Field Emission Modeling M Santana Leitner Field Emission
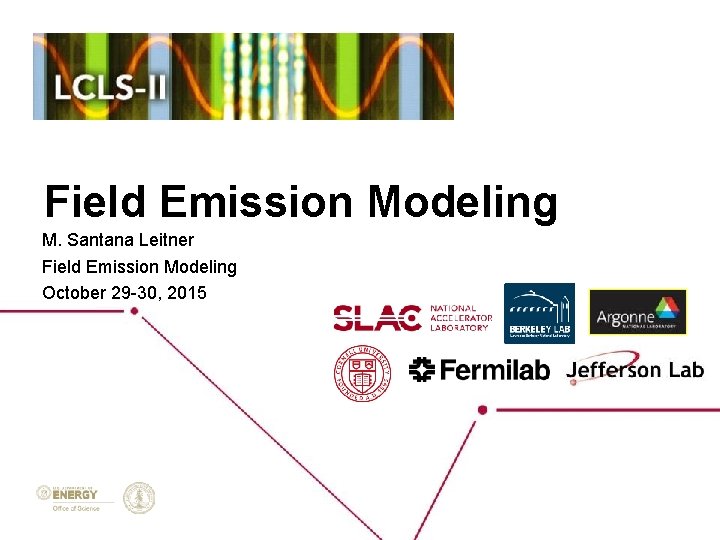
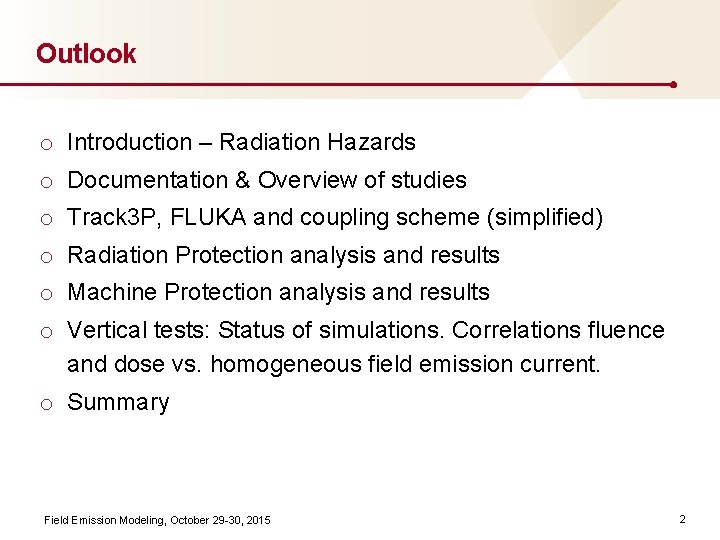
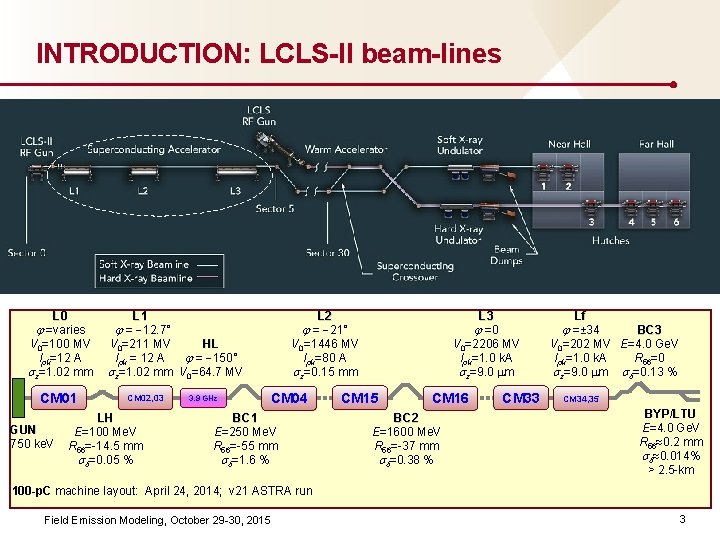
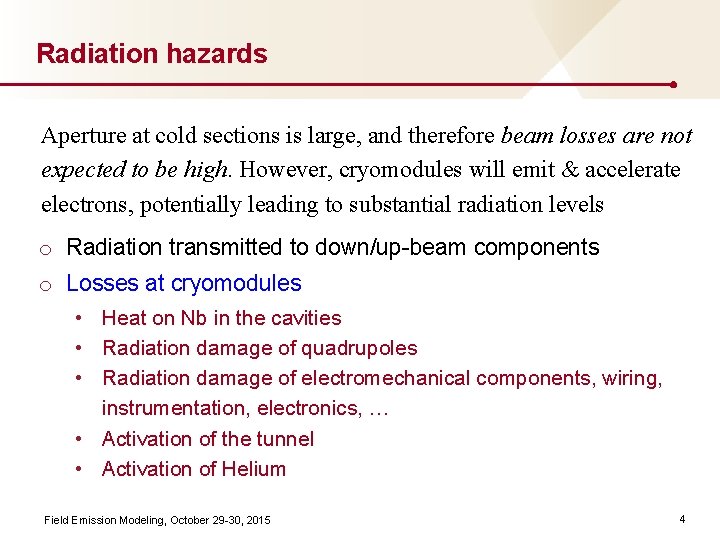
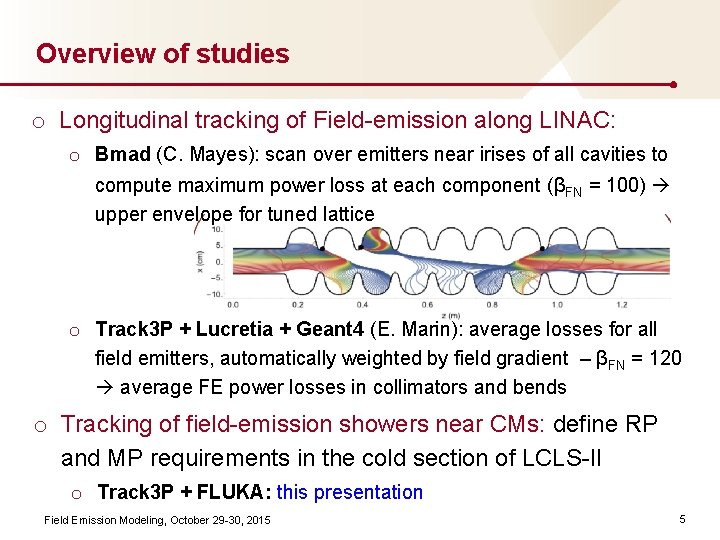
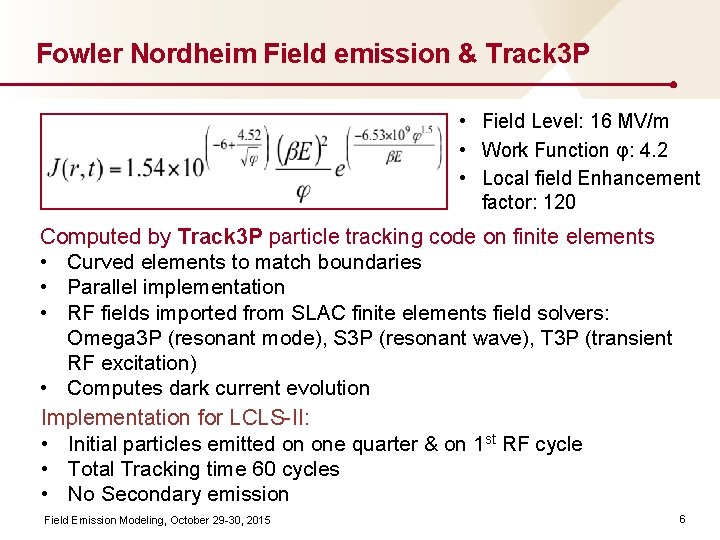
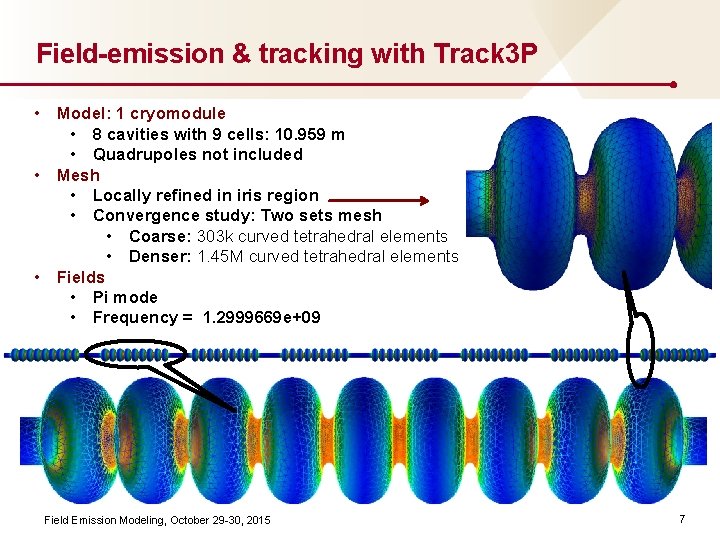
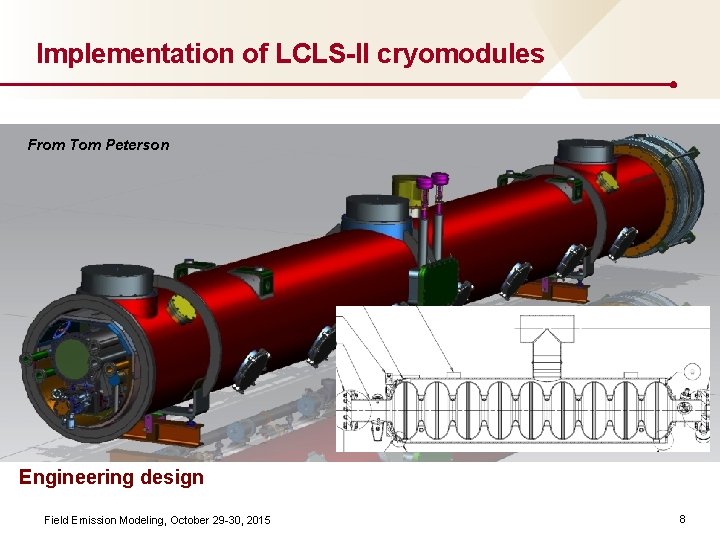
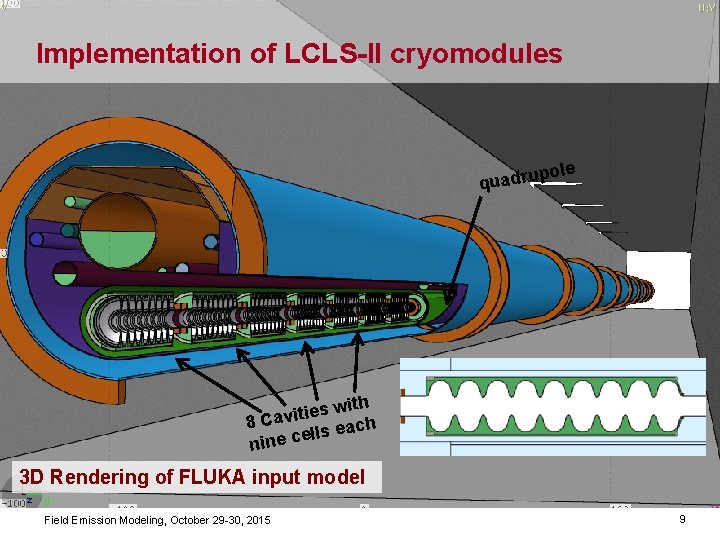
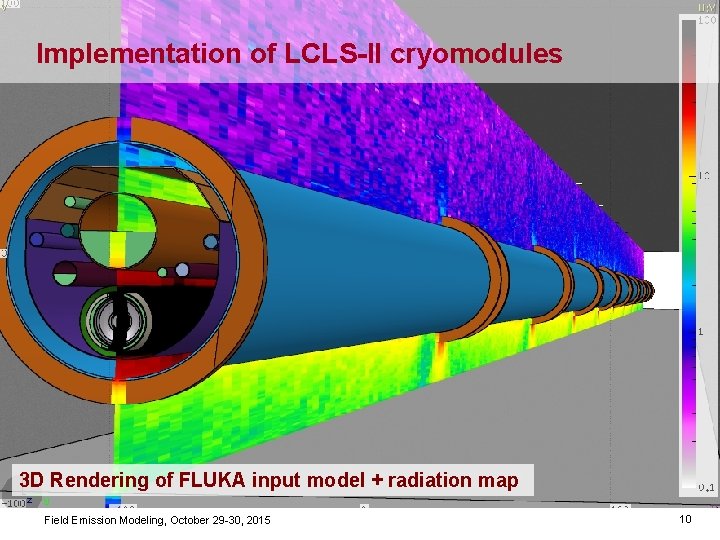
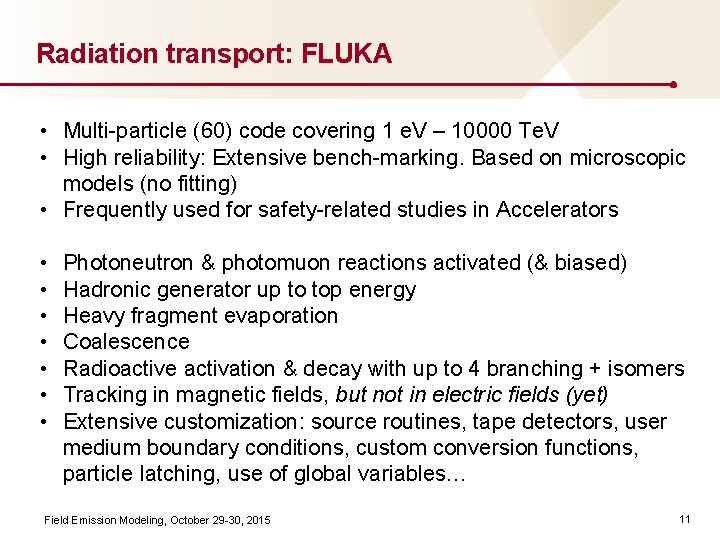
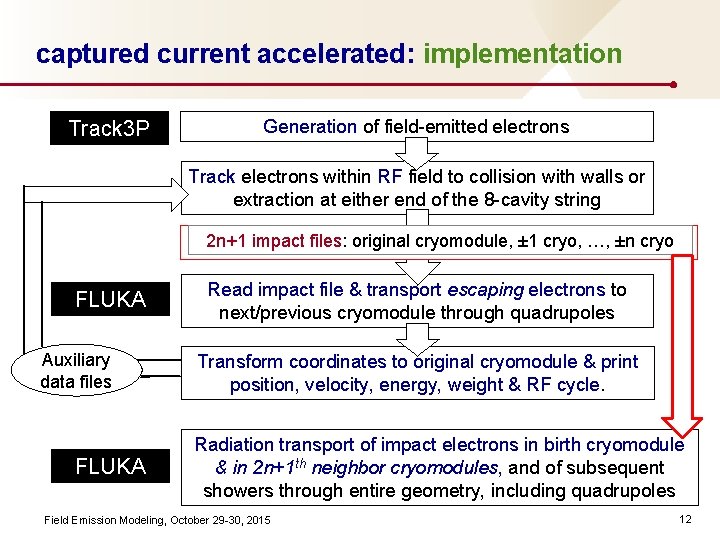
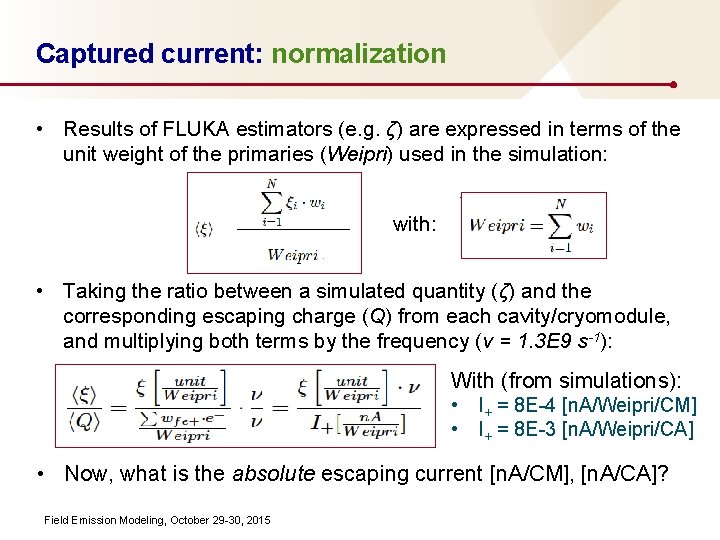
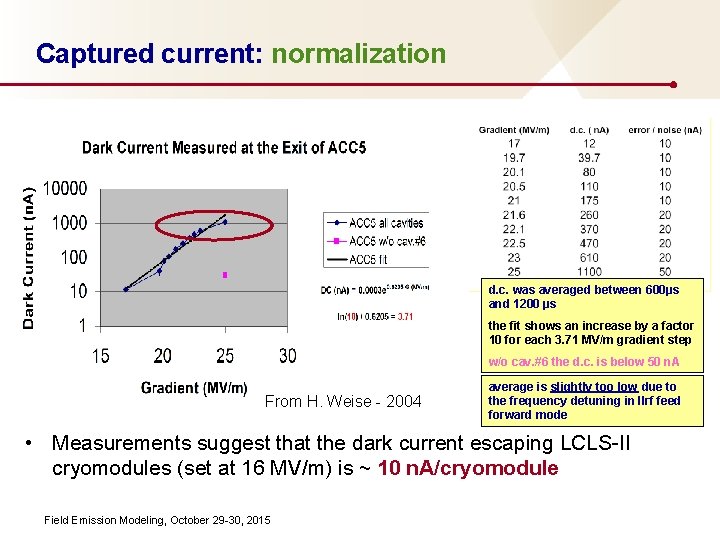
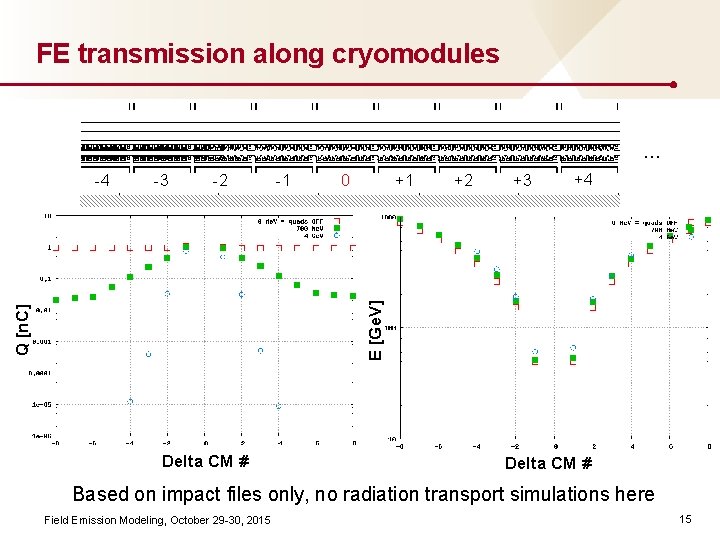
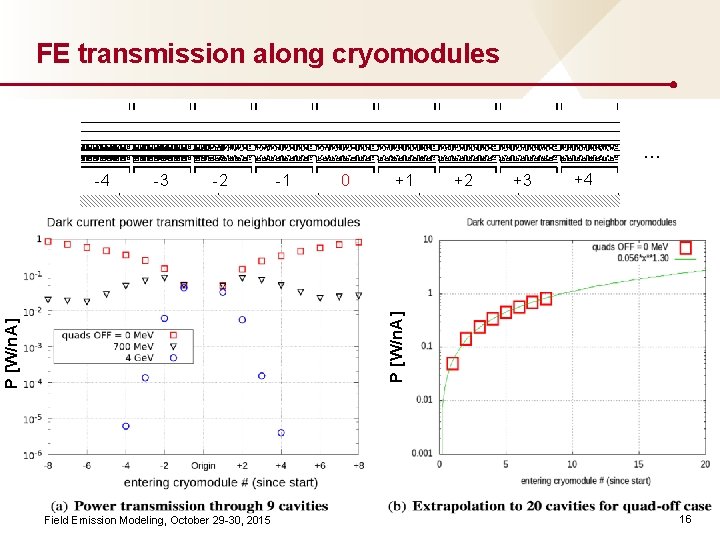
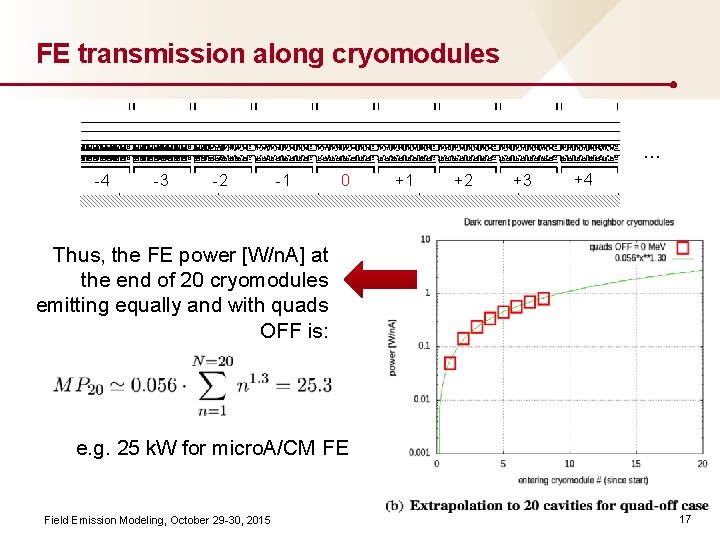
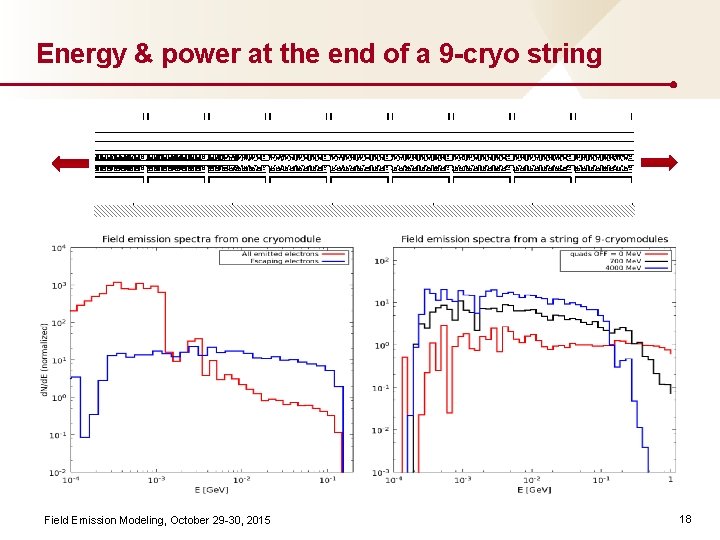
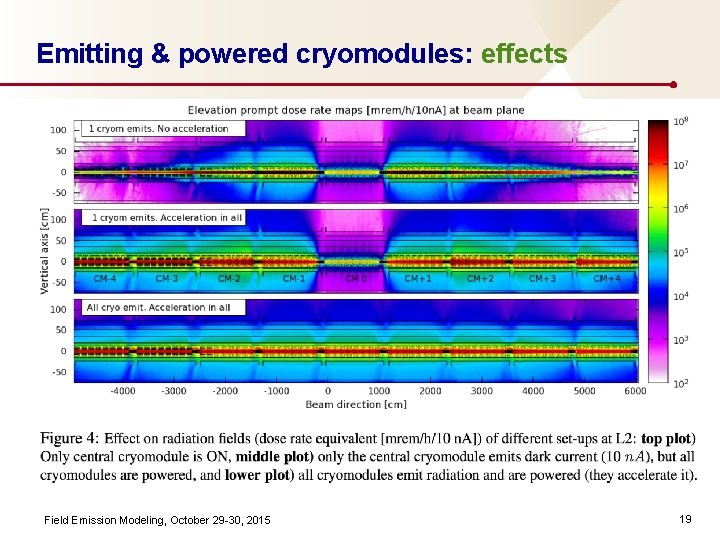
![Vertical [cm] [Gy/300 d/n. A] Vertical [cm] Effect of quad settings on radiation fields Vertical [cm] [Gy/300 d/n. A] Vertical [cm] Effect of quad settings on radiation fields](https://slidetodoc.com/presentation_image_h2/0991c3995f7973166186f6a6d2d69aef/image-20.jpg)
![0 Me. V Vertical [cm] Settings at 700 Me. V are the 700 worst 0 Me. V Vertical [cm] Settings at 700 Me. V are the 700 worst](https://slidetodoc.com/presentation_image_h2/0991c3995f7973166186f6a6d2d69aef/image-21.jpg)
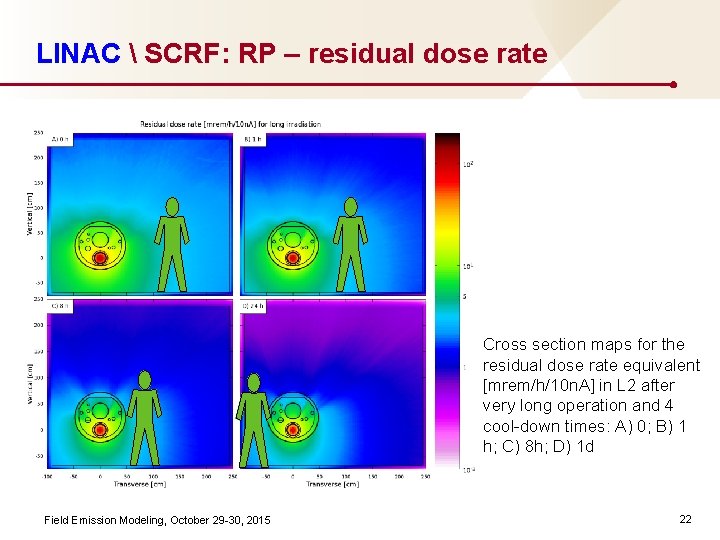
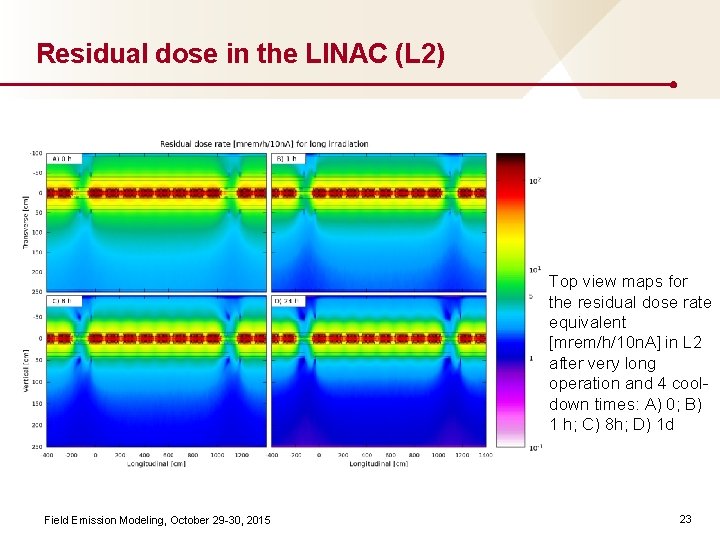
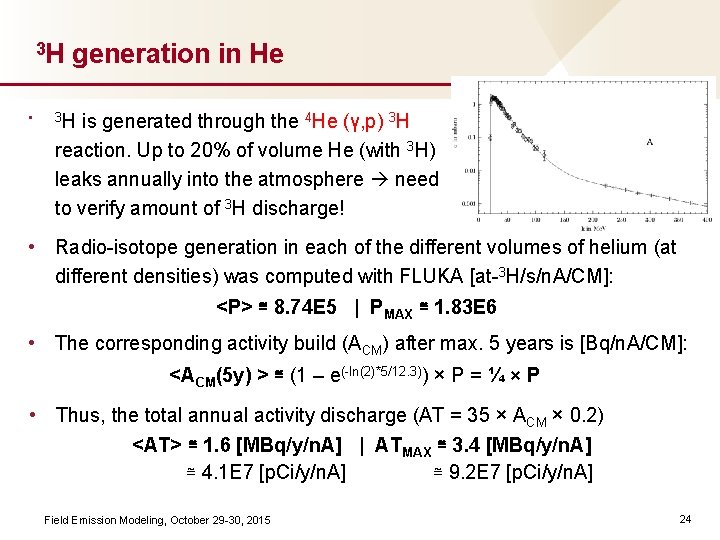
![Total power deposited by field radiation on the cavities < 50 [m. W/n. A] Total power deposited by field radiation on the cavities < 50 [m. W/n. A]](https://slidetodoc.com/presentation_image_h2/0991c3995f7973166186f6a6d2d69aef/image-25.jpg)
![Absorbed dose in L 2 Longitudinal [cm] Annual absorbed [Gy/n. A] at the cryomodule Absorbed dose in L 2 Longitudinal [cm] Annual absorbed [Gy/n. A] at the cryomodule](https://slidetodoc.com/presentation_image_h2/0991c3995f7973166186f6a6d2d69aef/image-26.jpg)
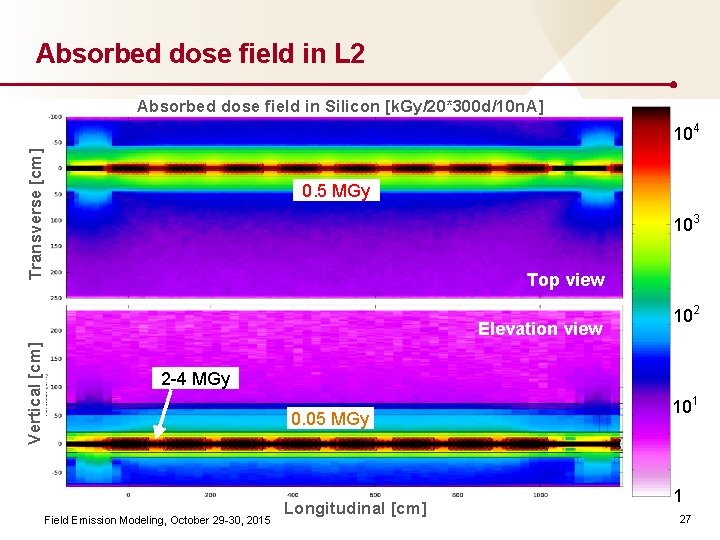
![Absorbed dose field in L 2 Absorbed dose rate maps [k. Gy] in plastics Absorbed dose field in L 2 Absorbed dose rate maps [k. Gy] in plastics](https://slidetodoc.com/presentation_image_h2/0991c3995f7973166186f6a6d2d69aef/image-28.jpg)
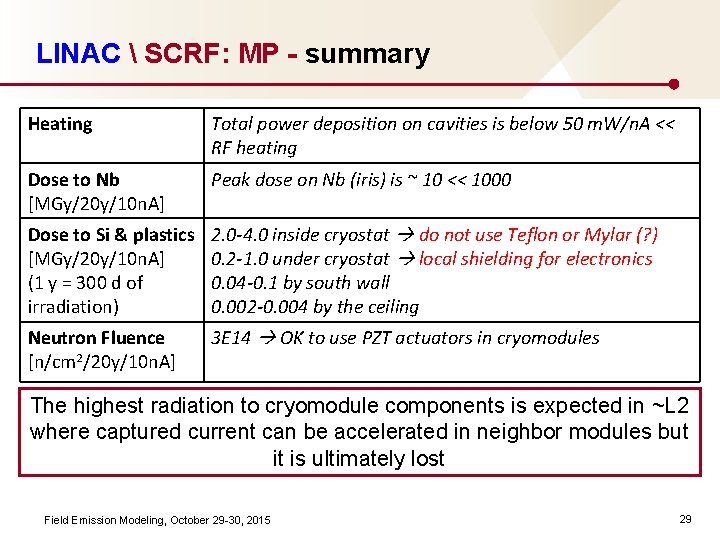
![Vertical tests - calibration Ongoing simulations to correlate field emission [n. A/cavity] with measured Vertical tests - calibration Ongoing simulations to correlate field emission [n. A/cavity] with measured](https://slidetodoc.com/presentation_image_h2/0991c3995f7973166186f6a6d2d69aef/image-30.jpg)
![Vertical tests – calibration: fluence vs. cc. T L A T O [cm-2/s/n. A] Vertical tests – calibration: fluence vs. cc. T L A T O [cm-2/s/n. A]](https://slidetodoc.com/presentation_image_h2/0991c3995f7973166186f6a6d2d69aef/image-31.jpg)
![Vertical tests – calibration dose eq. vs. cc. 10 [mrem/h/n. A] 2 [mrem/h/n. A] Vertical tests – calibration dose eq. vs. cc. 10 [mrem/h/n. A] 2 [mrem/h/n. A]](https://slidetodoc.com/presentation_image_h2/0991c3995f7973166186f6a6d2d69aef/image-32.jpg)
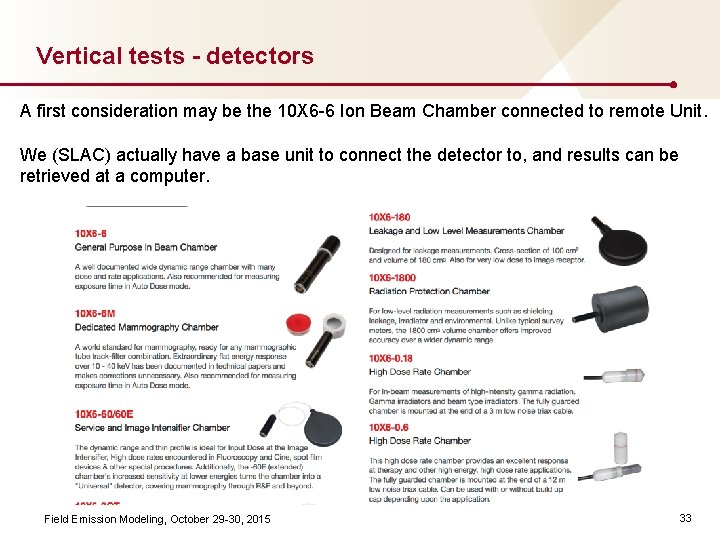
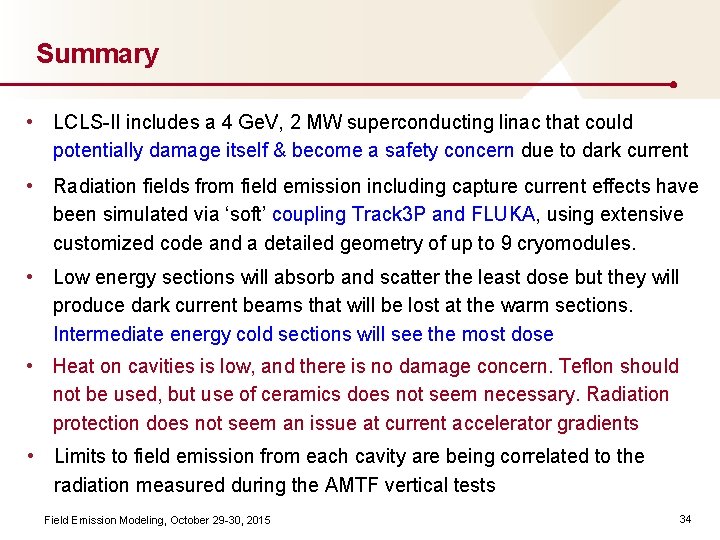
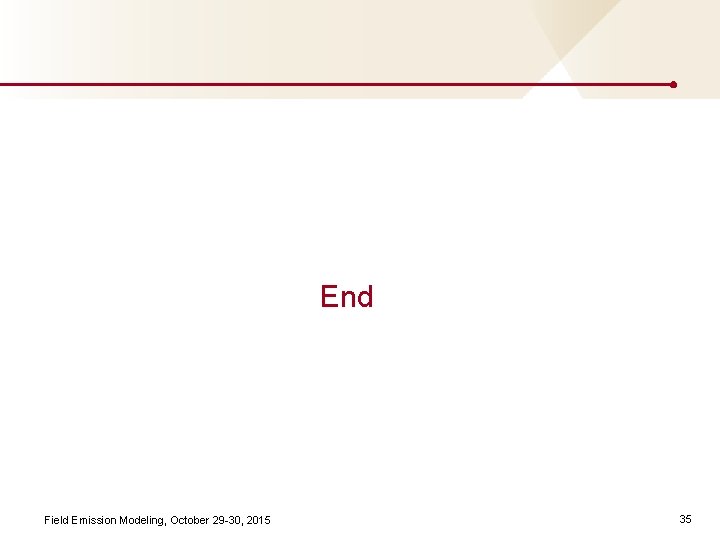
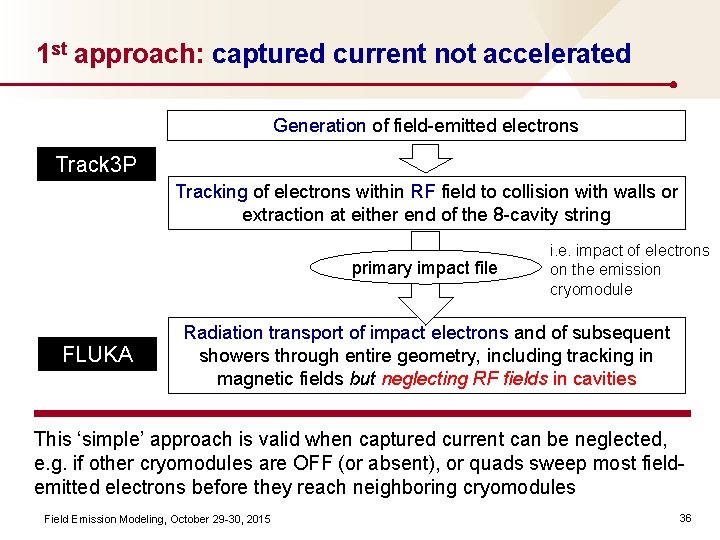
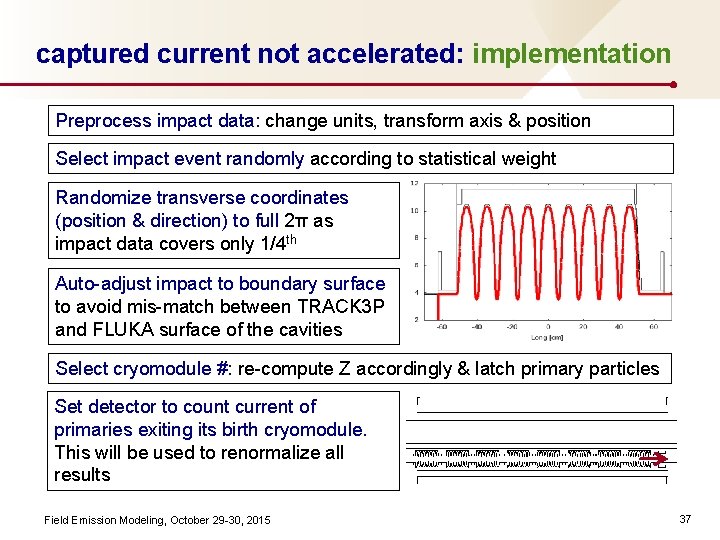
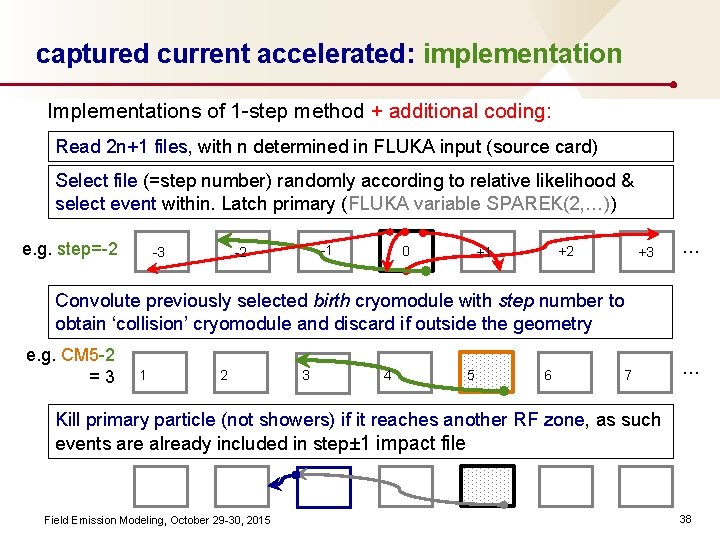
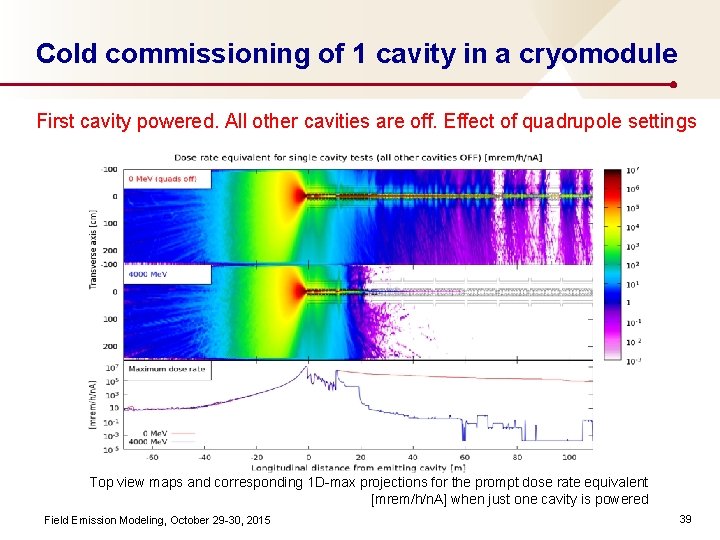
![Effect of captured current acceleration Vertical [cm] no acceleration in other cryomodules [mrem/h/10 n. Effect of captured current acceleration Vertical [cm] no acceleration in other cryomodules [mrem/h/10 n.](https://slidetodoc.com/presentation_image_h2/0991c3995f7973166186f6a6d2d69aef/image-40.jpg)
- Slides: 40
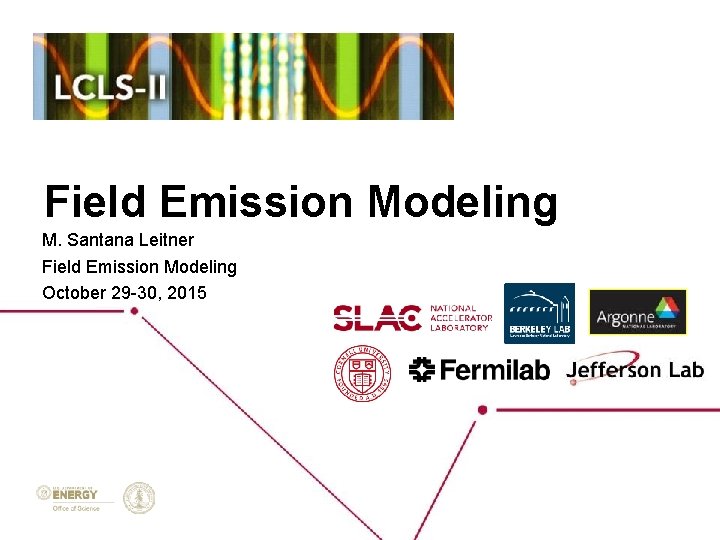
Field Emission Modeling M. Santana Leitner Field Emission Modeling October 29 -30, 2015
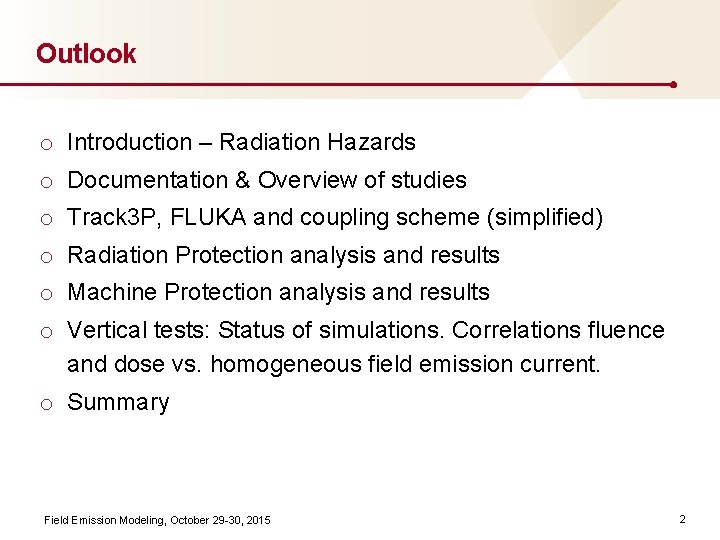
Outlook o Introduction – Radiation Hazards o Documentation & Overview of studies o Track 3 P, FLUKA and coupling scheme (simplified) o Radiation Protection analysis and results o Machine Protection analysis and results o Vertical tests: Status of simulations. Correlations fluence and dose vs. homogeneous field emission current. o Summary Field Emission Modeling, October 29 -30, 2015 2
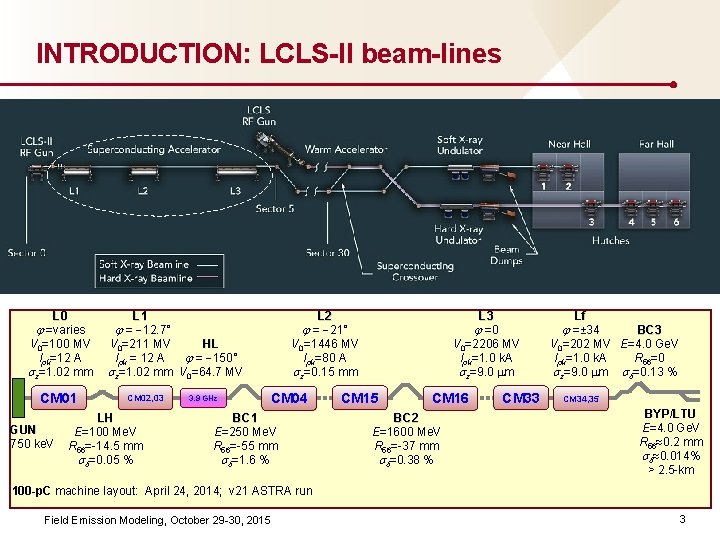
INTRODUCTION: LCLS-II beam-lines L 0 j =varies V 0=100 MV Ipk=12 A sz=1. 02 mm CM 01 GUN 750 ke. V L 2 j = -21° V 0=1446 MV Ipk=80 A sz=0. 15 mm L 1 j = -12. 7° HL V 0=211 MV j = -150° Ipk = 12 A V sz=1. 02 mm 0=64. 7 MV CM 02, 03 LH E=100 Me. V R 56=-14. 5 mm sd=0. 05 % 3. 9 GHz CM 04 BC 1 E=250 Me. V R 56=-55 mm sd=1. 6 % L 3 j =0 V 0=2206 MV Ipk=1. 0 k. A sz=9. 0 mm CM 15 CM 16 BC 2 E=1600 Me. V R 56=-37 mm sd=0. 38 % CM 33 Lf j =± 34 BC 3 V 0=202 MV E=4. 0 Ge. V Ipk=1. 0 k. A R 56=0 sz=9. 0 mm sd=0. 13 % CM 34, 35 BYP/LTU E=4. 0 Ge. V R 56 0. 2 mm sd 0. 014% > 2. 5 -km 100 -p. C machine layout: April 24, 2014; v 21 ASTRA run Field Emission Modeling, October 29 -30, 2015 3
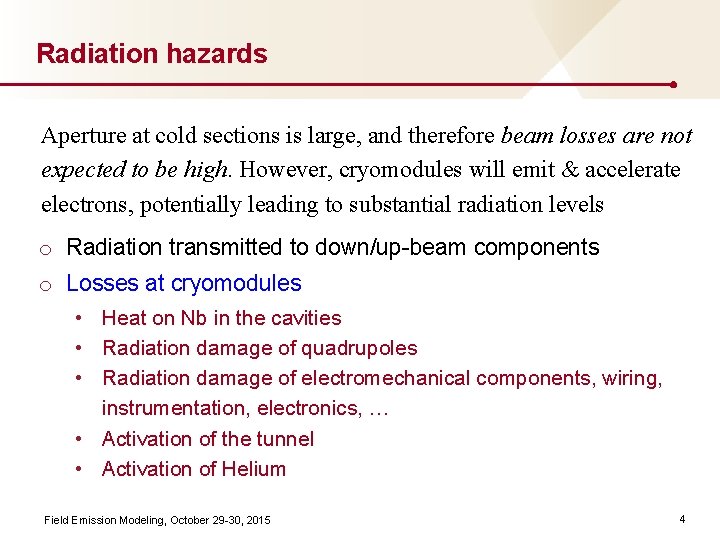
Radiation hazards Aperture at cold sections is large, and therefore beam losses are not expected to be high. However, cryomodules will emit & accelerate electrons, potentially leading to substantial radiation levels o Radiation transmitted to down/up-beam components o Losses at cryomodules • Heat on Nb in the cavities • Radiation damage of quadrupoles • Radiation damage of electromechanical components, wiring, instrumentation, electronics, … • Activation of the tunnel • Activation of Helium Field Emission Modeling, October 29 -30, 2015 4
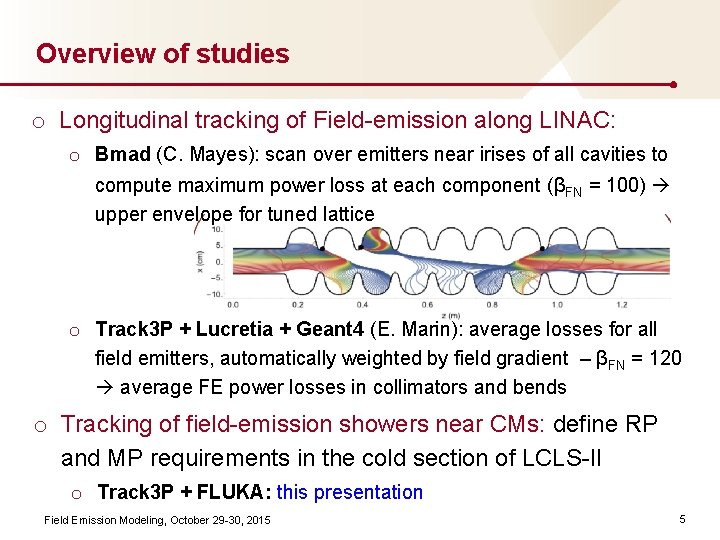
Overview of studies o Longitudinal tracking of Field-emission along LINAC: o Bmad (C. Mayes): scan over emitters near irises of all cavities to compute maximum power loss at each component (βFN = 100) upper envelope for tuned lattice o Track 3 P + Lucretia + Geant 4 (E. Marin): average losses for all field emitters, automatically weighted by field gradient – βFN = 120 average FE power losses in collimators and bends o Tracking of field-emission showers near CMs: define RP and MP requirements in the cold section of LCLS-II o Track 3 P + FLUKA: this presentation Field Emission Modeling, October 29 -30, 2015 5
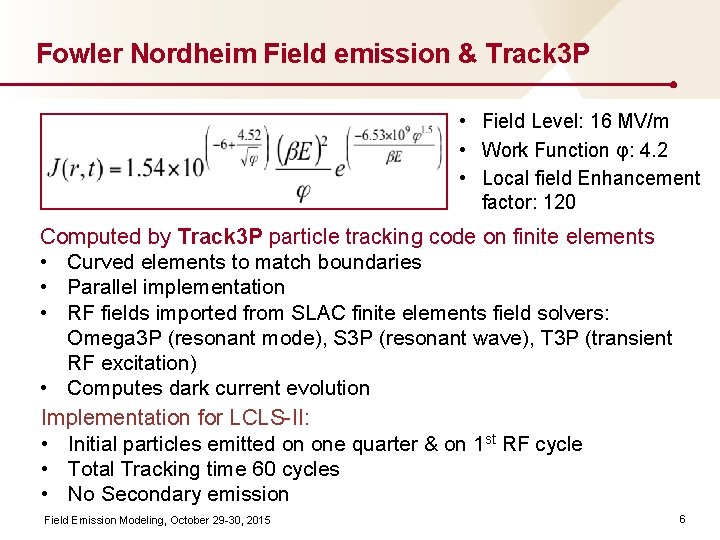
Fowler Nordheim Field emission & Track 3 P • Field Level: 16 MV/m • Work Function φ: 4. 2 • Local field Enhancement factor: 120 Computed by Track 3 P particle tracking code on finite elements • Curved elements to match boundaries • Parallel implementation • RF fields imported from SLAC finite elements field solvers: Omega 3 P (resonant mode), S 3 P (resonant wave), T 3 P (transient RF excitation) • Computes dark current evolution Implementation for LCLS-II: • Initial particles emitted on one quarter & on 1 st RF cycle • Total Tracking time 60 cycles • No Secondary emission Field Emission Modeling, October 29 -30, 2015 6
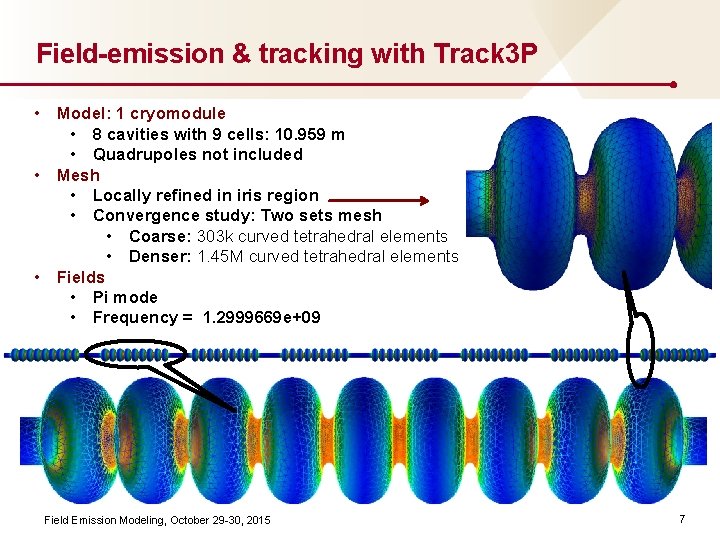
Field-emission & tracking with Track 3 P • Model: 1 cryomodule • 8 cavities with 9 cells: 10. 959 m • Quadrupoles not included • Mesh • Locally refined in iris region • Convergence study: Two sets mesh • Coarse: 303 k curved tetrahedral elements • Denser: 1. 45 M curved tetrahedral elements • Fields • Pi mode • Frequency = 1. 2999669 e+09 Field Emission Modeling, October 29 -30, 2015 7
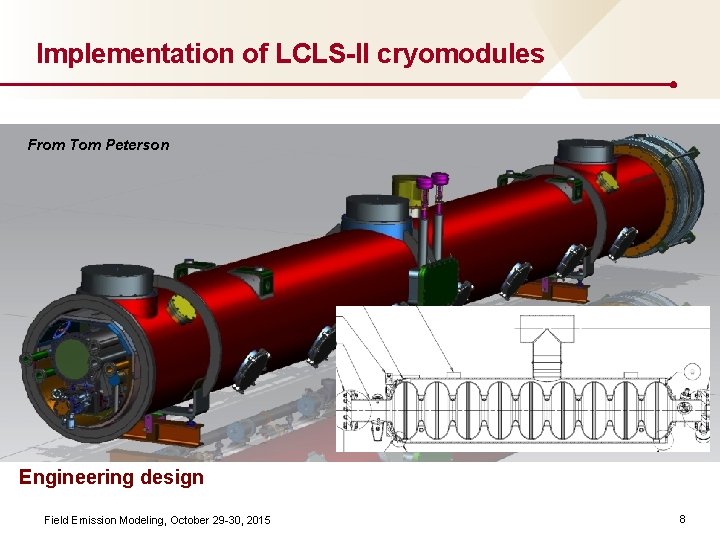
Implementation of LCLS-II cryomodules From Tom Peterson Engineering design Field Emission Modeling, October 29 -30, 2015 8
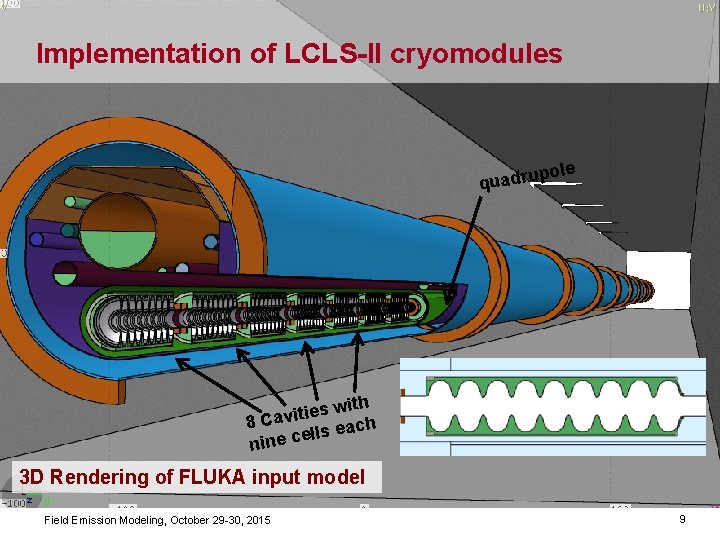
Implementation of LCLS-II cryomodules le po quadru with s e i t i v 8 Ca each s l l e c nine 3 D Rendering of FLUKA input model Field Emission Modeling, October 29 -30, 2015 9
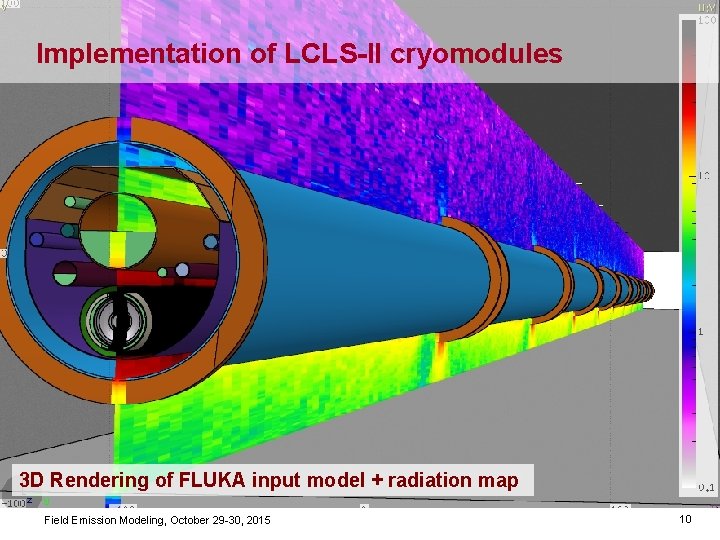
Implementation of LCLS-II cryomodules 3 D Rendering of FLUKA input model + radiation map Field Emission Modeling, October 29 -30, 2015 10
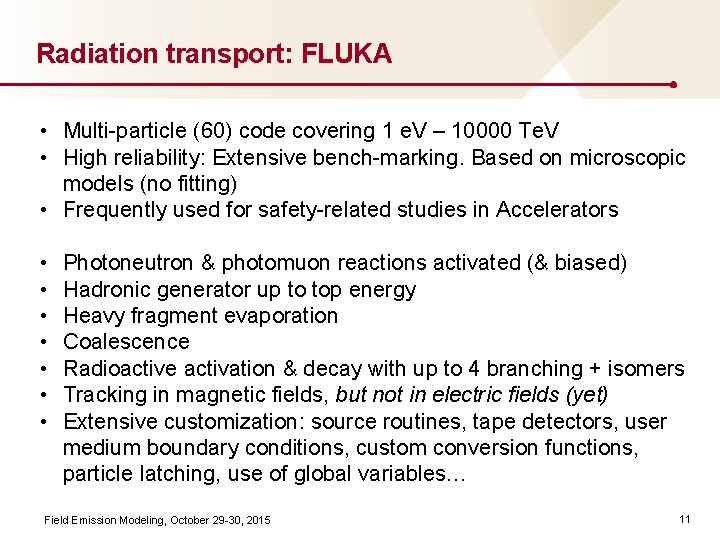
Radiation transport: FLUKA • Multi-particle (60) code covering 1 e. V – 10000 Te. V • High reliability: Extensive bench-marking. Based on microscopic models (no fitting) • Frequently used for safety-related studies in Accelerators • • Photoneutron & photomuon reactions activated (& biased) Hadronic generator up to top energy Heavy fragment evaporation Coalescence Radioactive activation & decay with up to 4 branching + isomers Tracking in magnetic fields, but not in electric fields (yet) Extensive customization: source routines, tape detectors, user medium boundary conditions, custom conversion functions, particle latching, use of global variables… Field Emission Modeling, October 29 -30, 2015 11
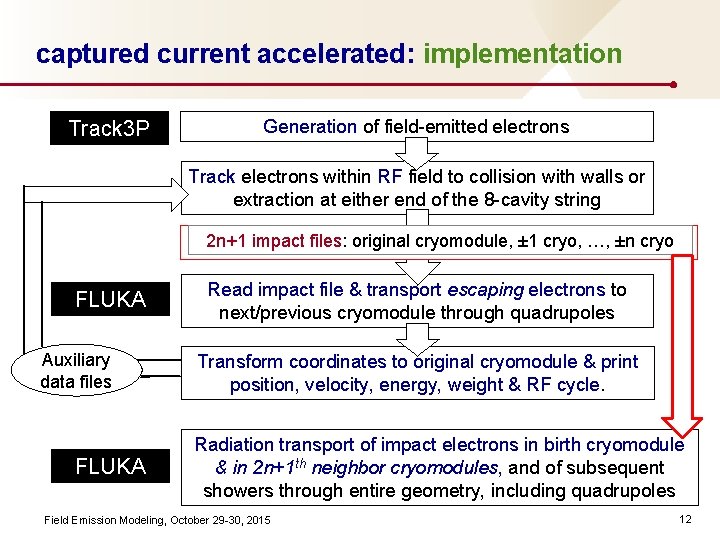
captured current accelerated: implementation Track 3 P Generation of field-emitted electrons Track electrons within RF field to collision with walls or extraction at either end of the 8 -cavity string 2 n+1 impact files: original cryomodule, ± 1 cryo, …, ±n cryo FLUKA Auxiliary data files FLUKA Read impact file & transport escaping electrons to next/previous cryomodule through quadrupoles Transform coordinates to original cryomodule & print position, velocity, energy, weight & RF cycle. Radiation transport of impact electrons in birth cryomodule & in 2 n+1 th neighbor cryomodules, and of subsequent showers through entire geometry, including quadrupoles Field Emission Modeling, October 29 -30, 2015 12
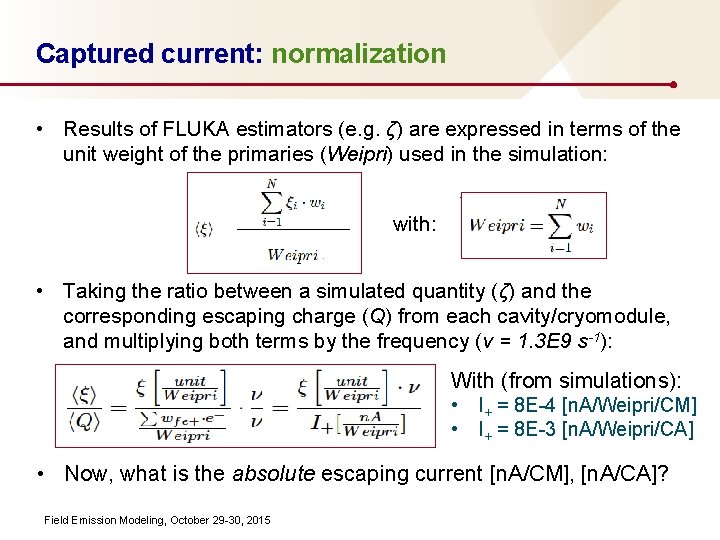
Captured current: normalization • Results of FLUKA estimators (e. g. ζ) are expressed in terms of the unit weight of the primaries (Weipri) used in the simulation: with: • Taking the ratio between a simulated quantity (ζ) and the corresponding escaping charge (Q) from each cavity/cryomodule, and multiplying both terms by the frequency (ν = 1. 3 E 9 s-1): With (from simulations): • I+ = 8 E-4 [n. A/Weipri/CM] • I+ = 8 E-3 [n. A/Weipri/CA] • Now, what is the absolute escaping current [n. A/CM], [n. A/CA]? Field Emission Modeling, October 29 -30, 2015
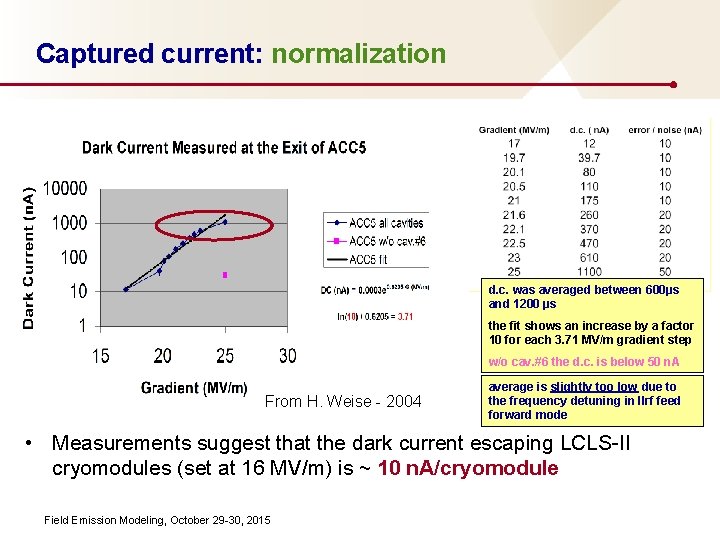
Captured current: normalization d. c. was averaged between 600µs and 1200 µs the fit shows an increase by a factor 10 for each 3. 71 MV/m gradient step w/o cav. #6 the d. c. is below 50 n. A From H. Weise - 2004 average is slightly too low due to the frequency detuning in llrf feed forward mode • Measurements suggest that the dark current escaping LCLS-II cryomodules (set at 16 MV/m) is ~ 10 n. A/cryomodule Field Emission Modeling, October 29 -30, 2015
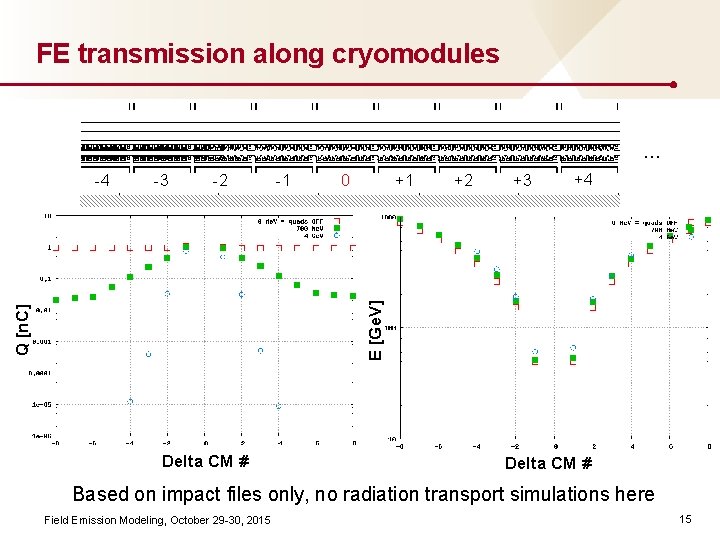
FE transmission along cryomodules … -3 -2 -1 0 +1 +2 +3 +4 Q [n. C] E [Ge. V] -4 Delta CM # Based on impact files only, no radiation transport simulations here Field Emission Modeling, October 29 -30, 2015 15
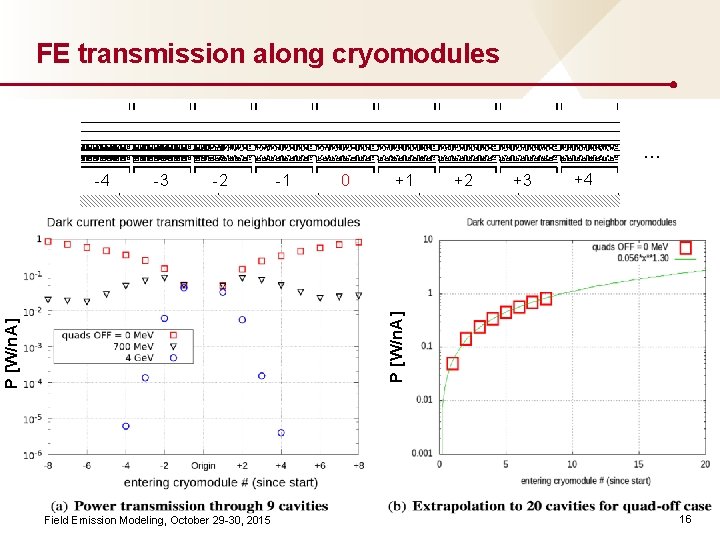
FE transmission along cryomodules … -3 -2 -1 0 +1 +2 +3 +4 P [W/n. A] -4 Field Emission Modeling, October 29 -30, 2015 16
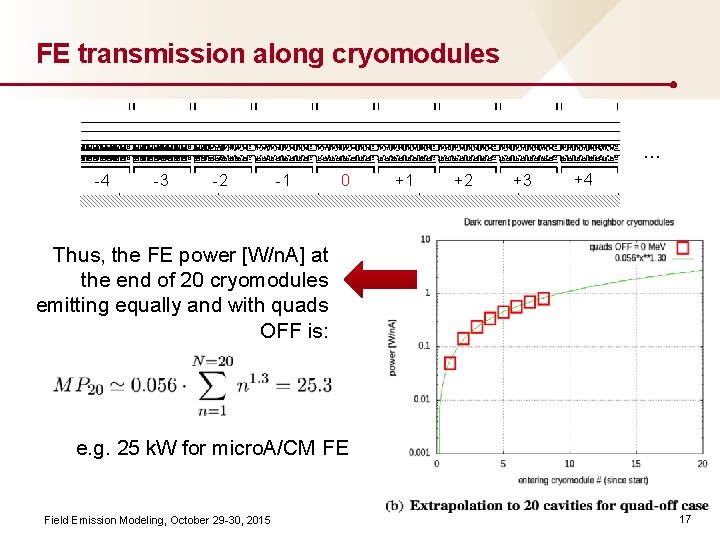
FE transmission along cryomodules … -4 -3 -2 -1 0 +1 +2 +3 +4 Thus, the FE power [W/n. A] at the end of 20 cryomodules emitting equally and with quads OFF is: e. g. 25 k. W for micro. A/CM FE Field Emission Modeling, October 29 -30, 2015 17
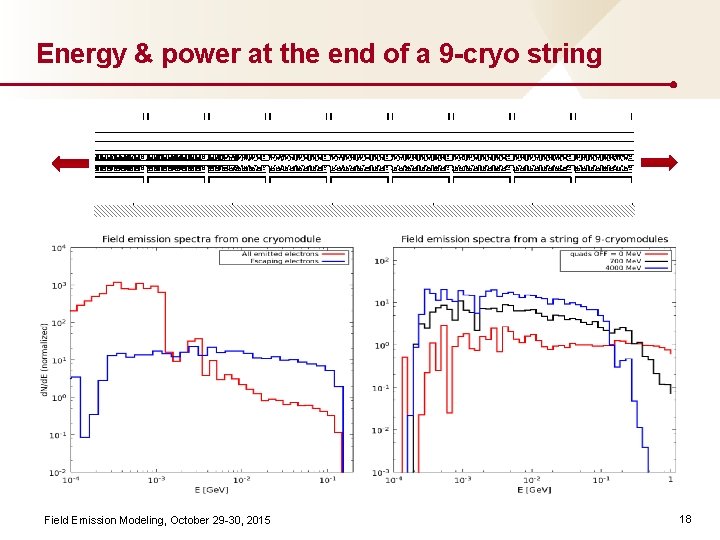
Energy & power at the end of a 9 -cryo string Field Emission Modeling, October 29 -30, 2015 18
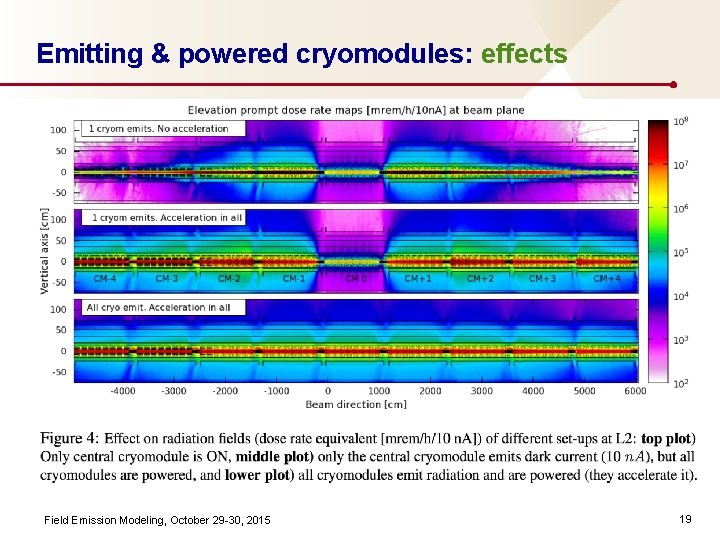
Emitting & powered cryomodules: effects Field Emission Modeling, October 29 -30, 2015 19
![Vertical cm Gy300 dn A Vertical cm Effect of quad settings on radiation fields Vertical [cm] [Gy/300 d/n. A] Vertical [cm] Effect of quad settings on radiation fields](https://slidetodoc.com/presentation_image_h2/0991c3995f7973166186f6a6d2d69aef/image-20.jpg)
Vertical [cm] [Gy/300 d/n. A] Vertical [cm] Effect of quad settings on radiation fields Field Emission Modeling, October 29 -30, 2015 0 Me. V 700 Me. V 4000 Me. V Longitudinal [cm] 20
![0 Me V Vertical cm Settings at 700 Me V are the 700 worst 0 Me. V Vertical [cm] Settings at 700 Me. V are the 700 worst](https://slidetodoc.com/presentation_image_h2/0991c3995f7973166186f6a6d2d69aef/image-21.jpg)
0 Me. V Vertical [cm] Settings at 700 Me. V are the 700 worst Me. V for radiation to the cryomodules as field emission is accelerated but ultimately is lost, depositing most energy Vertical [cm] [Gy/300 d/n. A] Vertical [cm] Effect of quad settings on radiation fields Field Emission Modeling, October 29 -30, 2015 4000 Me. V Longitudinal [cm] 21
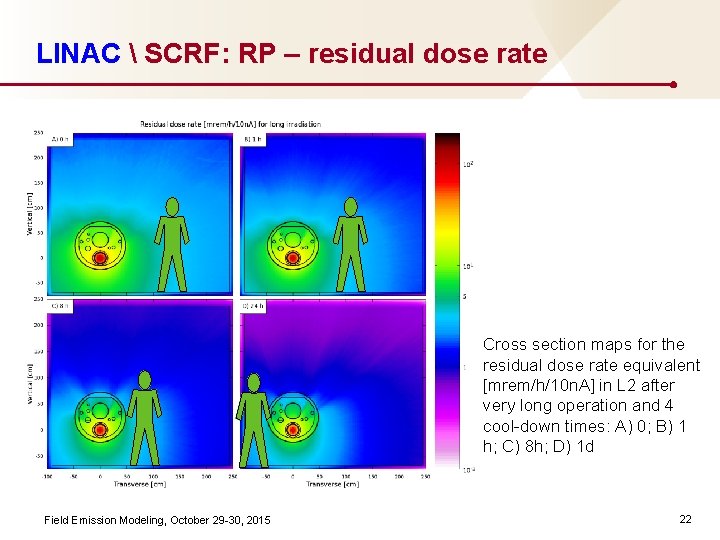
LINAC SCRF: RP – residual dose rate Cross section maps for the residual dose rate equivalent [mrem/h/10 n. A] in L 2 after very long operation and 4 cool-down times: A) 0; B) 1 h; C) 8 h; D) 1 d Field Emission Modeling, October 29 -30, 2015 22
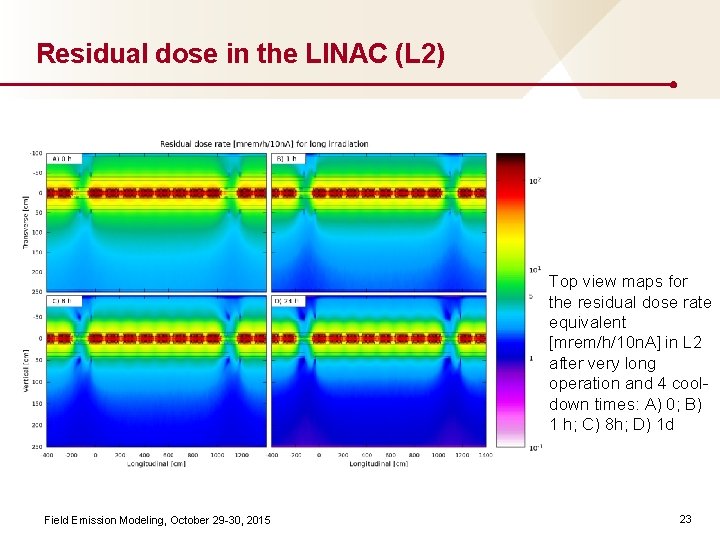
Residual dose in the LINAC (L 2) Top view maps for the residual dose rate equivalent [mrem/h/10 n. A] in L 2 after very long operation and 4 cooldown times: A) 0; B) 1 h; C) 8 h; D) 1 d Field Emission Modeling, October 29 -30, 2015 23
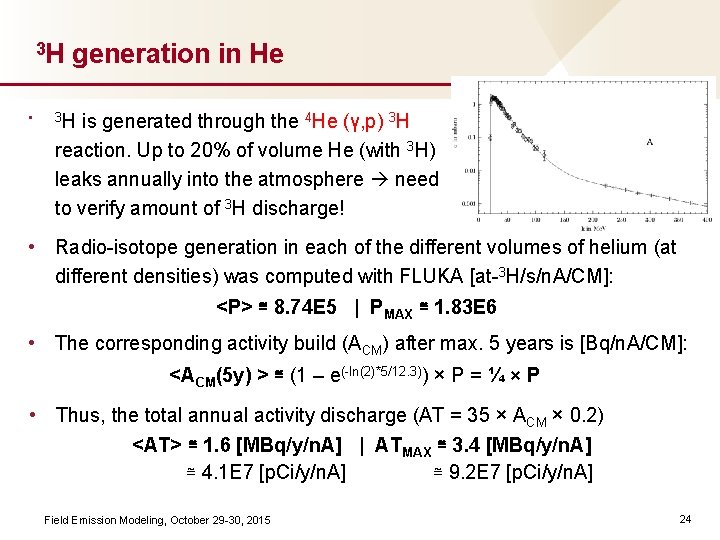
3 H • generation in He 3 H is generated through the 4 He (γ, p) 3 H reaction. Up to 20% of volume He (with 3 H) leaks annually into the atmosphere need to verify amount of 3 H discharge! • Radio-isotope generation in each of the different volumes of helium (at different densities) was computed with FLUKA [at-3 H/s/n. A/CM]: <P> ≅ 8. 74 E 5 | PMAX ≅ 1. 83 E 6 • The corresponding activity build (ACM) after max. 5 years is [Bq/n. A/CM]: <ACM(5 y) > ≅ (1 – e(-ln(2)*5/12. 3)) × P = ¼ × P • Thus, the total annual activity discharge (AT = 35 × ACM × 0. 2) <AT> ≅ 1. 6 [MBq/y/n. A] | ATMAX ≅ 3. 4 [MBq/y/n. A] ≅ 4. 1 E 7 [p. Ci/y/n. A] ≅ 9. 2 E 7 [p. Ci/y/n. A] Field Emission Modeling, October 29 -30, 2015 24
![Total power deposited by field radiation on the cavities 50 m Wn A Total power deposited by field radiation on the cavities < 50 [m. W/n. A]](https://slidetodoc.com/presentation_image_h2/0991c3995f7973166186f6a6d2d69aef/image-25.jpg)
Total power deposited by field radiation on the cavities < 50 [m. W/n. A] This is negligible when compared to the 100 W from RF heating <10 No damage to Nb is expected [MGy/20 y/10 n. A] Dose to cavities [Gy/y/n. A] Field Emission Modeling, October 29 -30, 2015 25
![Absorbed dose in L 2 Longitudinal cm Annual absorbed Gyn A at the cryomodule Absorbed dose in L 2 Longitudinal [cm] Annual absorbed [Gy/n. A] at the cryomodule](https://slidetodoc.com/presentation_image_h2/0991c3995f7973166186f6a6d2d69aef/image-26.jpg)
Absorbed dose in L 2 Longitudinal [cm] Annual absorbed [Gy/n. A] at the cryomodule quadrupoles of L 2 Vertical [cm] Annual absorbed dose field for Si [Gy/n. A] between cryomodules at L 2 Transverse [cm] Field Emission Modeling, October 29 -30, 2015 26
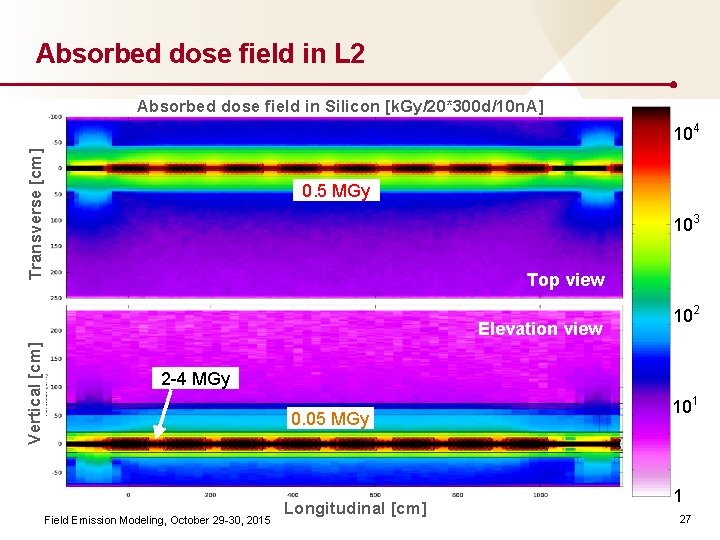
Absorbed dose field in L 2 Absorbed dose field in Silicon [k. Gy/20*300 d/10 n. A] Transverse [cm] 104 0. 5 MGy 103 Top view Vertical [cm] Elevation view 102 2 -4 MGy 0. 05 MGy Field Emission Modeling, October 29 -30, 2015 Longitudinal [cm] 101 1 27
![Absorbed dose field in L 2 Absorbed dose rate maps k Gy in plastics Absorbed dose field in L 2 Absorbed dose rate maps [k. Gy] in plastics](https://slidetodoc.com/presentation_image_h2/0991c3995f7973166186f6a6d2d69aef/image-28.jpg)
Absorbed dose field in L 2 Absorbed dose rate maps [k. Gy] in plastics and light materials for 20 year operation (300 day/year) at 10 [n. A/cryomodule] 2 -4 k. Gy 104 Vertical [cm] 103 102 1. 0 MGy 0. 1 MGy 101 Transverse [cm] 1 Field Emission Modeling, October 29 -30, 2015 28
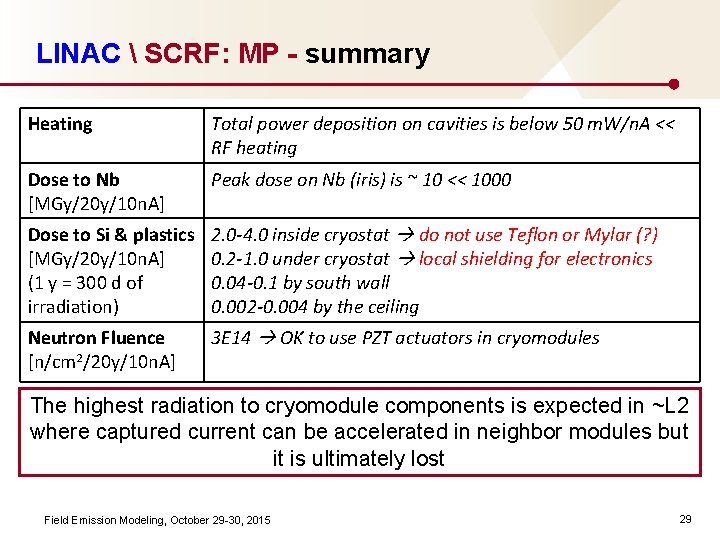
LINAC SCRF: MP - summary Heating Total power deposition on cavities is below 50 m. W/n. A << RF heating Dose to Nb [MGy/20 y/10 n. A] Peak dose on Nb (iris) is ~ 10 << 1000 Dose to Si & plastics [MGy/20 y/10 n. A] (1 y = 300 d of irradiation) 2. 0 -4. 0 inside cryostat do not use Teflon or Mylar (? ) 0. 2 -1. 0 under cryostat local shielding for electronics 0. 04 -0. 1 by south wall 0. 002 -0. 004 by the ceiling Neutron Fluence [n/cm 2/20 y/10 n. A] 3 E 14 OK to use PZT actuators in cryomodules The highest radiation to cryomodule components is expected in ~L 2 where captured current can be accelerated in neighbor modules but it is ultimately lost Field Emission Modeling, October 29 -30, 2015 29
![Vertical tests calibration Ongoing simulations to correlate field emission n Acavity with measured Vertical tests - calibration Ongoing simulations to correlate field emission [n. A/cavity] with measured](https://slidetodoc.com/presentation_image_h2/0991c3995f7973166186f6a6d2d69aef/image-30.jpg)
Vertical tests - calibration Ongoing simulations to correlate field emission [n. A/cavity] with measured radiation at the vertical tests FLUKA Model Field Emission Modeling, October 29 -30, 2015 30
![Vertical tests calibration fluence vs cc T L A T O cm2sn A Vertical tests – calibration: fluence vs. cc. T L A T O [cm-2/s/n. A]](https://slidetodoc.com/presentation_image_h2/0991c3995f7973166186f6a6d2d69aef/image-31.jpg)
Vertical tests – calibration: fluence vs. cc. T L A T O [cm-2/s/n. A] 20000 [cm-2/s/n. A] PHO TON 10000 [cm-2/s/n. A] Field Emission Modeling, October 29 -30, 2015 31
![Vertical tests calibration dose eq vs cc 10 mremhn A 2 mremhn A Vertical tests – calibration dose eq. vs. cc. 10 [mrem/h/n. A] 2 [mrem/h/n. A]](https://slidetodoc.com/presentation_image_h2/0991c3995f7973166186f6a6d2d69aef/image-32.jpg)
Vertical tests – calibration dose eq. vs. cc. 10 [mrem/h/n. A] 2 [mrem/h/n. A] Field Emission Modeling, October 29 -30, 2015 32
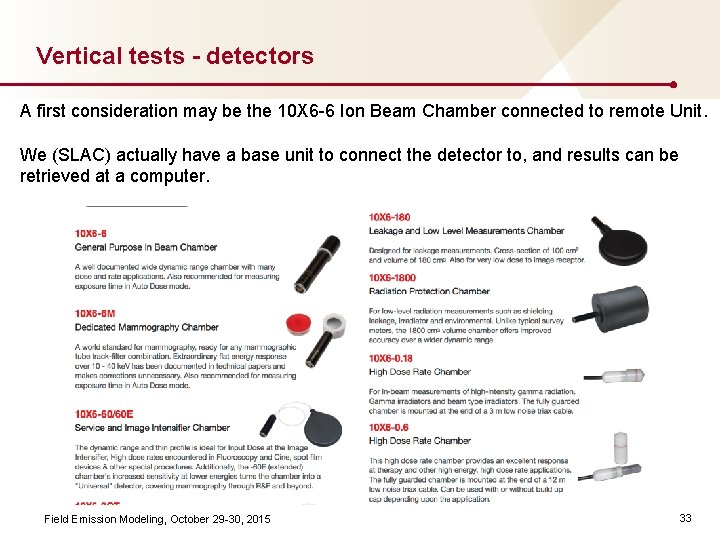
Vertical tests - detectors A first consideration may be the 10 X 6 -6 Ion Beam Chamber connected to remote Unit. We (SLAC) actually have a base unit to connect the detector to, and results can be retrieved at a computer. Field Emission Modeling, October 29 -30, 2015 33
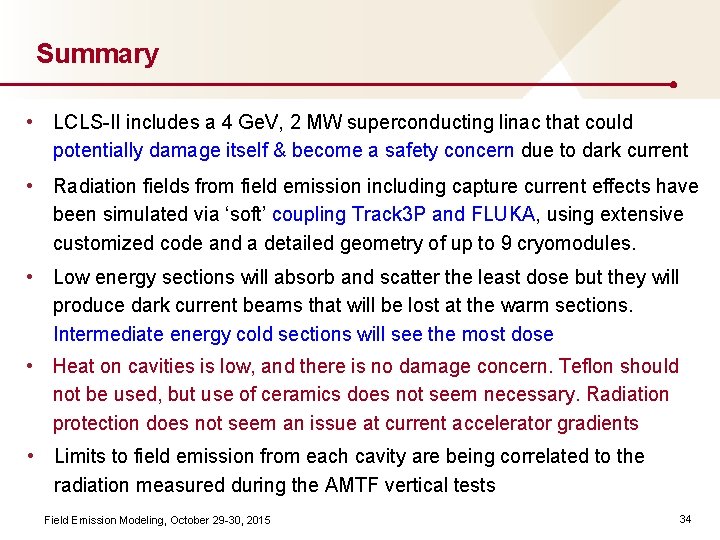
Summary • LCLS-II includes a 4 Ge. V, 2 MW superconducting linac that could potentially damage itself & become a safety concern due to dark current • Radiation fields from field emission including capture current effects have been simulated via ‘soft’ coupling Track 3 P and FLUKA, using extensive customized code and a detailed geometry of up to 9 cryomodules. • Low energy sections will absorb and scatter the least dose but they will produce dark current beams that will be lost at the warm sections. Intermediate energy cold sections will see the most dose • Heat on cavities is low, and there is no damage concern. Teflon should not be used, but use of ceramics does not seem necessary. Radiation protection does not seem an issue at current accelerator gradients • Limits to field emission from each cavity are being correlated to the radiation measured during the AMTF vertical tests Field Emission Modeling, October 29 -30, 2015 34
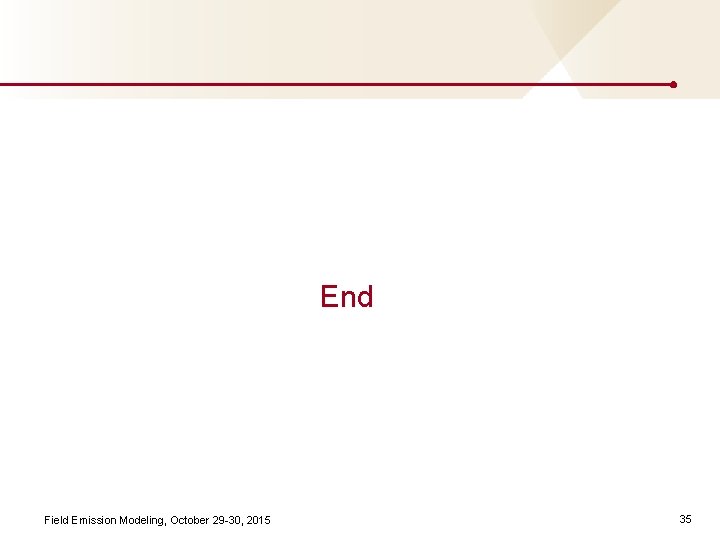
End Field Emission Modeling, October 29 -30, 2015 35
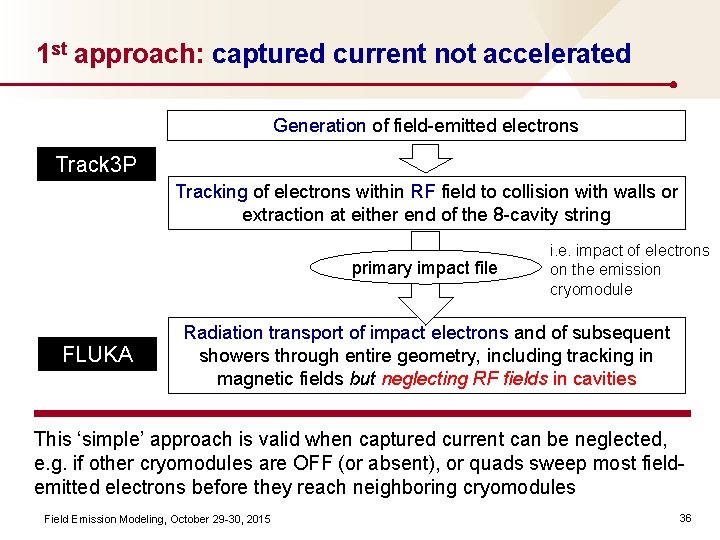
1 st approach: captured current not accelerated Generation of field-emitted electrons Track 3 P Tracking of electrons within RF field to collision with walls or extraction at either end of the 8 -cavity string primary impact file FLUKA i. e. impact of electrons on the emission cryomodule Radiation transport of impact electrons and of subsequent showers through entire geometry, including tracking in magnetic fields but neglecting RF fields in cavities This ‘simple’ approach is valid when captured current can be neglected, e. g. if other cryomodules are OFF (or absent), or quads sweep most fieldemitted electrons before they reach neighboring cryomodules Field Emission Modeling, October 29 -30, 2015 36
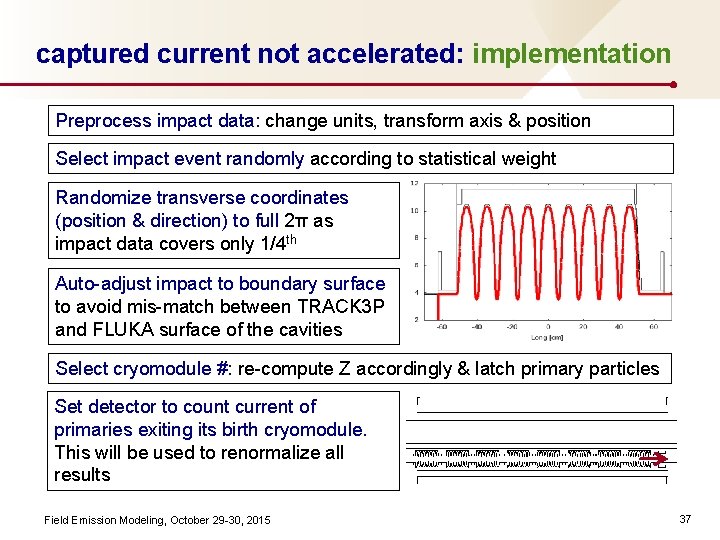
captured current not accelerated: implementation Preprocess impact data: change units, transform axis & position Select impact event randomly according to statistical weight Randomize transverse coordinates (position & direction) to full 2π as impact data covers only 1/4 th Auto-adjust impact to boundary surface to avoid mis-match between TRACK 3 P and FLUKA surface of the cavities Select cryomodule #: re-compute Z accordingly & latch primary particles Set detector to count current of primaries exiting its birth cryomodule. This will be used to renormalize all results Field Emission Modeling, October 29 -30, 2015 37
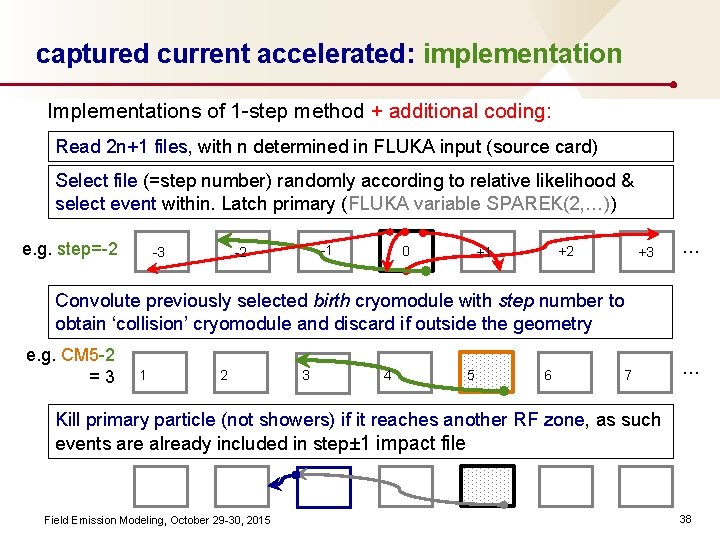
captured current accelerated: implementation Implementations of 1 -step method + additional coding: Read 2 n+1 files, with n determined in FLUKA input (source card) Select file (=step number) randomly according to relative likelihood & select event within. Latch primary (FLUKA variable SPAREK(2, …)) e. g. step=-2 -3 -1 -2 0 +2 +1 +3 … Convolute previously selected birth cryomodule with step number to obtain ‘collision’ cryomodule and discard if outside the geometry e. g. CM 5 -2 =3 1 2 3 4 5 6 7 … Kill primary particle (not showers) if it reaches another RF zone, as such events are already included in step± 1 impact file Field Emission Modeling, October 29 -30, 2015 38
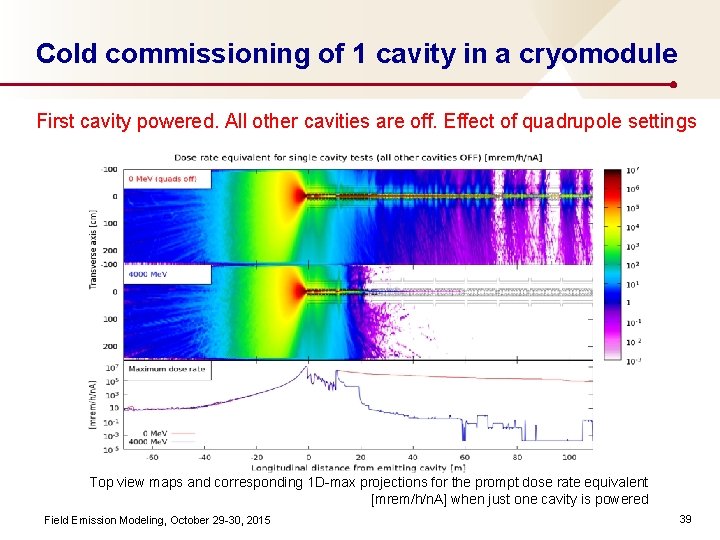
Cold commissioning of 1 cavity in a cryomodule First cavity powered. All other cavities are off. Effect of quadrupole settings Top view maps and corresponding 1 D-max projections for the prompt dose rate equivalent [mrem/h/n. A] when just one cavity is powered Field Emission Modeling, October 29 -30, 2015 39
![Effect of captured current acceleration Vertical cm no acceleration in other cryomodules mremh10 n Effect of captured current acceleration Vertical [cm] no acceleration in other cryomodules [mrem/h/10 n.](https://slidetodoc.com/presentation_image_h2/0991c3995f7973166186f6a6d2d69aef/image-40.jpg)
Effect of captured current acceleration Vertical [cm] no acceleration in other cryomodules [mrem/h/10 n. A] Vertical [cm] acceleration in other cryomodules Longitudinal [cm] Field Emission Modeling, October 29 -30, 2015 40
Carl leitner
Chamilo st louis
Rupert leitner
Relational modeling vs dimensional modeling
Model and role modeling theory
Edith santana
Dr jales benevides
Arrorro duerme ya a la luz estelar
Santana definition
Zaida padilla santana
Blog do vinicius de santana
Parque dos ipes santana do livramento
Imperfect subjunctive endings
Carlos augusto alves santana
Carlos santana childhood
Ritualer i hinduismen
Santana dharma
Vasco santana escola
Emelina santana paez
Carlos santana e john smith
Helig veckodag hinduism
Dharma hinduismen
Gilda santana
Lidia santana vega
Softwares can_ -in designing and modeling in every field
Field dependent vs field independent
Database field types and field properties
Distinguish between magnetic and nonmagnetic materials
Field dependent and field independent
Magnitude of magnetic force
Difference between electric field and magnetic field
Waveguide cutoff frequency
Individual differences factors
Cispr 22 radiated emission limits
Photo emission microscopy failure analysis
Half-life formula
Difference between absorption and emission spectrum
Positron emission tomography
Spontaneous emission
Emission
Cobalt 60 nuclear equation