Chapter 1 Diodes Gopika Sood Assistant Professor in
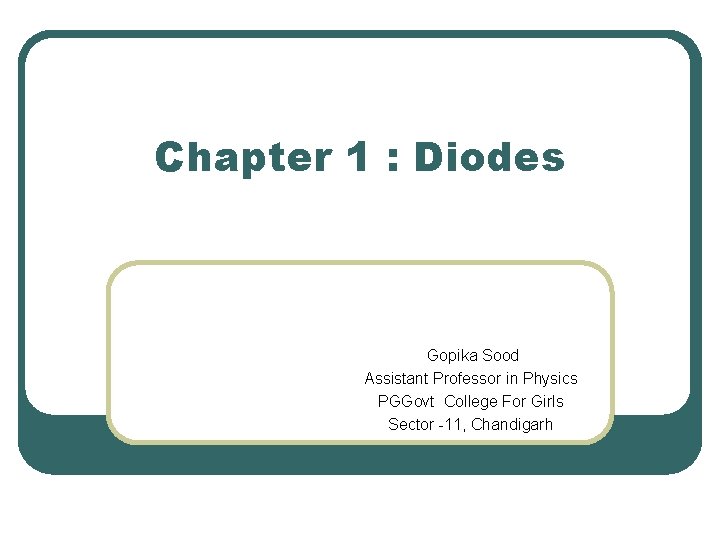
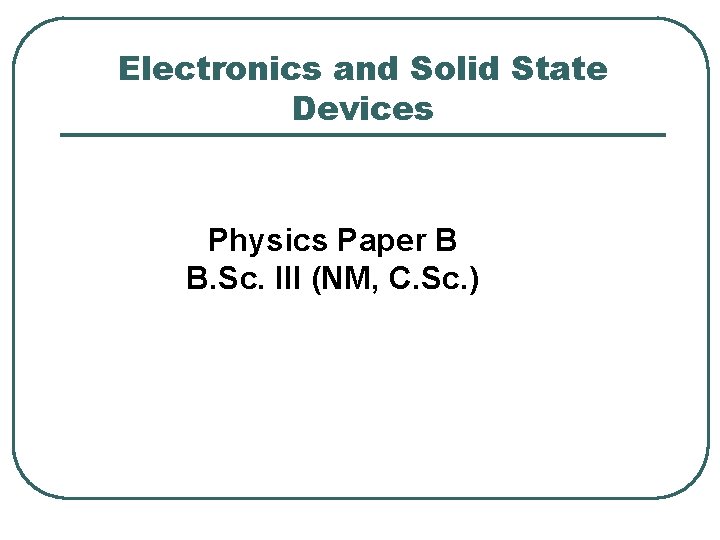
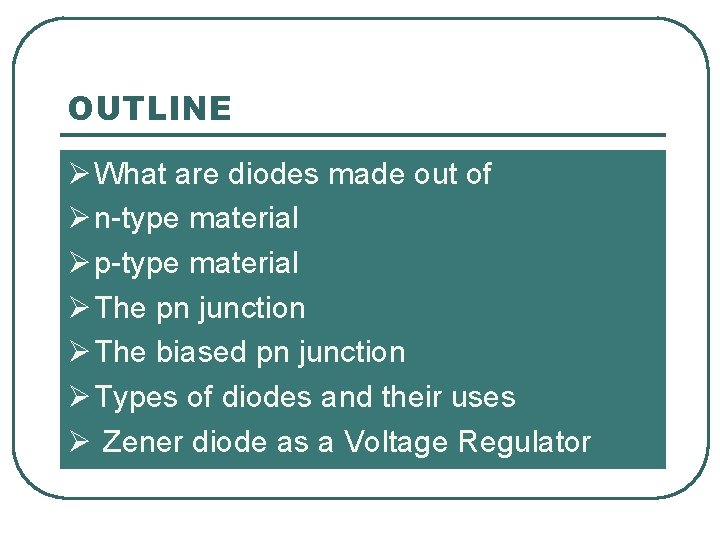
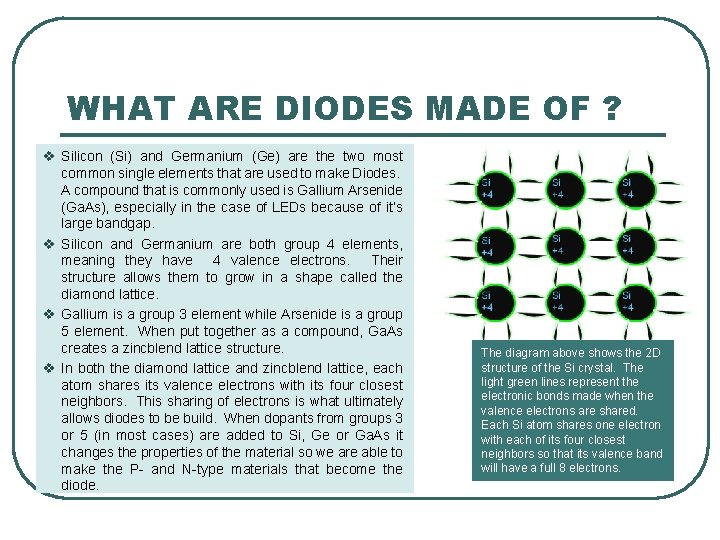
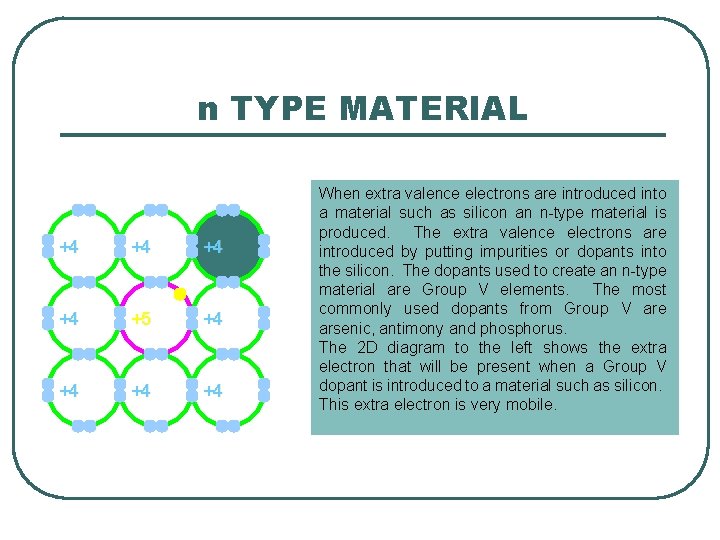
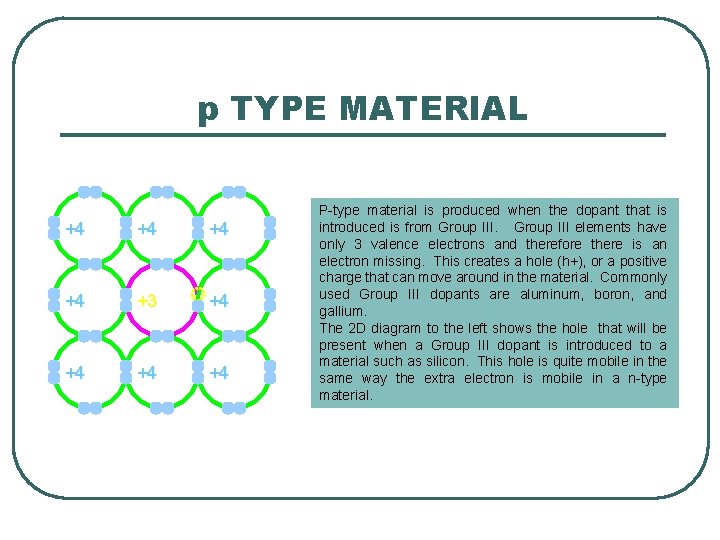
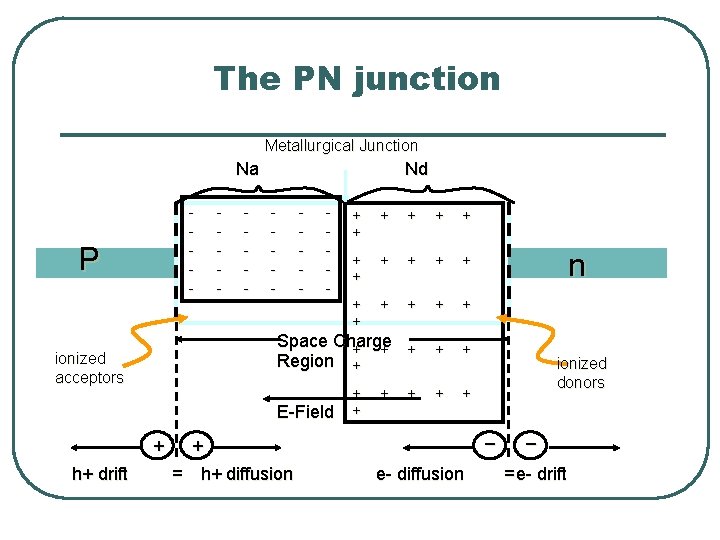
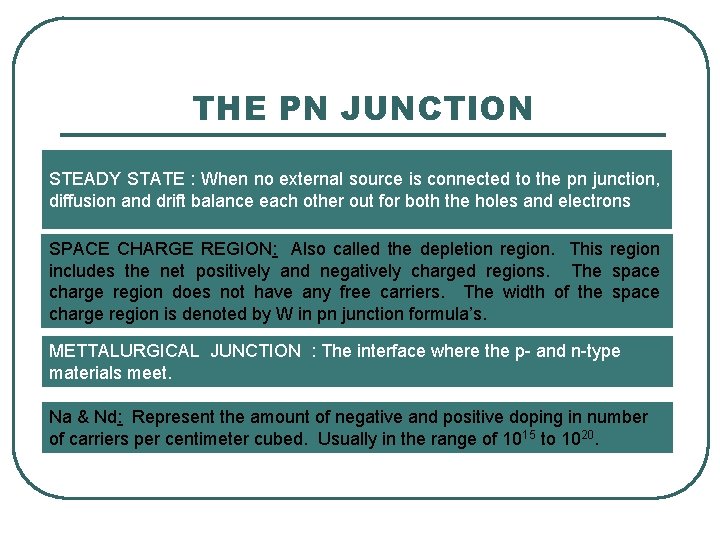
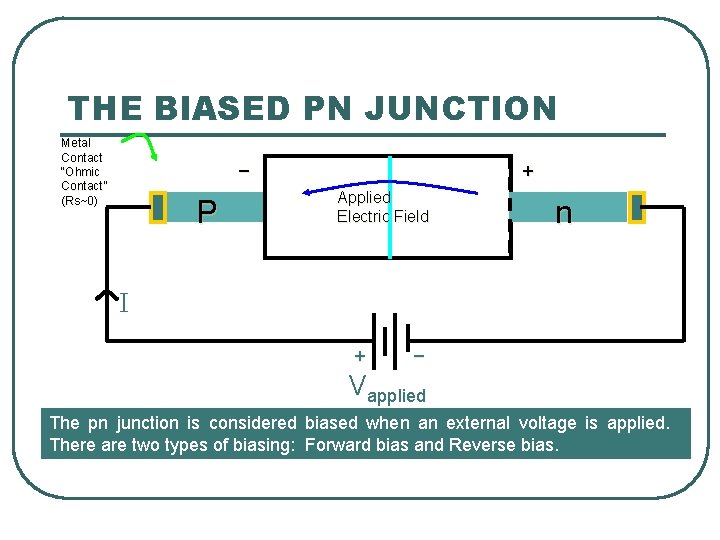
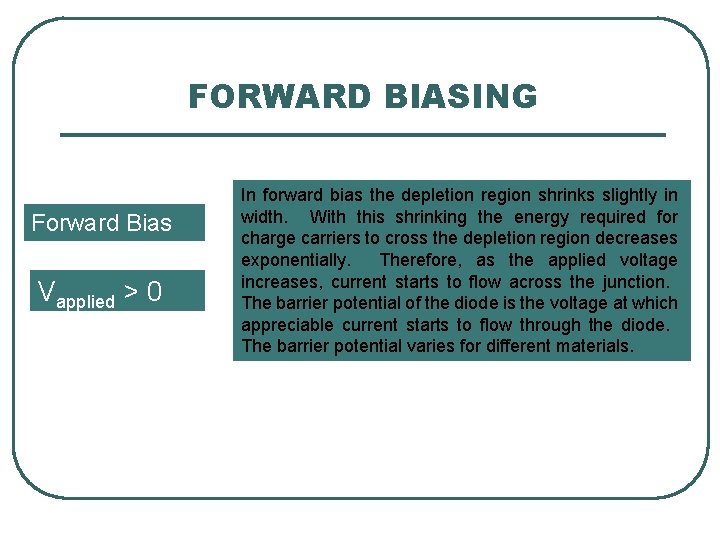
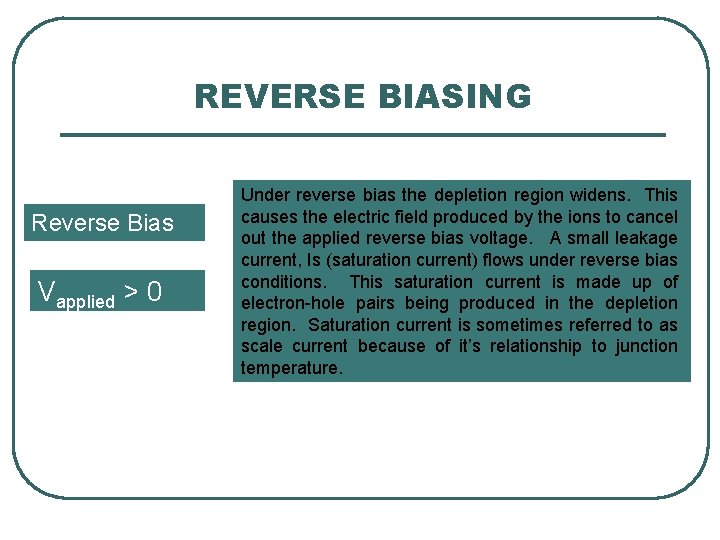
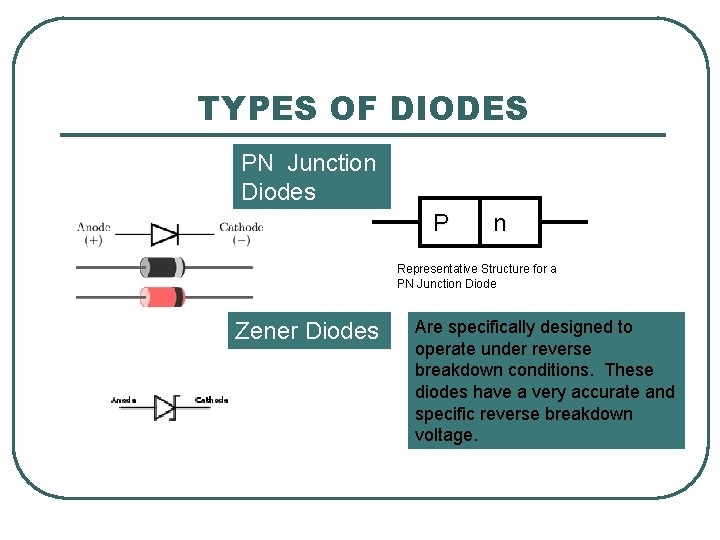
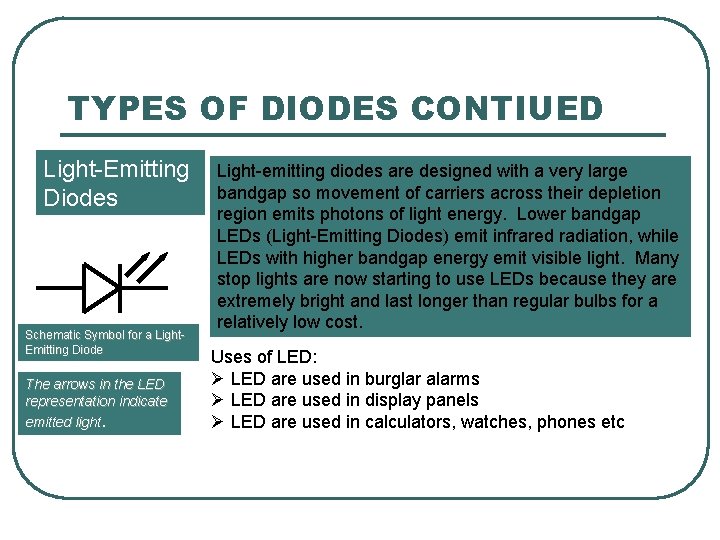
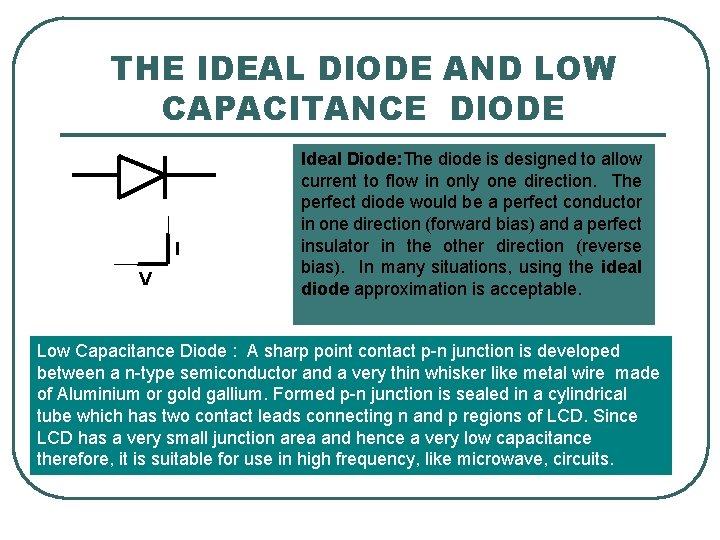
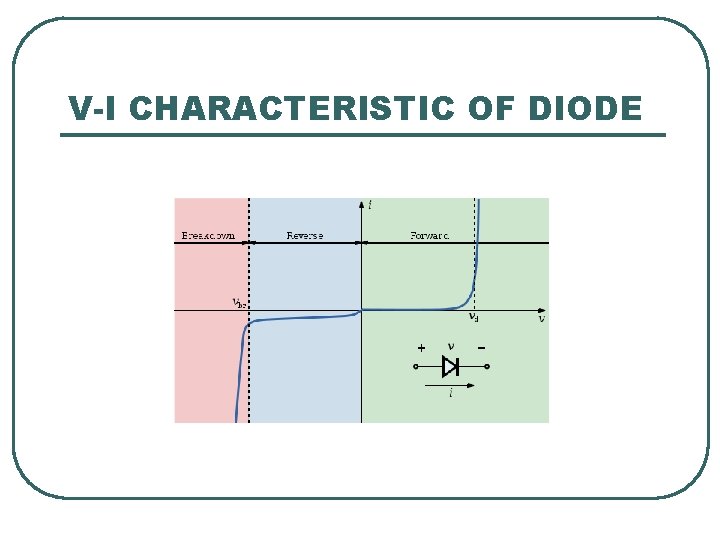
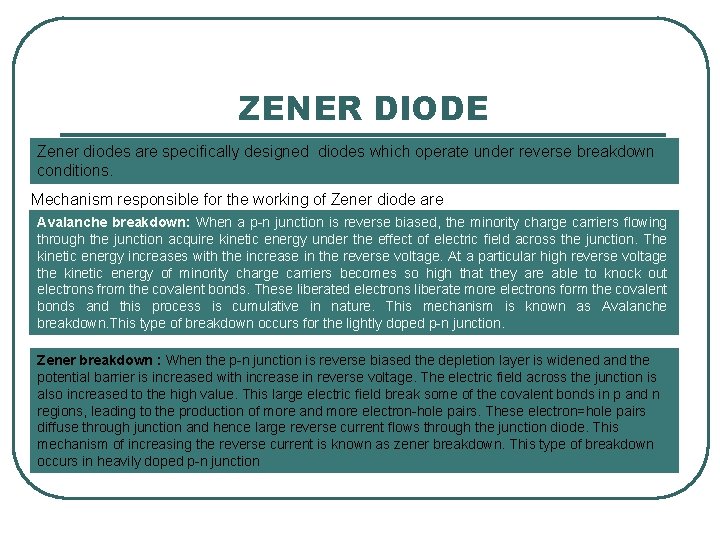
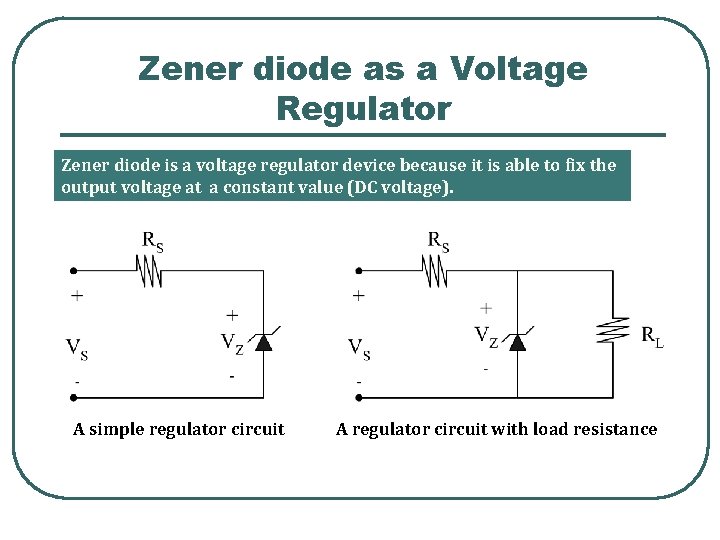
- Slides: 17
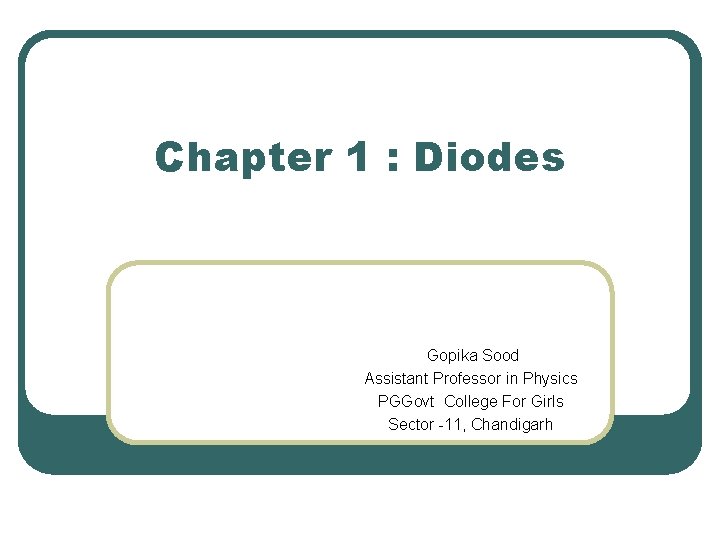
Chapter 1 : Diodes Gopika Sood Assistant Professor in Physics PGGovt College For Girls Sector -11, Chandigarh
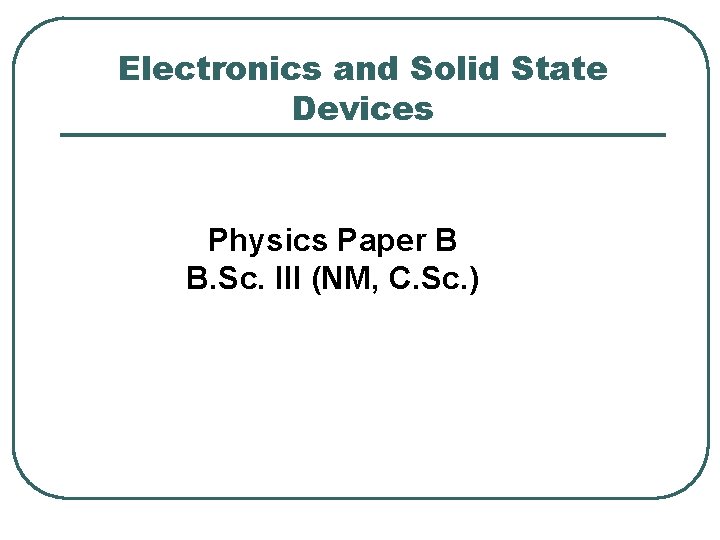
Electronics and Solid State Devices Physics Paper B B. Sc. III (NM, C. Sc. )
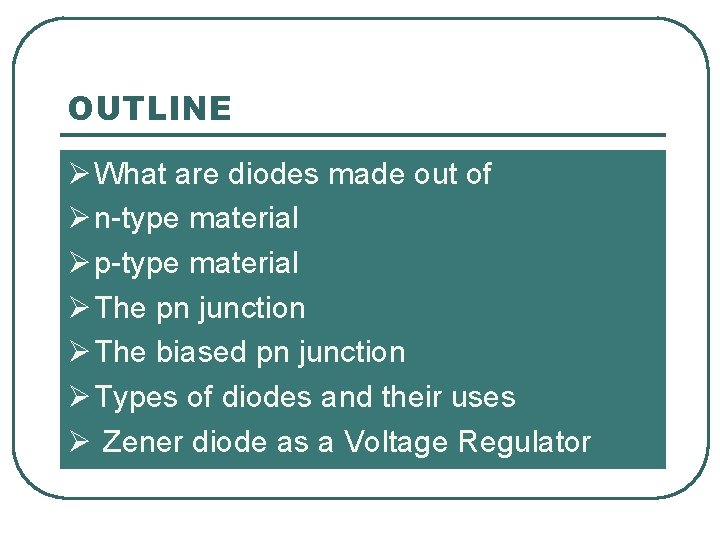
OUTLINE Ø What are diodes made out of Ø n-type material Ø p-type material Ø The pn junction Ø The biased pn junction Ø Types of diodes and their uses Ø Zener diode as a Voltage Regulator
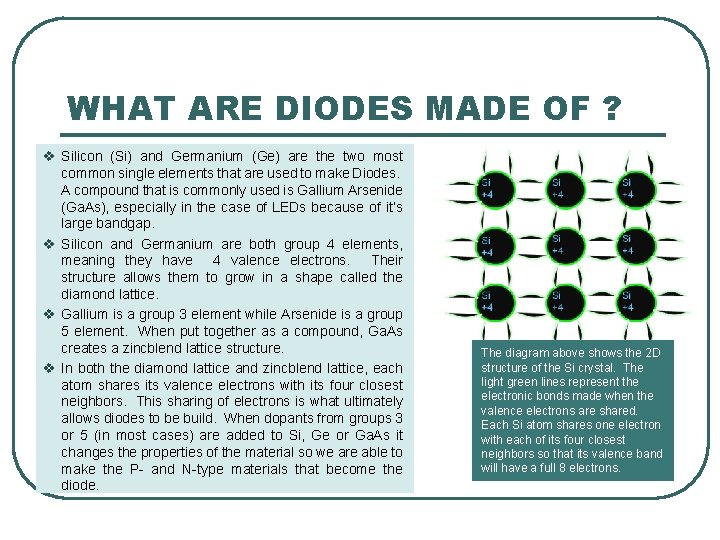
WHAT ARE DIODES MADE OF ? v Silicon (Si) and Germanium (Ge) are the two most common single elements that are used to make Diodes. A compound that is commonly used is Gallium Arsenide (Ga. As), especially in the case of LEDs because of it’s large bandgap. v Silicon and Germanium are both group 4 elements, meaning they have 4 valence electrons. Their structure allows them to grow in a shape called the diamond lattice. v Gallium is a group 3 element while Arsenide is a group 5 element. When put together as a compound, Ga. As creates a zincblend lattice structure. v In both the diamond lattice and zincblend lattice, each atom shares its valence electrons with its four closest neighbors. This sharing of electrons is what ultimately allows diodes to be build. When dopants from groups 3 or 5 (in most cases) are added to Si, Ge or Ga. As it changes the properties of the material so we are able to make the P- and N-type materials that become the diode. The diagram above shows the 2 D structure of the Si crystal. The light green lines represent the electronic bonds made when the valence electrons are shared. Each Si atom shares one electron with each of its four closest neighbors so that its valence band will have a full 8 electrons.
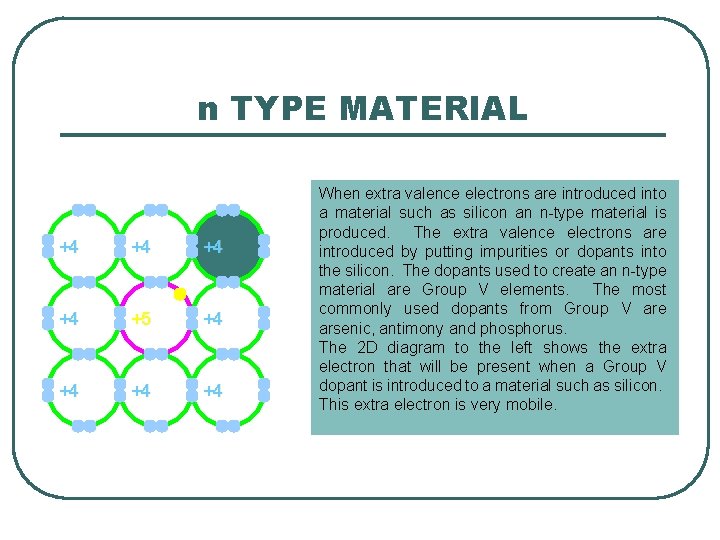
n TYPE MATERIAL +4 +4 +5 +4 +4 When extra valence electrons are introduced into a material such as silicon an n-type material is produced. The extra valence electrons are introduced by putting impurities or dopants into the silicon. The dopants used to create an n-type material are Group V elements. The most commonly used dopants from Group V are arsenic, antimony and phosphorus. The 2 D diagram to the left shows the extra electron that will be present when a Group V dopant is introduced to a material such as silicon. This extra electron is very mobile.
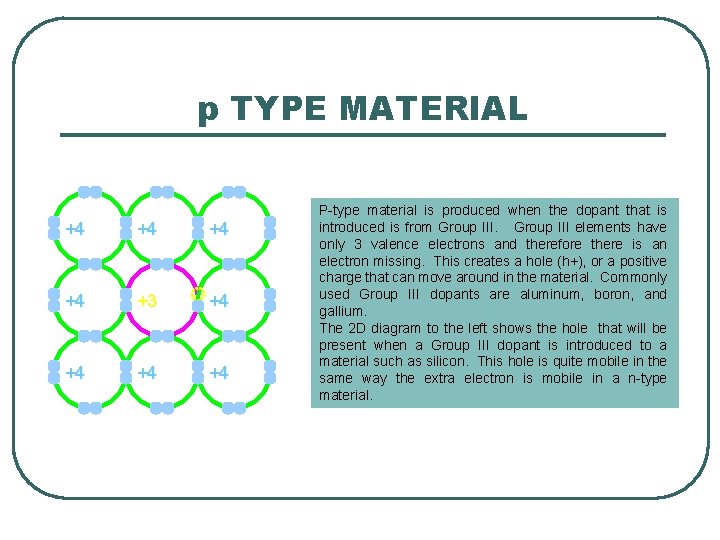
p TYPE MATERIAL +4 +4 +3 +4 +4 P-type material is produced when the dopant that is introduced is from Group III elements have only 3 valence electrons and therefore there is an electron missing. This creates a hole (h+), or a positive charge that can move around in the material. Commonly used Group III dopants are aluminum, boron, and gallium. The 2 D diagram to the left shows the hole that will be present when a Group III dopant is introduced to a material such as silicon. This hole is quite mobile in the same way the extra electron is mobile in a n-type material.
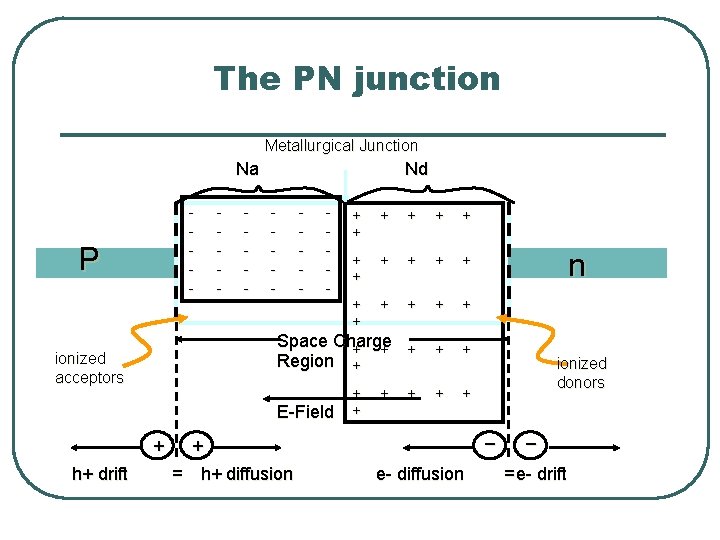
The PN junction Metallurgical Junction Na - P ionized acceptors - - Nd - - - + + + + + Space Charge + + Region + + + + E-Field + h+ drift + + + ionized donors _ + = h+ diffusion n e- diffusion _ = e- drift
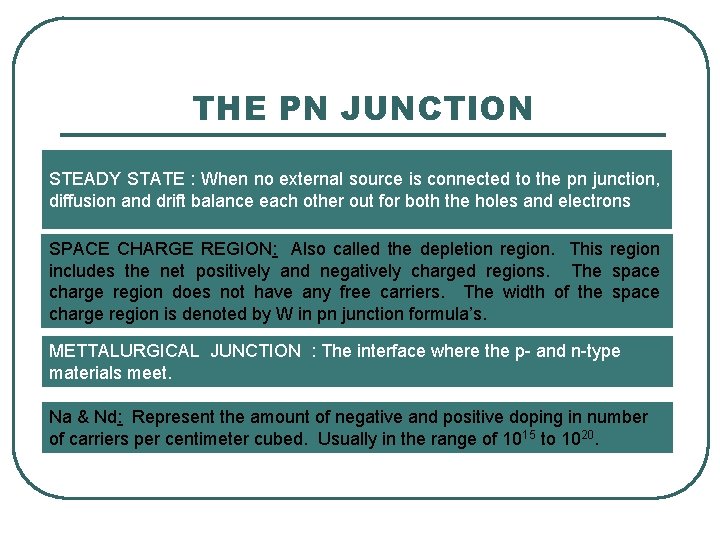
THE PN JUNCTION STEADY STATE : When no external source is connected to the pn junction, diffusion and drift balance each other out for both the holes and electrons SPACE CHARGE REGION: Also called the depletion region. This region includes the net positively and negatively charged regions. The space charge region does not have any free carriers. The width of the space charge region is denoted by W in pn junction formula’s. METTALURGICAL JUNCTION : The interface where the p- and n-type materials meet. Na & Nd: Represent the amount of negative and positive doping in number of carriers per centimeter cubed. Usually in the range of 1015 to 1020.
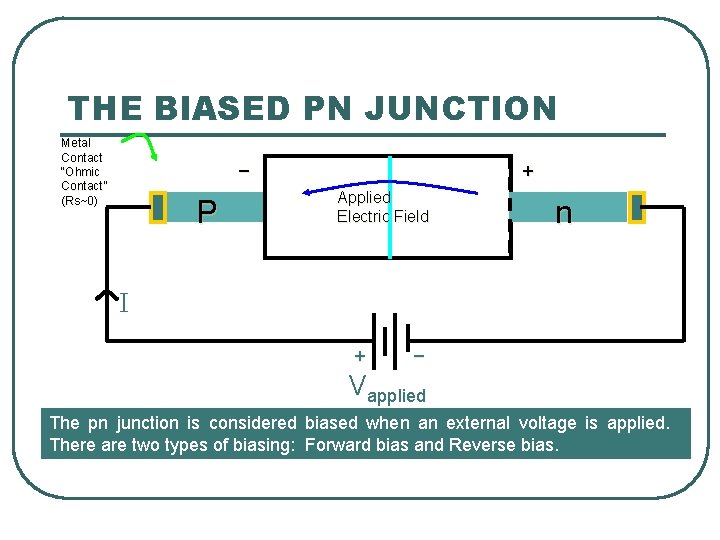
THE BIASED PN JUNCTION Metal Contact “Ohmic Contact” (Rs~0) _ P + Applied Electric Field n I + _ Vapplied The pn junction is considered biased when an external voltage is applied. There are two types of biasing: Forward bias and Reverse bias.
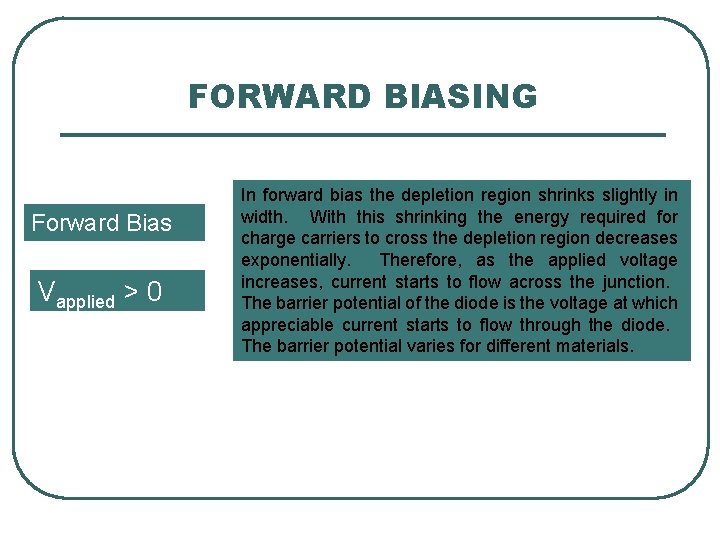
FORWARD BIASING Forward Bias Vapplied > 0 In forward bias the depletion region shrinks slightly in width. With this shrinking the energy required for charge carriers to cross the depletion region decreases exponentially. Therefore, as the applied voltage increases, current starts to flow across the junction. The barrier potential of the diode is the voltage at which appreciable current starts to flow through the diode. The barrier potential varies for different materials.
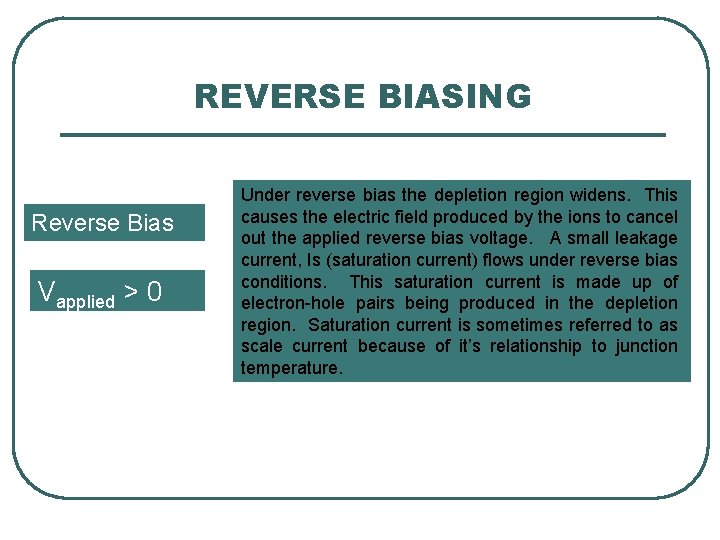
REVERSE BIASING Reverse Bias Vapplied > 0 Under reverse bias the depletion region widens. This causes the electric field produced by the ions to cancel out the applied reverse bias voltage. A small leakage current, Is (saturation current) flows under reverse bias conditions. This saturation current is made up of electron-hole pairs being produced in the depletion region. Saturation current is sometimes referred to as scale current because of it’s relationship to junction temperature.
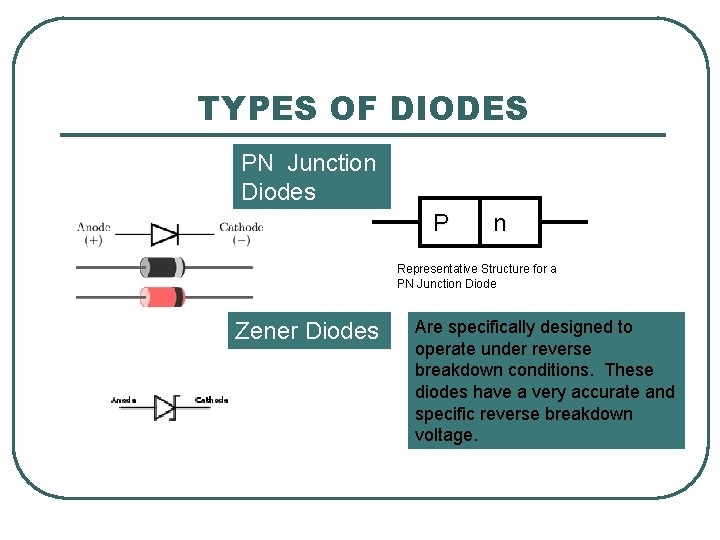
TYPES OF DIODES PN Junction Diodes P n Representative Structure for a PN Junction Diode Zener Diodes Are specifically designed to operate under reverse breakdown conditions. These diodes have a very accurate and specific reverse breakdown voltage.
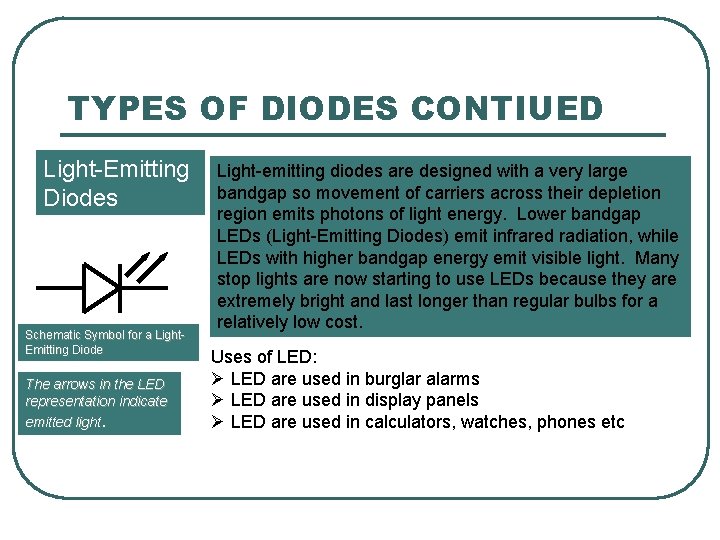
TYPES OF DIODES CONTIUED Light-Emitting Diodes Schematic Symbol for a Light. Emitting Diode The arrows in the LED representation indicate emitted light. Light-emitting diodes are designed with a very large bandgap so movement of carriers across their depletion region emits photons of light energy. Lower bandgap LEDs (Light-Emitting Diodes) emit infrared radiation, while LEDs with higher bandgap energy emit visible light. Many stop lights are now starting to use LEDs because they are extremely bright and last longer than regular bulbs for a relatively low cost. Uses of LED: Ø LED are used in burglar alarms Ø LED are used in display panels Ø LED are used in calculators, watches, phones etc
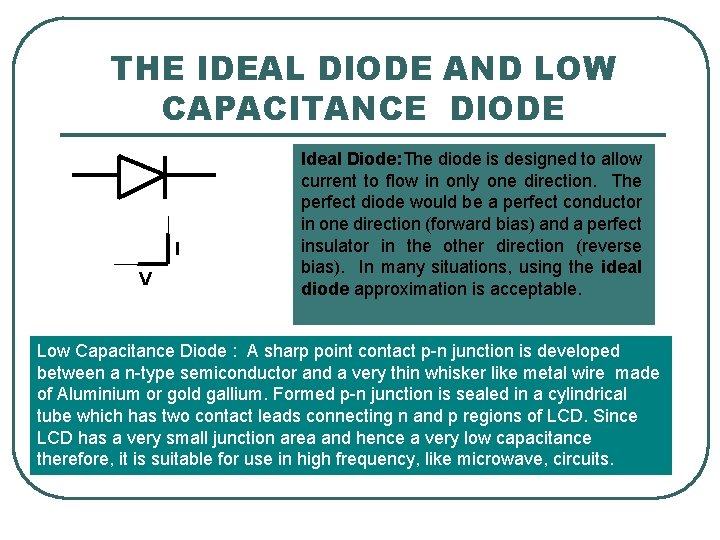
THE IDEAL DIODE AND LOW CAPACITANCE DIODE I V Ideal Diode: The diode is designed to allow current to flow in only one direction. The perfect diode would be a perfect conductor in one direction (forward bias) and a perfect insulator in the other direction (reverse bias). In many situations, using the ideal diode approximation is acceptable. Low Capacitance Diode : A sharp point contact p-n junction is developed between a n-type semiconductor and a very thin whisker like metal wire made of Aluminium or gold gallium. Formed p-n junction is sealed in a cylindrical tube which has two contact leads connecting n and p regions of LCD. Since LCD has a very small junction area and hence a very low capacitance therefore, it is suitable for use in high frequency, like microwave, circuits.
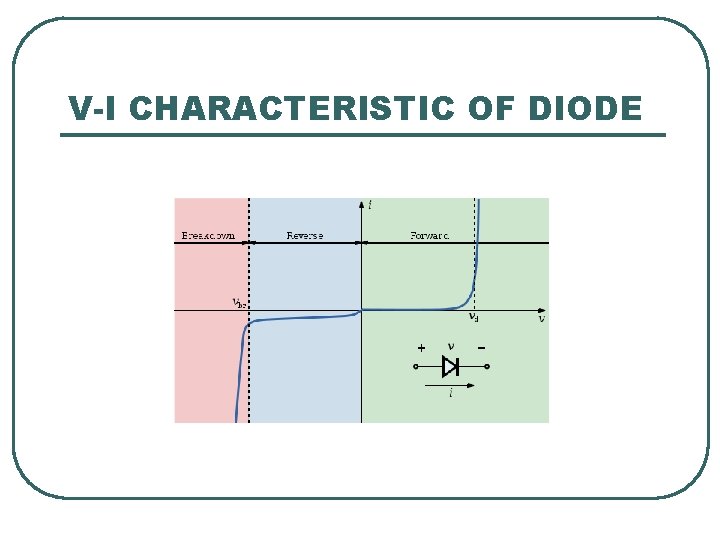
V-I CHARACTERISTIC OF DIODE
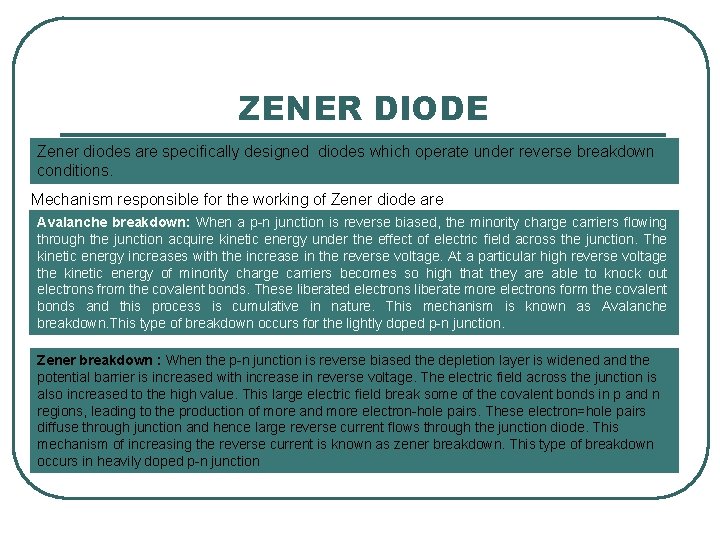
ZENER DIODE Zener diodes are specifically designed diodes which operate under reverse breakdown conditions. Mechanism responsible for the working of Zener diode are Avalanche breakdown: When a p-n junction is reverse biased, the minority charge carriers flowing through the junction acquire kinetic energy under the effect of electric field across the junction. The kinetic energy increases with the increase in the reverse voltage. At a particular high reverse voltage the kinetic energy of minority charge carriers becomes so high that they are able to knock out electrons from the covalent bonds. These liberated electrons liberate more electrons form the covalent bonds and this process is cumulative in nature. This mechanism is known as Avalanche breakdown. This type of breakdown occurs for the lightly doped p-n junction. Zener breakdown : When the p-n junction is reverse biased the depletion layer is widened and the potential barrier is increased with increase in reverse voltage. The electric field across the junction is also increased to the high value. This large electric field break some of the covalent bonds in p and n regions, leading to the production of more and more electron-hole pairs. These electron=hole pairs diffuse through junction and hence large reverse current flows through the junction diode. This mechanism of increasing the reverse current is known as zener breakdown. This type of breakdown occurs in heavily doped p-n junction
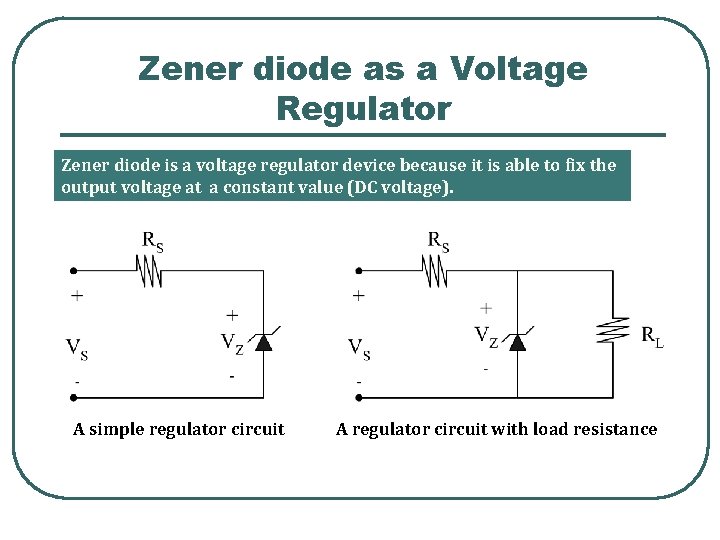
Zener diode as a Voltage Regulator Zener diode is a voltage regulator device because it is able to fix the output voltage at a constant value (DC voltage). A simple regulator circuit A regulator circuit with load resistance
Dr gopika chandra
Neonatal resuscitation definition
Dr sneha sood
Meera sood
Dopamine iv dose
Promotion from assistant to associate professor
Cuhk assistant professor salary
Diodes
Special purpose diode
What are diodes
Si
Zener diode
Diode small signal model
What are special purpose diodes
Solid state diode definition
Advantage of rectifier
Zener diode and its application
Power diodes types