Direct Wakefield measurement of CLIC accelerating structure in
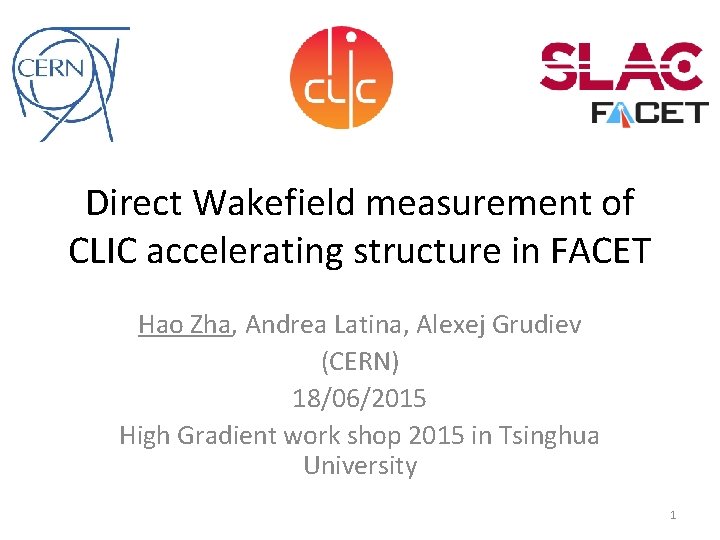
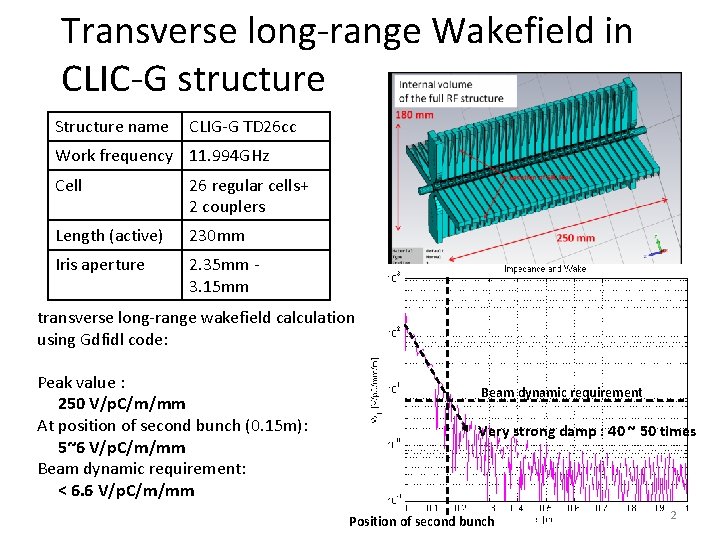
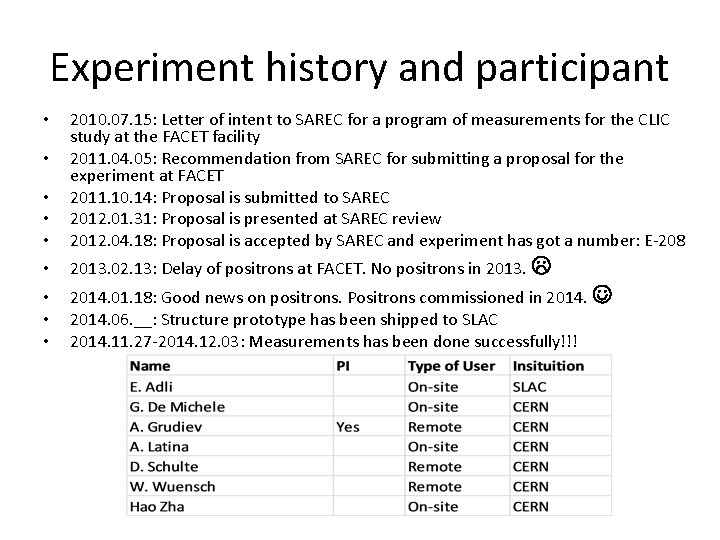
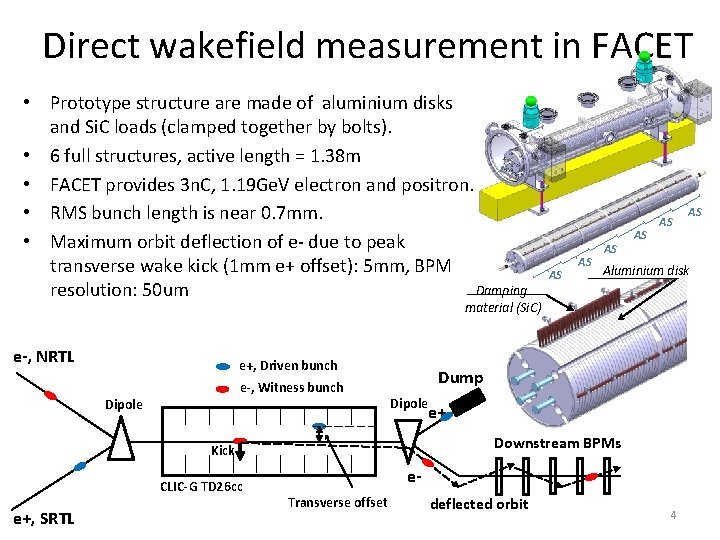
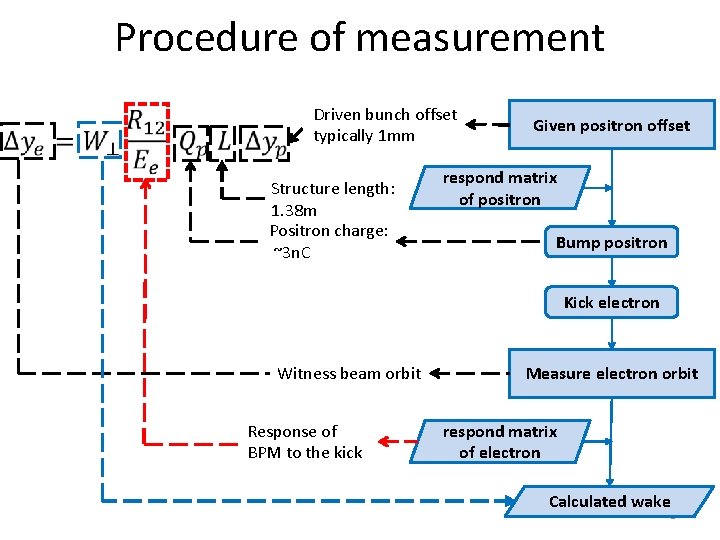
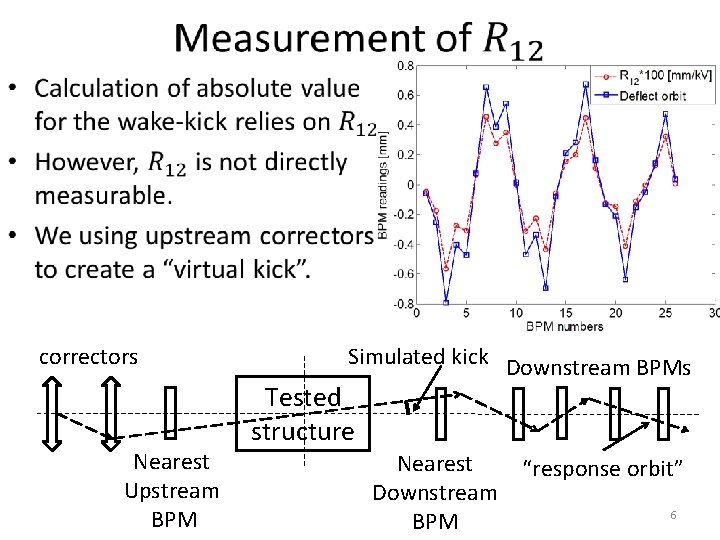
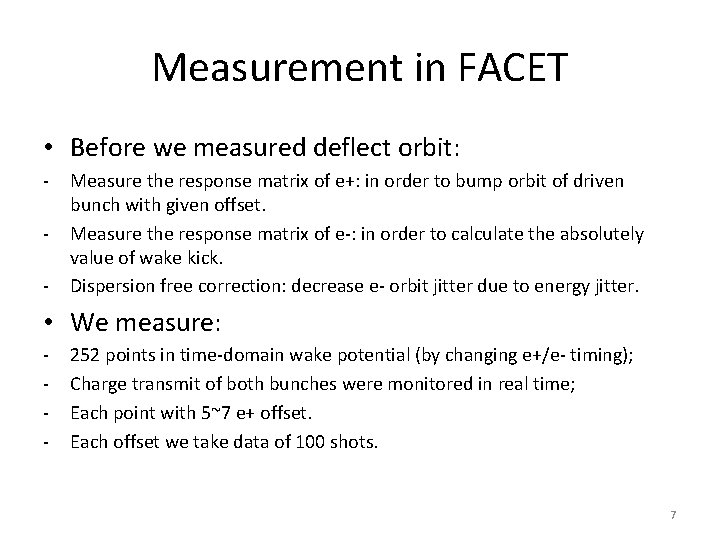
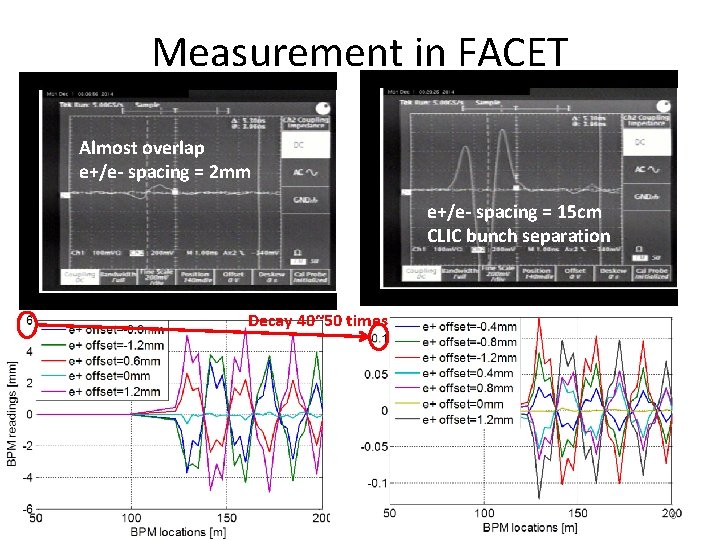
![Wakefield analysis Deflected Orbit [mm] Response orbit R 12[mm] of 1 Ke. V kick Wakefield analysis Deflected Orbit [mm] Response orbit R 12[mm] of 1 Ke. V kick](https://slidetodoc.com/presentation_image_h2/ef4ec4b1d043beb5df7c0d7d97fd86eb/image-9.jpg)
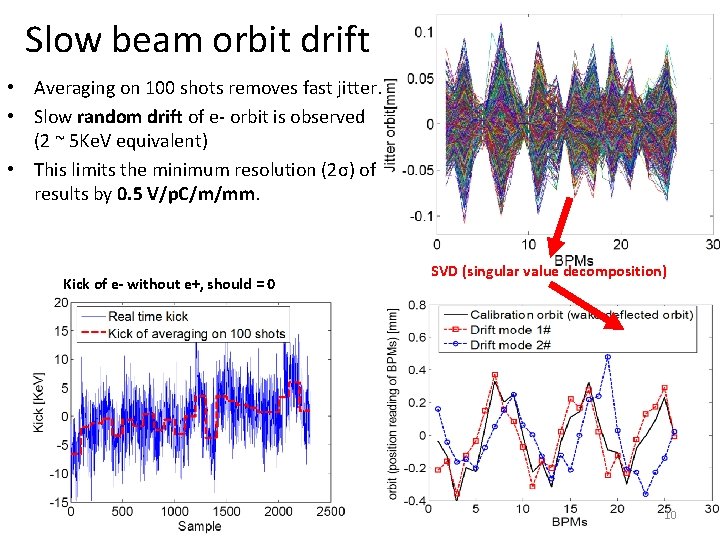
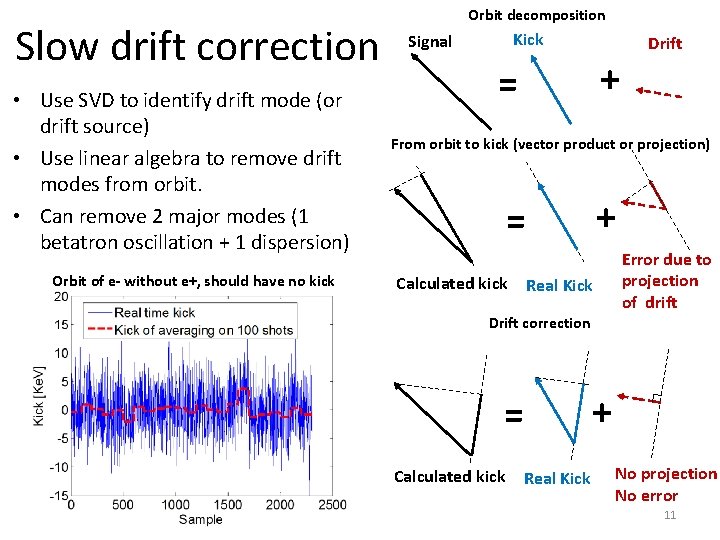
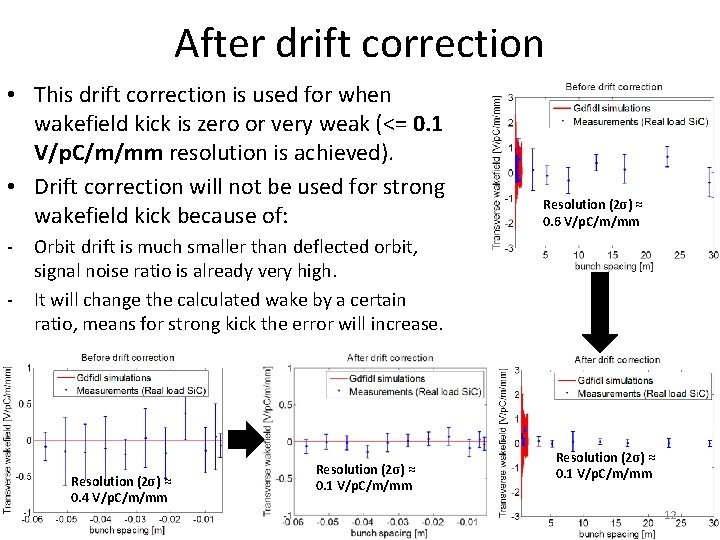
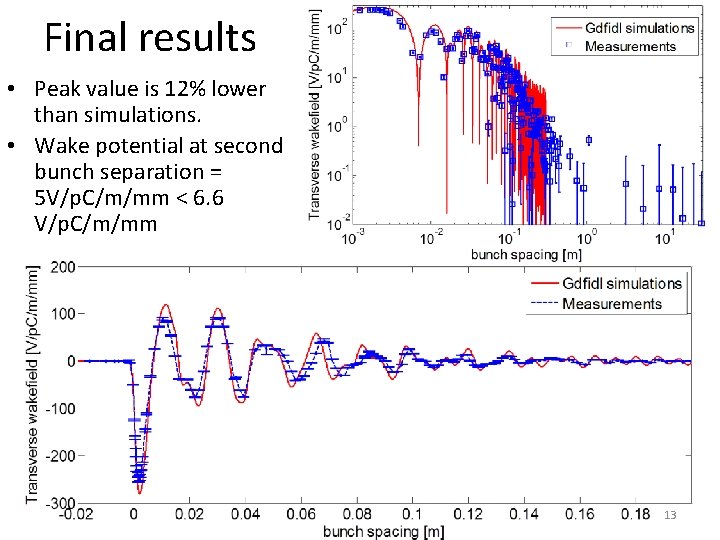
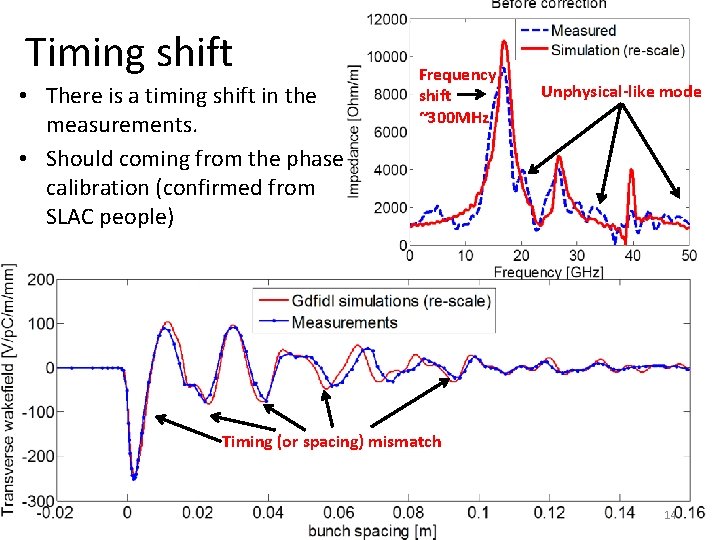
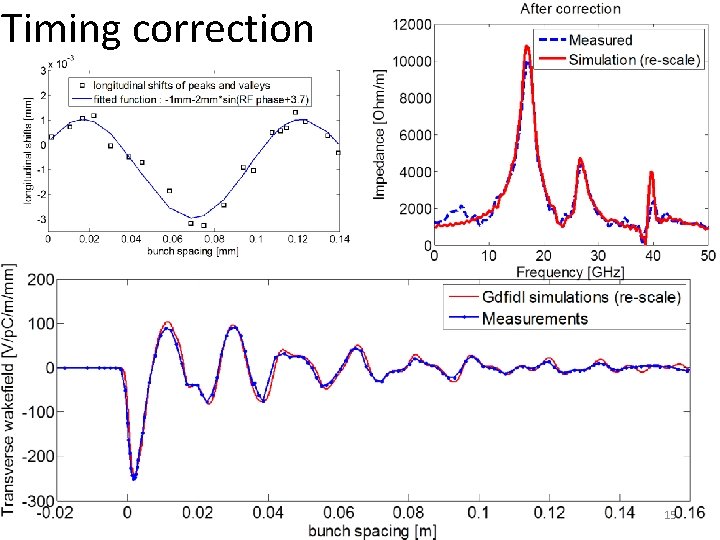
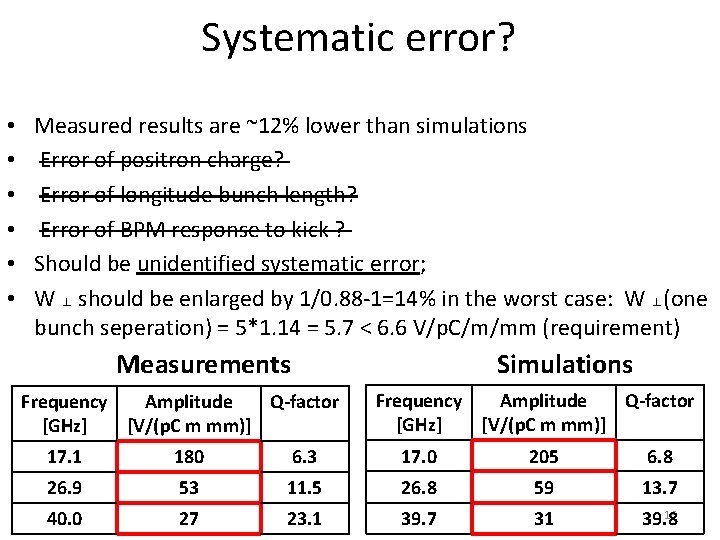
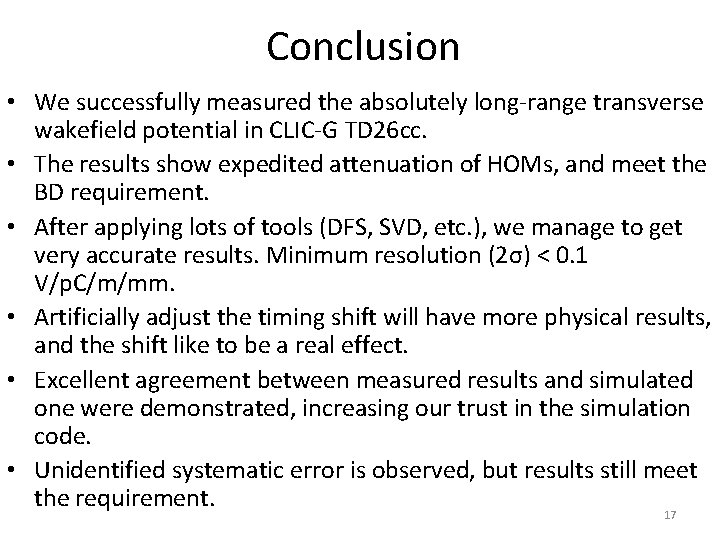
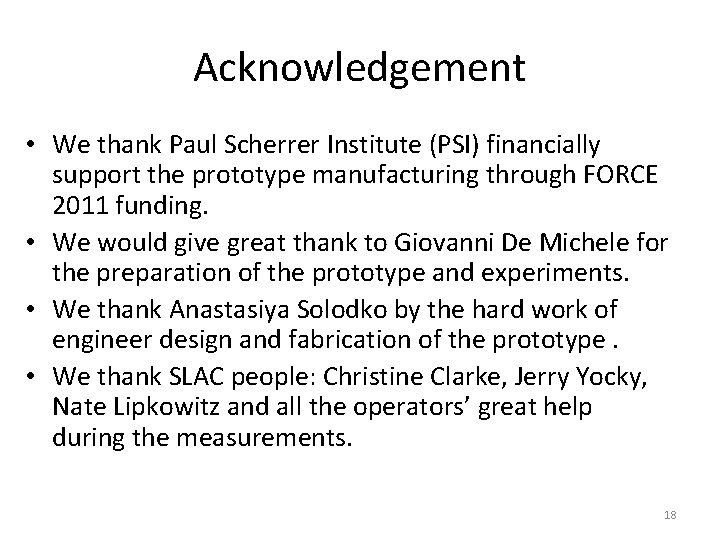
- Slides: 18
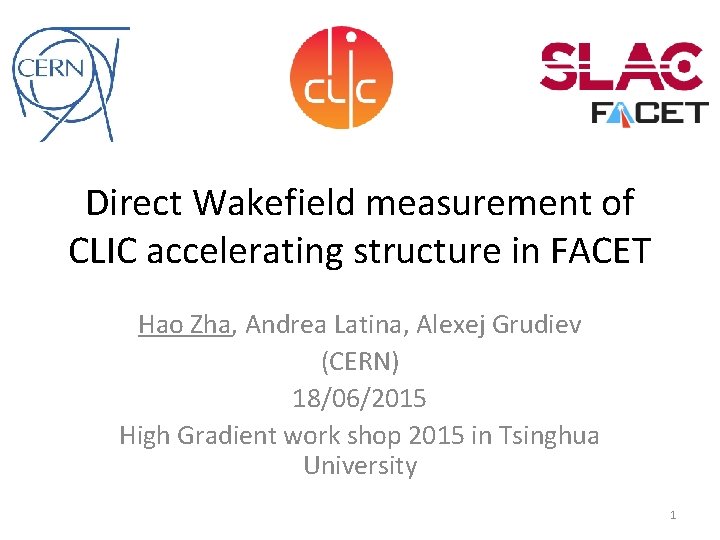
Direct Wakefield measurement of CLIC accelerating structure in FACET Hao Zha, Andrea Latina, Alexej Grudiev (CERN) 18/06/2015 High Gradient work shop 2015 in Tsinghua University 1
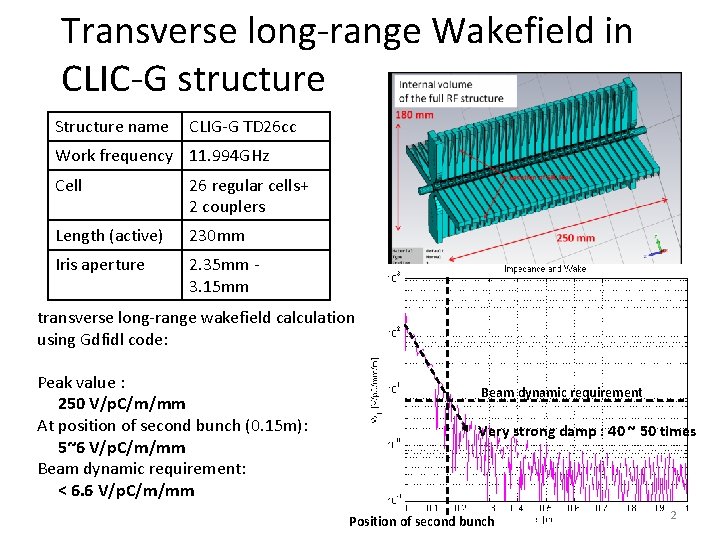
Transverse long-range Wakefield in CLIC-G structure Structure name CLIG-G TD 26 cc Work frequency 11. 994 GHz Cell 26 regular cells+ 2 couplers Length (active) 230 mm Iris aperture 2. 35 mm 3. 15 mm transverse long-range wakefield calculation using Gdfidl code: Peak value : 250 V/p. C/m/mm At position of second bunch (0. 15 m): 5~6 V/p. C/m/mm Beam dynamic requirement: < 6. 6 V/p. C/m/mm Beam dynamic requirement Very strong damp : 40 ~ 50 times Position of second bunch 2
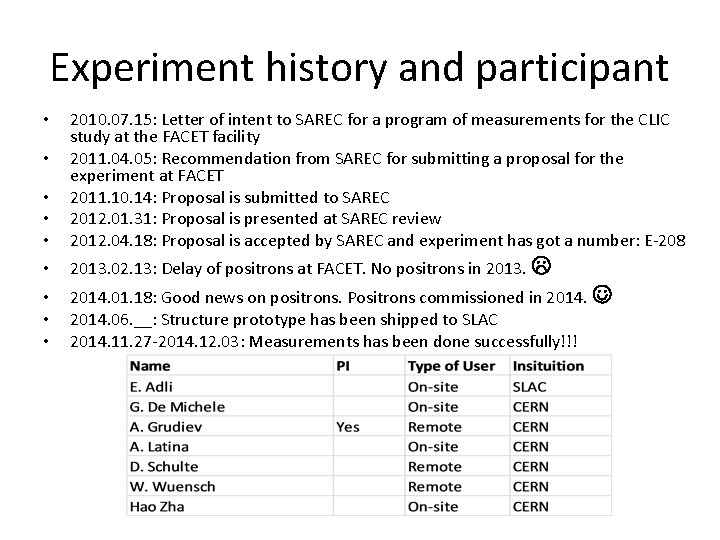
Experiment history and participant • • • 2010. 07. 15: Letter of intent to SAREC for a program of measurements for the CLIC study at the FACET facility 2011. 04. 05: Recommendation from SAREC for submitting a proposal for the experiment at FACET 2011. 10. 14: Proposal is submitted to SAREC 2012. 01. 31: Proposal is presented at SAREC review 2012. 04. 18: Proposal is accepted by SAREC and experiment has got a number: E-208 • 2013. 02. 13: Delay of positrons at FACET. No positrons in 2013. • • • 2014. 01. 18: Good news on positrons. Positrons commissioned in 2014. 06. __: Structure prototype has been shipped to SLAC 2014. 11. 27 -2014. 12. 03: Measurements has been done successfully!!! • •
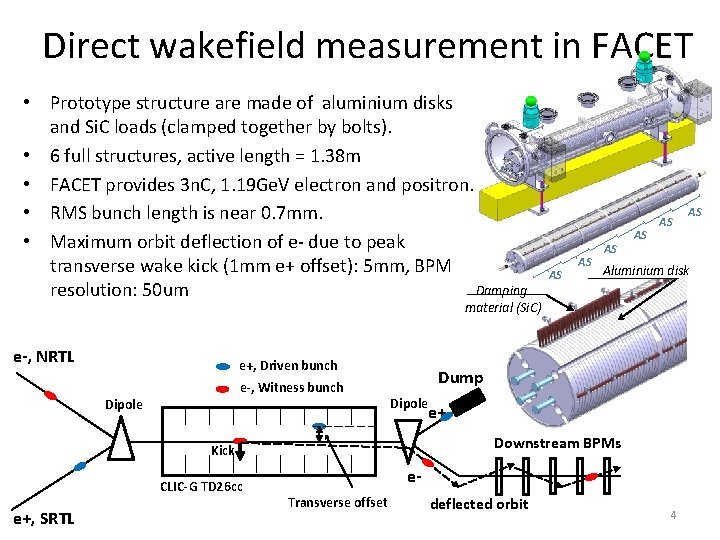
Direct wakefield measurement in FACET • Prototype structure are made of aluminium disks and Si. C loads (clamped together by bolts). • 6 full structures, active length = 1. 38 m • FACET provides 3 n. C, 1. 19 Ge. V electron and positron. • RMS bunch length is near 0. 7 mm. • Maximum orbit deflection of e- due to peak transverse wake kick (1 mm e+ offset): 5 mm, BPM Damping resolution: 50 um AS AS AS Aluminium disk material (Si. C) e-, NRTL e+, Driven bunch e-, Witness bunch Dipole Dump Dipole e+ Downstream BPMs Kick CLIC-G TD 26 cc e+, SRTL e. Transverse offset deflected orbit AS 4
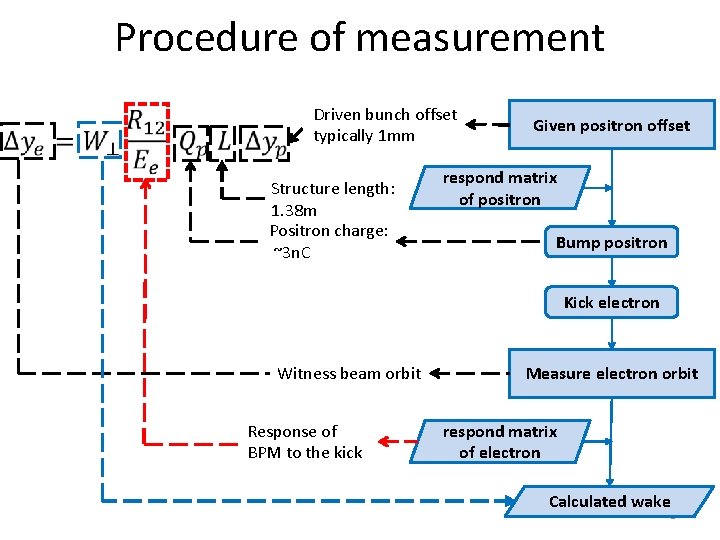
Procedure of measurement Driven bunch offset typically 1 mm Structure length: 1. 38 m Positron charge: ~3 n. C Given positron offset respond matrix of positron Bump positron Kick electron Witness beam orbit Response of BPM to the kick Measure electron orbit respond matrix of electron Calculated wake 5
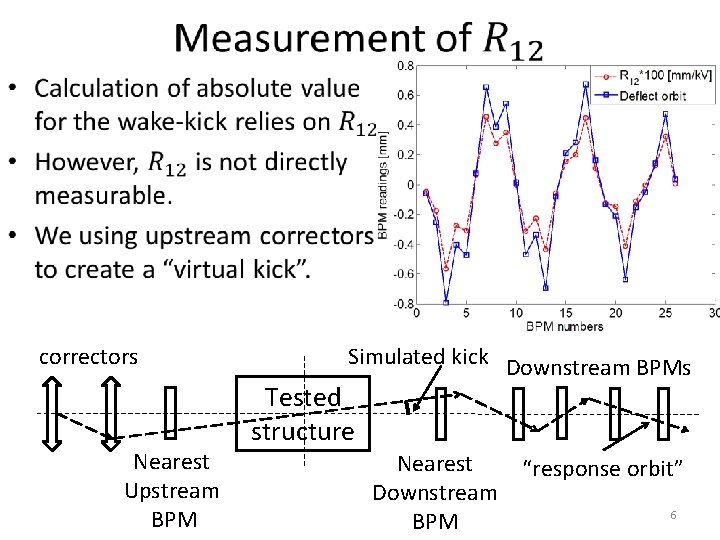
• correctors Nearest Upstream BPM Simulated kick Downstream BPMs Tested structure Nearest Downstream BPM “response orbit” 6
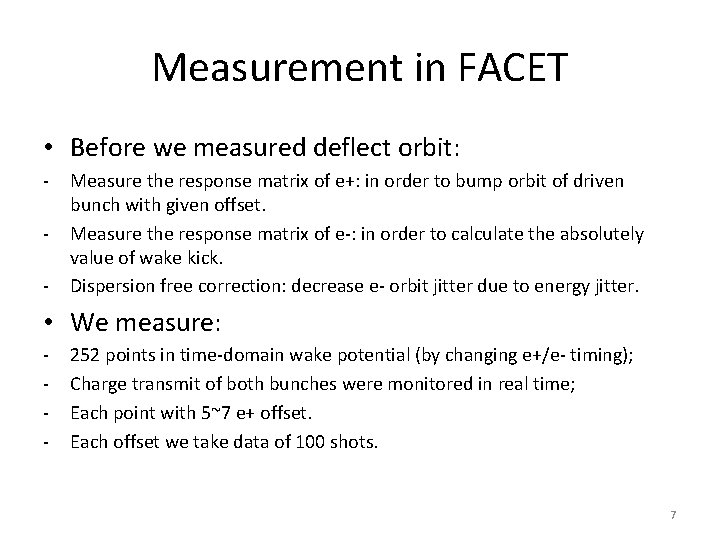
Measurement in FACET • Before we measured deflect orbit: - Measure the response matrix of e+: in order to bump orbit of driven bunch with given offset. Measure the response matrix of e-: in order to calculate the absolutely value of wake kick. Dispersion free correction: decrease e- orbit jitter due to energy jitter. • We measure: - 252 points in time-domain wake potential (by changing e+/e- timing); Charge transmit of both bunches were monitored in real time; Each point with 5~7 e+ offset. Each offset we take data of 100 shots. 7
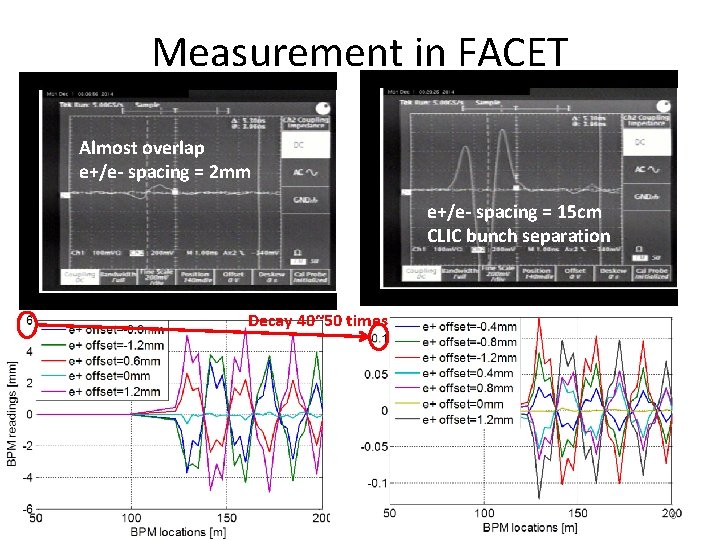
Measurement in FACET Almost overlap e+/e- spacing = 2 mm e+/e- spacing = 15 cm CLIC bunch separation Decay 40~50 times 8
![Wakefield analysis Deflected Orbit mm Response orbit R 12mm of 1 Ke V kick Wakefield analysis Deflected Orbit [mm] Response orbit R 12[mm] of 1 Ke. V kick](https://slidetodoc.com/presentation_image_h2/ef4ec4b1d043beb5df7c0d7d97fd86eb/image-9.jpg)
Wakefield analysis Deflected Orbit [mm] Response orbit R 12[mm] of 1 Ke. V kick One point Shot 1 …… Shot 100 Offset 1 kick 11 …… Kick 1, 100 Offset 2 kick 21 …… Kick 2, 100 ⁞ ⁞ …⁞ … ⁞ Offset 7 kick 71 …… Kick 7, 100 ⁞ 9
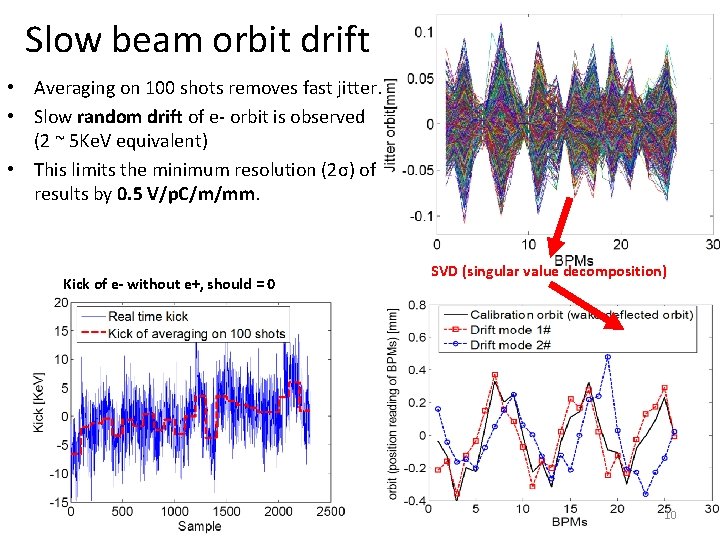
Slow beam orbit drift • Averaging on 100 shots removes fast jitter. • Slow random drift of e- orbit is observed (2 ~ 5 Ke. V equivalent) • This limits the minimum resolution (2σ) of results by 0. 5 V/p. C/m/mm. Kick of e- without e+, should = 0 SVD (singular value decomposition) 10
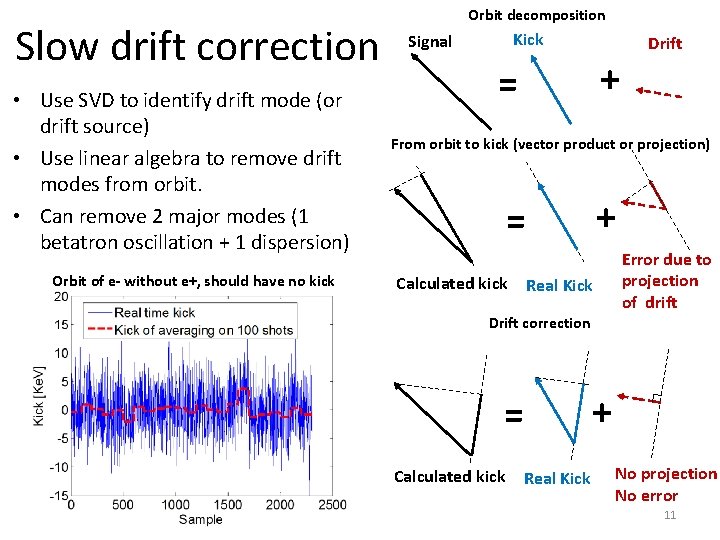
Slow drift correction • Use SVD to identify drift mode (or drift source) • Use linear algebra to remove drift modes from orbit. • Can remove 2 major modes (1 betatron oscillation + 1 dispersion) Orbit of e- without e+, should have no kick Orbit decomposition Kick Signal + = Drift From orbit to kick (vector product or projection) + = Calculated kick Real Kick Drift correction = Calculated kick Real Kick Error due to projection of drift + No projection No error 11
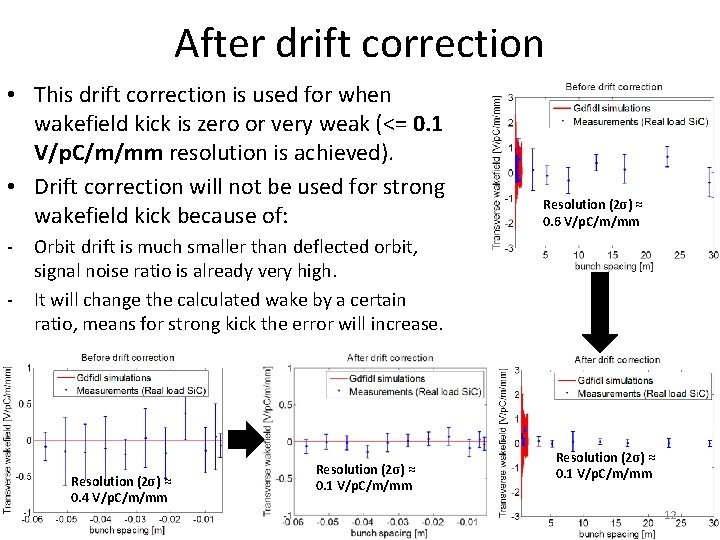
After drift correction • This drift correction is used for when wakefield kick is zero or very weak (<= 0. 1 V/p. C/m/mm resolution is achieved). • Drift correction will not be used for strong wakefield kick because of: - Resolution (2σ) ≈ 0. 6 V/p. C/m/mm Orbit drift is much smaller than deflected orbit, signal noise ratio is already very high. It will change the calculated wake by a certain ratio, means for strong kick the error will increase. Resolution (2σ) ≈ 0. 4 V/p. C/m/mm Resolution (2σ) ≈ 0. 1 V/p. C/m/mm 12
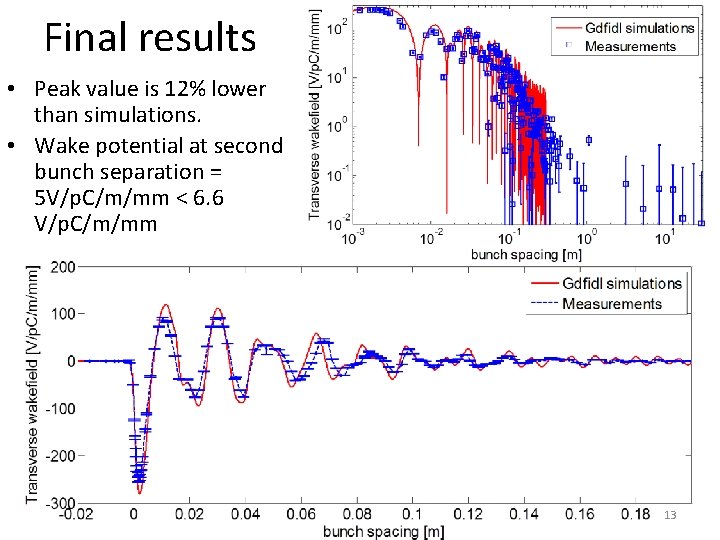
Final results • Peak value is 12% lower than simulations. • Wake potential at second bunch separation = 5 V/p. C/m/mm < 6. 6 V/p. C/m/mm 13
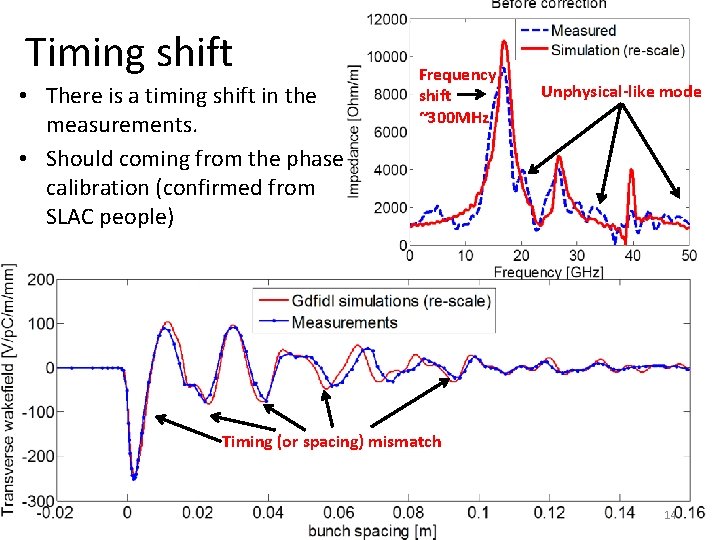
Timing shift • There is a timing shift in the measurements. • Should coming from the phase calibration (confirmed from SLAC people) Frequency shift ~300 MHz Unphysical-like mode Timing (or spacing) mismatch 14
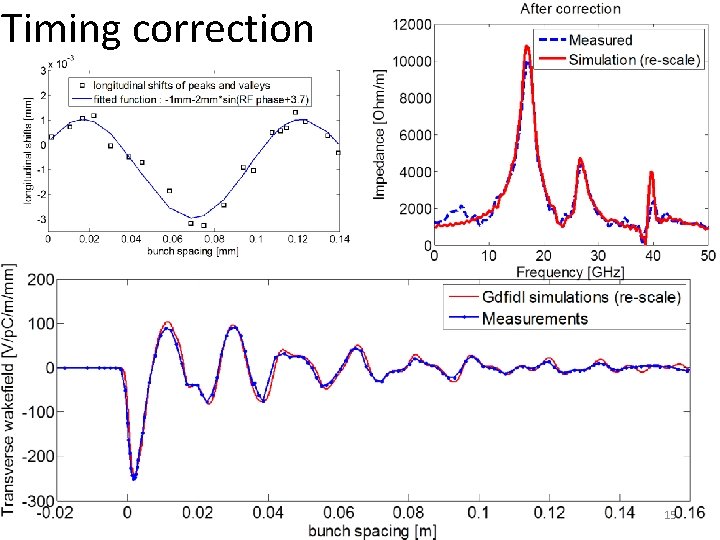
Timing correction 15
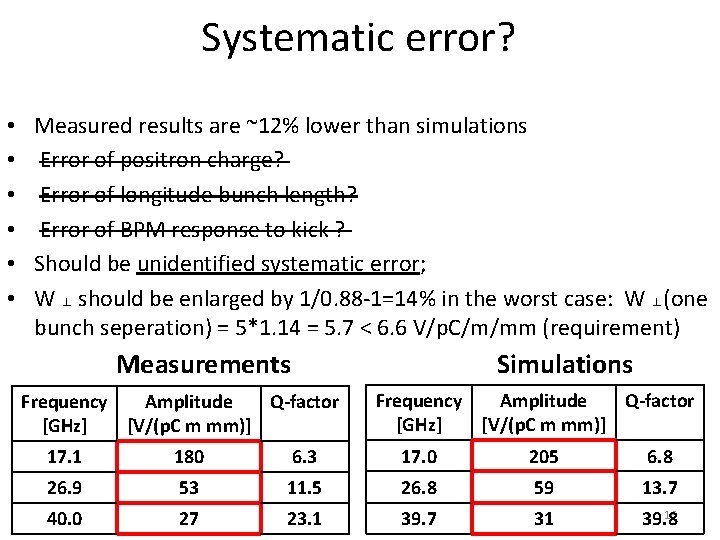
Systematic error? • • • Measured results are ~12% lower than simulations Error of positron charge? Error of longitude bunch length? Error of BPM response to kick ? Should be unidentified systematic error; W ⊥ should be enlarged by 1/0. 88 -1=14% in the worst case: W ⊥(one bunch seperation) = 5*1. 14 = 5. 7 < 6. 6 V/p. C/m/mm (requirement) Measurements Simulations Frequency Amplitude Q-factor [GHz] [V/(p. C m mm)] 17. 1 180 6. 3 17. 0 205 6. 8 26. 9 53 11. 5 26. 8 59 13. 7 40. 0 27 23. 1 39. 7 31 16 39. 8
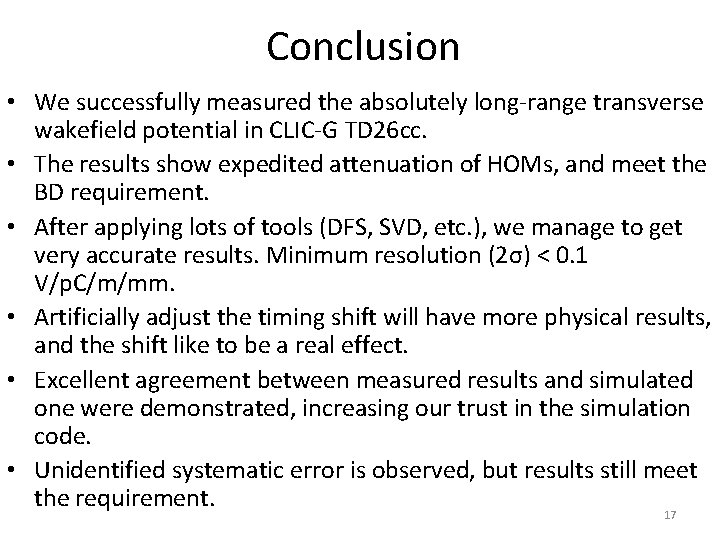
Conclusion • We successfully measured the absolutely long-range transverse wakefield potential in CLIC-G TD 26 cc. • The results show expedited attenuation of HOMs, and meet the BD requirement. • After applying lots of tools (DFS, SVD, etc. ), we manage to get very accurate results. Minimum resolution (2σ) < 0. 1 V/p. C/m/mm. • Artificially adjust the timing shift will have more physical results, and the shift like to be a real effect. • Excellent agreement between measured results and simulated one were demonstrated, increasing our trust in the simulation code. • Unidentified systematic error is observed, but results still meet the requirement. 17
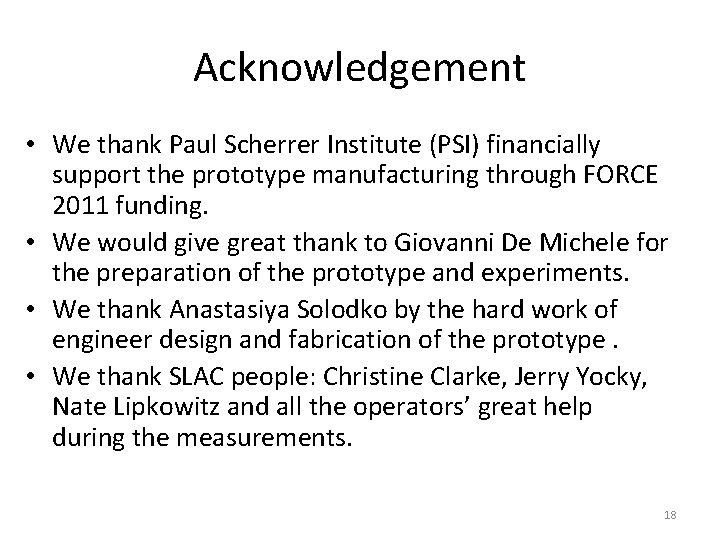
Acknowledgement • We thank Paul Scherrer Institute (PSI) financially support the prototype manufacturing through FORCE 2011 funding. • We would give great thank to Giovanni De Michele for the preparation of the prototype and experiments. • We thank Anastasiya Solodko by the hard work of engineer design and fabrication of the prototype. • We thank SLAC people: Christine Clarke, Jerry Yocky, Nate Lipkowitz and all the operators’ great help during the measurements. 18
Accelerating rate calorimeter
Law of accelerating returns
Effect of accelerating voltage on sem resolution
Accelerating implementation methodology
Accelerating high performance
Advanced materials
Wound healing definition
Wakefield middle school bell schedule
Wakefield youth offending team
Laser wakefield acceleration
Brendan white wakefield
For teen
Brady's place weymouth ma
Hmp wakefield
Public health mdc
Dr tamekia wakefield
Entrez donc saint nicolas
Double entry for sales invoice
Cuantas letras hay