EE 3321 Electromagnetic Field Theory Potential Energy Energy
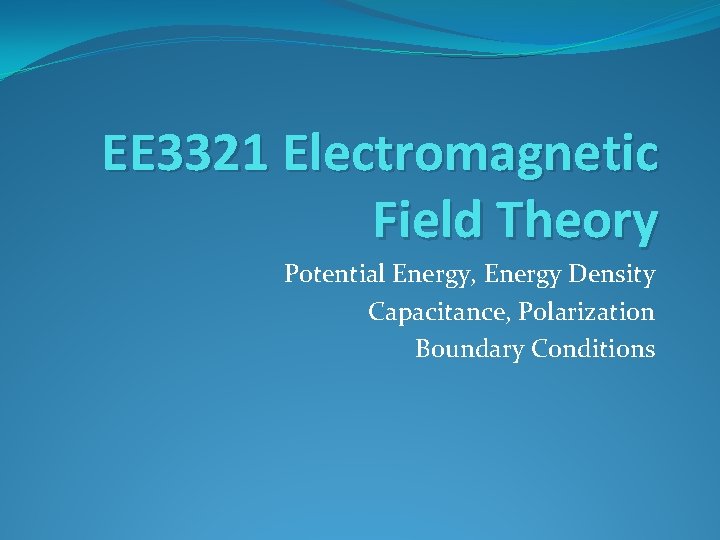
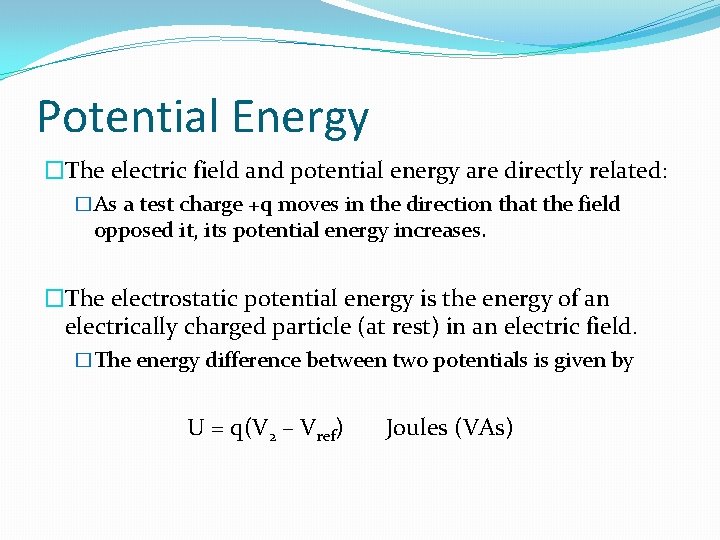
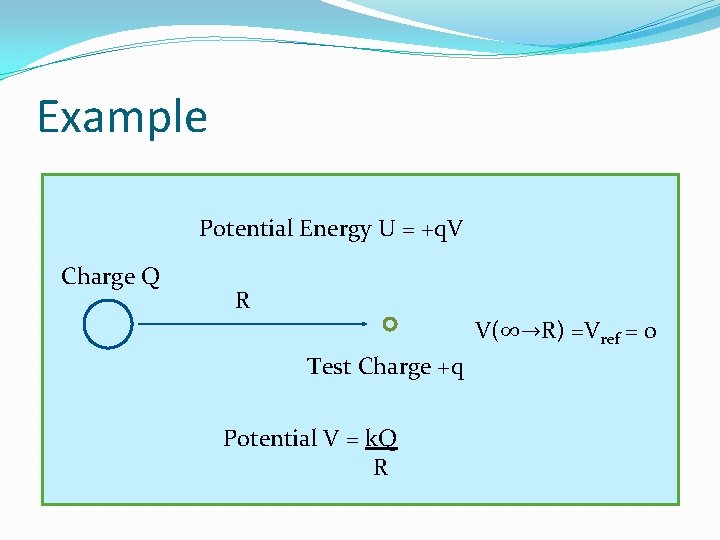
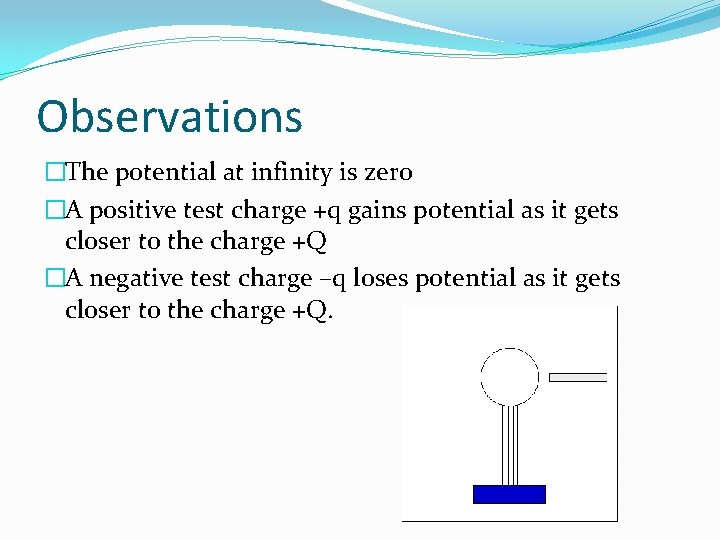
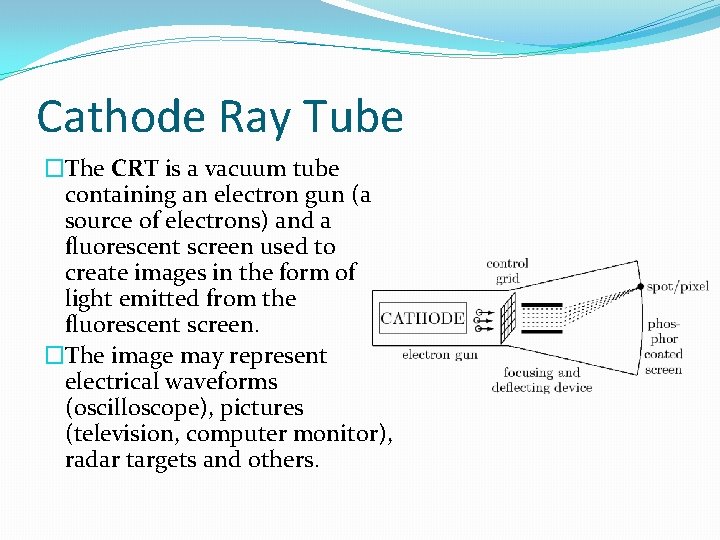
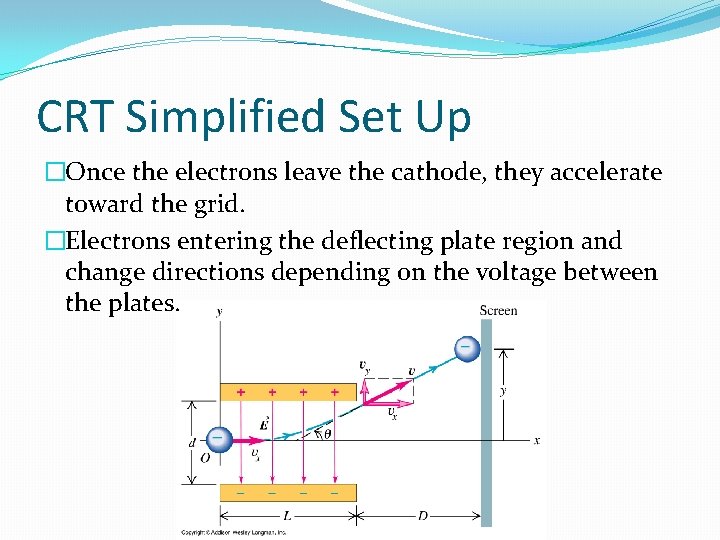
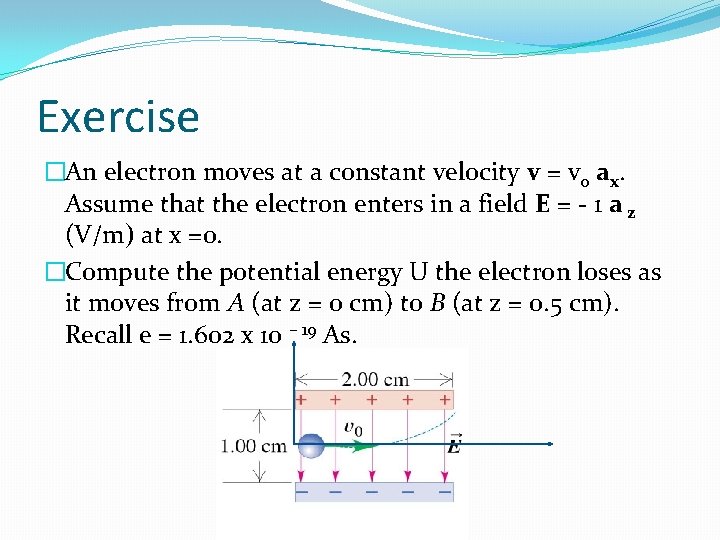
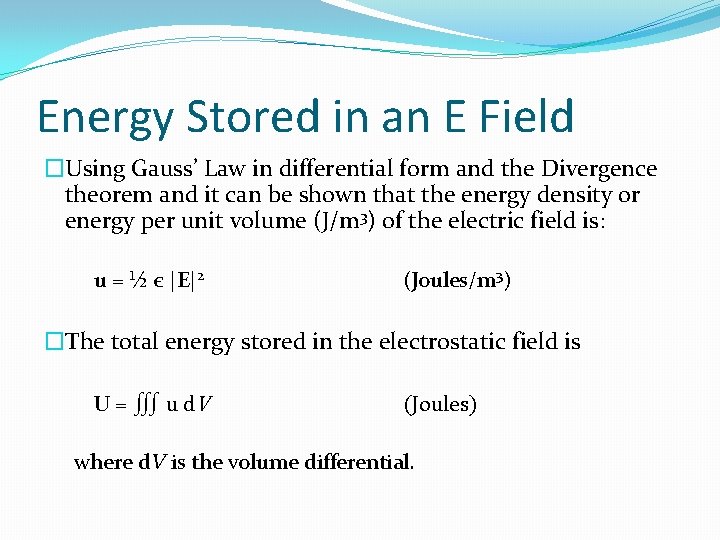
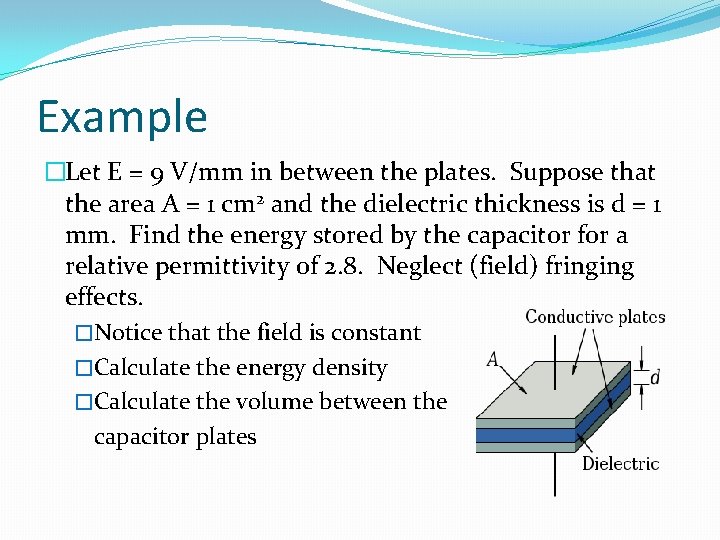
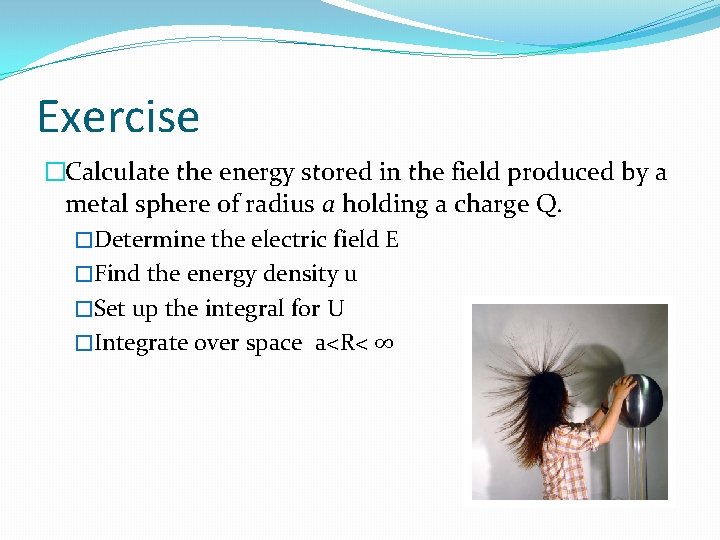
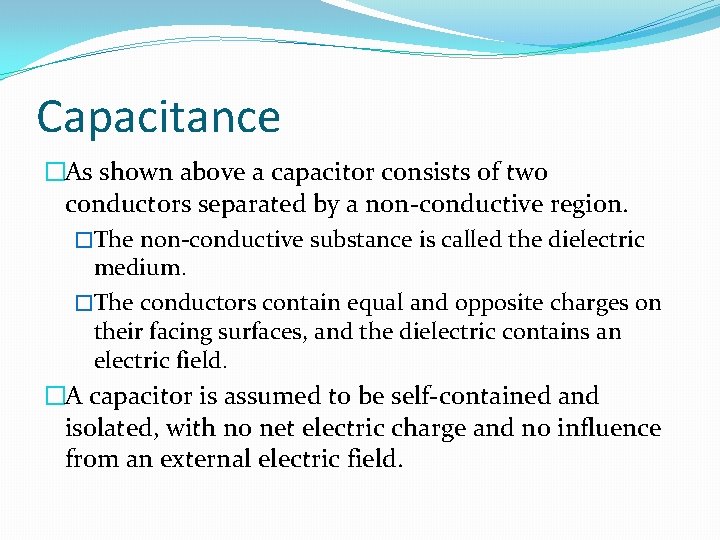
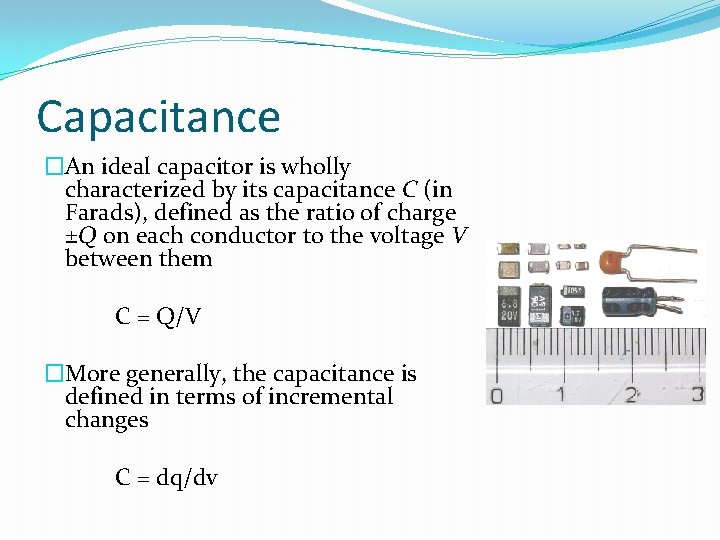
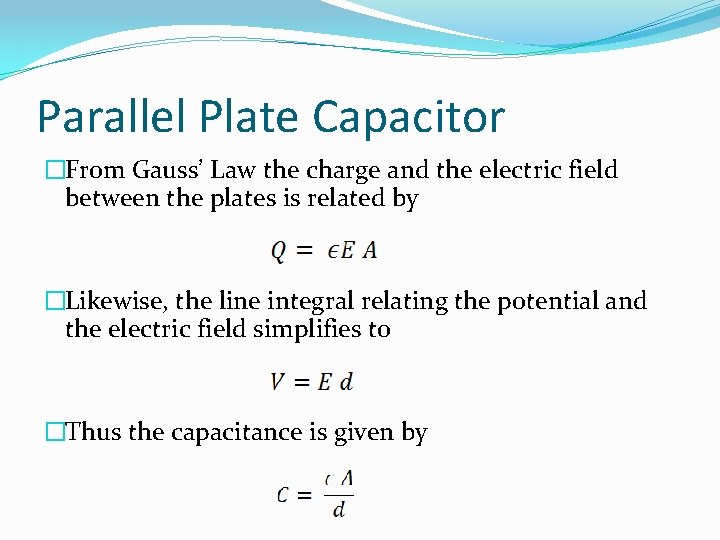
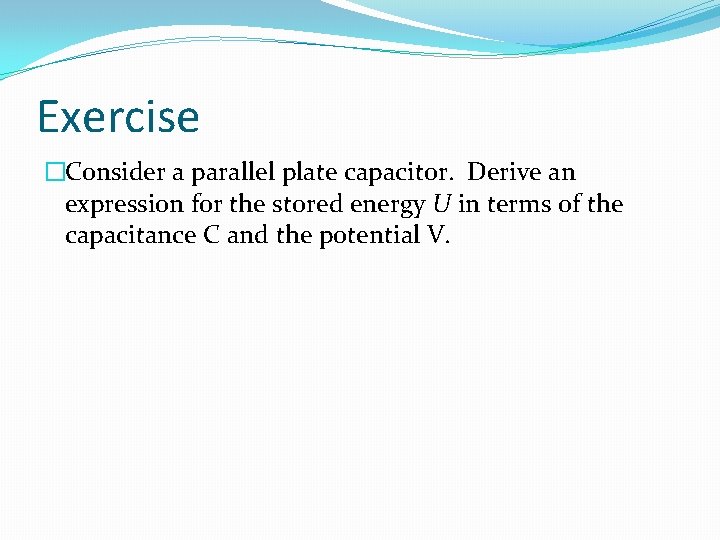
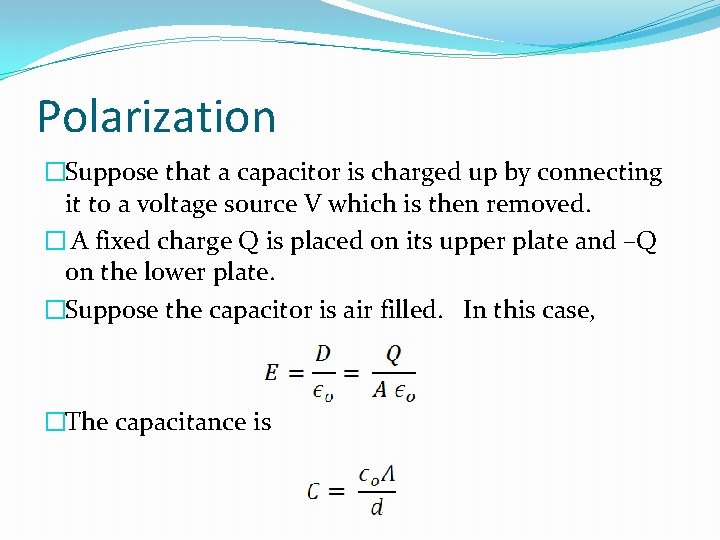
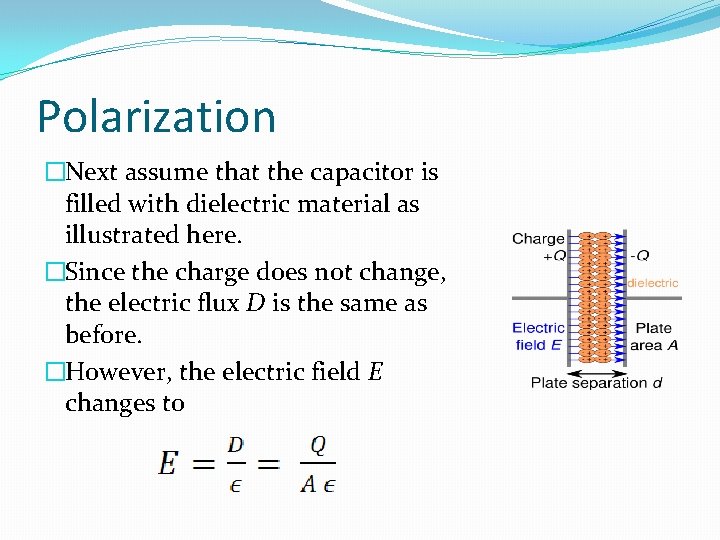
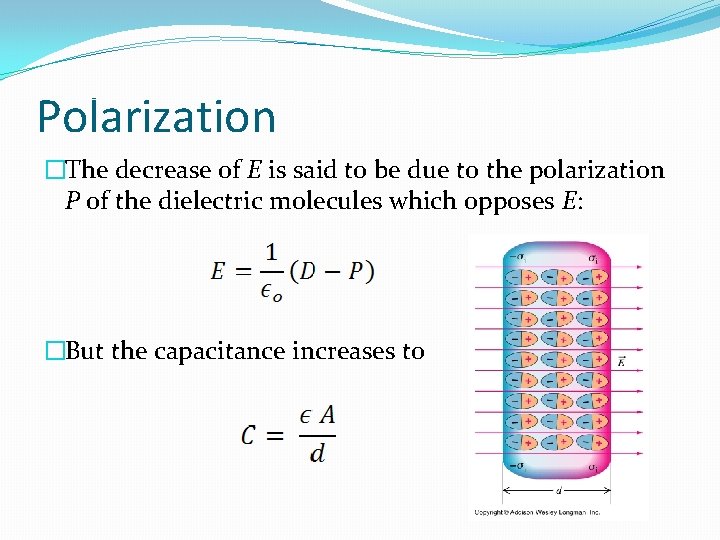
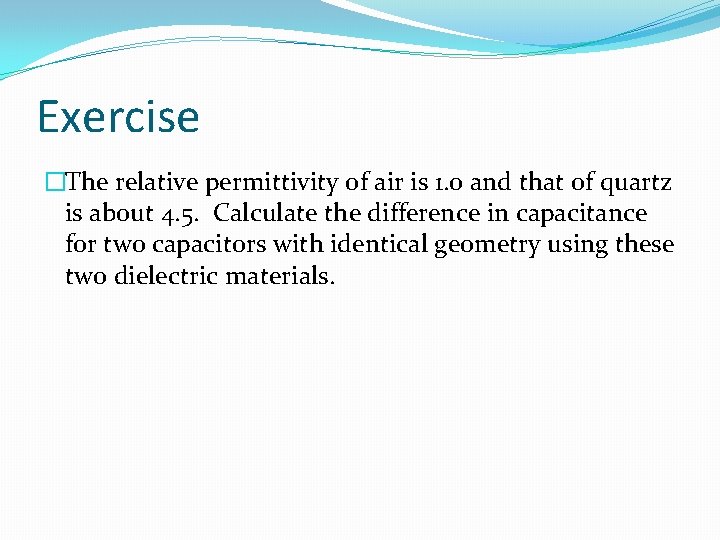
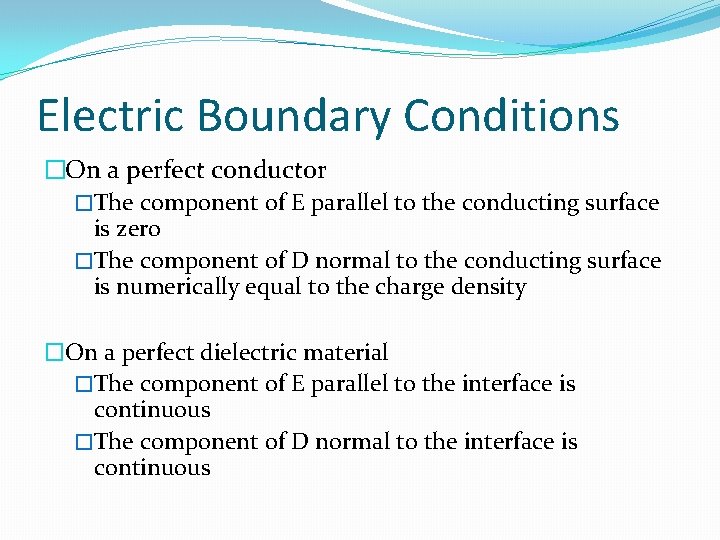
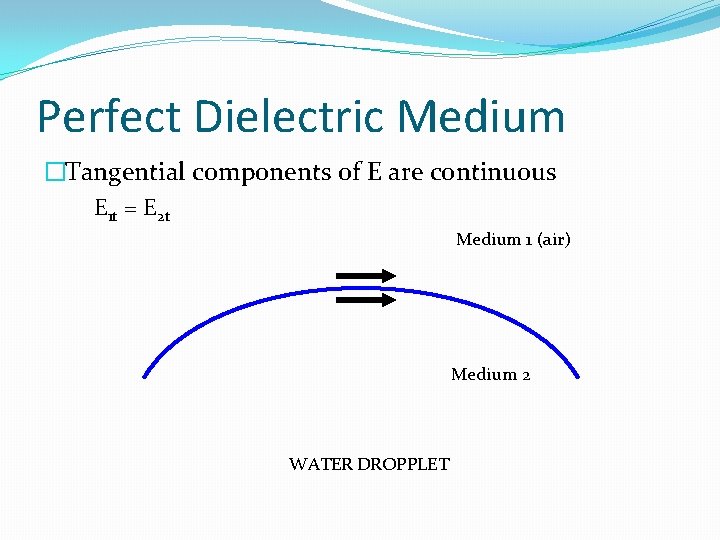
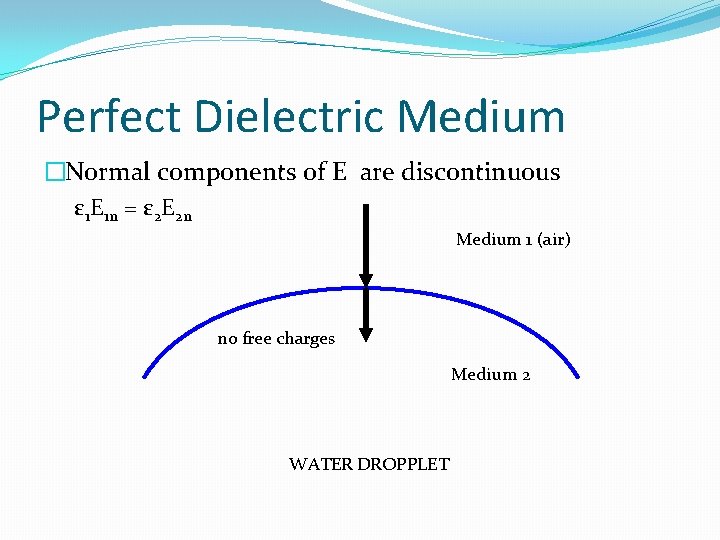
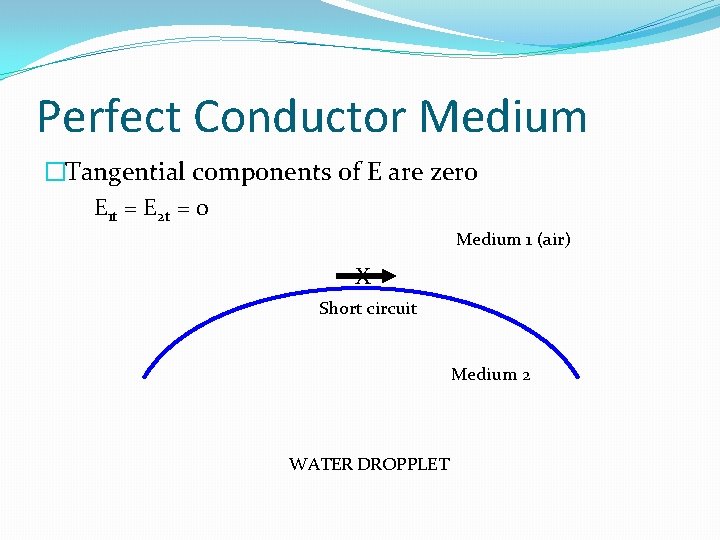
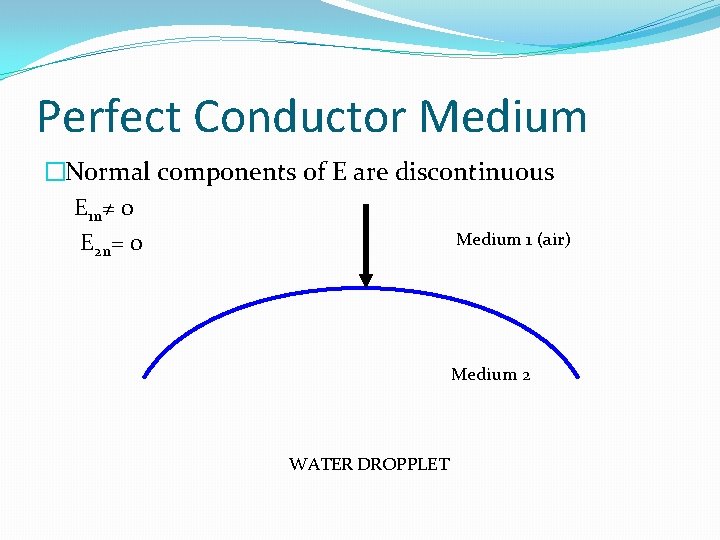
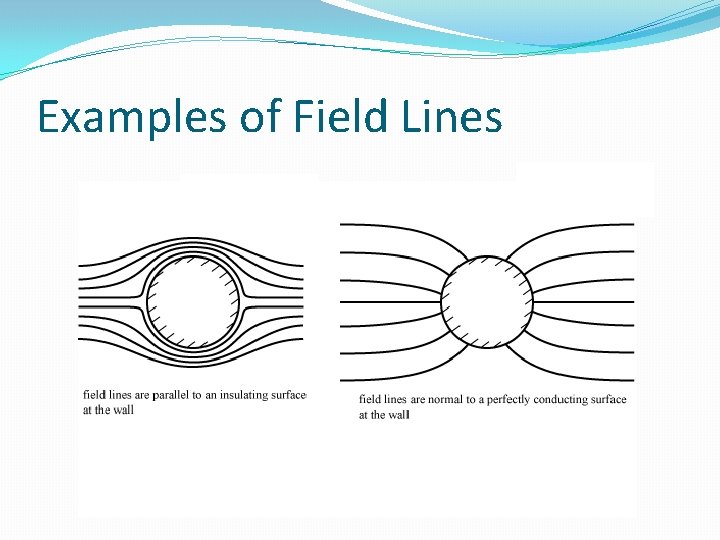
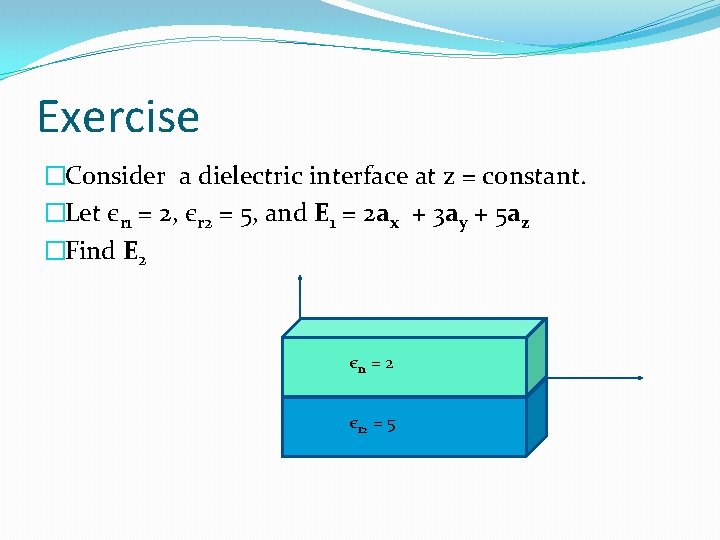
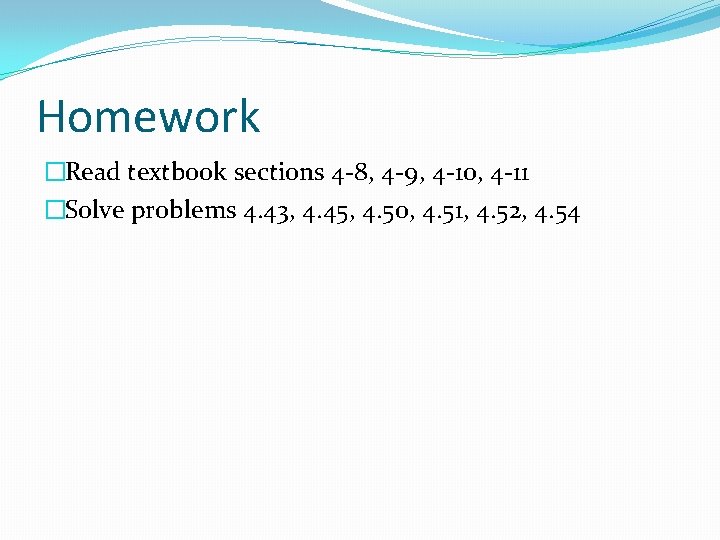
- Slides: 26
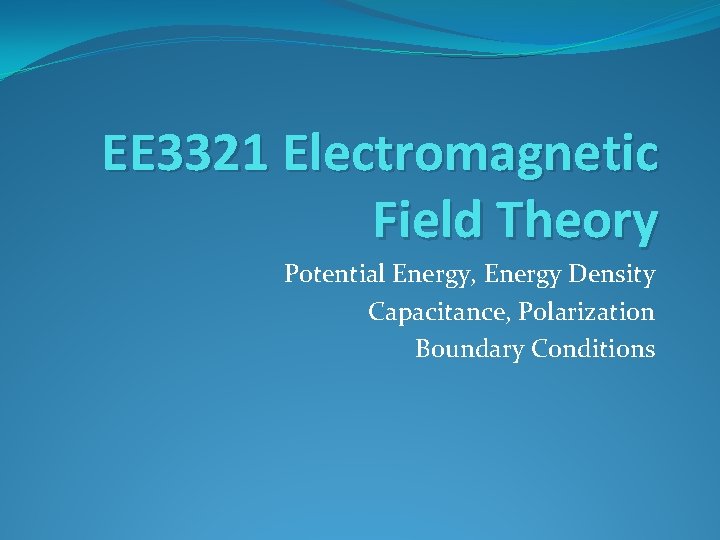
EE 3321 Electromagnetic Field Theory Potential Energy, Energy Density Capacitance, Polarization Boundary Conditions
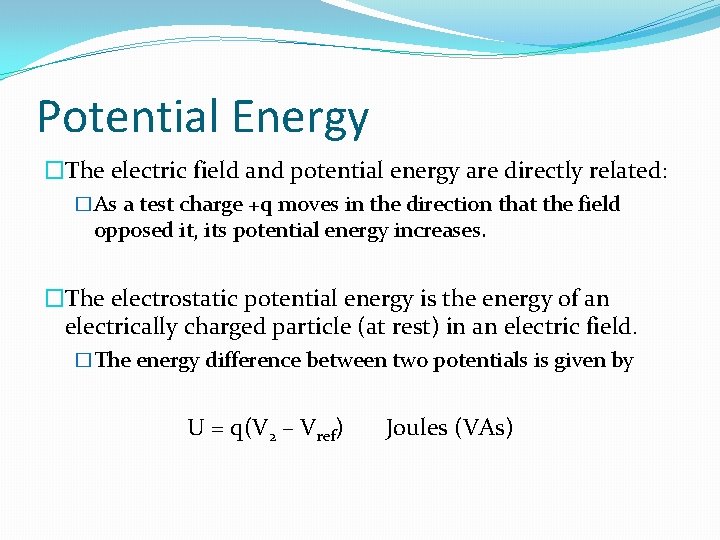
Potential Energy �The electric field and potential energy are directly related: �As a test charge +q moves in the direction that the field opposed it, its potential energy increases. �The electrostatic potential energy is the energy of an electrically charged particle (at rest) in an electric field. �The energy difference between two potentials is given by U = q(V 2 – Vref) Joules (VAs)
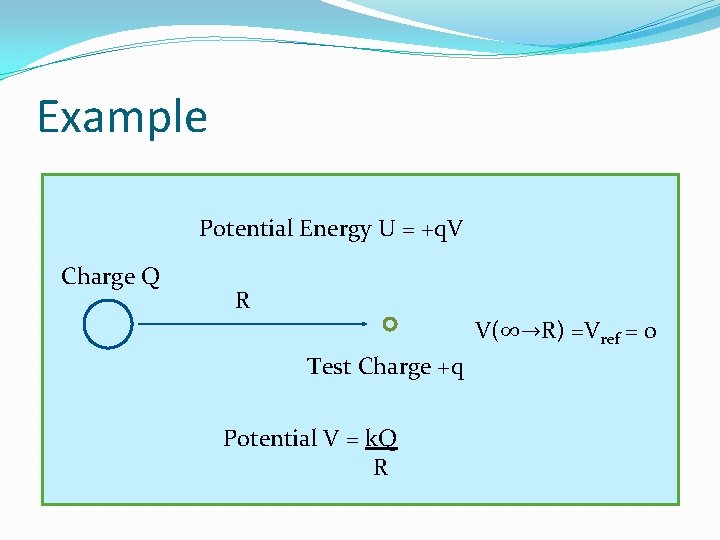
Example Potential Energy U = +q. V Charge Q R V(∞→R) =Vref = 0 Test Charge +q Potential V = k. Q R
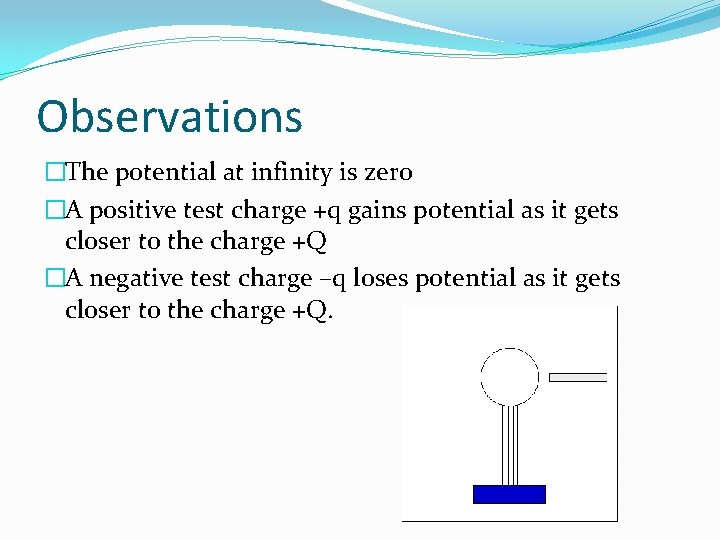
Observations �The potential at infinity is zero �A positive test charge +q gains potential as it gets closer to the charge +Q �A negative test charge –q loses potential as it gets closer to the charge +Q.
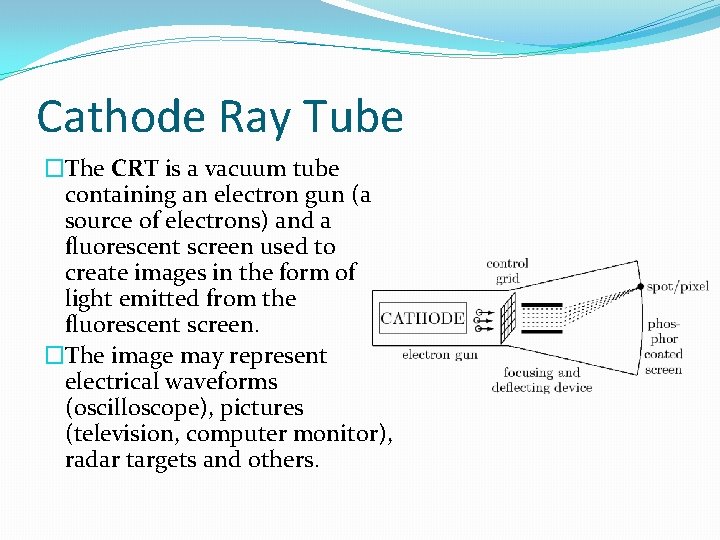
Cathode Ray Tube �The CRT is a vacuum tube containing an electron gun (a source of electrons) and a fluorescent screen used to create images in the form of light emitted from the fluorescent screen. �The image may represent electrical waveforms (oscilloscope), pictures (television, computer monitor), radar targets and others.
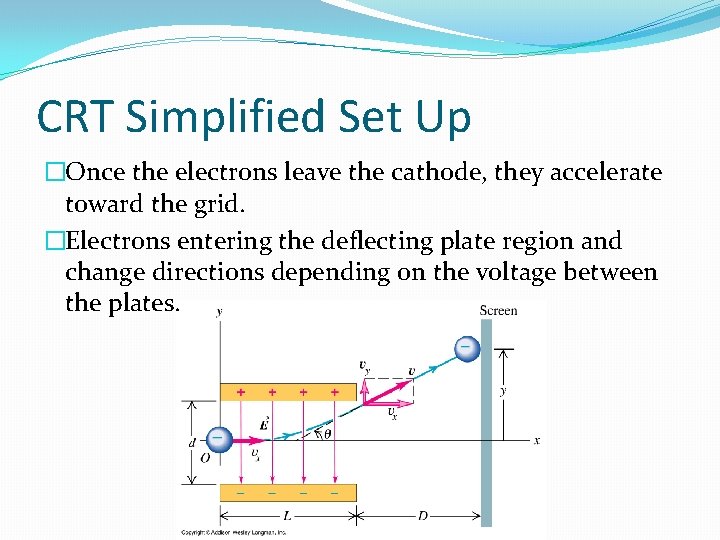
CRT Simplified Set Up �Once the electrons leave the cathode, they accelerate toward the grid. �Electrons entering the deflecting plate region and change directions depending on the voltage between the plates.
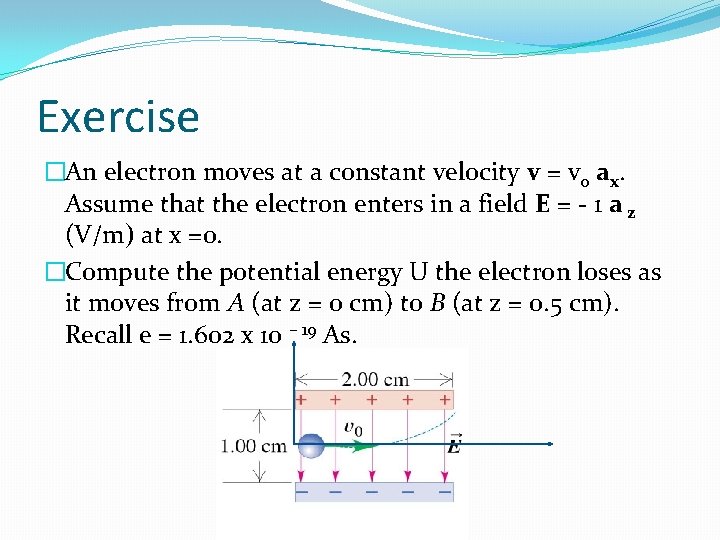
Exercise �An electron moves at a constant velocity v = vo ax. Assume that the electron enters in a field E = - 1 a z (V/m) at x =0. �Compute the potential energy U the electron loses as it moves from A (at z = 0 cm) to B (at z = 0. 5 cm). Recall e = 1. 602 x 10 – 19 As.
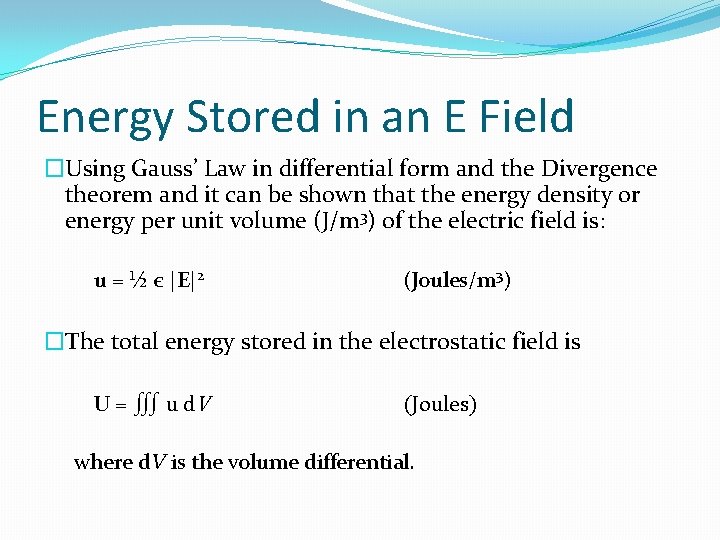
Energy Stored in an E Field �Using Gauss’ Law in differential form and the Divergence theorem and it can be shown that the energy density or energy per unit volume (J/m 3) of the electric field is: u = ½ є |E|2 (Joules/m 3) �The total energy stored in the electrostatic field is U = ∭ u d. V (Joules) where d. V is the volume differential.
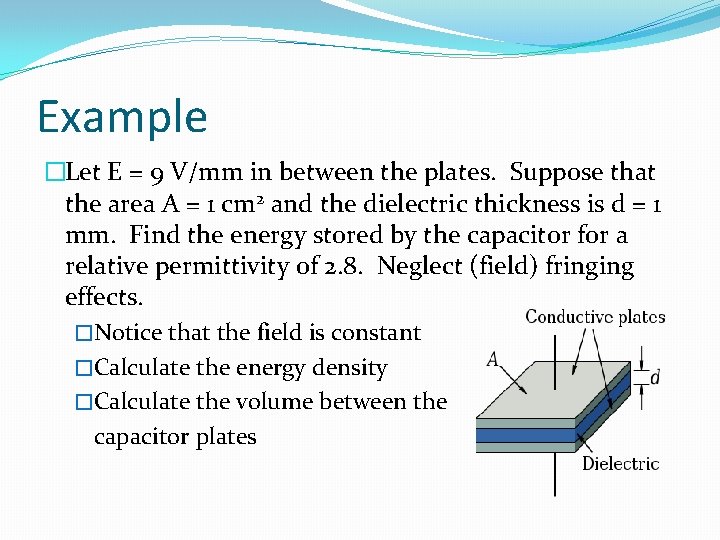
Example �Let E = 9 V/mm in between the plates. Suppose that the area A = 1 cm 2 and the dielectric thickness is d = 1 mm. Find the energy stored by the capacitor for a relative permittivity of 2. 8. Neglect (field) fringing effects. �Notice that the field is constant �Calculate the energy density �Calculate the volume between the capacitor plates
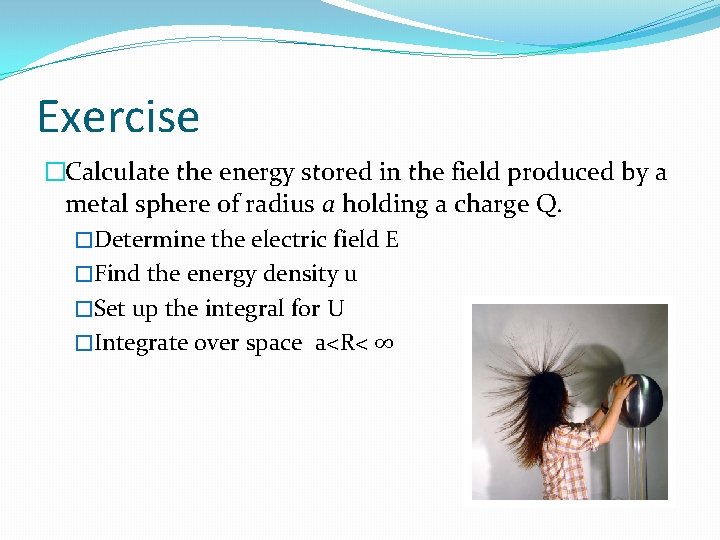
Exercise �Calculate the energy stored in the field produced by a metal sphere of radius a holding a charge Q. �Determine the electric field E �Find the energy density u �Set up the integral for U �Integrate over space a<R< ∞
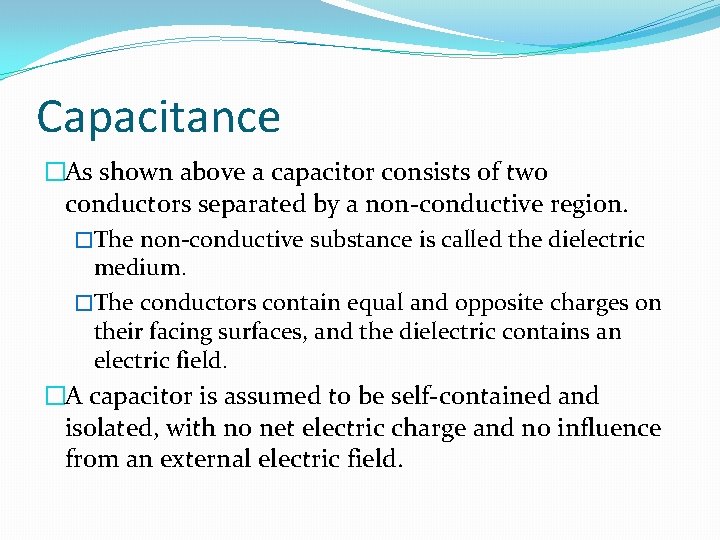
Capacitance �As shown above a capacitor consists of two conductors separated by a non-conductive region. �The non-conductive substance is called the dielectric medium. �The conductors contain equal and opposite charges on their facing surfaces, and the dielectric contains an electric field. �A capacitor is assumed to be self-contained and isolated, with no net electric charge and no influence from an external electric field.
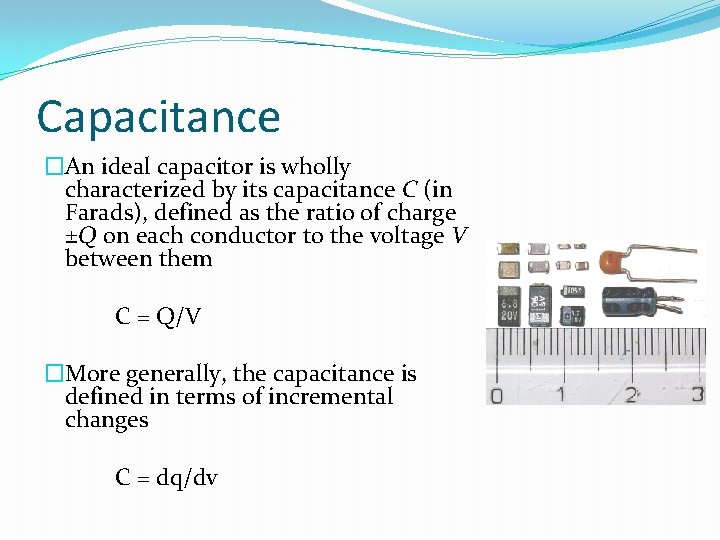
Capacitance �An ideal capacitor is wholly characterized by its capacitance C (in Farads), defined as the ratio of charge ±Q on each conductor to the voltage V between them C = Q/V �More generally, the capacitance is defined in terms of incremental changes C = dq/dv
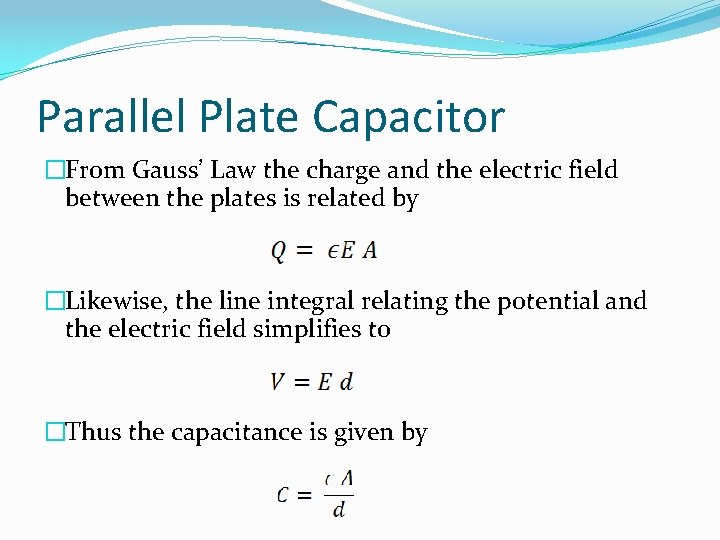
Parallel Plate Capacitor �From Gauss’ Law the charge and the electric field between the plates is related by �Likewise, the line integral relating the potential and the electric field simplifies to �Thus the capacitance is given by
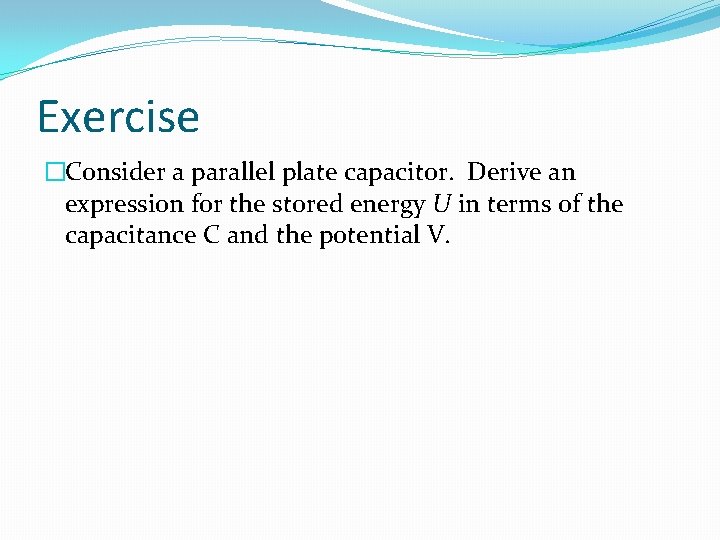
Exercise �Consider a parallel plate capacitor. Derive an expression for the stored energy U in terms of the capacitance C and the potential V.
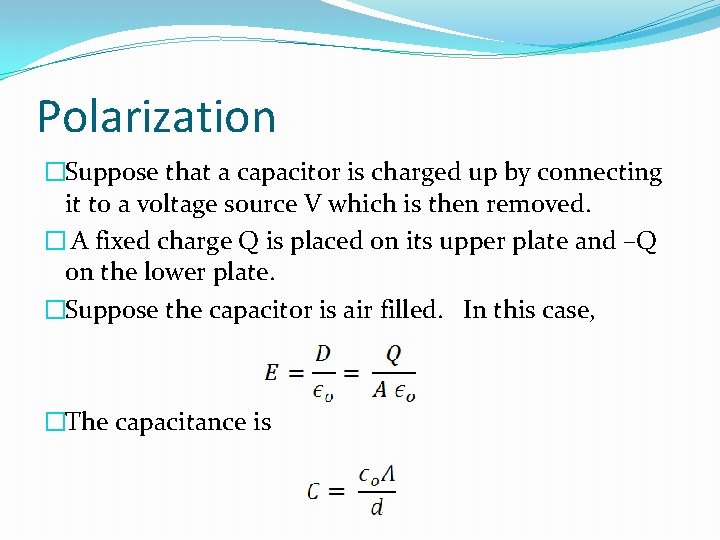
Polarization �Suppose that a capacitor is charged up by connecting it to a voltage source V which is then removed. � A fixed charge Q is placed on its upper plate and –Q on the lower plate. �Suppose the capacitor is air filled. In this case, �The capacitance is
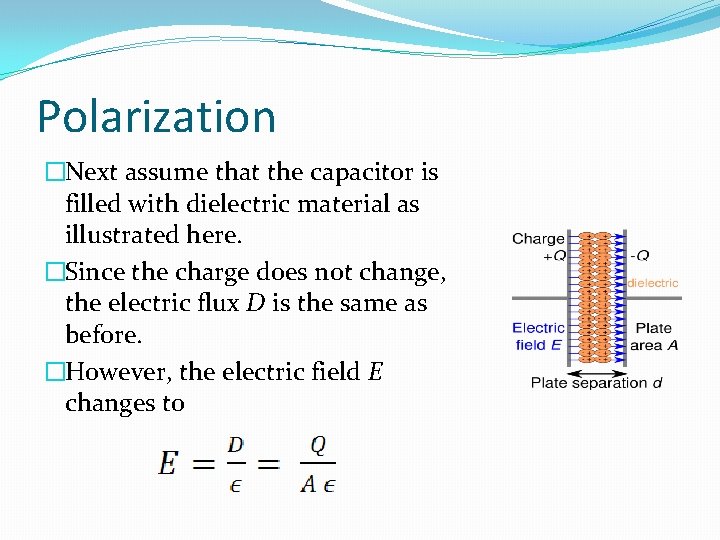
Polarization �Next assume that the capacitor is filled with dielectric material as illustrated here. �Since the charge does not change, the electric flux D is the same as before. �However, the electric field E changes to
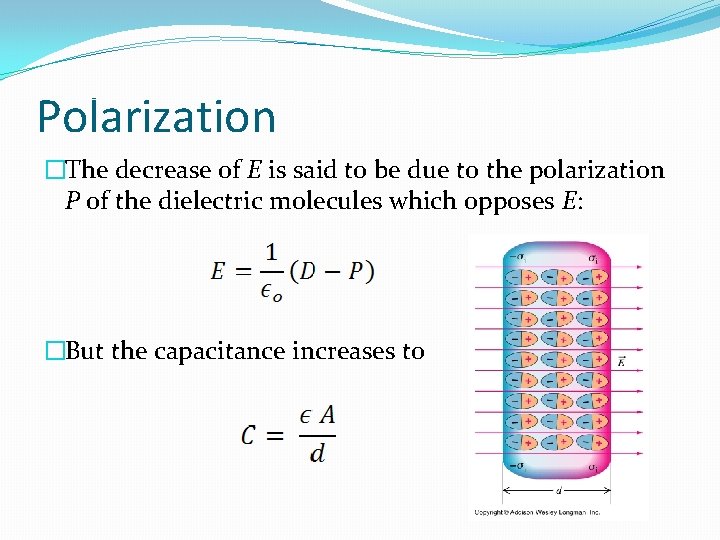
Polarization �The decrease of E is said to be due to the polarization P of the dielectric molecules which opposes E: �But the capacitance increases to
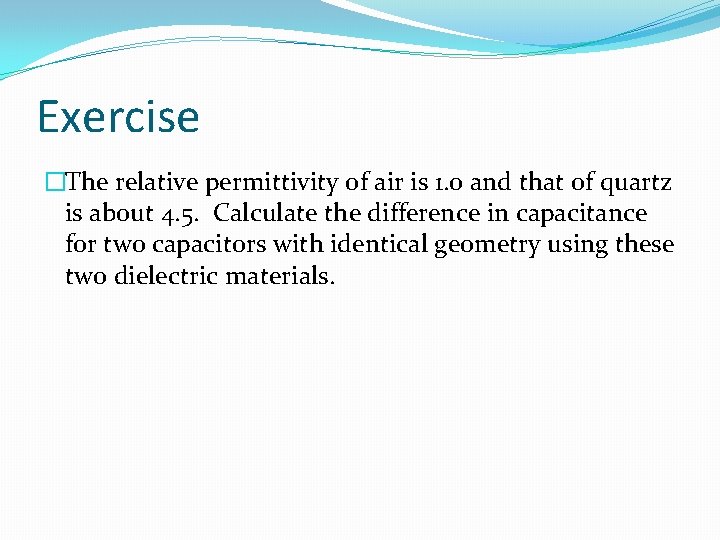
Exercise �The relative permittivity of air is 1. 0 and that of quartz is about 4. 5. Calculate the difference in capacitance for two capacitors with identical geometry using these two dielectric materials.
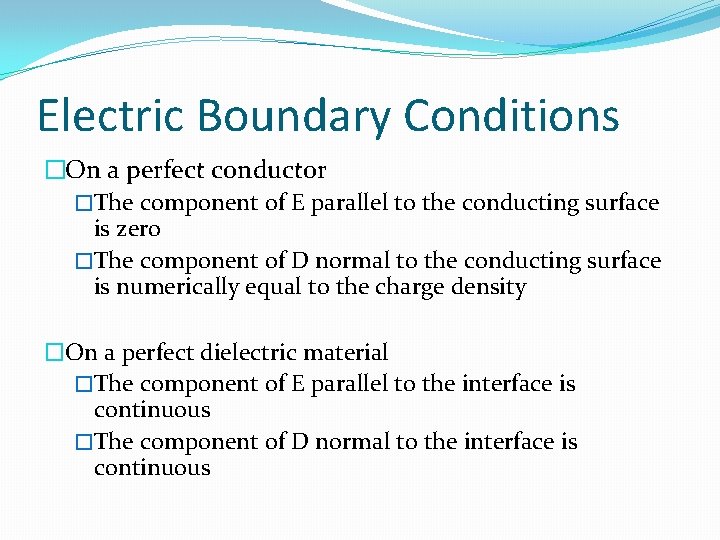
Electric Boundary Conditions �On a perfect conductor �The component of E parallel to the conducting surface is zero �The component of D normal to the conducting surface is numerically equal to the charge density �On a perfect dielectric material �The component of E parallel to the interface is continuous �The component of D normal to the interface is continuous
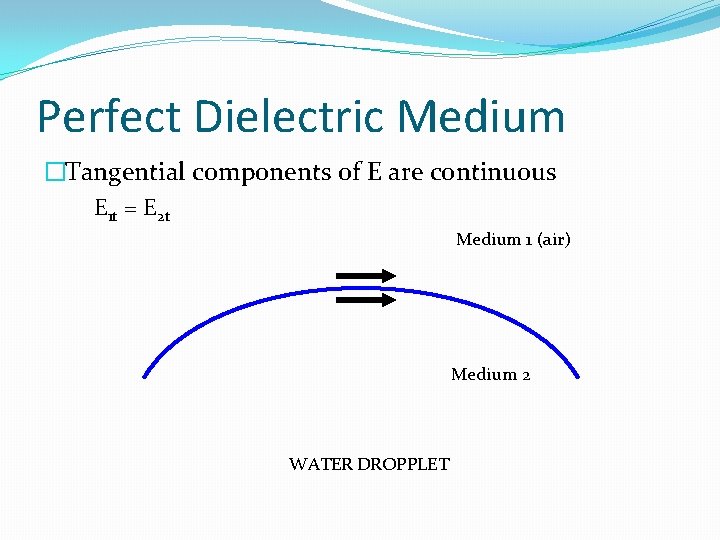
Perfect Dielectric Medium �Tangential components of E are continuous E 1 t = E 2 t Medium 1 (air) Medium 2 WATER DROPPLET
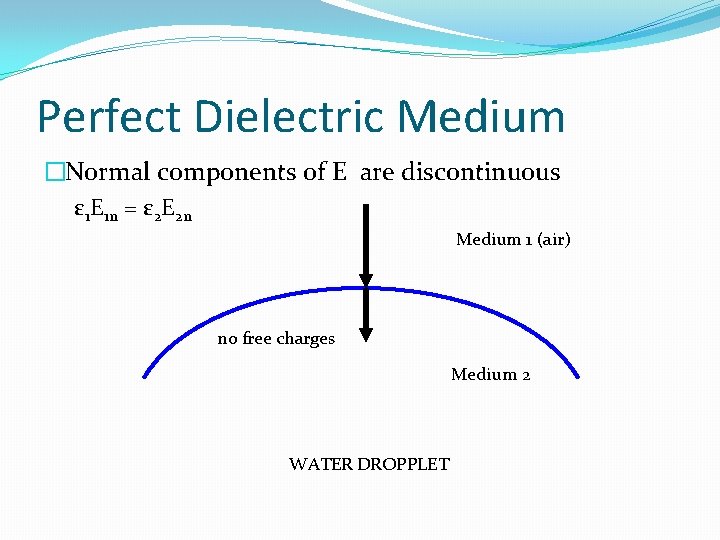
Perfect Dielectric Medium �Normal components of E are discontinuous ε 1 E 1 n = ε 2 E 2 n Medium 1 (air) no free charges Medium 2 WATER DROPPLET
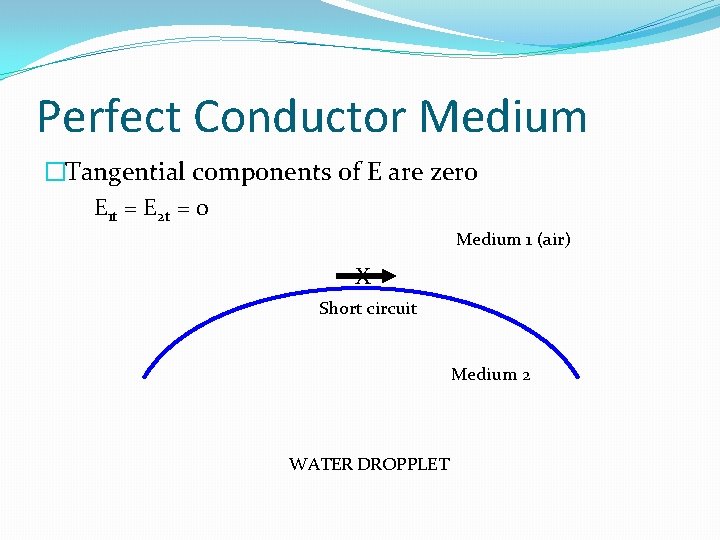
Perfect Conductor Medium �Tangential components of E are zero E 1 t = E 2 t = 0 Medium 1 (air) X Short circuit Medium 2 WATER DROPPLET
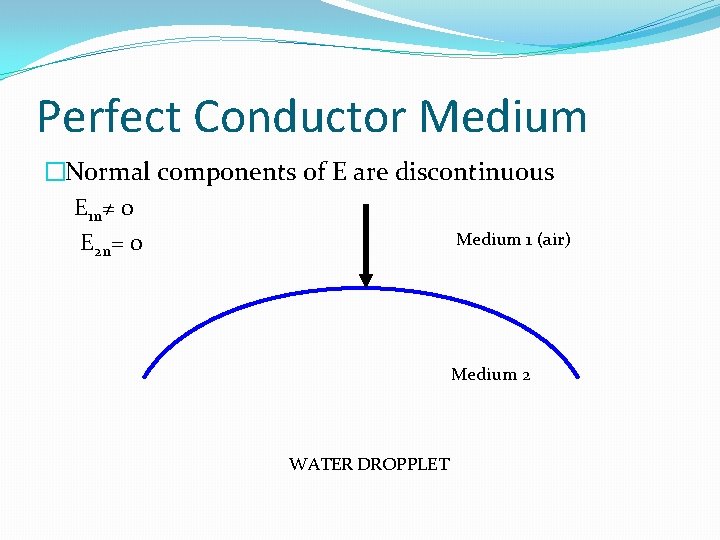
Perfect Conductor Medium �Normal components of E are discontinuous E 1 n≠ 0 Medium 1 (air) E 2 n= 0 Medium 2 WATER DROPPLET
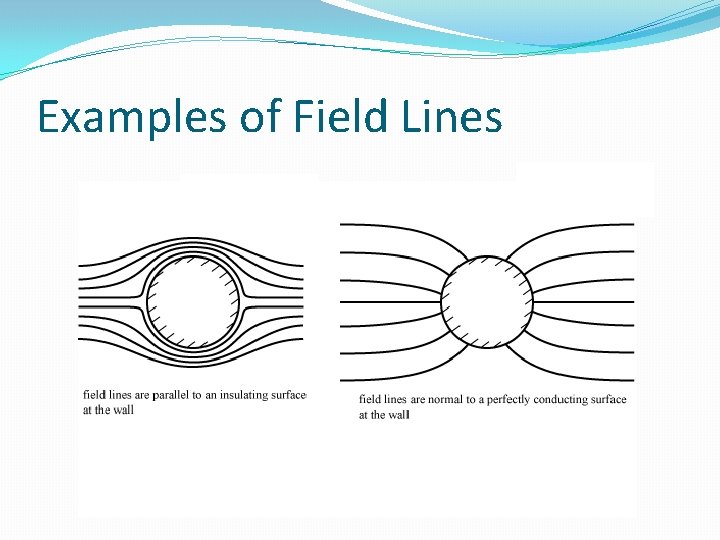
Examples of Field Lines
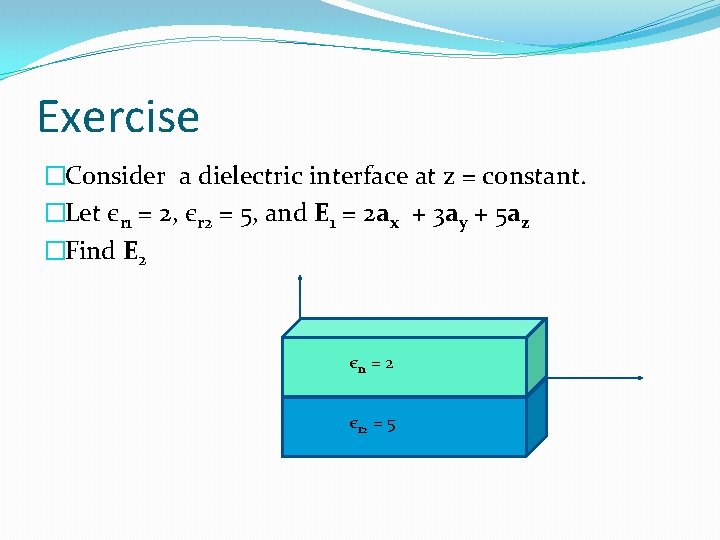
Exercise �Consider a dielectric interface at z = constant. �Let єr 1 = 2, єr 2 = 5, and E 1 = 2 ax + 3 ay + 5 az �Find E 2 єr 1 = 2 єr 2 = 5
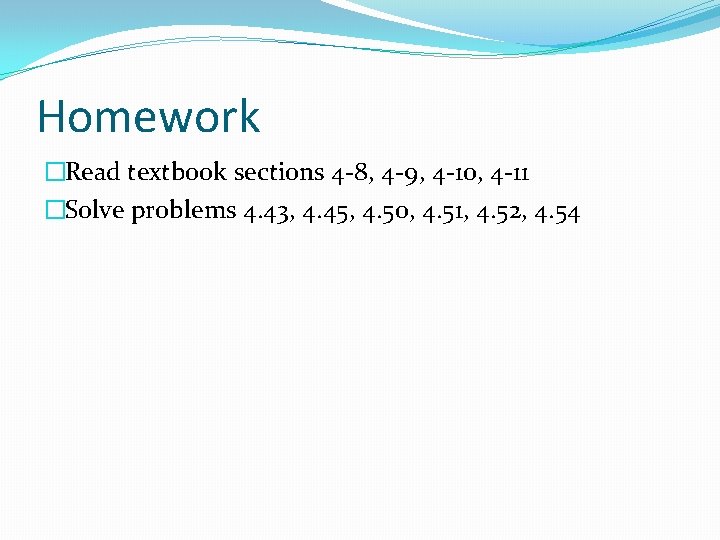
Homework �Read textbook sections 4 -8, 4 -9, 4 -10, 4 -11 �Solve problems 4. 43, 4. 45, 4. 50, 4. 51, 4. 52, 4. 54
Electric potential energy
Potential energy of an electric field
V = pe/q
V and e relation
Potential energy magnetic field
Potential energy of an electric field
Potential energy magnetic field
Potential due to a dipole
Relation between potential energy and electric field
Momentum of electromagnetic field
Energy formula in electricity
Formula for total mechanical energy
Elastic potential energy
What is energy
Gravitational potential energy vs kinetic energy
The law of conservation of energy states that
Example of potential energy
The change in mechanical energy
Gravitational potential energy
The change in mechanical energy
Why is water potential measured in pascals
Water potential equation
Understanding water potential
Neuronal pool
Graded potential and action potential
Graded potential definition
Graded vs action potential