ENHANCED LASERDRIVEN PROTON ACCELERATION IN MASSLIMITED TARGETS Jan
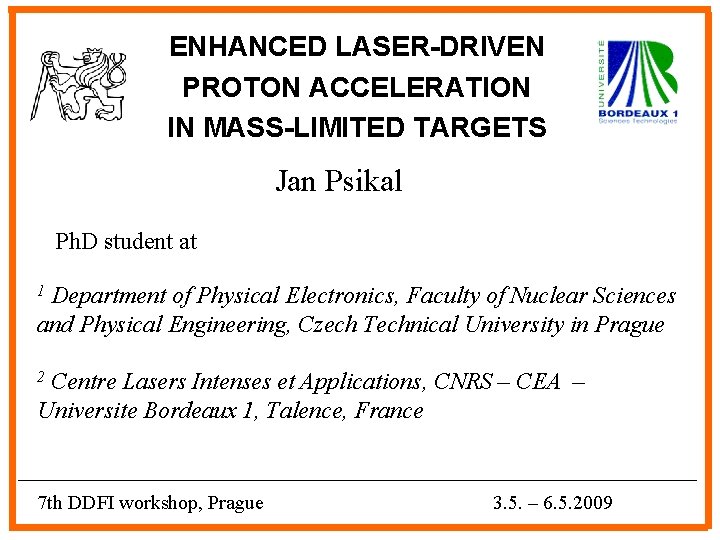
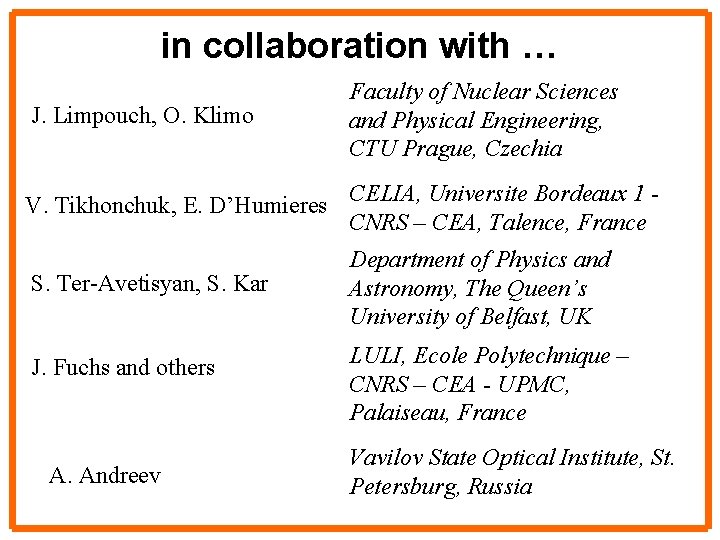
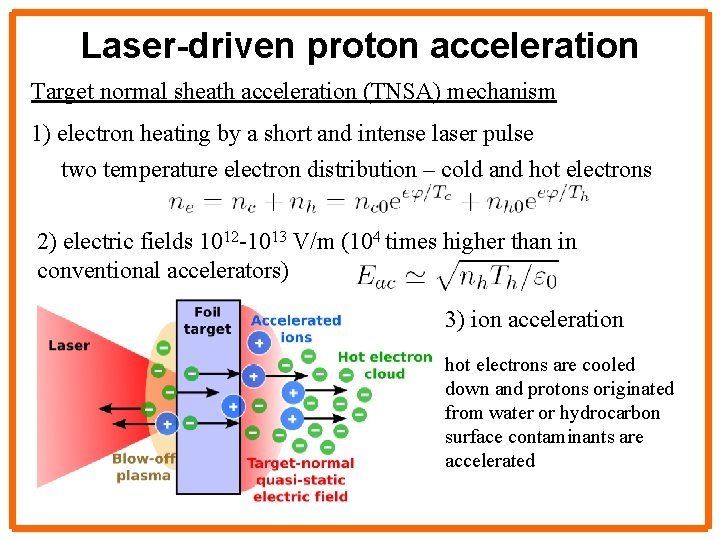
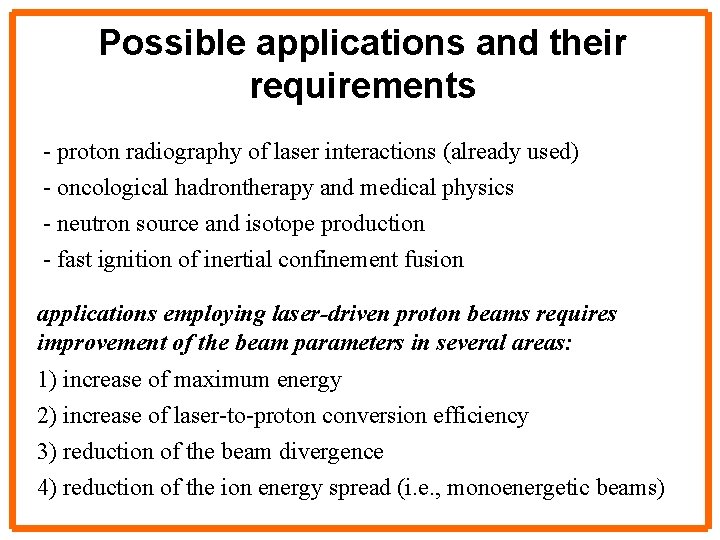
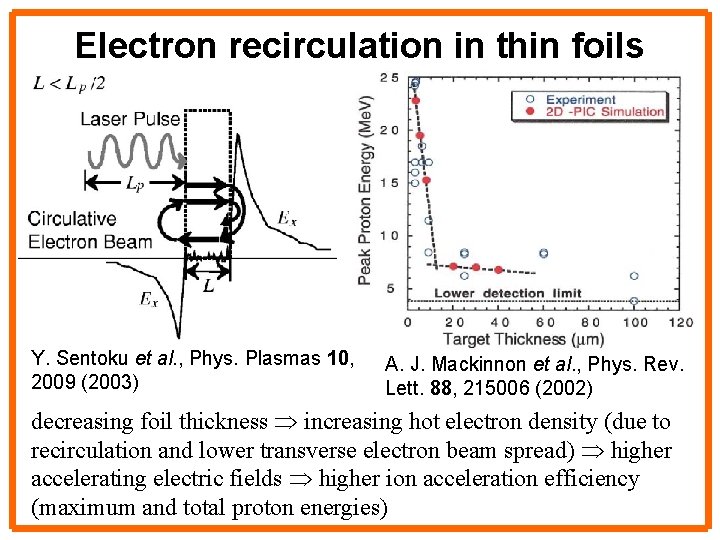
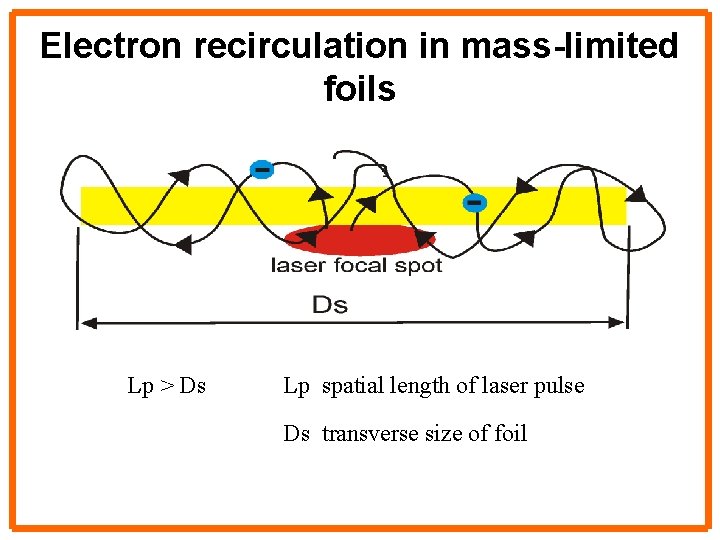
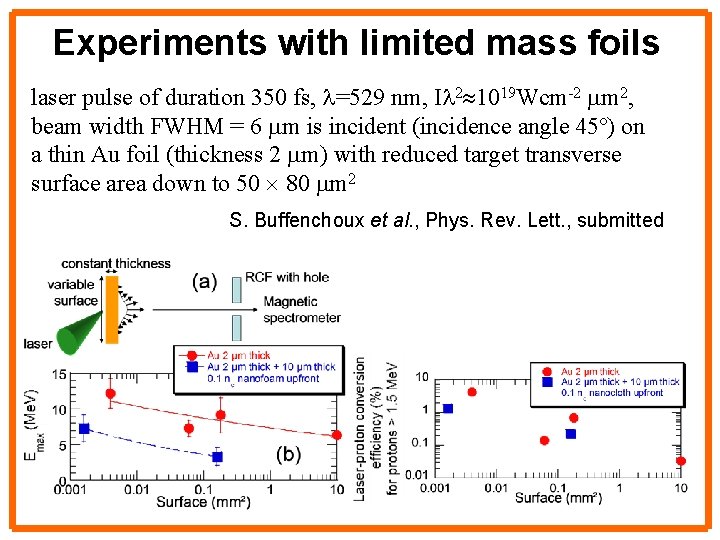
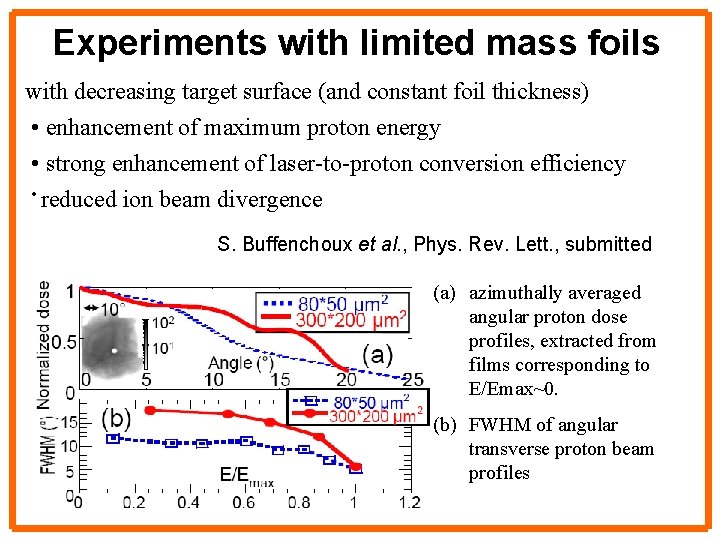
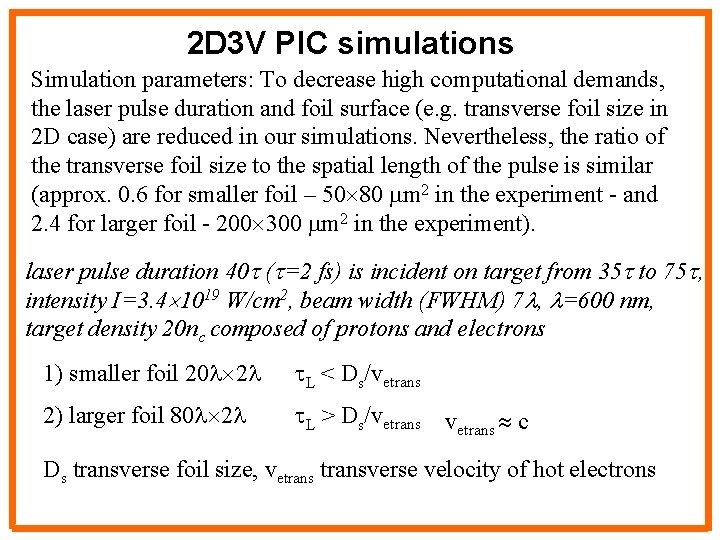
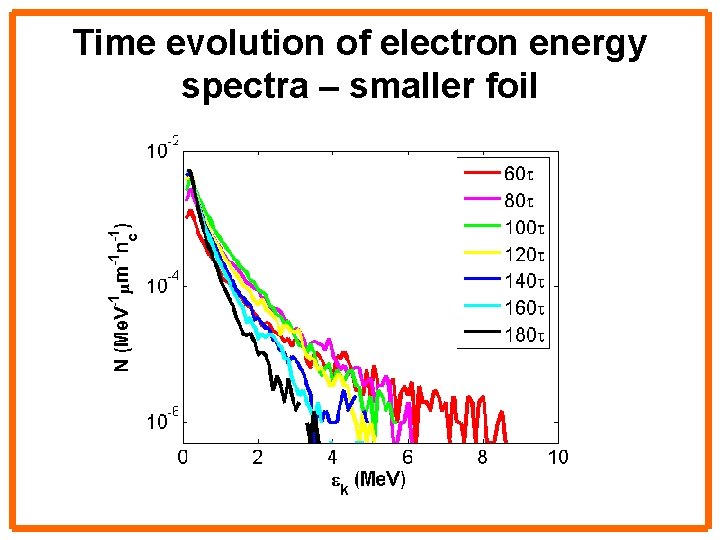
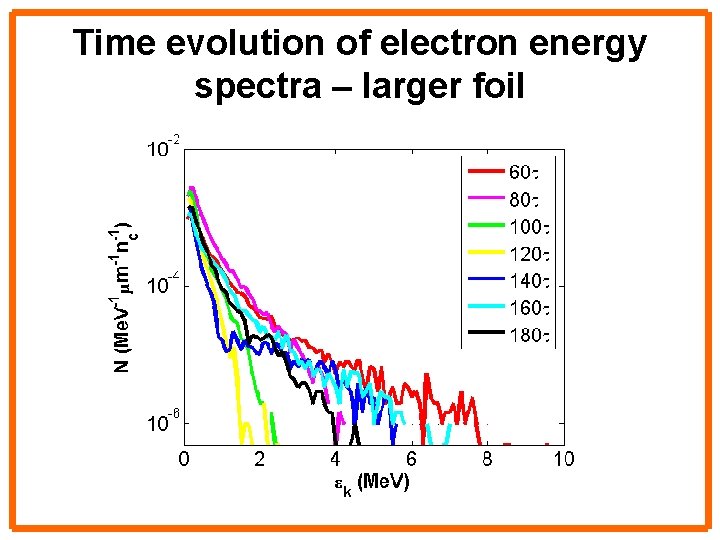
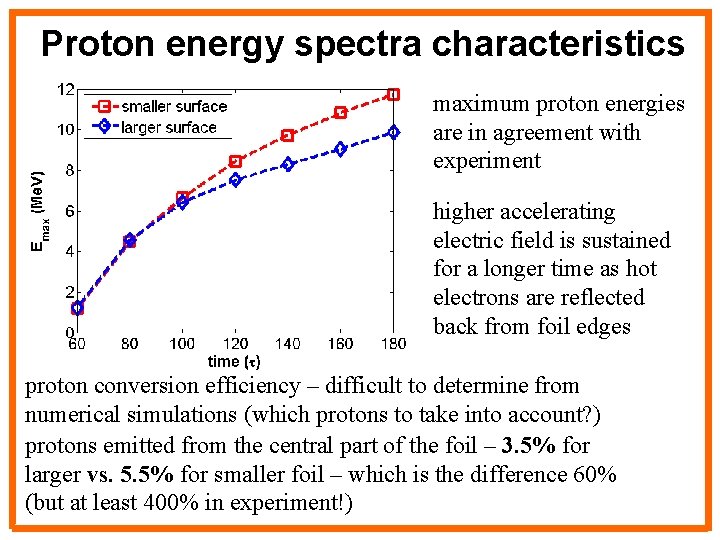
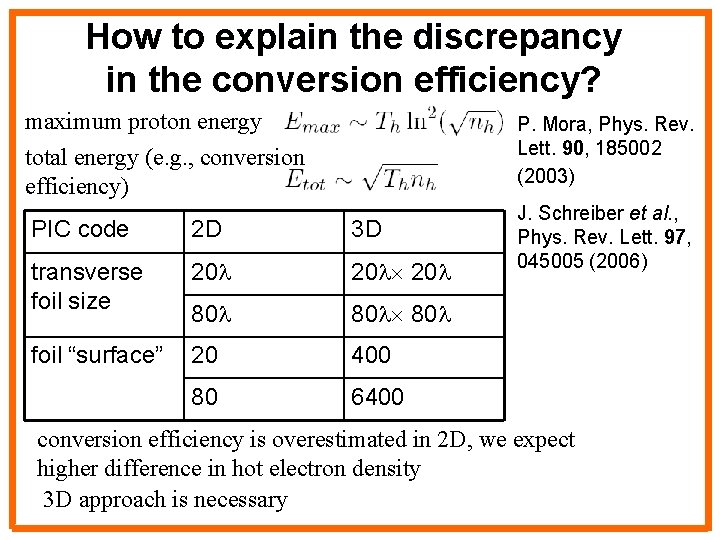
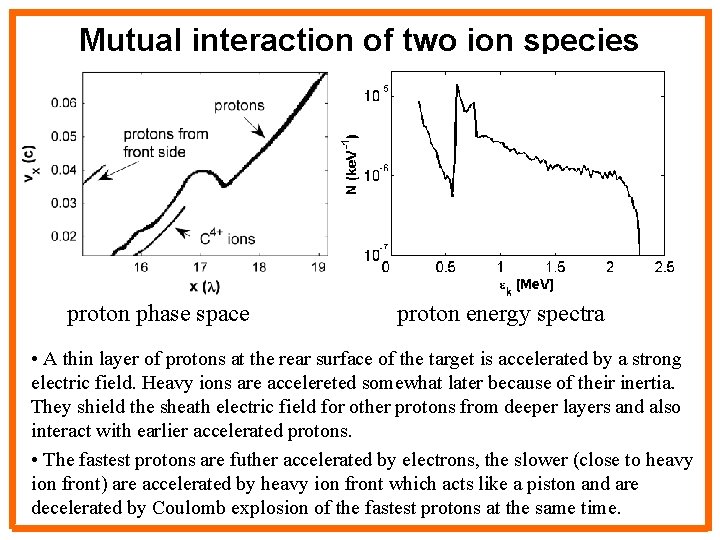
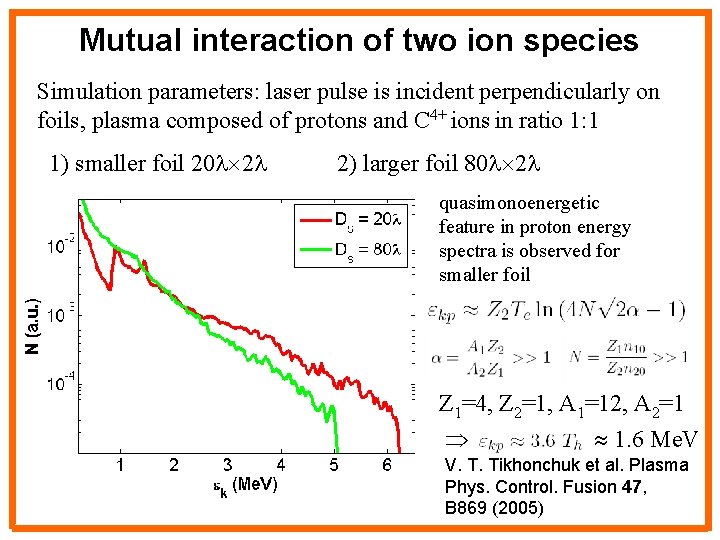
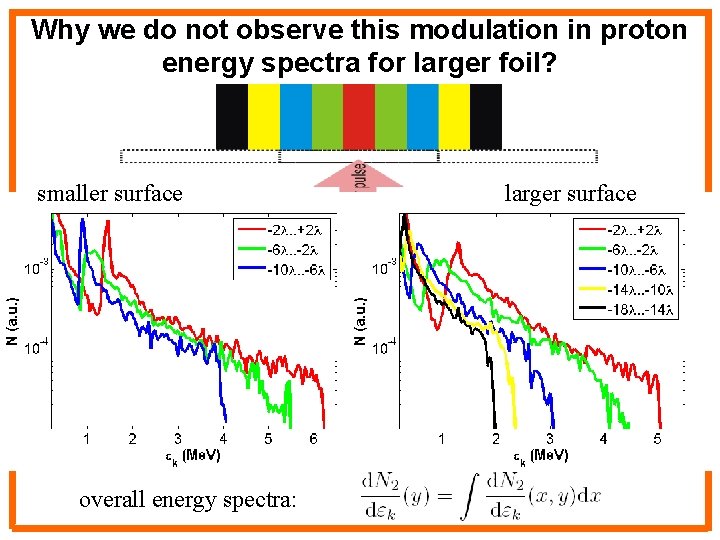
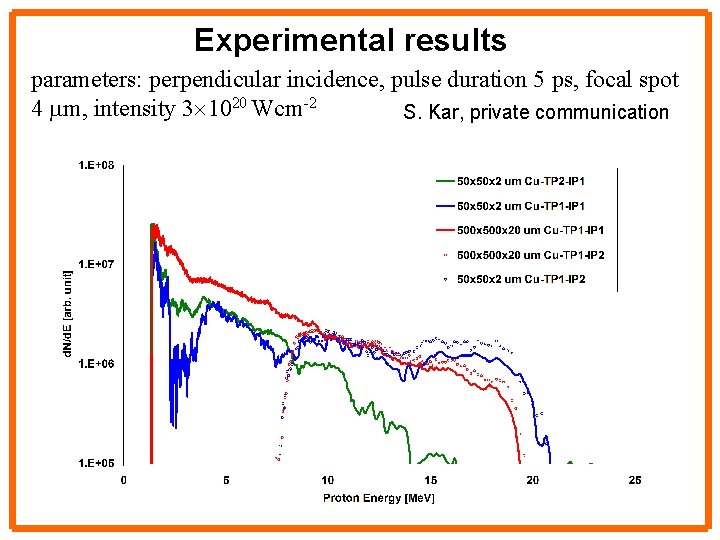
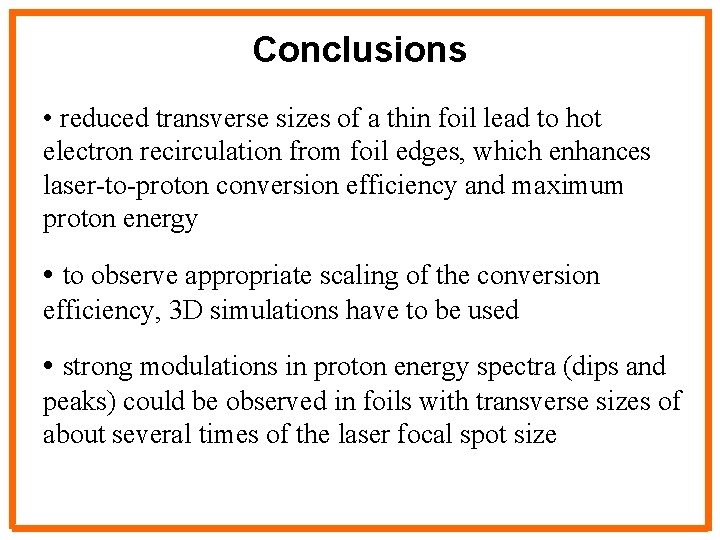
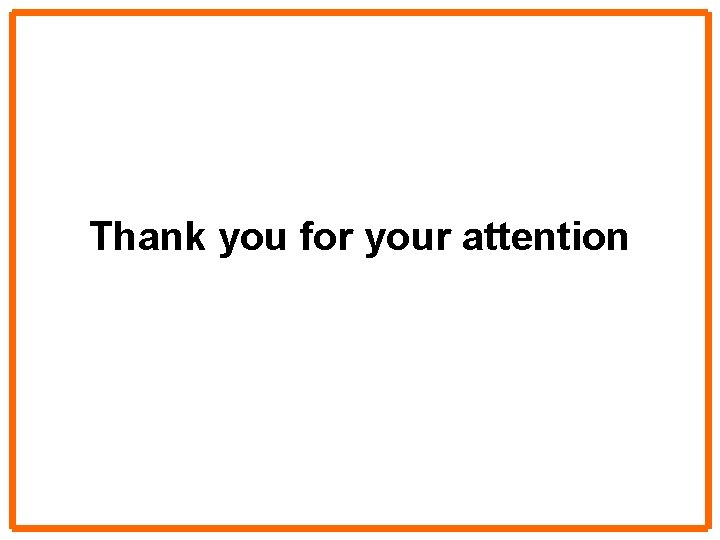
- Slides: 19
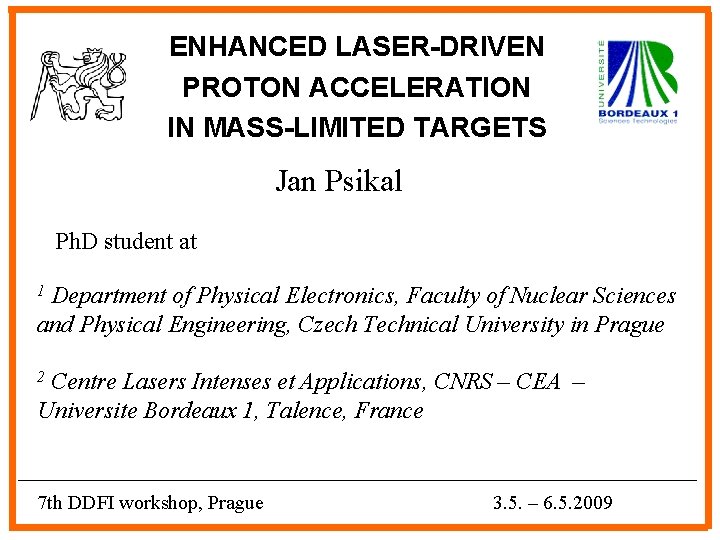
ENHANCED LASER-DRIVEN PROTON ACCELERATION IN MASS-LIMITED TARGETS Jan Psikal Ph. D student at Department of Physical Electronics, Faculty of Nuclear Sciences and Physical Engineering, Czech Technical University in Prague 1 Centre Lasers Intenses et Applications, CNRS – CEA – Universite Bordeaux 1, Talence, France 2 7 th DDFI workshop, Prague 3. 5. – 6. 5. 2009
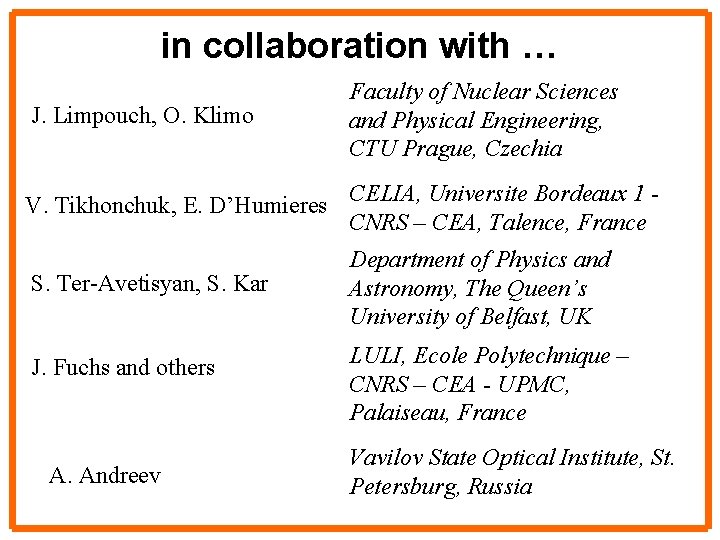
in collaboration with … J. Limpouch, O. Klimo Faculty of Nuclear Sciences and Physical Engineering, CTU Prague, Czechia V. Tikhonchuk, E. D’Humieres CELIA, Universite Bordeaux 1 CNRS – CEA, Talence, France S. Ter-Avetisyan, S. Kar J. Fuchs and others A. Andreev Department of Physics and Astronomy, The Queen’s University of Belfast, UK LULI, Ecole Polytechnique – CNRS – CEA - UPMC, Palaiseau, France Vavilov State Optical Institute, St. Petersburg, Russia
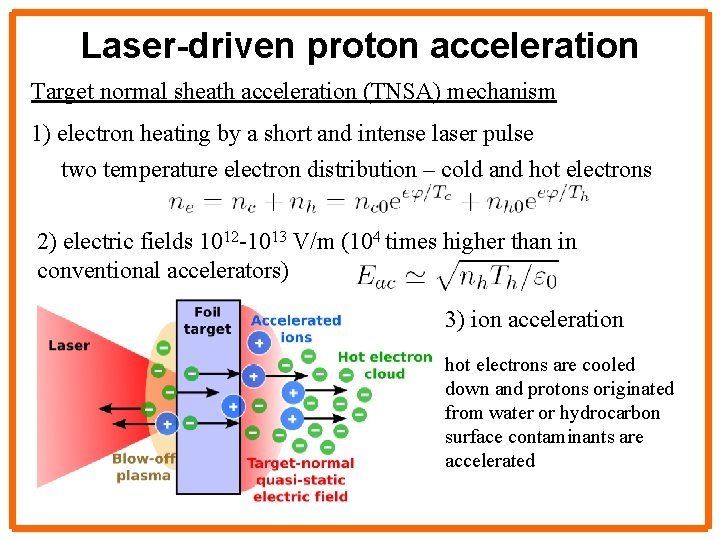
Laser-driven proton acceleration Target normal sheath acceleration (TNSA) mechanism 1) electron heating by a short and intense laser pulse two temperature electron distribution – cold and hot electrons 2) electric fields 1012 -1013 V/m (104 times higher than in conventional accelerators) 3) ion acceleration hot electrons are cooled down and protons originated from water or hydrocarbon surface contaminants are accelerated
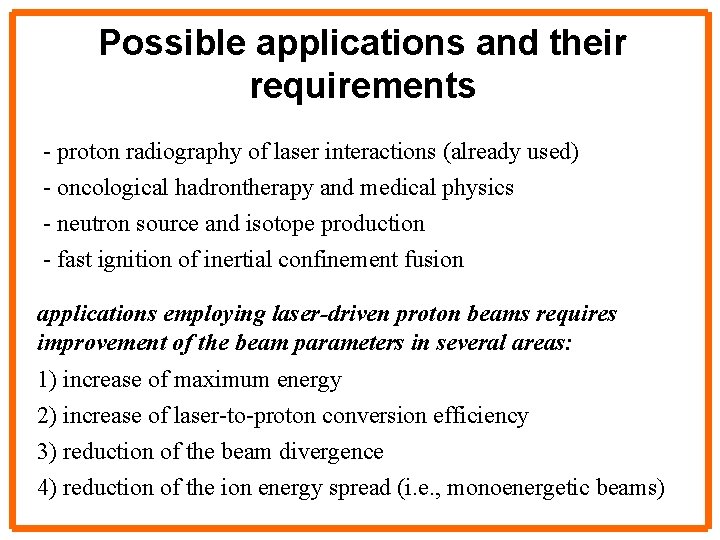
Possible applications and their requirements - proton radiography of laser interactions (already used) - oncological hadrontherapy and medical physics - neutron source and isotope production - fast ignition of inertial confinement fusion applications employing laser-driven proton beams requires improvement of the beam parameters in several areas: 1) increase of maximum energy 2) increase of laser-to-proton conversion efficiency 3) reduction of the beam divergence 4) reduction of the ion energy spread (i. e. , monoenergetic beams)
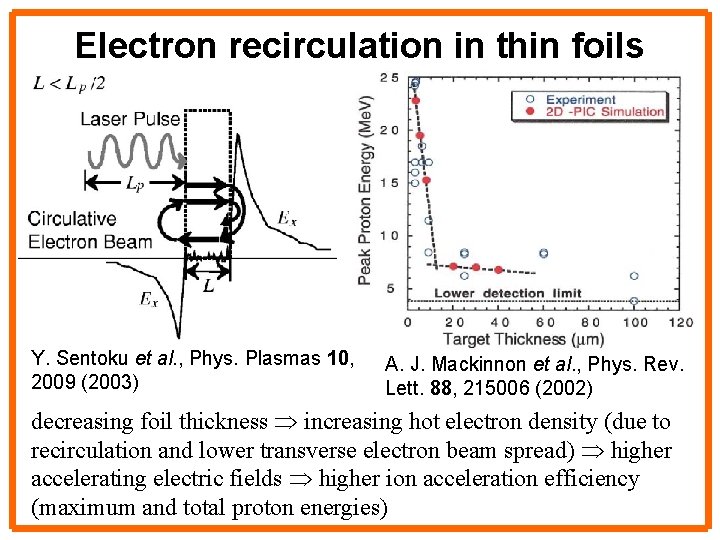
Electron recirculation in thin foils Y. Sentoku et al. , Phys. Plasmas 10, 2009 (2003) A. J. Mackinnon et al. , Phys. Rev. Lett. 88, 215006 (2002) decreasing foil thickness increasing hot electron density (due to recirculation and lower transverse electron beam spread) higher accelerating electric fields higher ion acceleration efficiency (maximum and total proton energies)
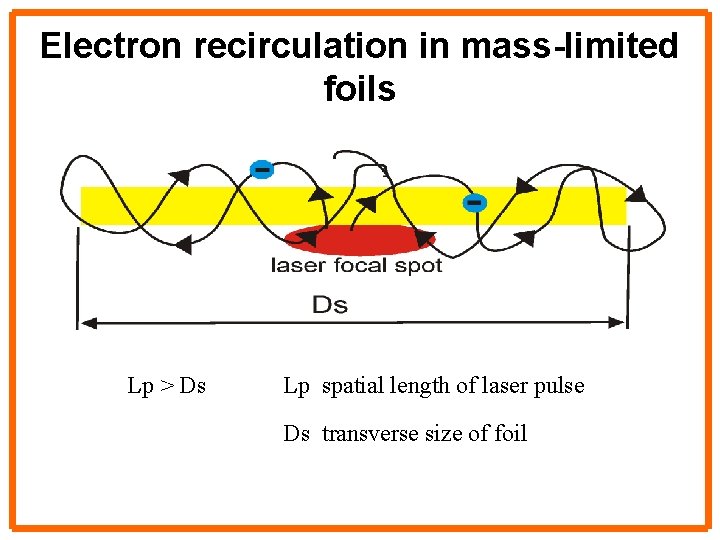
Electron recirculation in mass-limited foils Lp > Ds Lp spatial length of laser pulse Ds transverse size of foil
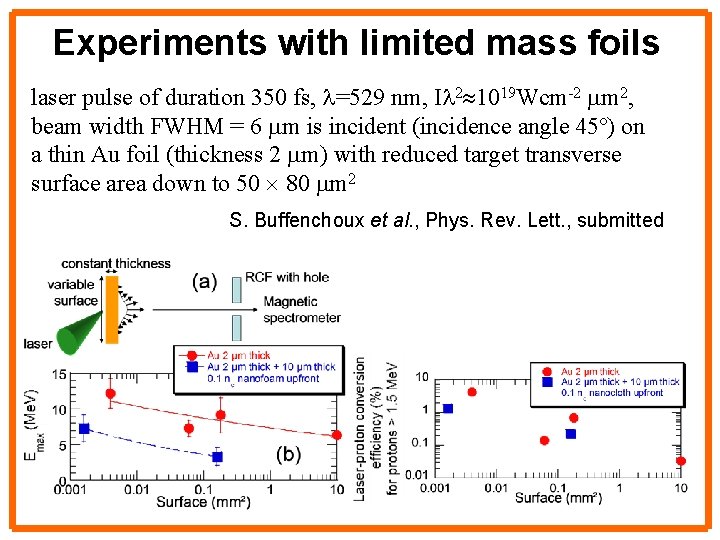
Experiments with limited mass foils laser pulse of duration 350 fs, =529 nm, I 2 1019 Wcm-2 m 2, beam width FWHM = 6 m is incident (incidence angle 45º) on a thin Au foil (thickness 2 m) with reduced target transverse surface area down to 50 80 m 2 S. Buffenchoux et al. , Phys. Rev. Lett. , submitted
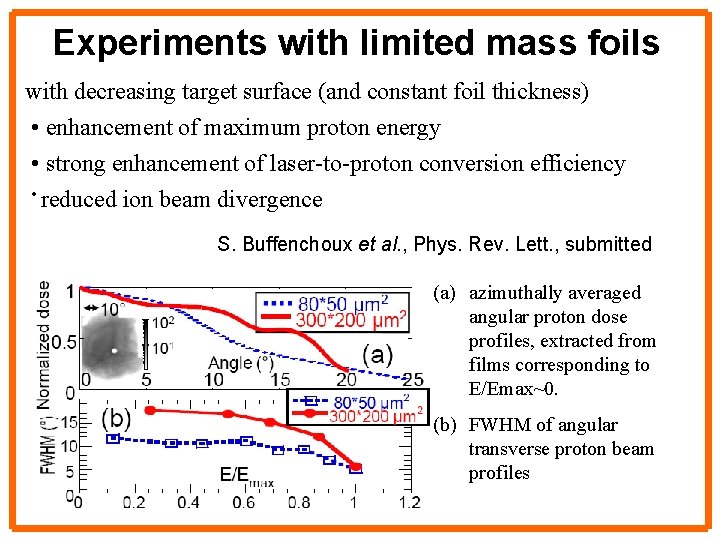
Experiments with limited mass foils with decreasing target surface (and constant foil thickness) • enhancement of maximum proton energy • strong enhancement of laser-to-proton conversion efficiency • reduced ion beam divergence S. Buffenchoux et al. , Phys. Rev. Lett. , submitted (a) azimuthally averaged angular proton dose profiles, extracted from films corresponding to E/Emax~0. (b) FWHM of angular transverse proton beam profiles
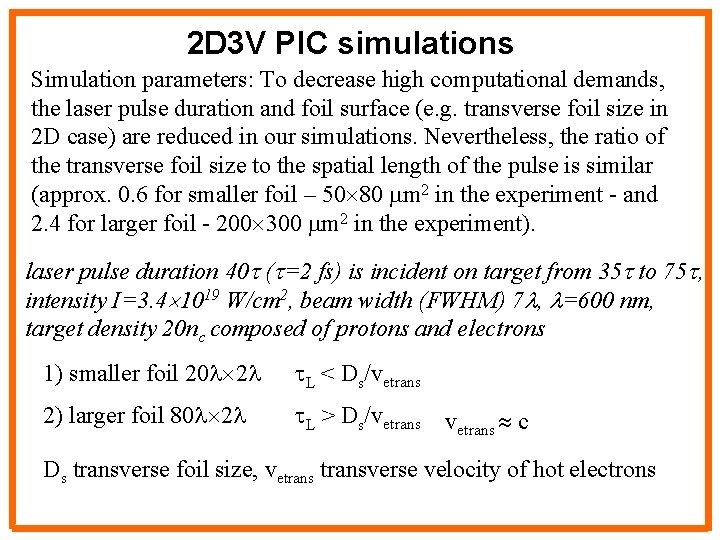
2 D 3 V PIC simulations Simulation parameters: To decrease high computational demands, the laser pulse duration and foil surface (e. g. transverse foil size in 2 D case) are reduced in our simulations. Nevertheless, the ratio of the transverse foil size to the spatial length of the pulse is similar (approx. 0. 6 for smaller foil – 50 80 m 2 in the experiment - and 2. 4 for larger foil - 200 300 m 2 in the experiment). laser pulse duration 40 ( =2 fs) is incident on target from 35 to 75 , intensity I=3. 4 1019 W/cm 2, beam width (FWHM) 7 , =600 nm, target density 20 nc composed of protons and electrons 1) smaller foil 20 2 L < Ds/vetrans 2) larger foil 80 2 L > Ds/vetrans c Ds transverse foil size, vetransverse velocity of hot electrons
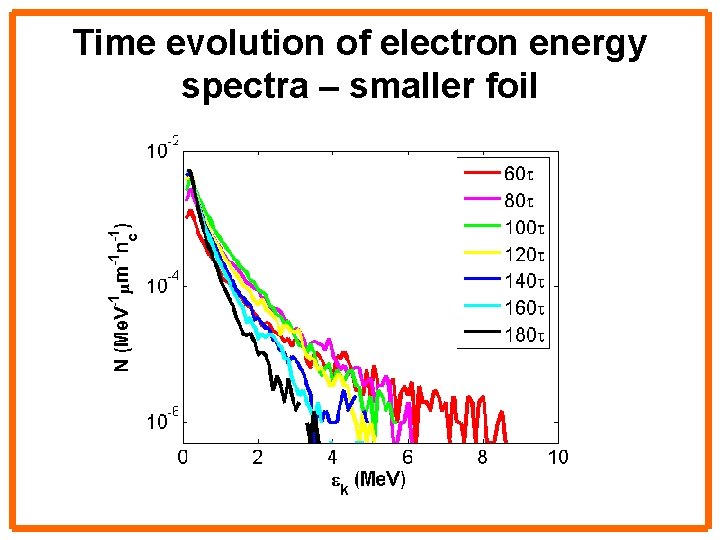
Time evolution of electron energy spectra – smaller foil
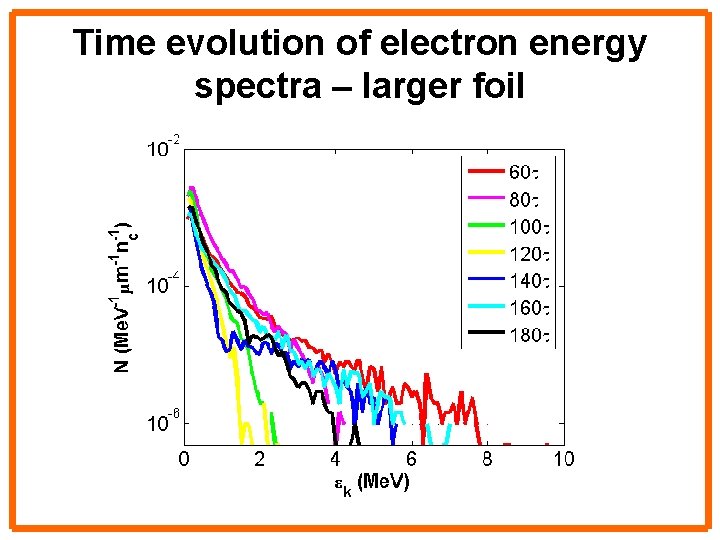
Time evolution of electron energy spectra – larger foil
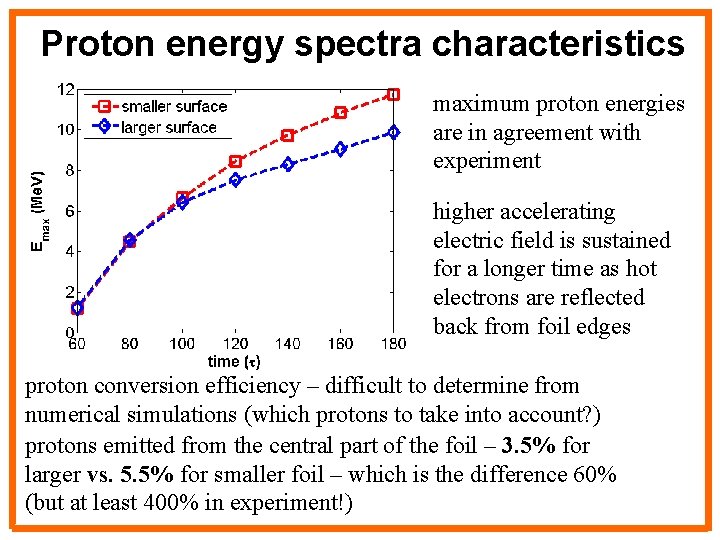
Proton energy spectra characteristics maximum proton energies are in agreement with experiment higher accelerating electric field is sustained for a longer time as hot electrons are reflected back from foil edges proton conversion efficiency – difficult to determine from numerical simulations (which protons to take into account? ) protons emitted from the central part of the foil – 3. 5% for larger vs. 5. 5% for smaller foil – which is the difference 60% (but at least 400% in experiment!)
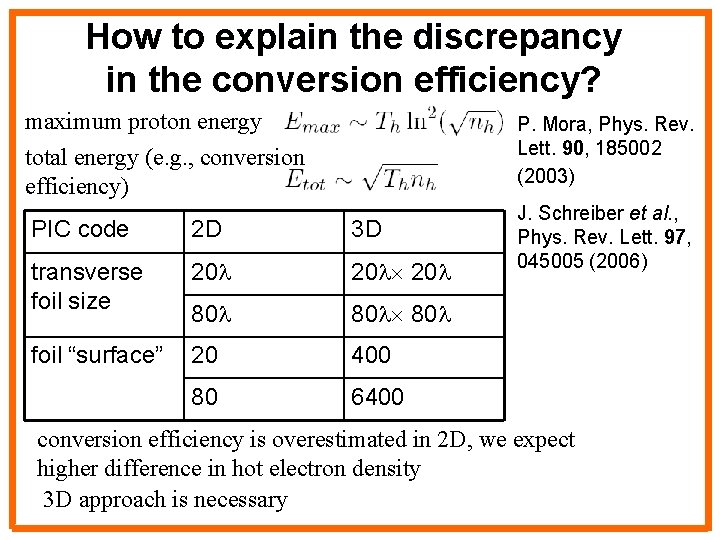
How to explain the discrepancy in the conversion efficiency? maximum proton energy P. Mora, Phys. Rev. Lett. 90, 185002 (2003) total energy (e. g. , conversion efficiency) PIC code 2 D 3 D transverse foil size 20 20 80 80 foil “surface” 20 400 80 6400 J. Schreiber et al. , Phys. Rev. Lett. 97, 045005 (2006) conversion efficiency is overestimated in 2 D, we expect higher difference in hot electron density 3 D approach is necessary
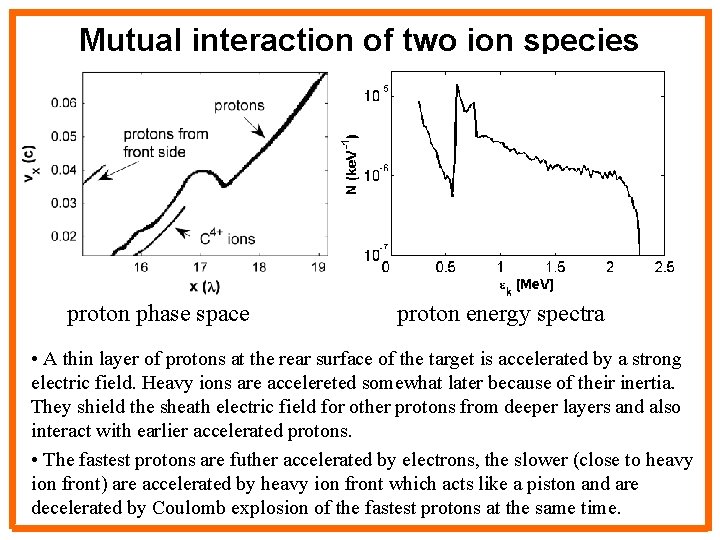
Mutual interaction of two ion species proton phase space proton energy spectra • A thin layer of protons at the rear surface of the target is accelerated by a strong electric field. Heavy ions are accelereted somewhat later because of their inertia. They shield the sheath electric field for other protons from deeper layers and also interact with earlier accelerated protons. • The fastest protons are futher accelerated by electrons, the slower (close to heavy ion front) are accelerated by heavy ion front which acts like a piston and are decelerated by Coulomb explosion of the fastest protons at the same time.
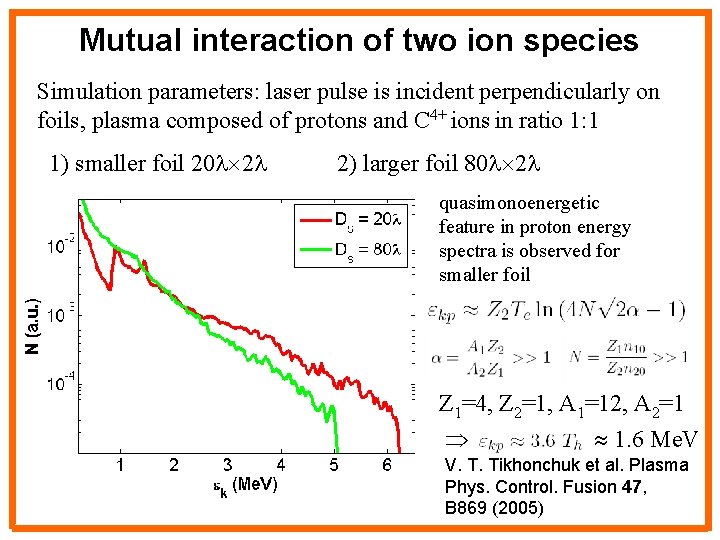
Mutual interaction of two ion species Simulation parameters: laser pulse is incident perpendicularly on foils, plasma composed of protons and C 4+ ions in ratio 1: 1 1) smaller foil 20 2 2) larger foil 80 2 quasimonoenergetic feature in proton energy spectra is observed for smaller foil Z 1=4, Z 2=1, A 1=12, A 2=1 1. 6 Me. V V. T. Tikhonchuk et al. Plasma Phys. Control. Fusion 47, B 869 (2005)
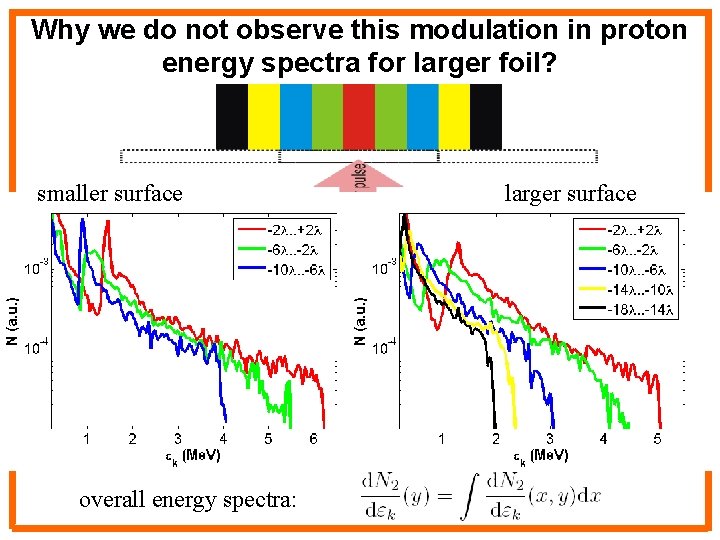
Why we do not observe this modulation in proton energy spectra for larger foil? smaller surface overall energy spectra: larger surface
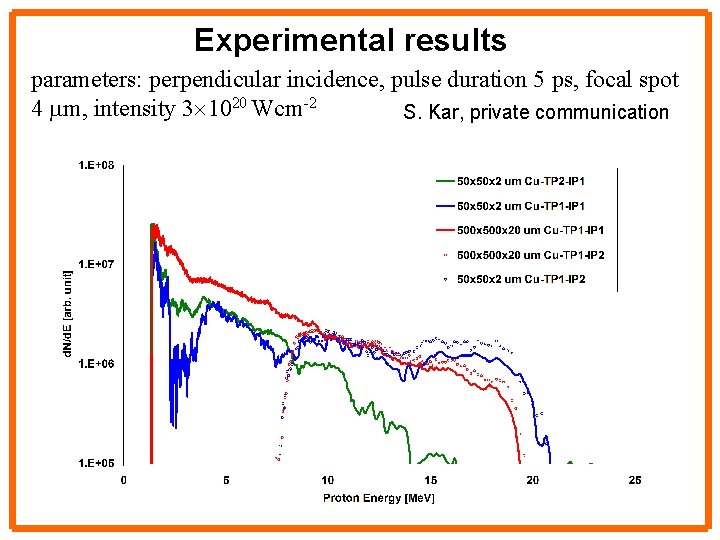
Experimental results parameters: perpendicular incidence, pulse duration 5 ps, focal spot 4 m, intensity 3 1020 Wcm-2 S. Kar, private communication
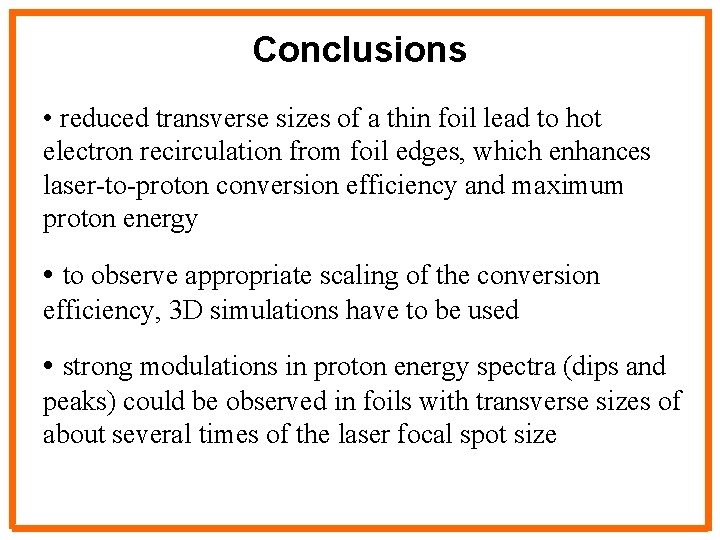
Conclusions • reduced transverse sizes of a thin foil lead to hot electron recirculation from foil edges, which enhances laser-to-proton conversion efficiency and maximum proton energy • to observe appropriate scaling of the conversion efficiency, 3 D simulations have to be used • strong modulations in proton energy spectra (dips and peaks) could be observed in foils with transverse sizes of about several times of the laser focal spot size
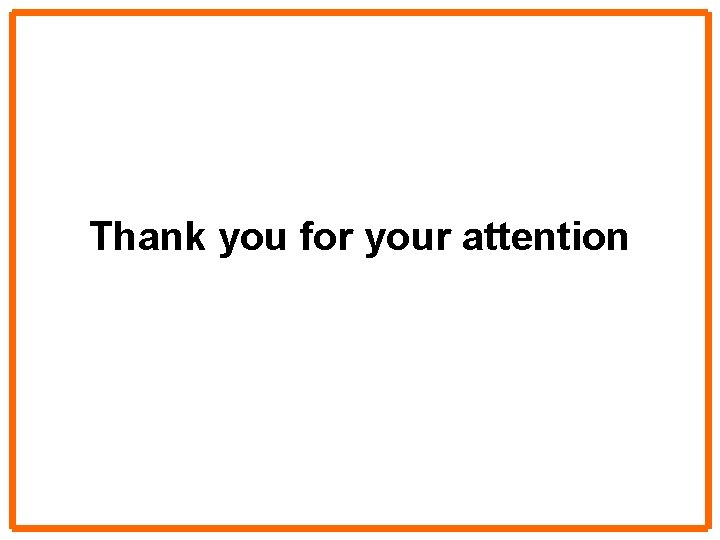
Thank you for your attention
Proton proton chain
Proton proton chain
Nuclear fission
Linear acceleration vs tangential acceleration
Solar eclipse accel
Centripetal acceleration tangential acceleration
Angular vs linear velocity
Is radial acceleration the same as centripetal acceleration
Finished sketch
Segment by segment invasion
Writing learning targets
Europe 2020 targets
Nyseslat proficiency levels
Smart target gcse pe
How to restart iptables
Five patterns of target market selection
Learning target examples math
Parathyroid gland chief cell
Jim crow laws in what region or regions did it exist
Europe 2020 targets