THE ROLE OF PROTEIN KINASE C EPSILON IN
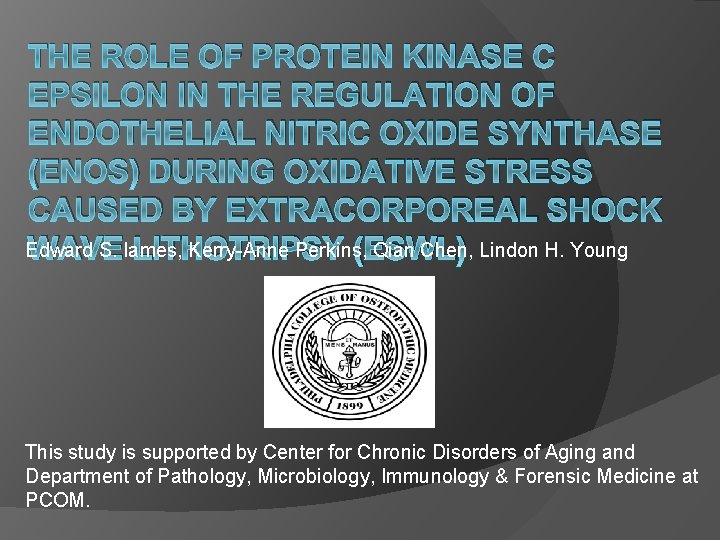
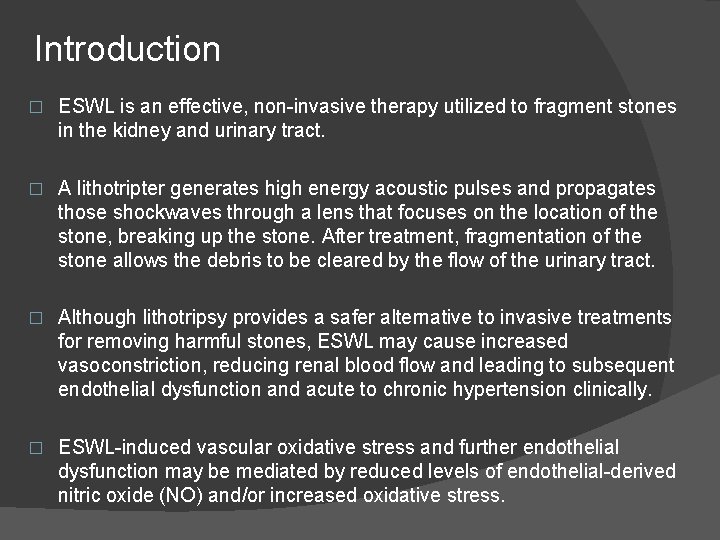
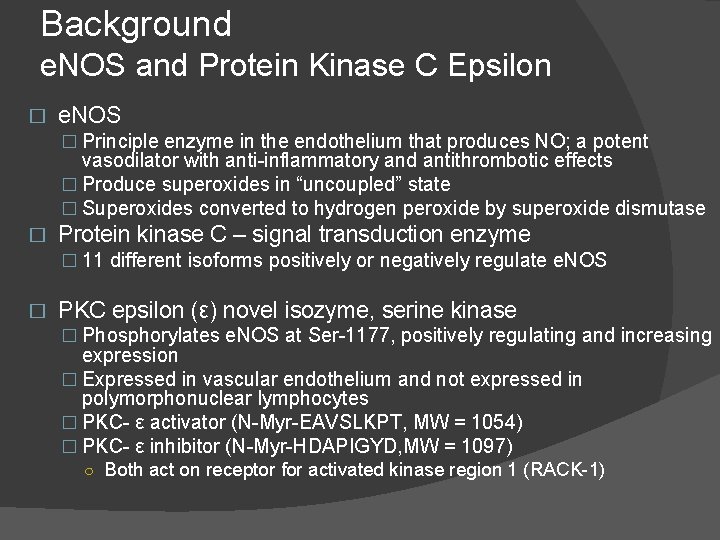
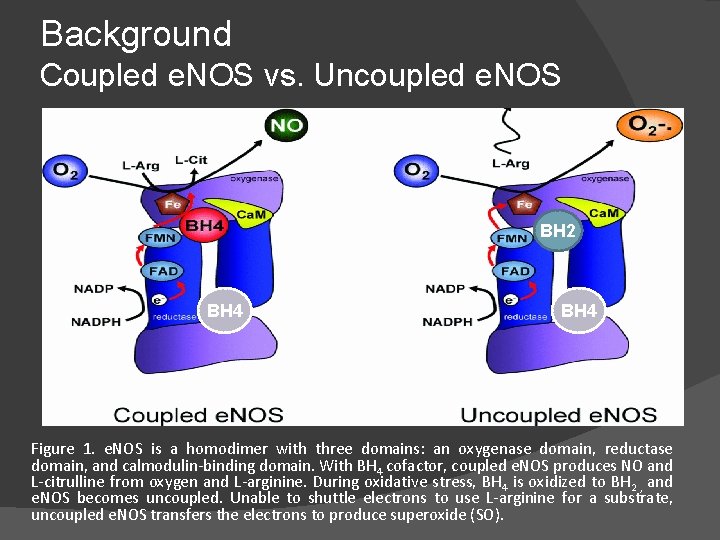
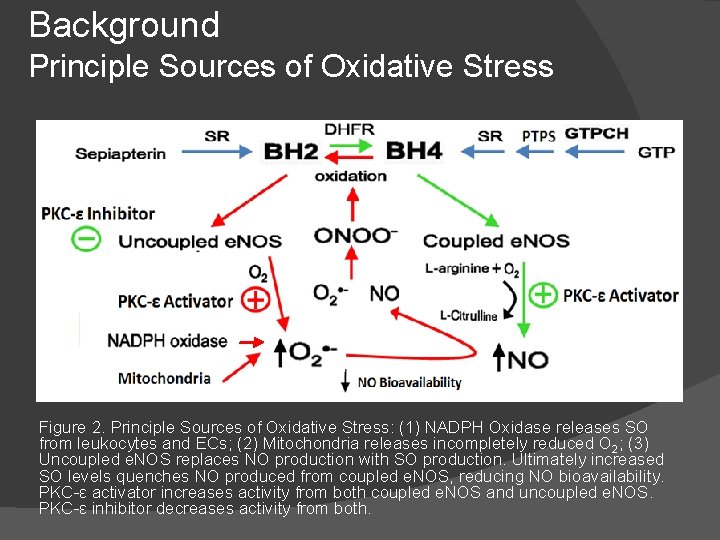
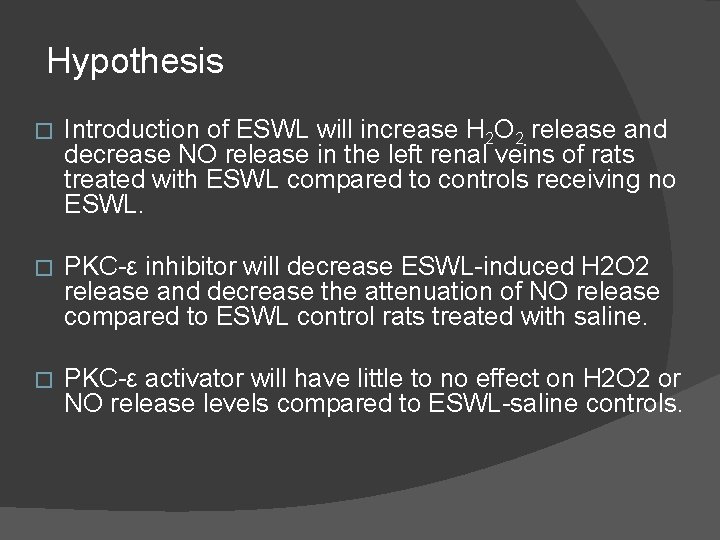
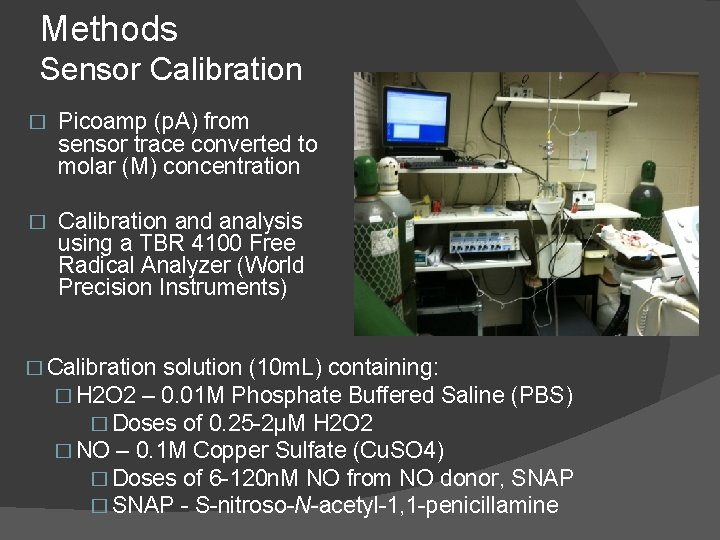
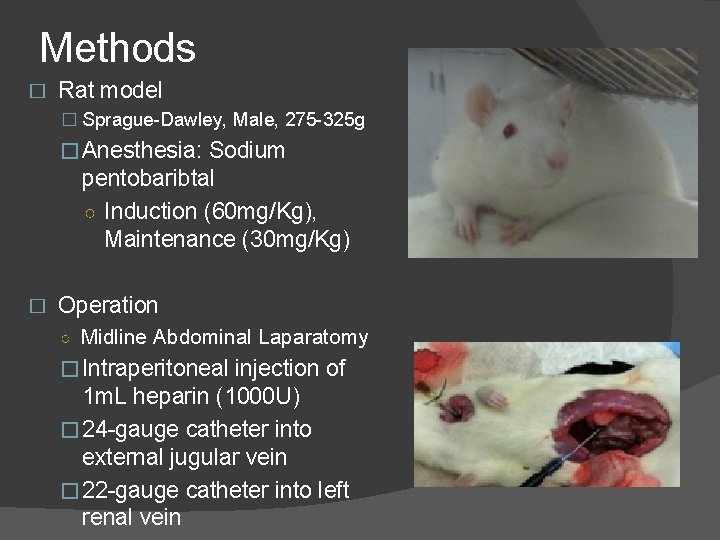
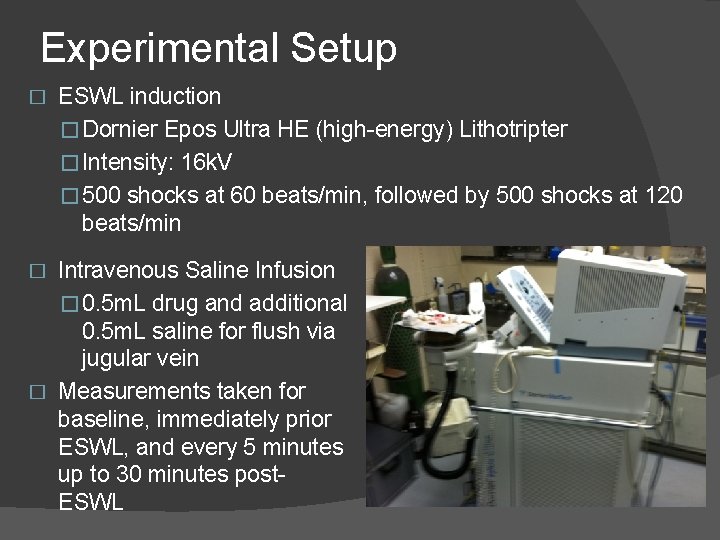
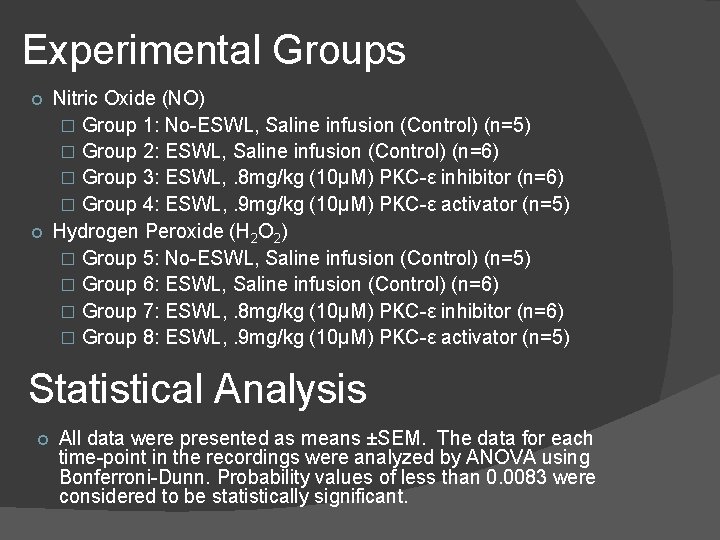
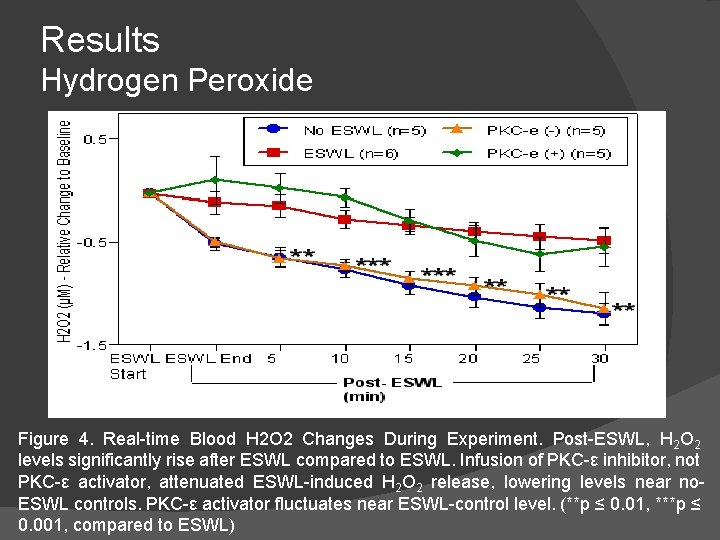
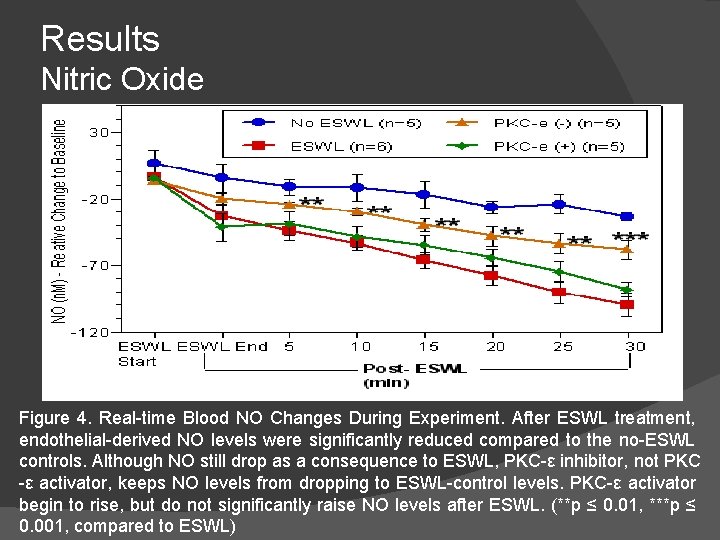
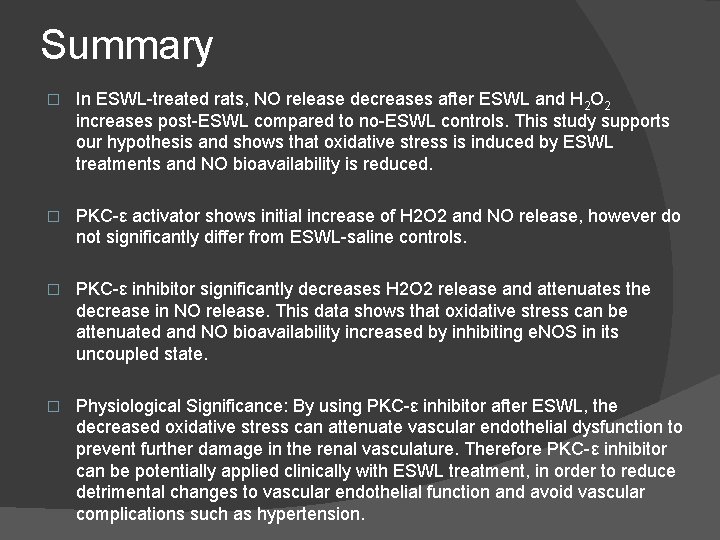
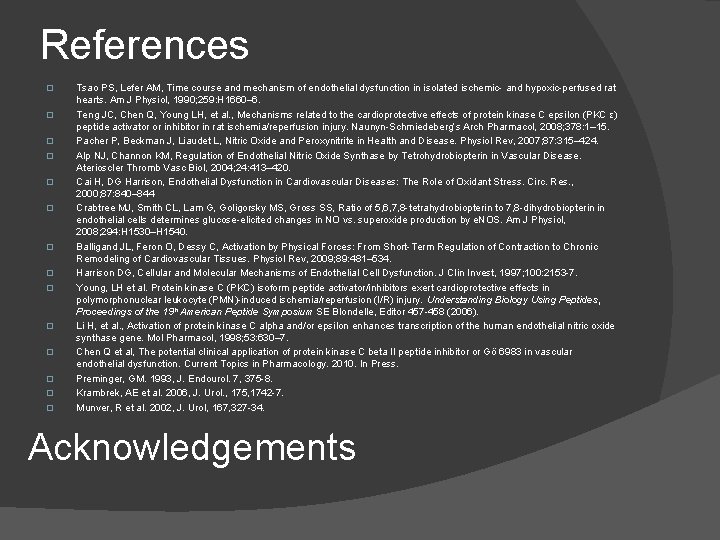
- Slides: 14
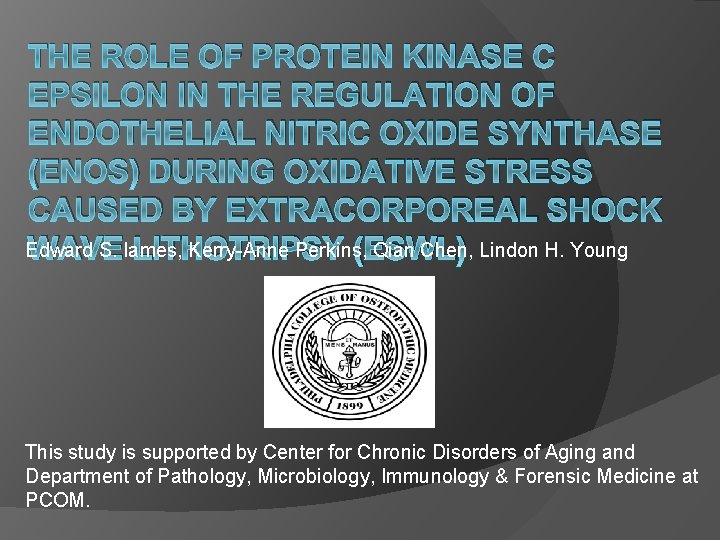
THE ROLE OF PROTEIN KINASE C EPSILON IN THE REGULATION OF ENDOTHELIAL NITRIC OXIDE SYNTHASE (ENOS) DURING OXIDATIVE STRESS CAUSED BY EXTRACORPOREAL SHOCK Edward S. Iames, Kerry-Anne Perkins, Qian Chen, Lindon H. Young WAVE LITHOTRIPSY (ESWL) This study is supported by Center for Chronic Disorders of Aging and Department of Pathology, Microbiology, Immunology & Forensic Medicine at PCOM.
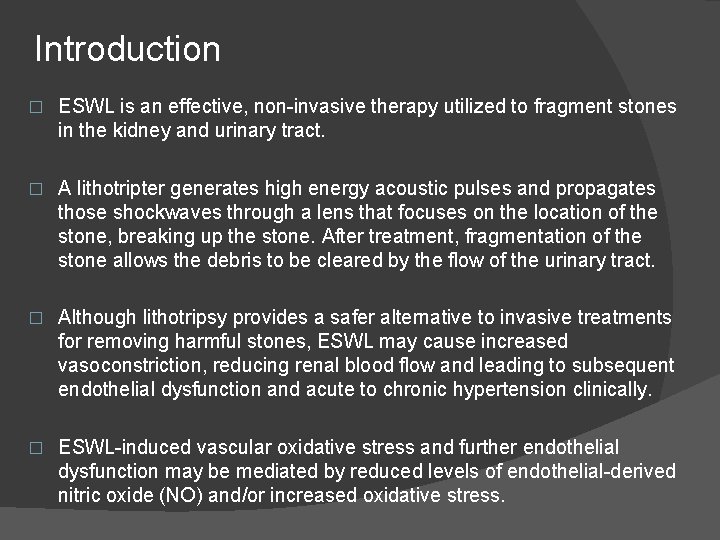
Introduction � ESWL is an effective, non-invasive therapy utilized to fragment stones in the kidney and urinary tract. � A lithotripter generates high energy acoustic pulses and propagates those shockwaves through a lens that focuses on the location of the stone, breaking up the stone. After treatment, fragmentation of the stone allows the debris to be cleared by the flow of the urinary tract. � Although lithotripsy provides a safer alternative to invasive treatments for removing harmful stones, ESWL may cause increased vasoconstriction, reducing renal blood flow and leading to subsequent endothelial dysfunction and acute to chronic hypertension clinically. � ESWL-induced vascular oxidative stress and further endothelial dysfunction may be mediated by reduced levels of endothelial-derived nitric oxide (NO) and/or increased oxidative stress.
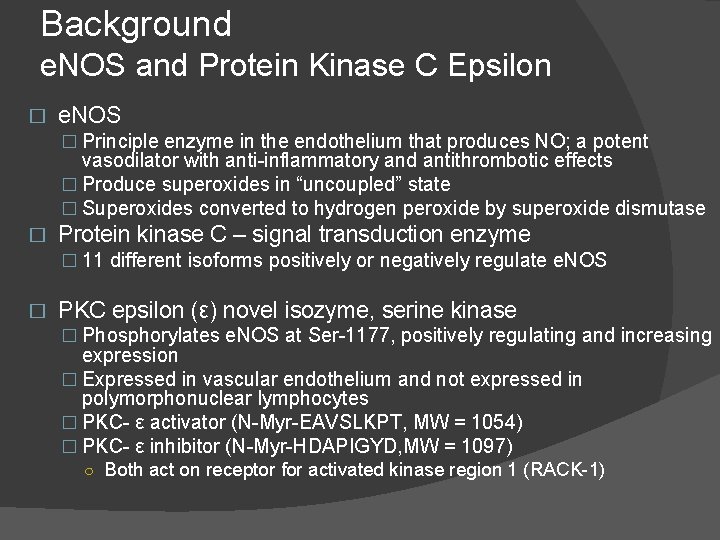
Background e. NOS and Protein Kinase C Epsilon � e. NOS � Principle enzyme in the endothelium that produces NO; a potent vasodilator with anti-inflammatory and antithrombotic effects � Produce superoxides in “uncoupled” state � Superoxides converted to hydrogen peroxide by superoxide dismutase � Protein kinase C – signal transduction enzyme � 11 different isoforms positively or negatively regulate e. NOS � PKC epsilon (ε) novel isozyme, serine kinase � Phosphorylates e. NOS at Ser-1177, positively regulating and increasing expression � Expressed in vascular endothelium and not expressed in polymorphonuclear lymphocytes � PKC- ε activator (N-Myr-EAVSLKPT, MW = 1054) � PKC- ε inhibitor (N-Myr-HDAPIGYD, MW = 1097) ○ Both act on receptor for activated kinase region 1 (RACK-1)
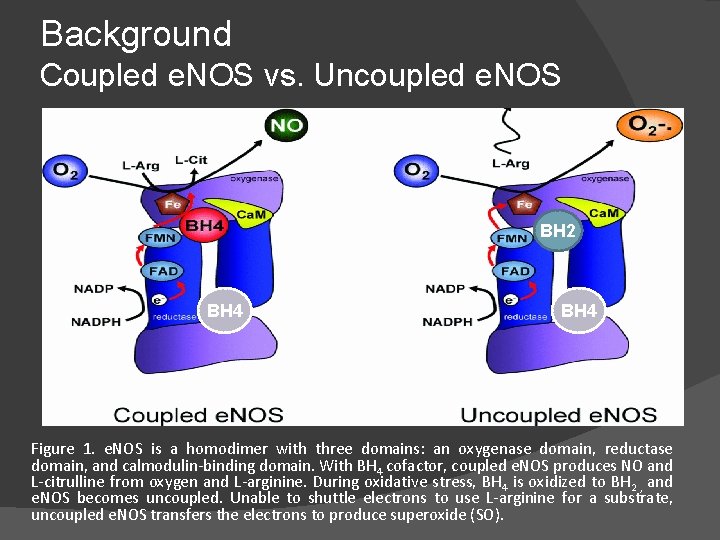
Background Coupled e. NOS vs. Uncoupled e. NOS BH 2 BH 4 Figure 1. e. NOS is a homodimer with three domains: an oxygenase domain, reductase domain, and calmodulin-binding domain. With BH 4 cofactor, coupled e. NOS produces NO and L-citrulline from oxygen and L-arginine. During oxidative stress, BH 4 is oxidized to BH 2 , and e. NOS becomes uncoupled. Unable to shuttle electrons to use L-arginine for a substrate, uncoupled e. NOS transfers the electrons to produce superoxide (SO).
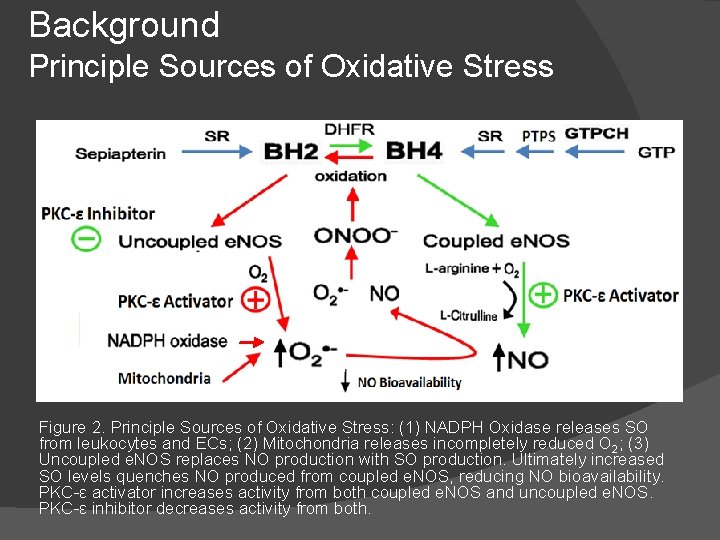
Background Principle Sources of Oxidative Stress Figure 2. Principle Sources of Oxidative Stress: (1) NADPH Oxidase releases SO from leukocytes and ECs; (2) Mitochondria releases incompletely reduced O 2; (3) Uncoupled e. NOS replaces NO production with SO production. Ultimately increased SO levels quenches NO produced from coupled e. NOS, reducing NO bioavailability. PKC-ε activator increases activity from both coupled e. NOS and uncoupled e. NOS. PKC-ε inhibitor decreases activity from both.
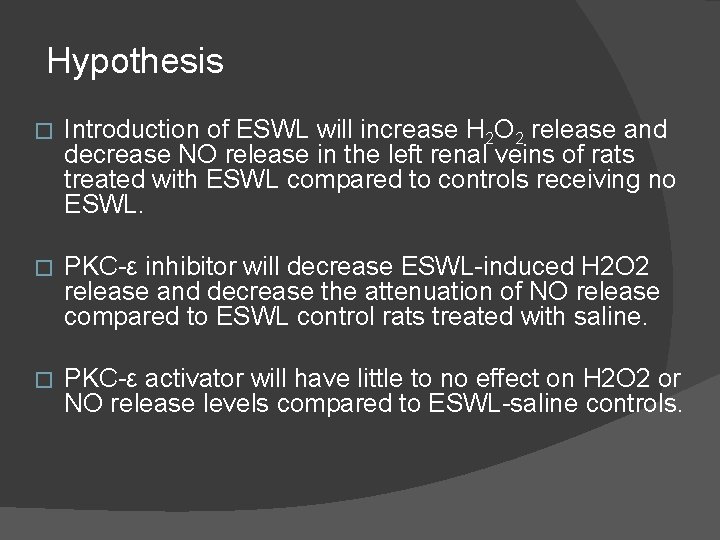
Hypothesis � Introduction of ESWL will increase H 2 O 2 release and decrease NO release in the left renal veins of rats treated with ESWL compared to controls receiving no ESWL. � PKC-ε inhibitor will decrease ESWL-induced H 2 O 2 release and decrease the attenuation of NO release compared to ESWL control rats treated with saline. � PKC-ε activator will have little to no effect on H 2 O 2 or NO release levels compared to ESWL-saline controls.
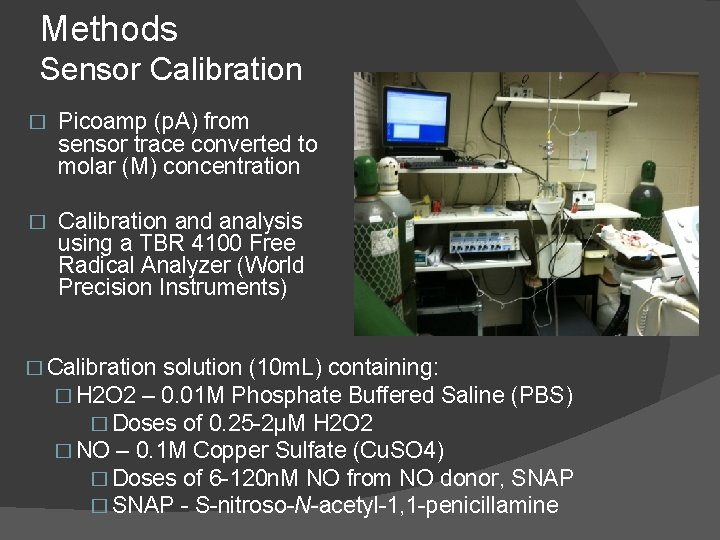
Methods Sensor Calibration � Picoamp (p. A) from sensor trace converted to molar (M) concentration � Calibration and analysis using a TBR 4100 Free Radical Analyzer (World Precision Instruments) � Calibration solution (10 m. L) containing: � H 2 O 2 – 0. 01 M Phosphate Buffered Saline (PBS) � Doses of 0. 25 -2μM H 2 O 2 � NO – 0. 1 M Copper Sulfate (Cu. SO 4) � Doses of 6 -120 n. M NO from NO donor, SNAP � SNAP - S-nitroso-N-acetyl-1, 1 -penicillamine
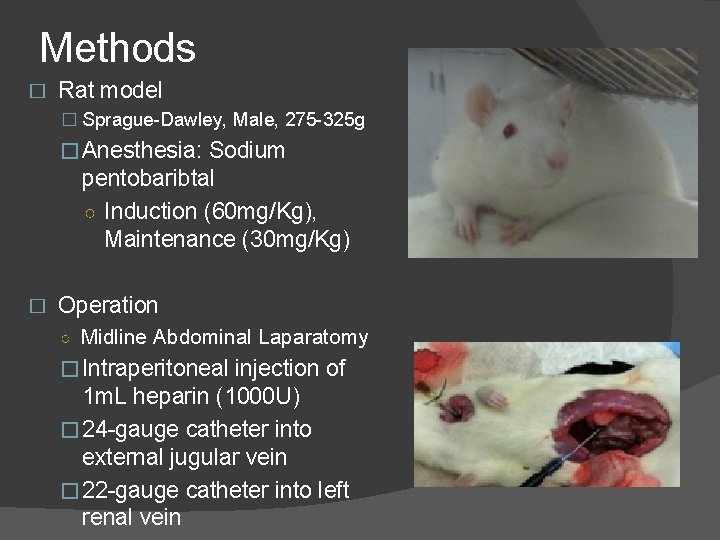
Methods � Rat model � Sprague-Dawley, Male, 275 -325 g �Anesthesia: Sodium pentobaribtal ○ Induction (60 mg/Kg), Maintenance (30 mg/Kg) � Operation ○ Midline Abdominal Laparatomy �Intraperitoneal injection of 1 m. L heparin (1000 U) � 24 -gauge catheter into external jugular vein � 22 -gauge catheter into left renal vein
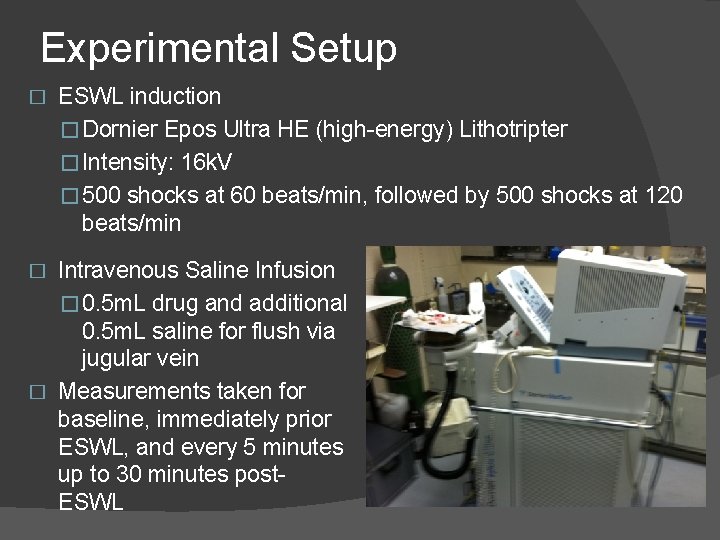
Experimental Setup � ESWL induction �Dornier Epos Ultra HE (high-energy) Lithotripter �Intensity: 16 k. V � 500 shocks at 60 beats/min, followed by 500 shocks at 120 beats/min Intravenous Saline Infusion � 0. 5 m. L drug and additional 0. 5 m. L saline for flush via jugular vein � Measurements taken for baseline, immediately prior ESWL, and every 5 minutes up to 30 minutes post. ESWL �
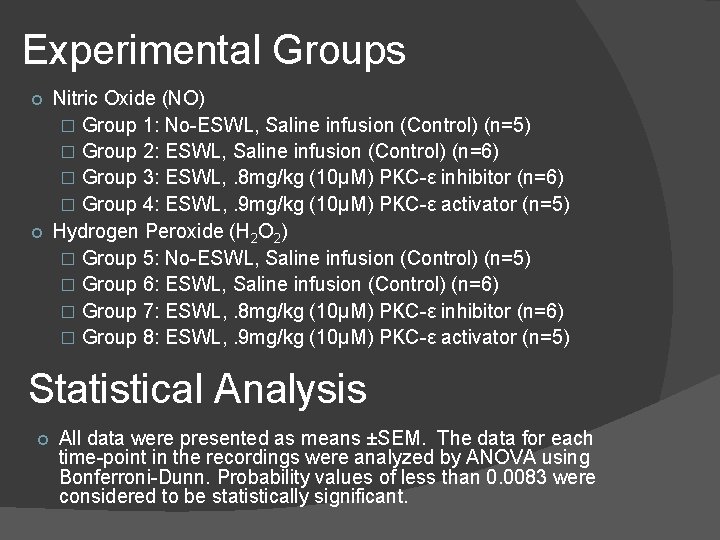
Experimental Groups Nitric Oxide (NO) � Group 1: No-ESWL, Saline infusion (Control) (n=5) � Group 2: ESWL, Saline infusion (Control) (n=6) � Group 3: ESWL, . 8 mg/kg (10μM) PKC-ε inhibitor (n=6) � Group 4: ESWL, . 9 mg/kg (10μM) PKC-ε activator (n=5) Hydrogen Peroxide (H 2 O 2) � Group 5: No-ESWL, Saline infusion (Control) (n=5) � Group 6: ESWL, Saline infusion (Control) (n=6) � Group 7: ESWL, . 8 mg/kg (10μM) PKC-ε inhibitor (n=6) � Group 8: ESWL, . 9 mg/kg (10μM) PKC-ε activator (n=5) Statistical Analysis All data were presented as means ±SEM. The data for each time-point in the recordings were analyzed by ANOVA using Bonferroni-Dunn. Probability values of less than 0. 0083 were considered to be statistically significant.
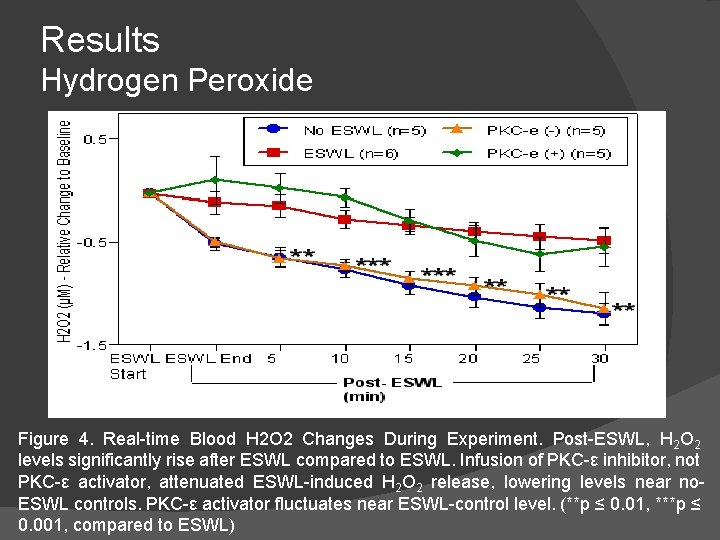
Results Hydrogen Peroxide Figure 4. Real-time Blood H 2 O 2 Changes During Experiment. Post-ESWL, H 2 O 2 levels significantly rise after ESWL compared to ESWL. Infusion of PKC-ε inhibitor, not PKC-ε activator, attenuated ESWL-induced H 2 O 2 release, lowering levels near no. ESWL controls. PKC-ε activator fluctuates near ESWL-control level. (**p ≤ 0. 01, ***p ≤ 0. 001, compared to ESWL)
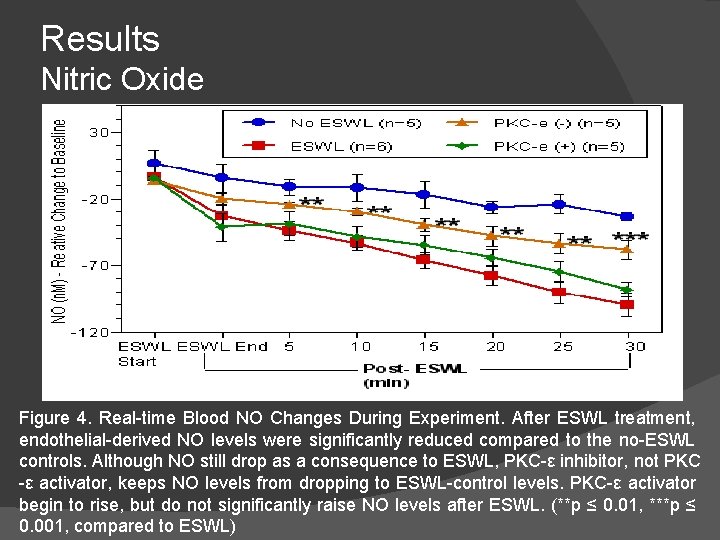
Results Nitric Oxide Figure 4. Real-time Blood NO Changes During Experiment. After ESWL treatment, endothelial-derived NO levels were significantly reduced compared to the no-ESWL controls. Although NO still drop as a consequence to ESWL, PKC-ε inhibitor, not PKC -ε activator, keeps NO levels from dropping to ESWL-control levels. PKC-ε activator begin to rise, but do not significantly raise NO levels after ESWL. (**p ≤ 0. 01, ***p ≤ 0. 001, compared to ESWL)
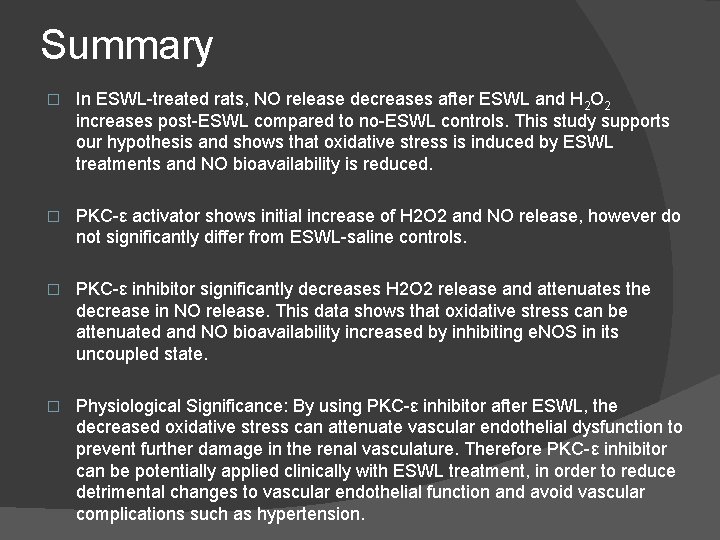
Summary � In ESWL-treated rats, NO release decreases after ESWL and H 2 O 2 increases post-ESWL compared to no-ESWL controls. This study supports our hypothesis and shows that oxidative stress is induced by ESWL treatments and NO bioavailability is reduced. � PKC-ε activator shows initial increase of H 2 O 2 and NO release, however do not significantly differ from ESWL-saline controls. � PKC-ε inhibitor significantly decreases H 2 O 2 release and attenuates the decrease in NO release. This data shows that oxidative stress can be attenuated and NO bioavailability increased by inhibiting e. NOS in its uncoupled state. � Physiological Significance: By using PKC-ε inhibitor after ESWL, the decreased oxidative stress can attenuate vascular endothelial dysfunction to prevent further damage in the renal vasculature. Therefore PKC-ε inhibitor can be potentially applied clinically with ESWL treatment, in order to reduce detrimental changes to vascular endothelial function and avoid vascular complications such as hypertension.
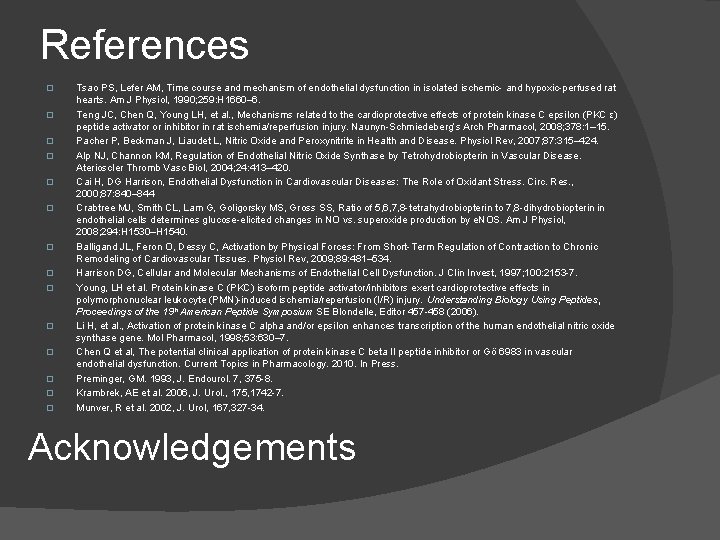
References � � � � Tsao PS, Lefer AM, Time course and mechanism of endothelial dysfunction in isolated ischemic- and hypoxic-perfused rat hearts. Am J Physiol, 1990; 259: H 1660– 6. Teng JC, Chen Q, Young LH, et al. , Mechanisms related to the cardioprotective effects of protein kinase C epsilon (PKC ε) peptide activator or inhibitor in rat ischemia/reperfusion injury. Naunyn-Schmiedeberg’s Arch Pharmacol, 2008; 378: 1– 15. Pacher P, Beckman J, Liaudet L, Nitric Oxide and Peroxynitrite in Health and Disease. Physiol Rev, 2007; 87: 315– 424. Alp NJ, Channon KM, Regulation of Endothelial Nitric Oxide Synthase by Tetrohydrobiopterin in Vascular Disease. Aterioscler Thromb Vasc Biol, 2004; 24: 413– 420. Cai H, DG Harrison, Endothelial Dysfunction in Cardiovascular Diseases: The Role of Oxidant Stress. Circ. Res. , 2000; 87: 840– 844 Crabtree MJ, Smith CL, Lam G, Goligorsky MS, Gross SS, Ratio of 5, 6, 7, 8 -tetrahydrobiopterin to 7, 8 -dihydrobiopterin in endothelial cells determines glucose-elicited changes in NO vs. superoxide production by e. NOS. Am J Physiol, 2008; 294: H 1530–H 1540. Balligand JL, Feron O, Dessy C, Activation by Physical Forces: From Short-Term Regulation of Contraction to Chronic Remodeling of Cardiovascular Tissues. Physiol Rev, 2009; 89: 481– 534. Harrison DG, Cellular and Molecular Mechanisms of Endothelial Cell Dysfunction. J Clin Invest, 1997; 100: 2153 -7. Young, LH et al. Protein kinase C (PKC) isoform peptide activator/inhibitors exert cardioprotective effects in polymorphonuclear leukocyte (PMN)-induced ischemia/reperfusion (I/R) injury. Understanding Biology Using Peptides, Proceedings of the 19 th American Peptide Symposium SE Blondelle, Editor 457 -458 (2006). Li H, et al. , Activation of protein kinase C alpha and/or epsilon enhances transcription of the human endothelial nitric oxide synthase gene. Mol Pharmacol, 1998; 53: 630– 7. Chen Q et al, The potential clinical application of protein kinase C beta II peptide inhibitor or Gö 6983 in vascular endothelial dysfunction. Current Topics in Pharmacology. 2010. In Press. Preminger, GM. 1993, J. Endourol. 7, 375 -8. Krambrek, AE et al. 2006, J. Urol. , 175, 1742 -7. Munver, R et al. 2002, J. Urol, 167, 327 -34. Acknowledgements