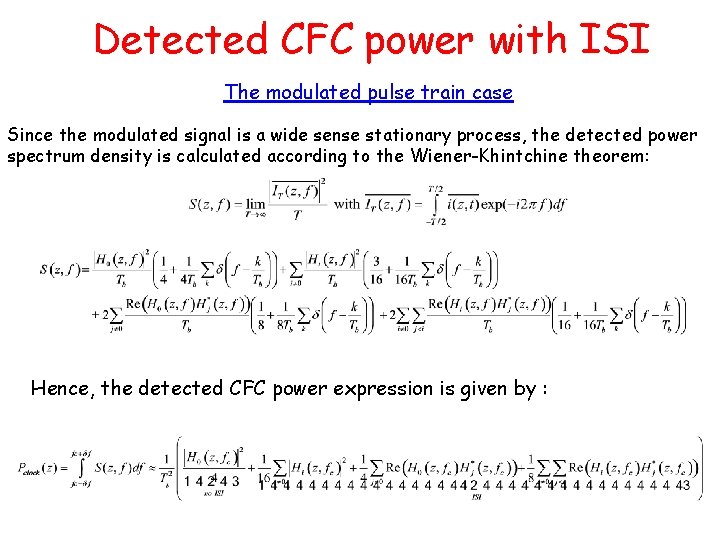
Detected CFC power with ISI The modulated pulse train case Since the modulated signal is a wide sense stationary process, the detected power spectrum density is calculated according to the Wiener-Khintchine theorem: Hence, the detected CFC power expression is given by :
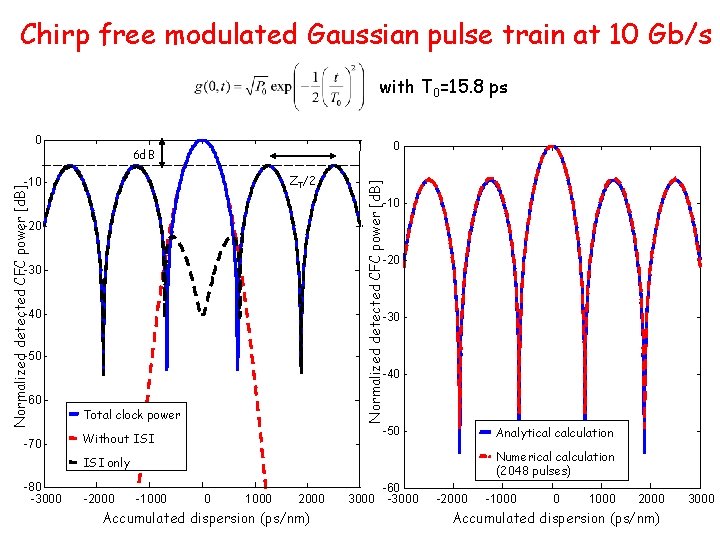
Chirp free modulated Gaussian pulse train at 10 Gb/s with T 0=15. 8 ps 0 0 ZT/2 Normalized clock power (d. B) Normalized detected CFC power [d. B] -10 -20 -30 -40 -30 -50 -60 -70 Normalized detected CFC power [d. B] 6 d. B -40 Total clock power Analytical calculation -50 Without ISI Numerical calculation (2048 pulses) ISI only -80 -3000 -2000 -1000 0 1000 2000 Accumulated dispersion (ps/nm) 3000 -60 -3000 -2000 -1000 0 1000 2000 Accumulated dispersion (ps/nm) 3000
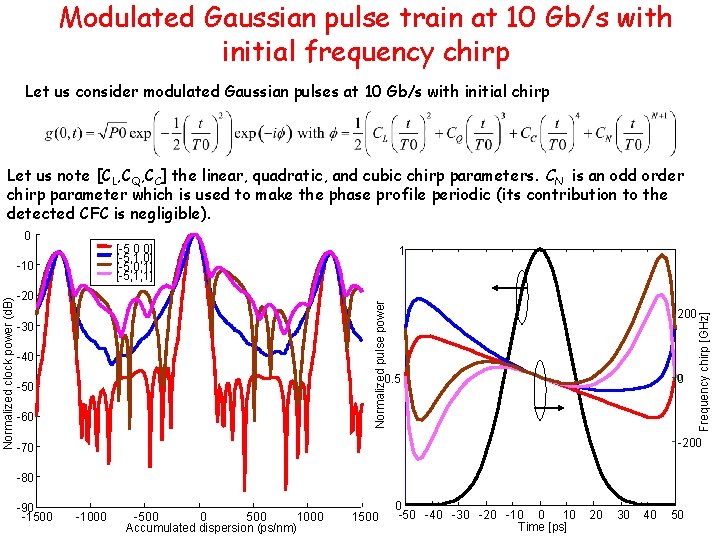
Modulated Gaussian pulse train at 10 Gb/s with initial frequency chirp Let us consider modulated Gaussian pulses at 10 Gb/s with initial chirp Let us note [CL, CQ, CC] the linear, quadratic, and cubic chirp parameters. CN is an odd order chirp parameter which is used to make the phase profile periodic (its contribution to the detected CFC is negligible). 0 [-5 0 0] [-5, 1, 0] [-5, 0, 1] [-5, 1, 1] -30 -40 200 0 0. 5 -50 -60 -200 -70 -80 -90 -1500 Frequency chirp [GHz] -20 Normalized pulse power Normalized clock power (d. B) -10 1 -1000 -500 0 500 1000 Accumulated dispersion (ps/nm) 1500 0 -50 -40 -30 -20 -10 0 10 Time [ps] 20 30 40 50
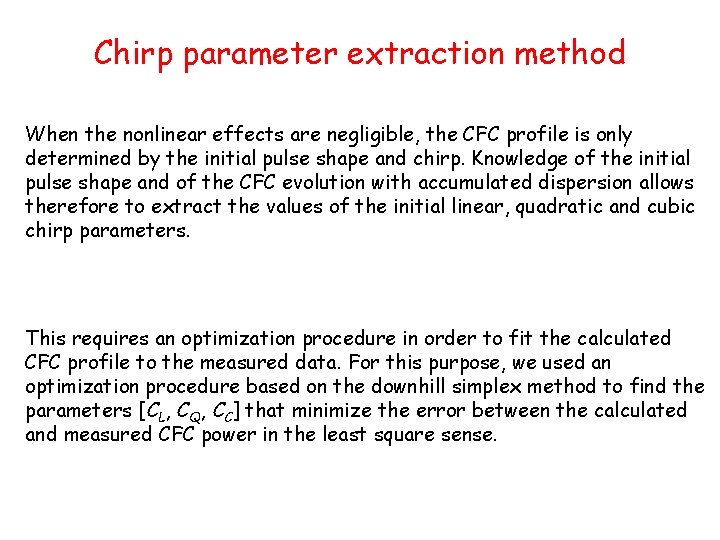
Chirp parameter extraction method When the nonlinear effects are negligible, the CFC profile is only determined by the initial pulse shape and chirp. Knowledge of the initial pulse shape and of the CFC evolution with accumulated dispersion allows therefore to extract the values of the initial linear, quadratic and cubic chirp parameters. This requires an optimization procedure in order to fit the calculated CFC profile to the measured data. For this purpose, we used an optimization procedure based on the downhill simplex method to find the parameters [CL, CQ, CC] that minimize the error between the calculated and measured CFC power in the least square sense.
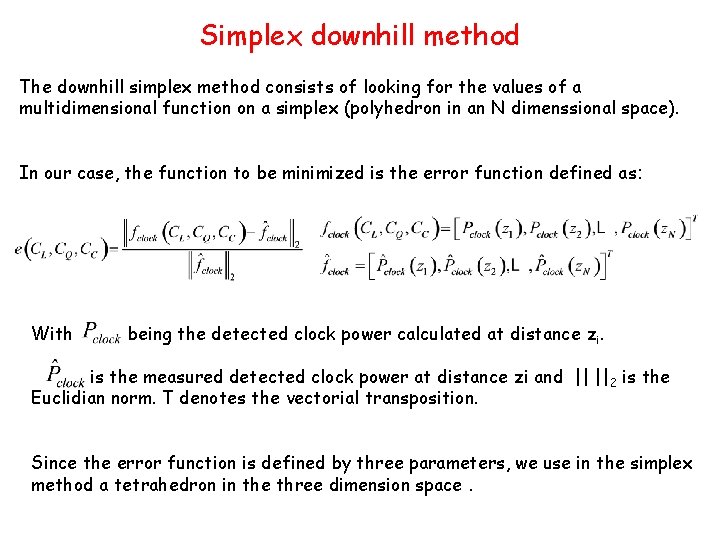
Simplex downhill method The downhill simplex method consists of looking for the values of a multidimensional function on a simplex (polyhedron in an N dimenssional space). In our case, the function to be minimized is the error function defined as: With being the detected clock power calculated at distance zi. is the measured detected clock power at distance zi and || ||2 is the Euclidian norm. T denotes the vectorial transposition. Since the error function is defined by three parameters, we use in the simplex method a tetrahedron in the three dimension space.
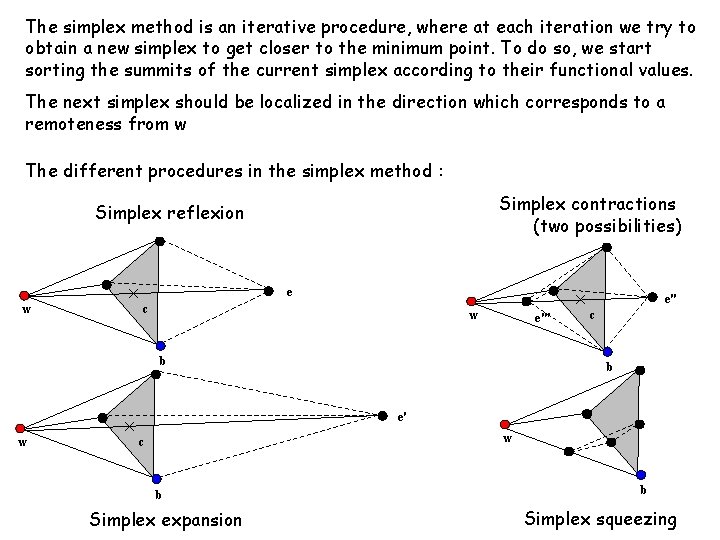
The simplex method is an iterative procedure, where at each iteration we try to obtain a new simplex to get closer to the minimum point. To do so, we start sorting the summits of the current simplex according to their functional values. The next simplex should be localized in the direction which corresponds to a remoteness from w The different procedures in the simplex method : Simplex contractions (two possibilities) Simplex reflexion e w e" c w e'" b c b e' w w c b Simplex expansion b Simplex squeezing
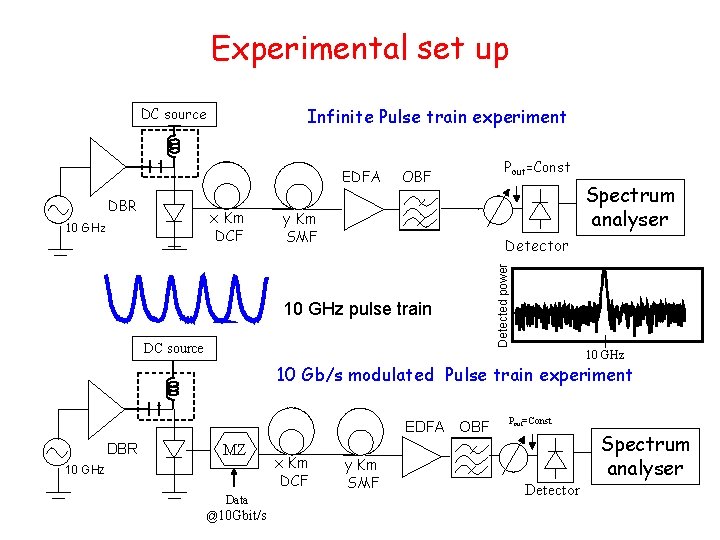
Experimental set up Infinite Pulse train experiment DBR EDFA x Km DCF 10 GHz OBF y Km SMF Pout=Const Spectrum analyser Detector 10 GHz pulse train DC source Detected power DC source 10 GHz 10 Gb/s modulated Pulse train experiment EDFA OBF DBR MZ 10 GHz Data @10 Gbit/s x Km DCF y Km SMF Pout=Const Detector Spectrum analyser
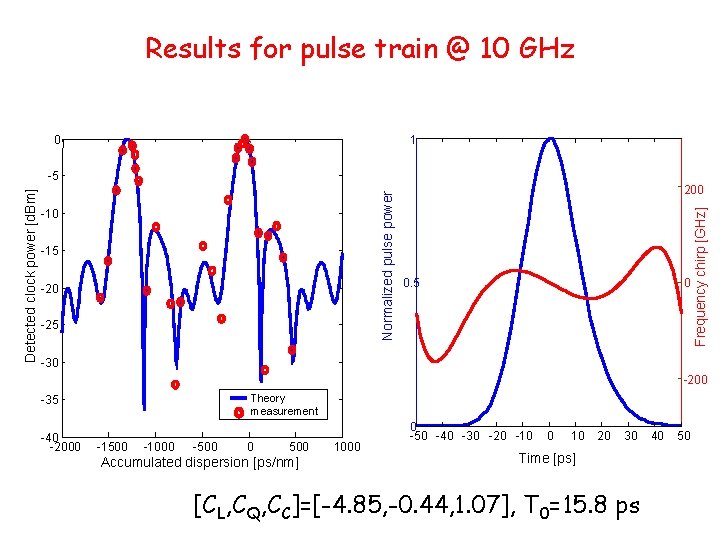
Results for pulse train @ 10 GHz 1 Normalized pulse power Detected clock power [d. Bm] -5 -10 -15 -20 -25 200 0. 5 0 Frequency chirp [GHz] 0 -30 -200 Theory measurement -35 -40 -2000 -1500 -1000 -500 0 500 Accumulated dispersion [ps/nm] 1000 0 -50 -40 -30 -20 -10 0 10 20 30 Time [ps] [CL, CQ, CC]=[-4. 85, -0. 44, 1. 07], T 0=15. 8 ps 40 50
![Results for Modulated pulses 10 Gbits 200 50 60 Frequency chirp GHz 40 Results for Modulated pulses @ 10 Gbit/s 200 -50 -60 Frequency chirp [GHz] -40](https://slidetodoc.com/presentation_image/31be3493e9723a92d669c61c863eea23/image-15.jpg)
Results for Modulated pulses @ 10 Gbit/s 200 -50 -60 Frequency chirp [GHz] -40 1 Theory measurement Normalized pulse power Detected clock power [d. Bm] -30 0. 5 -70 0 -200 -80 -90 -2000 -1500 -1000 -500 0 500 Accumulated dispersion [ps/nm] 1000 0 -50 -40 -30 -20 -10 0 10 20 30 40 Time [ps] [CL, CQ, CC]=[-4. 82, -0. 41, 1. 08], T 0=15. 8 ps As expected, the Mach Zendher doesn’t add significant chirp (symmetric configuration) 50
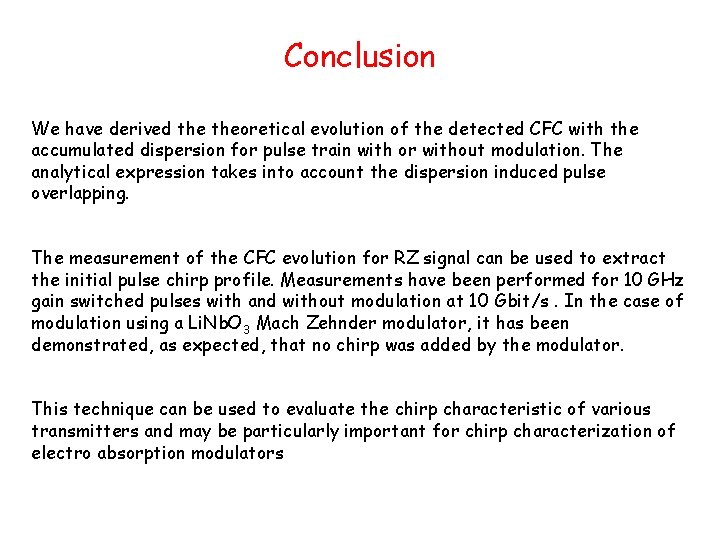
Conclusion We have derived theoretical evolution of the detected CFC with the accumulated dispersion for pulse train with or without modulation. The analytical expression takes into account the dispersion induced pulse overlapping. The measurement of the CFC evolution for RZ signal can be used to extract the initial pulse chirp profile. Measurements have been performed for 10 GHz gain switched pulses with and without modulation at 10 Gbit/s. In the case of modulation using a Li. Nb. O 3 Mach Zehnder modulator, it has been demonstrated, as expected, that no chirp was added by the modulator. This technique can be used to evaluate the chirp characteristic of various transmitters and may be particularly important for chirp characterization of electro absorption modulators
Chromatic dispersion analyzer
Chromatic dispersion management
Dispersion phase and dispersion medium
Protective colloid
Signal distortion in optical fiber
Chirp & oink
Chirp vaccine
Aracebo telescope
What is chromatic number
What will be the chromatic number of the following graph? *
Chromatic number
Bob chromatic scale
Intensity to color transformation
Means one color
Chromatic light
Chromatic number
Chromatic number