Soil Relations of Plants Soil structure is a
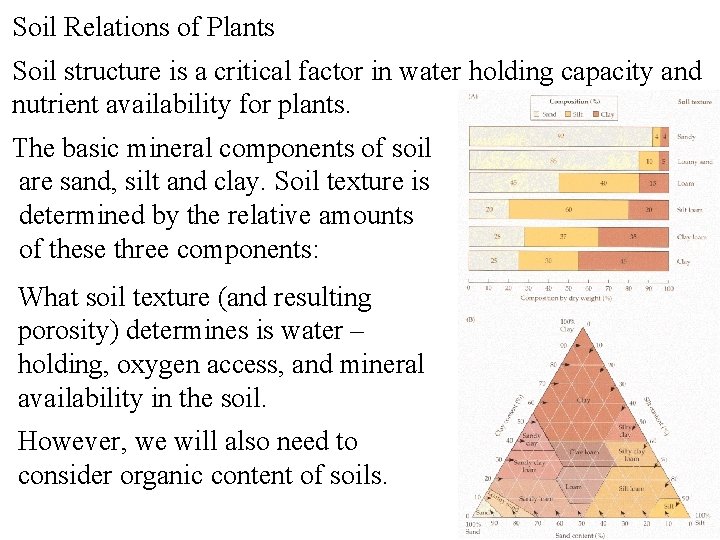
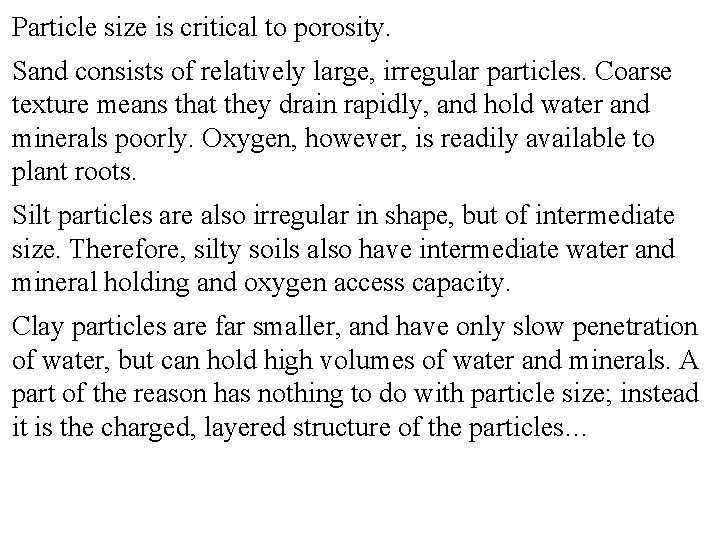
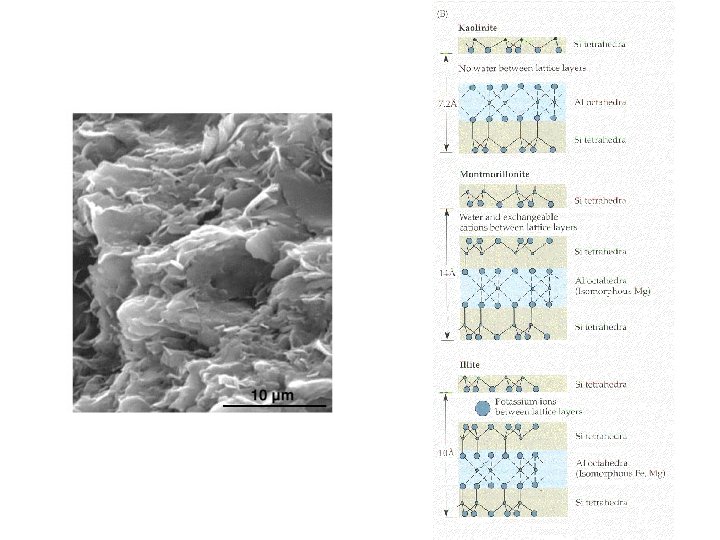
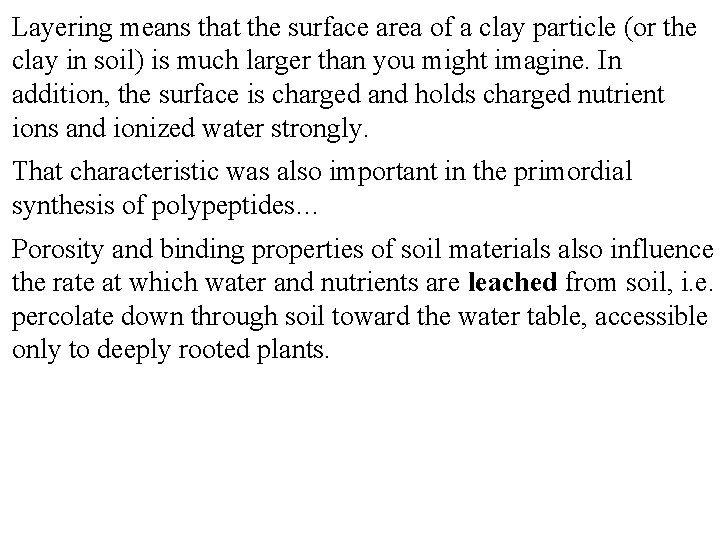
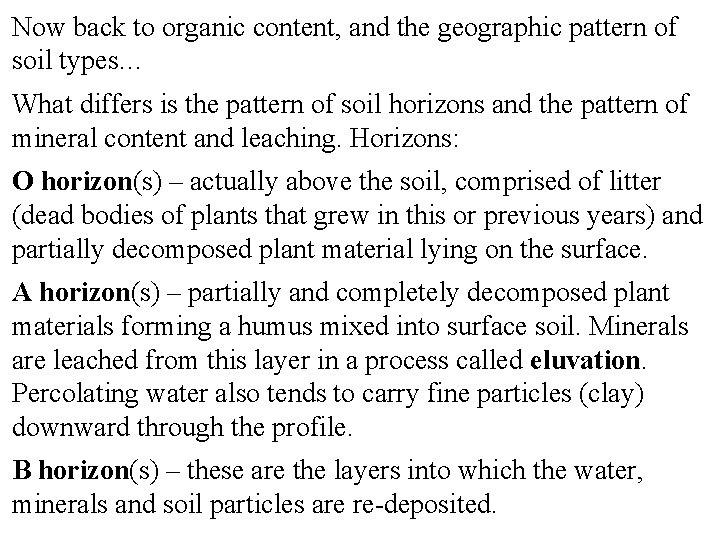
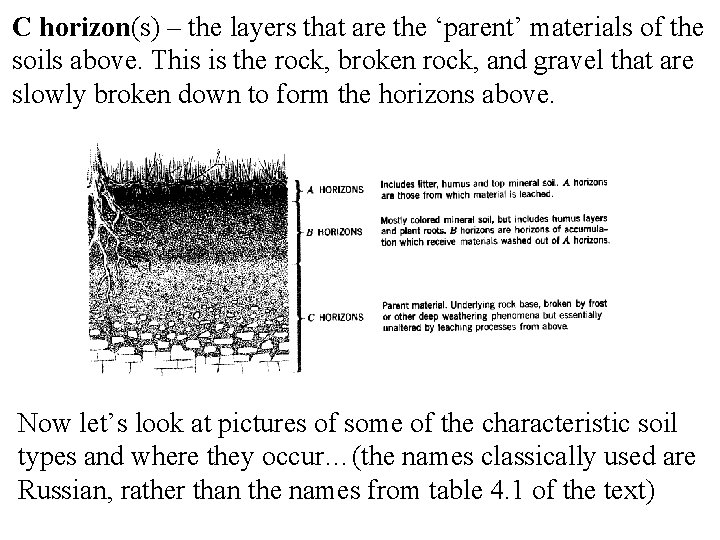
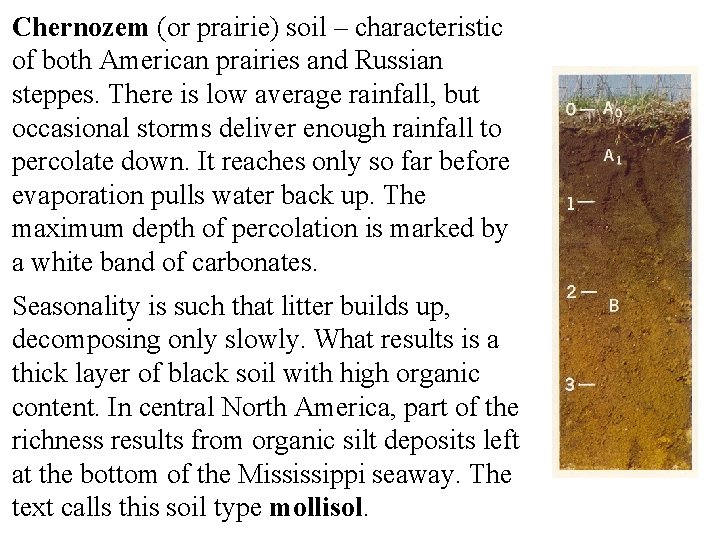
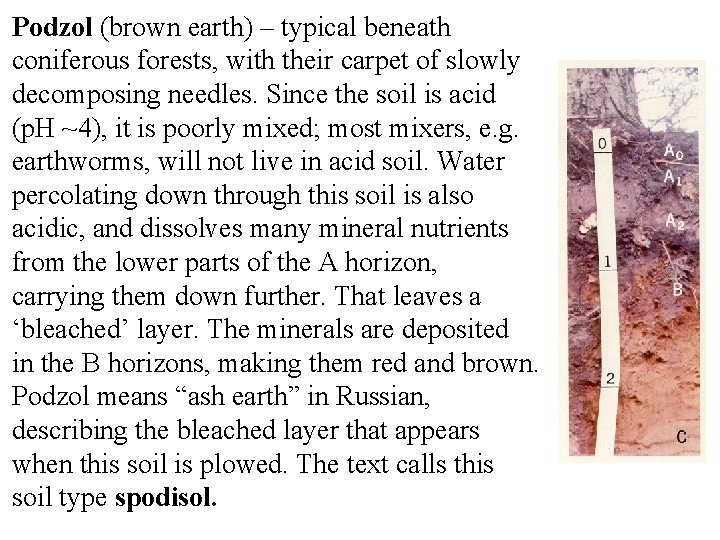
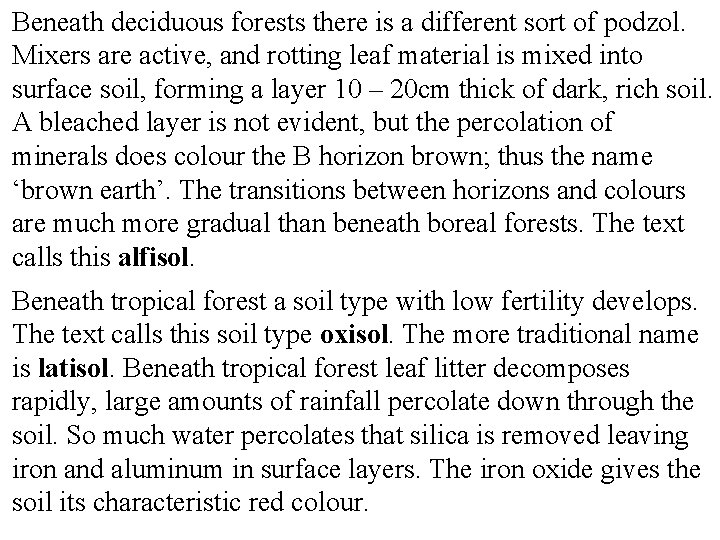
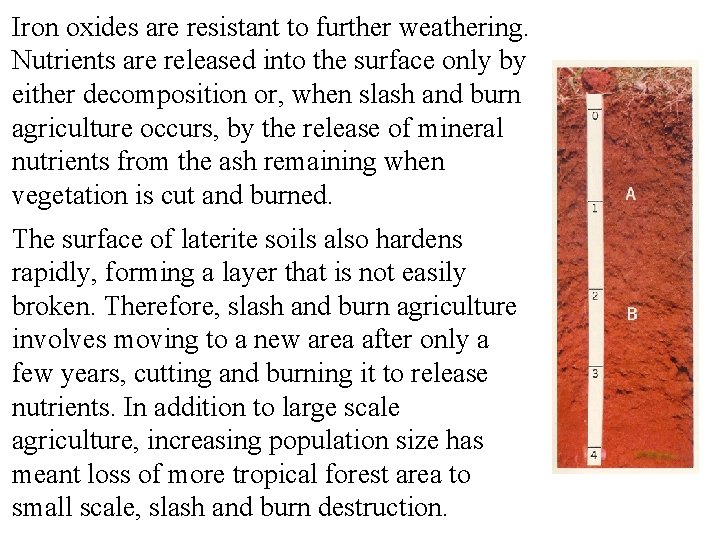
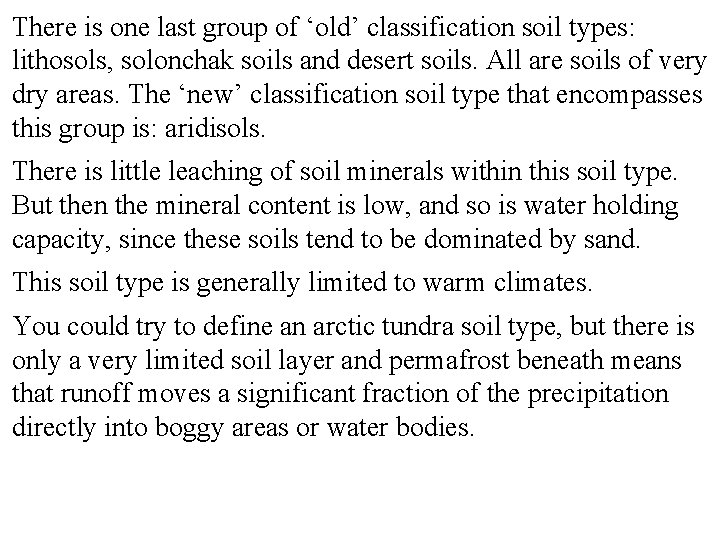
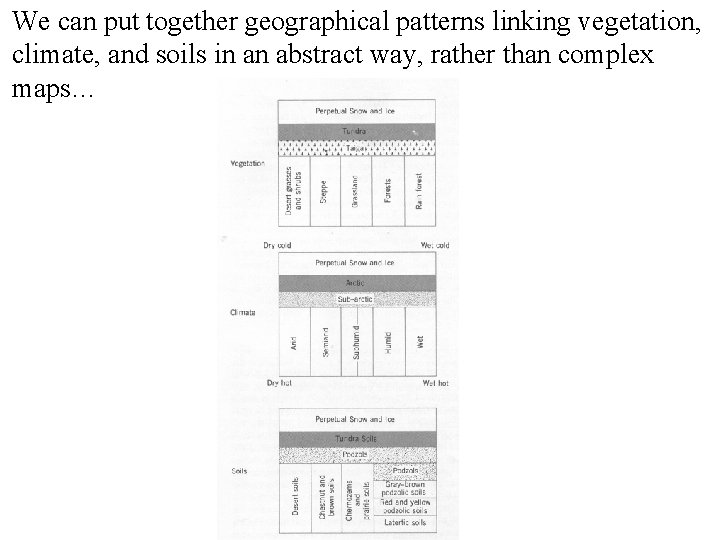
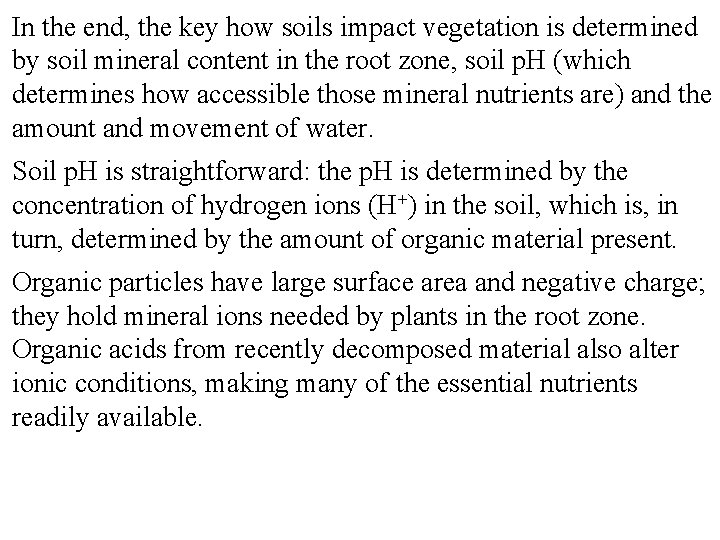
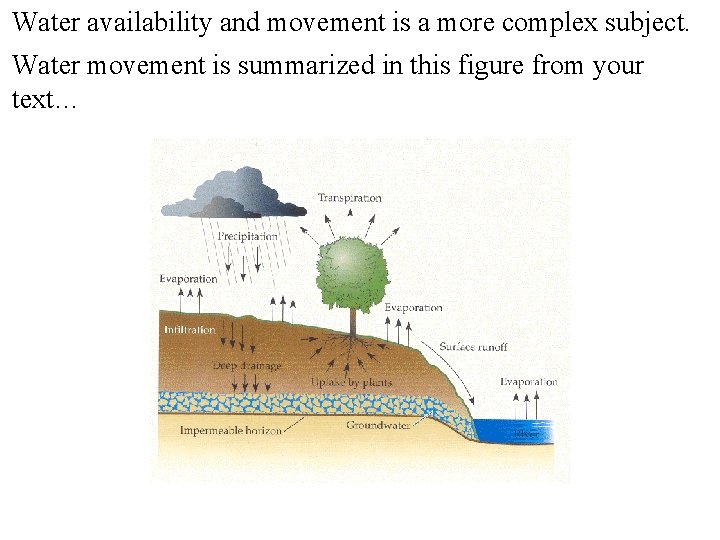
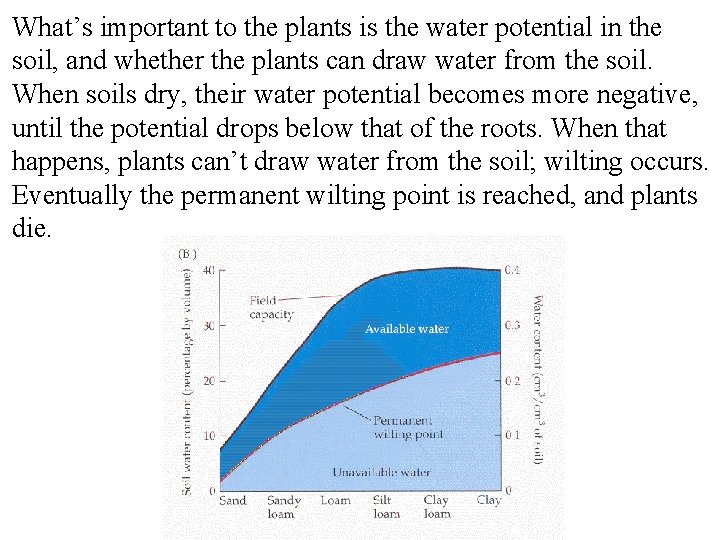
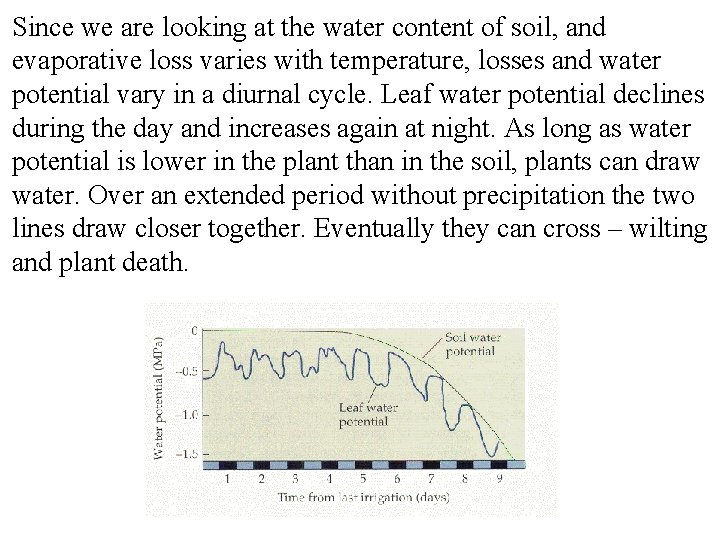
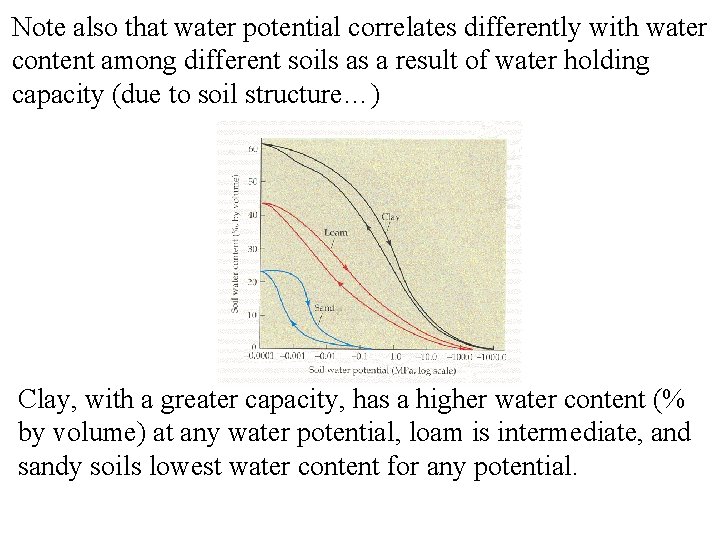
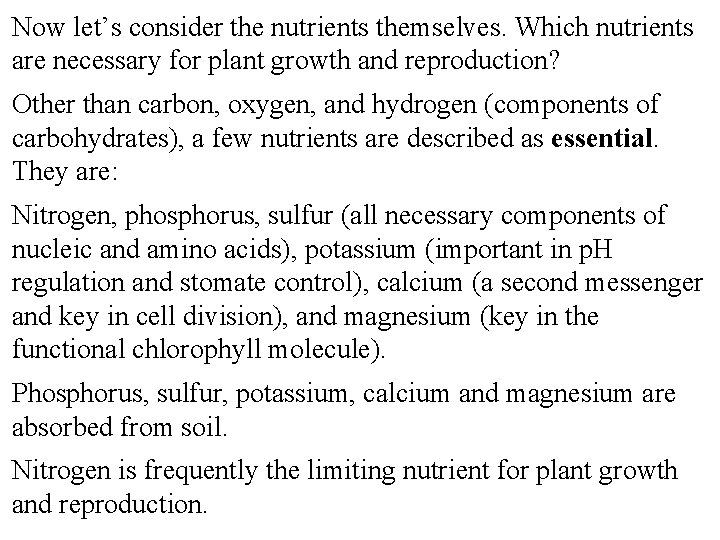
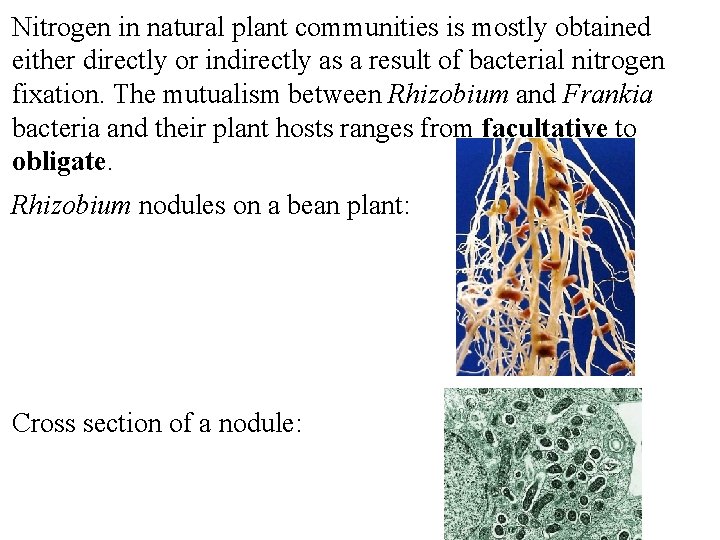
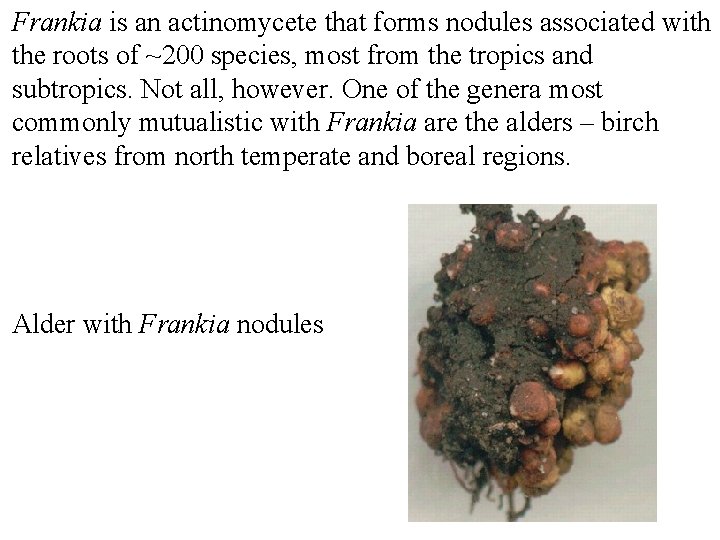
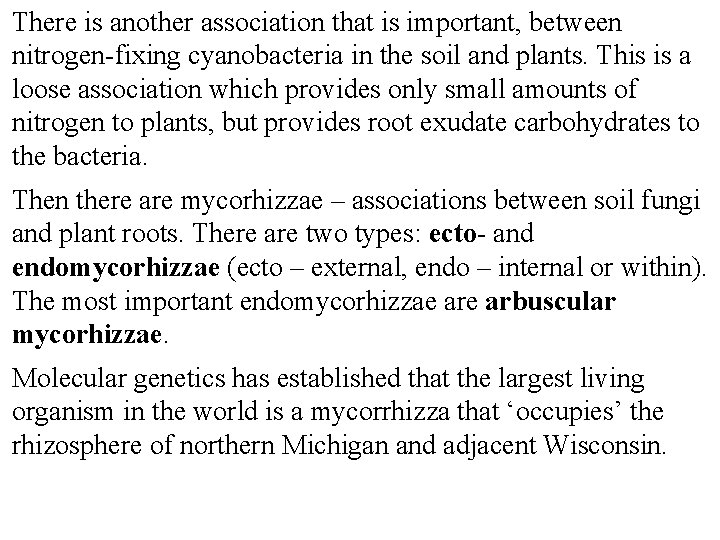
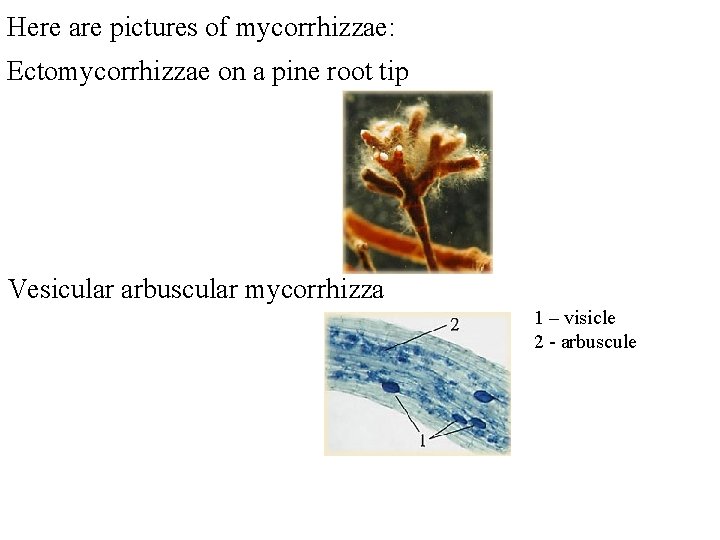
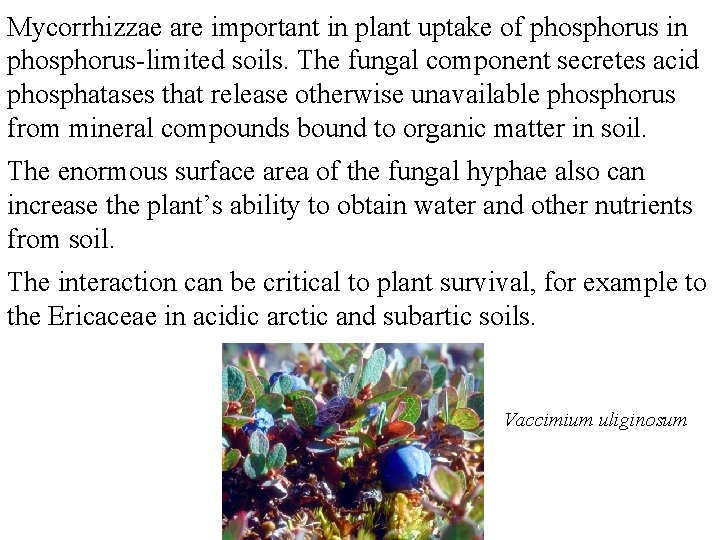
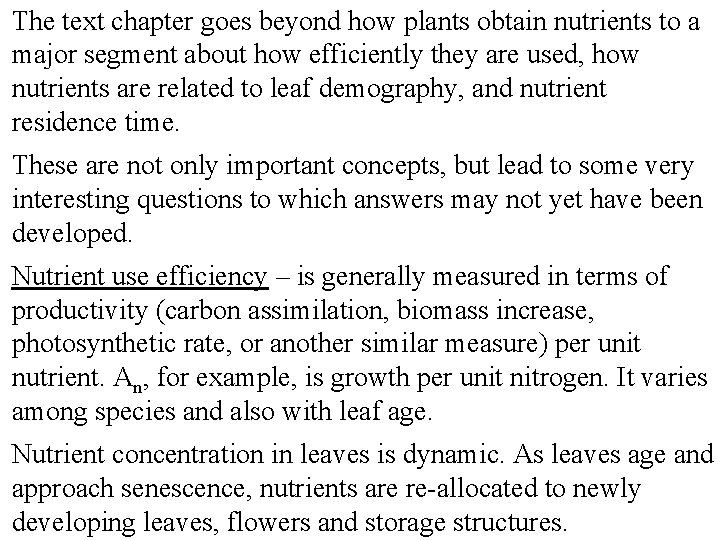
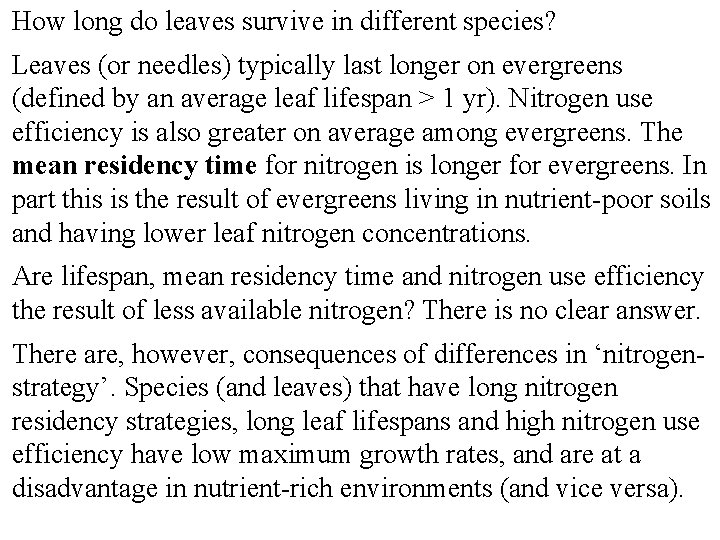
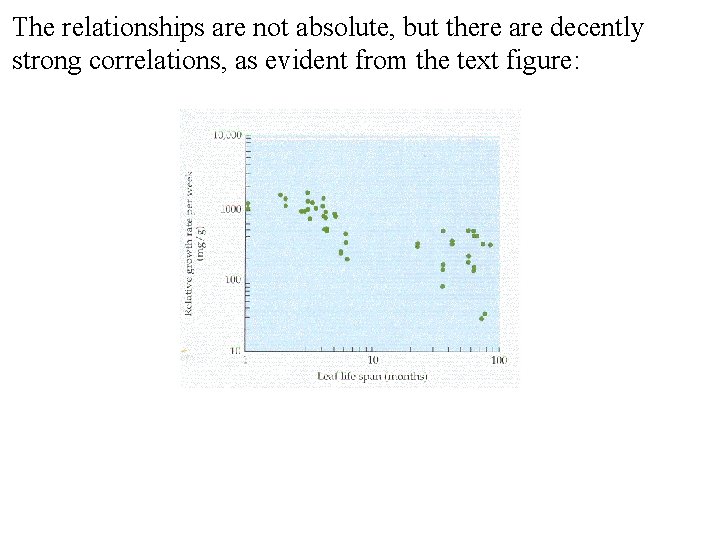
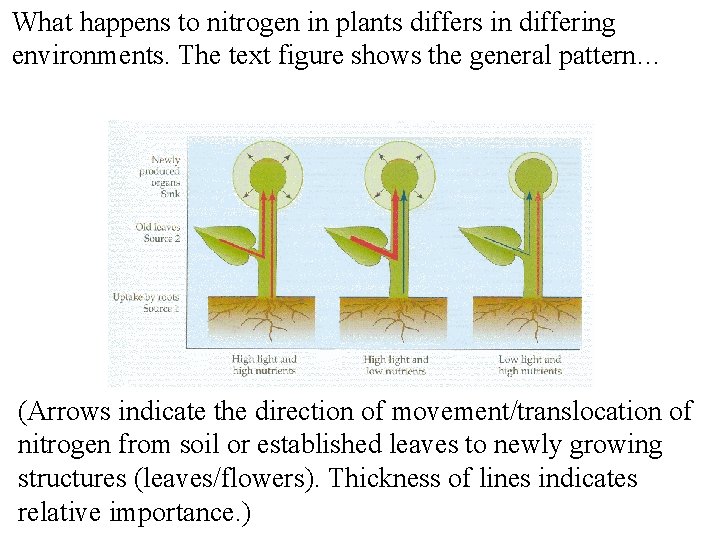
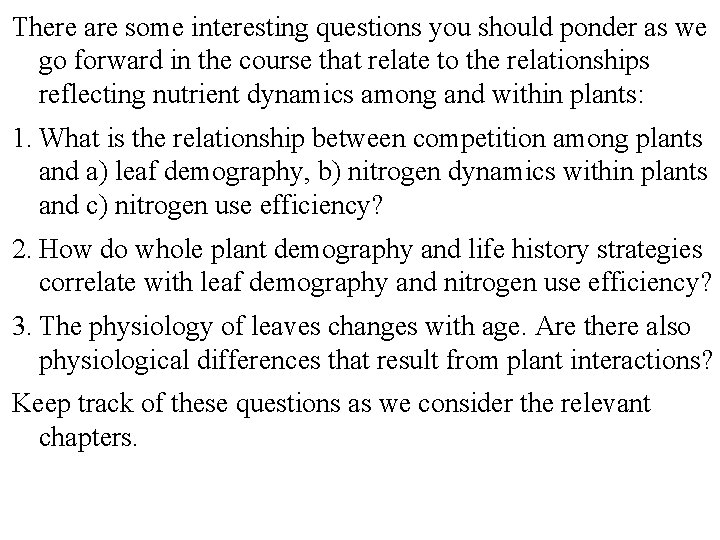
- Slides: 28
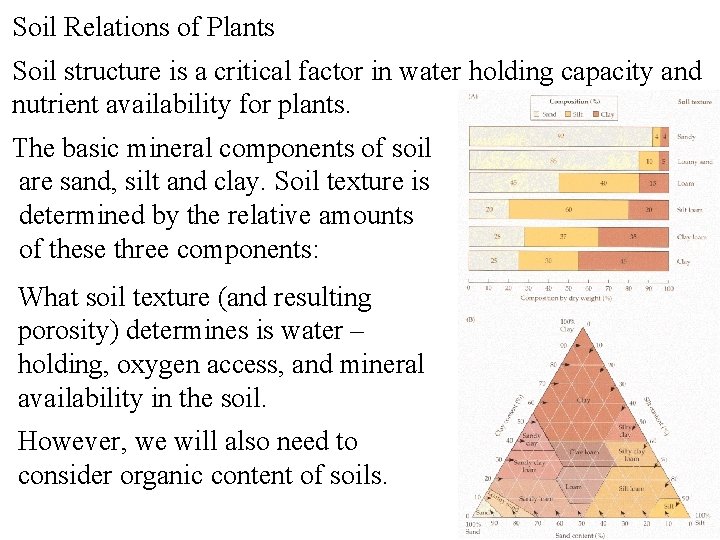
Soil Relations of Plants Soil structure is a critical factor in water holding capacity and nutrient availability for plants. The basic mineral components of soil are sand, silt and clay. Soil texture is determined by the relative amounts of these three components: What soil texture (and resulting porosity) determines is water – holding, oxygen access, and mineral availability in the soil. However, we will also need to consider organic content of soils.
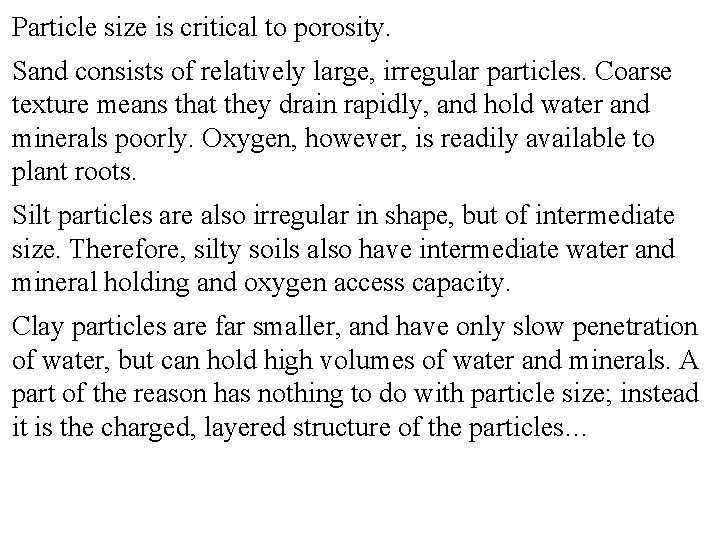
Particle size is critical to porosity. Sand consists of relatively large, irregular particles. Coarse texture means that they drain rapidly, and hold water and minerals poorly. Oxygen, however, is readily available to plant roots. Silt particles are also irregular in shape, but of intermediate size. Therefore, silty soils also have intermediate water and mineral holding and oxygen access capacity. Clay particles are far smaller, and have only slow penetration of water, but can hold high volumes of water and minerals. A part of the reason has nothing to do with particle size; instead it is the charged, layered structure of the particles…
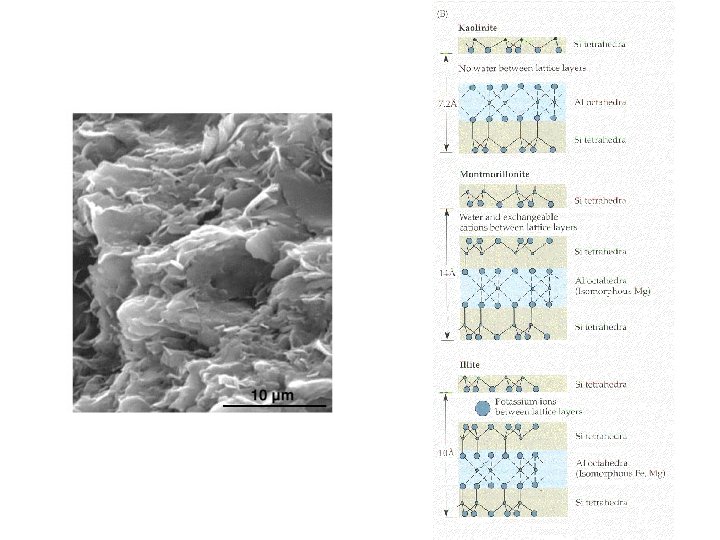
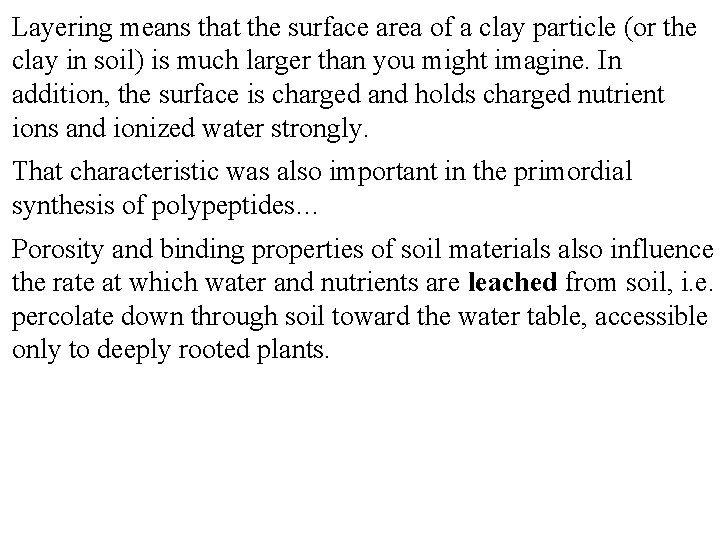
Layering means that the surface area of a clay particle (or the clay in soil) is much larger than you might imagine. In addition, the surface is charged and holds charged nutrient ions and ionized water strongly. That characteristic was also important in the primordial synthesis of polypeptides… Porosity and binding properties of soil materials also influence the rate at which water and nutrients are leached from soil, i. e. percolate down through soil toward the water table, accessible only to deeply rooted plants.
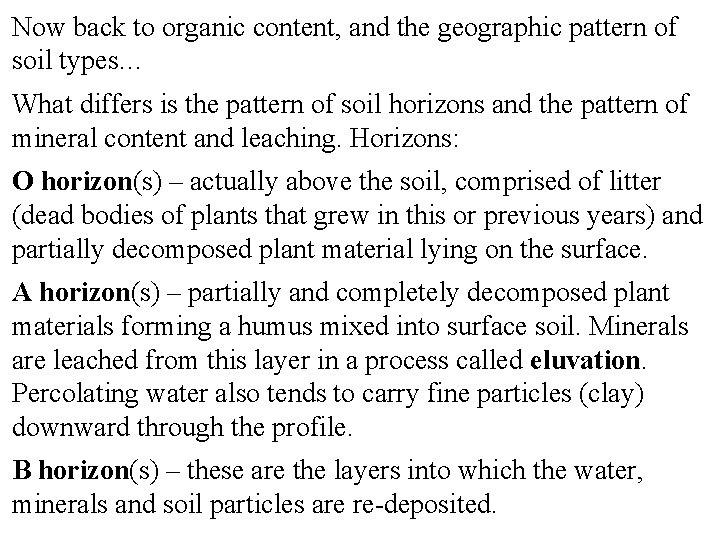
Now back to organic content, and the geographic pattern of soil types… What differs is the pattern of soil horizons and the pattern of mineral content and leaching. Horizons: O horizon(s) – actually above the soil, comprised of litter (dead bodies of plants that grew in this or previous years) and partially decomposed plant material lying on the surface. A horizon(s) – partially and completely decomposed plant materials forming a humus mixed into surface soil. Minerals are leached from this layer in a process called eluvation. Percolating water also tends to carry fine particles (clay) downward through the profile. B horizon(s) – these are the layers into which the water, minerals and soil particles are re-deposited.
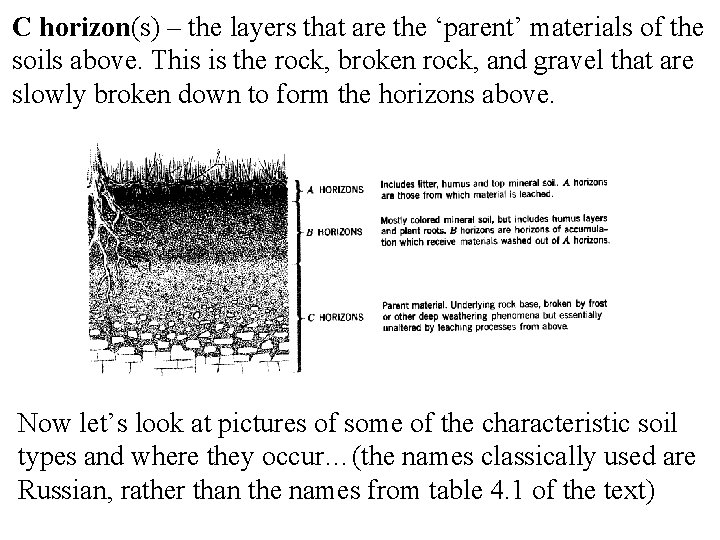
C horizon(s) – the layers that are the ‘parent’ materials of the soils above. This is the rock, broken rock, and gravel that are slowly broken down to form the horizons above. Now let’s look at pictures of some of the characteristic soil types and where they occur…(the names classically used are Russian, rather than the names from table 4. 1 of the text)
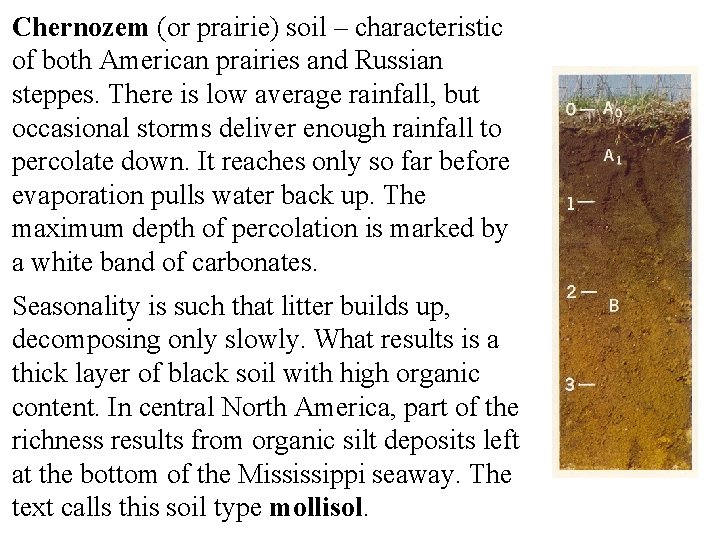
Chernozem (or prairie) soil – characteristic of both American prairies and Russian steppes. There is low average rainfall, but occasional storms deliver enough rainfall to percolate down. It reaches only so far before evaporation pulls water back up. The maximum depth of percolation is marked by a white band of carbonates. Seasonality is such that litter builds up, decomposing only slowly. What results is a thick layer of black soil with high organic content. In central North America, part of the richness results from organic silt deposits left at the bottom of the Mississippi seaway. The text calls this soil type mollisol.
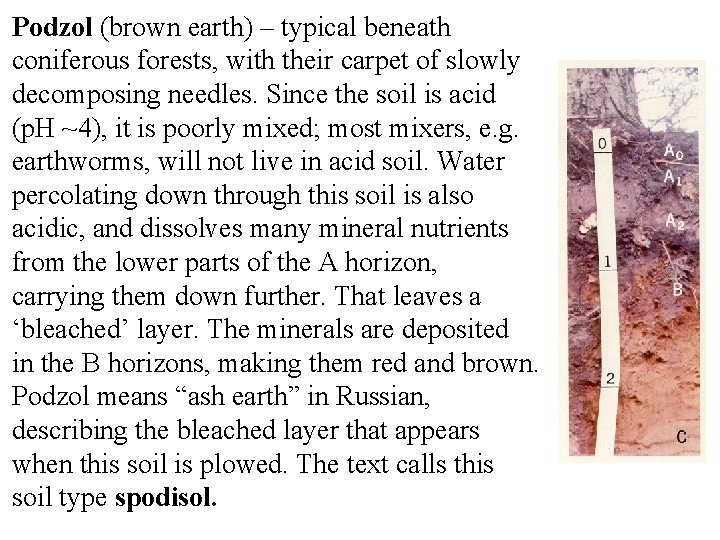
Podzol (brown earth) – typical beneath coniferous forests, with their carpet of slowly decomposing needles. Since the soil is acid (p. H ~4), it is poorly mixed; most mixers, e. g. earthworms, will not live in acid soil. Water percolating down through this soil is also acidic, and dissolves many mineral nutrients from the lower parts of the A horizon, carrying them down further. That leaves a ‘bleached’ layer. The minerals are deposited in the B horizons, making them red and brown. Podzol means “ash earth” in Russian, describing the bleached layer that appears when this soil is plowed. The text calls this soil type spodisol.
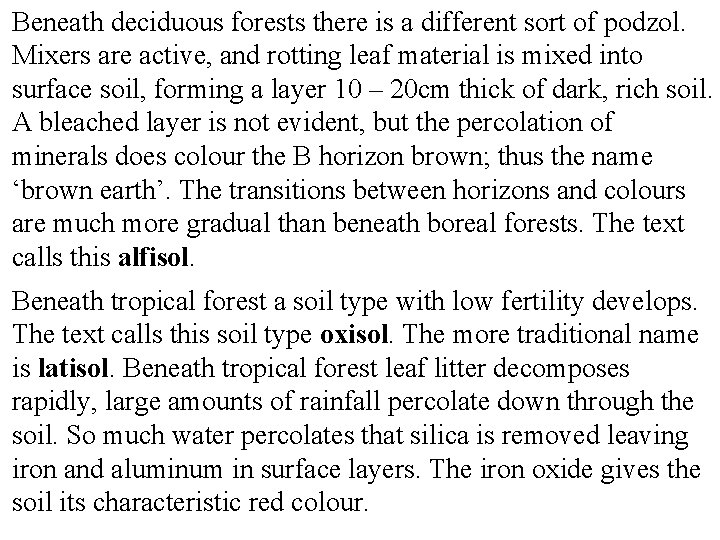
Beneath deciduous forests there is a different sort of podzol. Mixers are active, and rotting leaf material is mixed into surface soil, forming a layer 10 – 20 cm thick of dark, rich soil. A bleached layer is not evident, but the percolation of minerals does colour the B horizon brown; thus the name ‘brown earth’. The transitions between horizons and colours are much more gradual than beneath boreal forests. The text calls this alfisol. Beneath tropical forest a soil type with low fertility develops. The text calls this soil type oxisol. The more traditional name is latisol. Beneath tropical forest leaf litter decomposes rapidly, large amounts of rainfall percolate down through the soil. So much water percolates that silica is removed leaving iron and aluminum in surface layers. The iron oxide gives the soil its characteristic red colour.
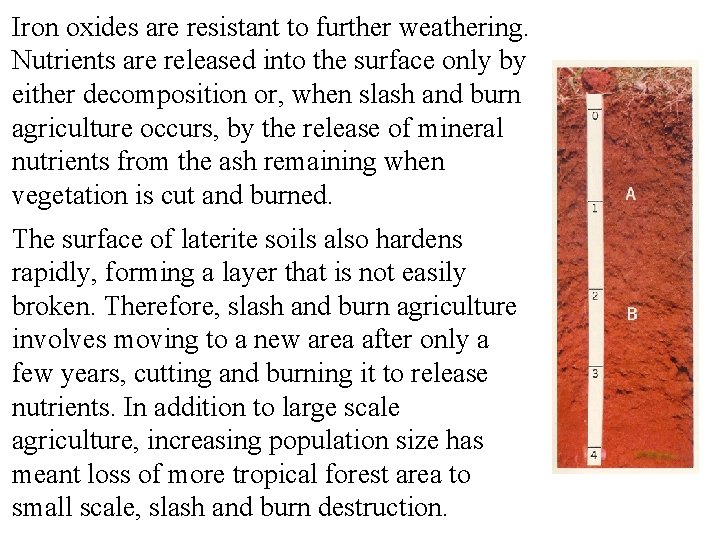
Iron oxides are resistant to further weathering. Nutrients are released into the surface only by either decomposition or, when slash and burn agriculture occurs, by the release of mineral nutrients from the ash remaining when vegetation is cut and burned. The surface of laterite soils also hardens rapidly, forming a layer that is not easily broken. Therefore, slash and burn agriculture involves moving to a new area after only a few years, cutting and burning it to release nutrients. In addition to large scale agriculture, increasing population size has meant loss of more tropical forest area to small scale, slash and burn destruction.
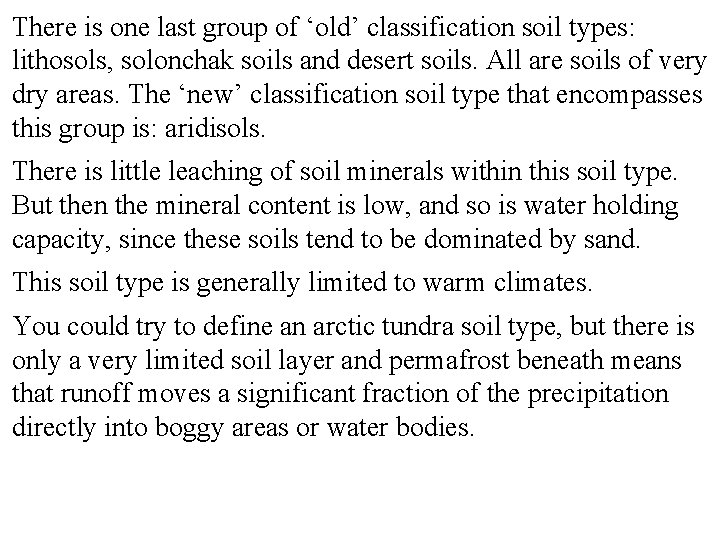
There is one last group of ‘old’ classification soil types: lithosols, solonchak soils and desert soils. All are soils of very dry areas. The ‘new’ classification soil type that encompasses this group is: aridisols. There is little leaching of soil minerals within this soil type. But then the mineral content is low, and so is water holding capacity, since these soils tend to be dominated by sand. This soil type is generally limited to warm climates. You could try to define an arctic tundra soil type, but there is only a very limited soil layer and permafrost beneath means that runoff moves a significant fraction of the precipitation directly into boggy areas or water bodies.
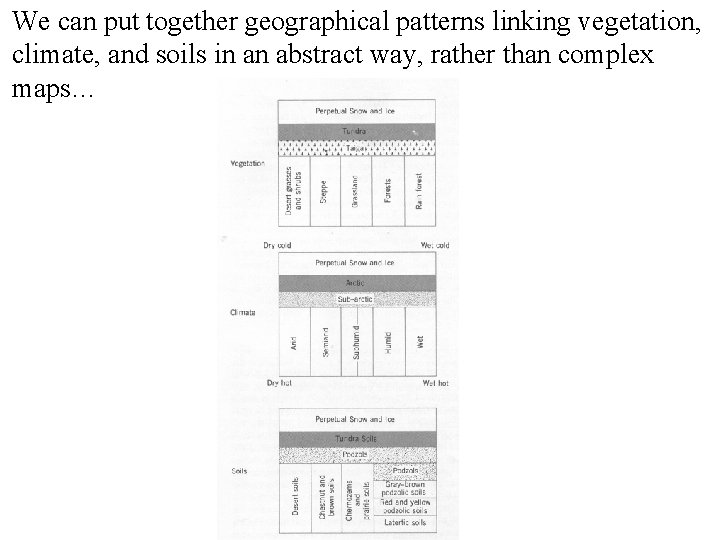
We can put together geographical patterns linking vegetation, climate, and soils in an abstract way, rather than complex maps…
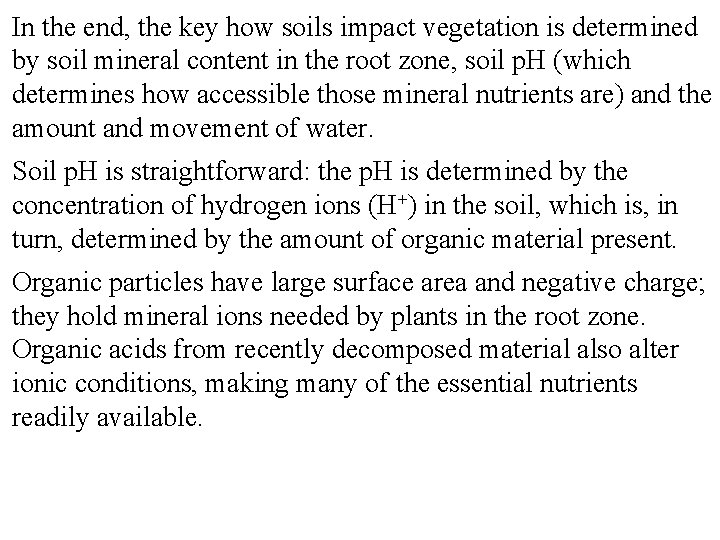
In the end, the key how soils impact vegetation is determined by soil mineral content in the root zone, soil p. H (which determines how accessible those mineral nutrients are) and the amount and movement of water. Soil p. H is straightforward: the p. H is determined by the concentration of hydrogen ions (H+) in the soil, which is, in turn, determined by the amount of organic material present. Organic particles have large surface area and negative charge; they hold mineral ions needed by plants in the root zone. Organic acids from recently decomposed material also alter ionic conditions, making many of the essential nutrients readily available.
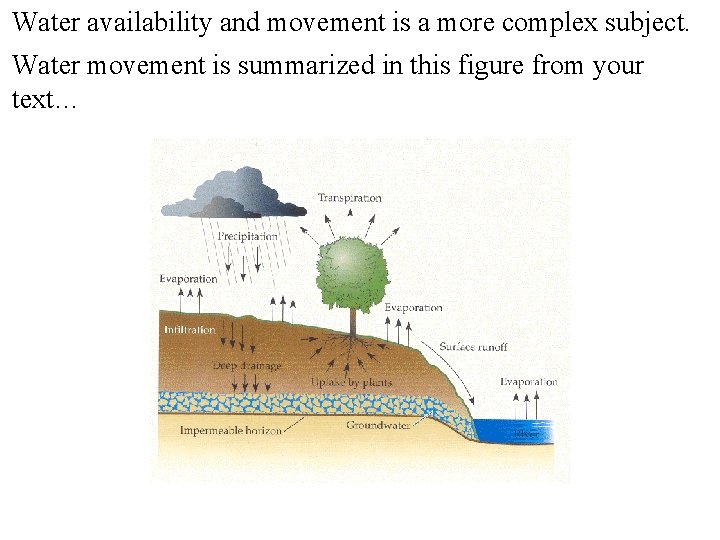
Water availability and movement is a more complex subject. Water movement is summarized in this figure from your text…
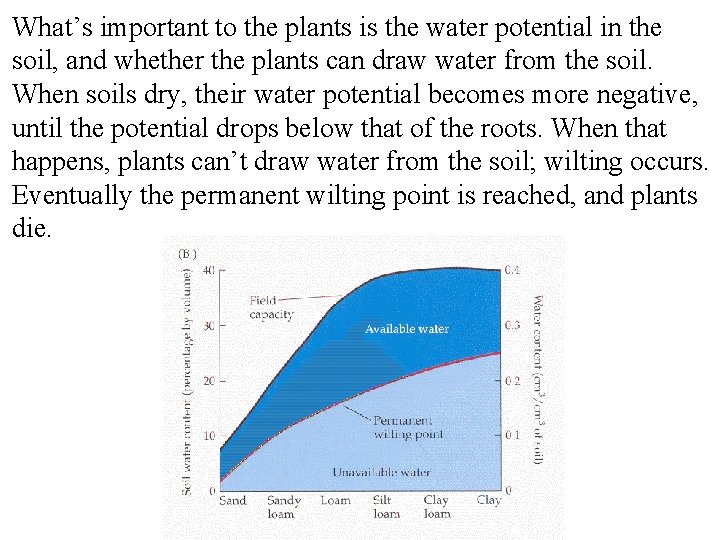
What’s important to the plants is the water potential in the soil, and whether the plants can draw water from the soil. When soils dry, their water potential becomes more negative, until the potential drops below that of the roots. When that happens, plants can’t draw water from the soil; wilting occurs. Eventually the permanent wilting point is reached, and plants die.
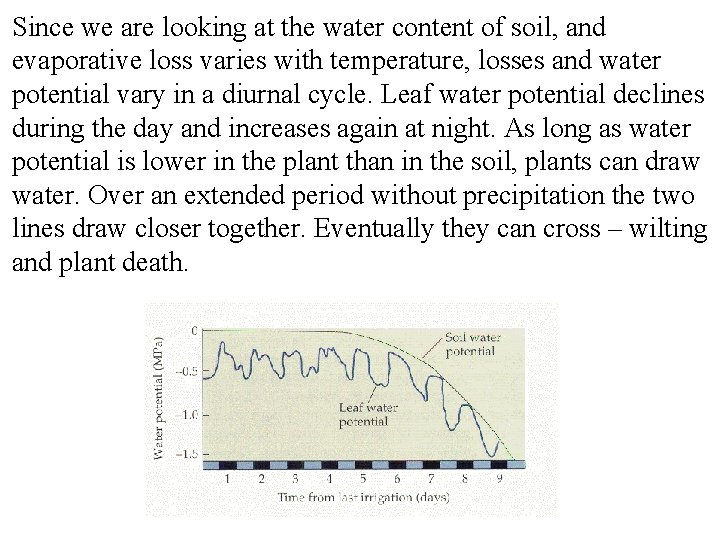
Since we are looking at the water content of soil, and evaporative loss varies with temperature, losses and water potential vary in a diurnal cycle. Leaf water potential declines during the day and increases again at night. As long as water potential is lower in the plant than in the soil, plants can draw water. Over an extended period without precipitation the two lines draw closer together. Eventually they can cross – wilting and plant death.
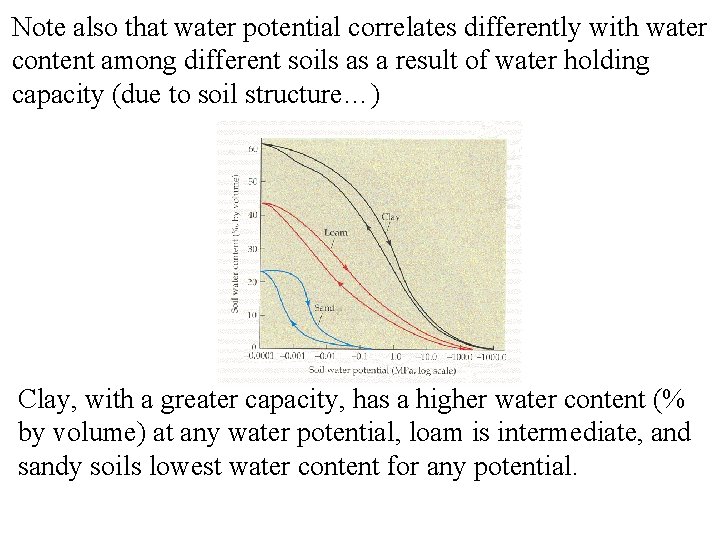
Note also that water potential correlates differently with water content among different soils as a result of water holding capacity (due to soil structure…) Clay, with a greater capacity, has a higher water content (% by volume) at any water potential, loam is intermediate, and sandy soils lowest water content for any potential.
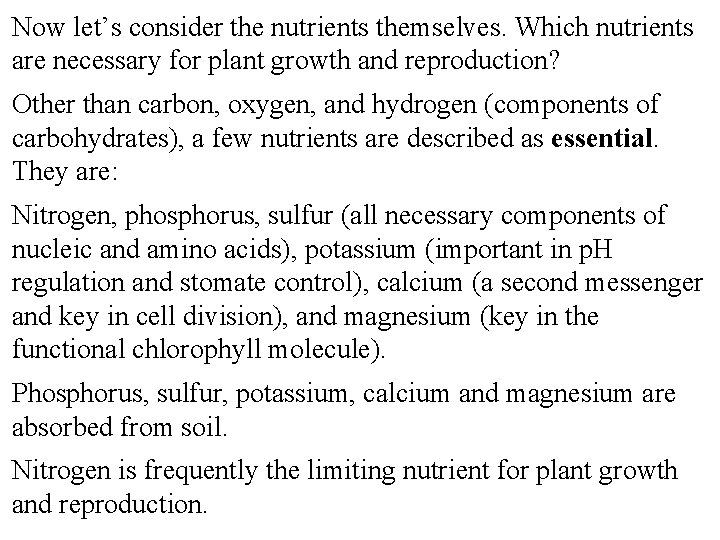
Now let’s consider the nutrients themselves. Which nutrients are necessary for plant growth and reproduction? Other than carbon, oxygen, and hydrogen (components of carbohydrates), a few nutrients are described as essential. They are: Nitrogen, phosphorus, sulfur (all necessary components of nucleic and amino acids), potassium (important in p. H regulation and stomate control), calcium (a second messenger and key in cell division), and magnesium (key in the functional chlorophyll molecule). Phosphorus, sulfur, potassium, calcium and magnesium are absorbed from soil. Nitrogen is frequently the limiting nutrient for plant growth and reproduction.
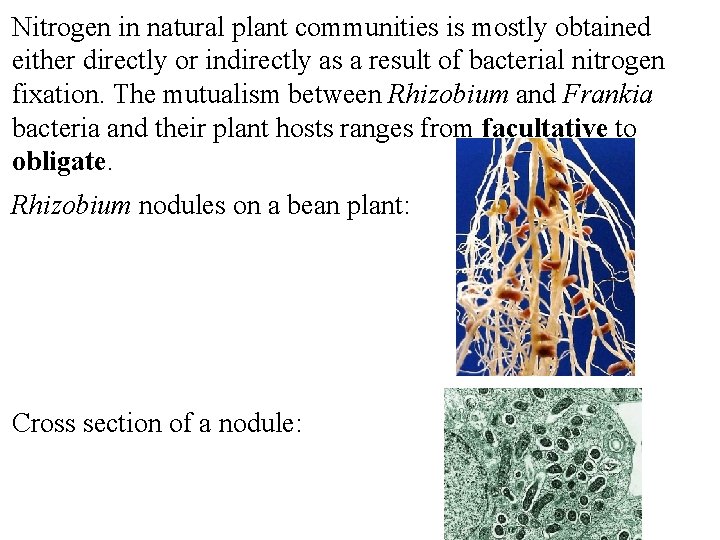
Nitrogen in natural plant communities is mostly obtained either directly or indirectly as a result of bacterial nitrogen fixation. The mutualism between Rhizobium and Frankia bacteria and their plant hosts ranges from facultative to obligate. Rhizobium nodules on a bean plant: Cross section of a nodule:
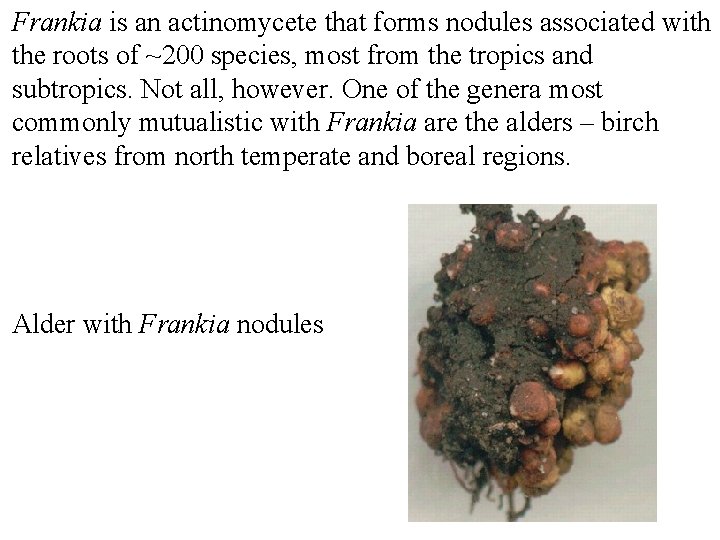
Frankia is an actinomycete that forms nodules associated with the roots of ~200 species, most from the tropics and subtropics. Not all, however. One of the genera most commonly mutualistic with Frankia are the alders – birch relatives from north temperate and boreal regions. Alder with Frankia nodules
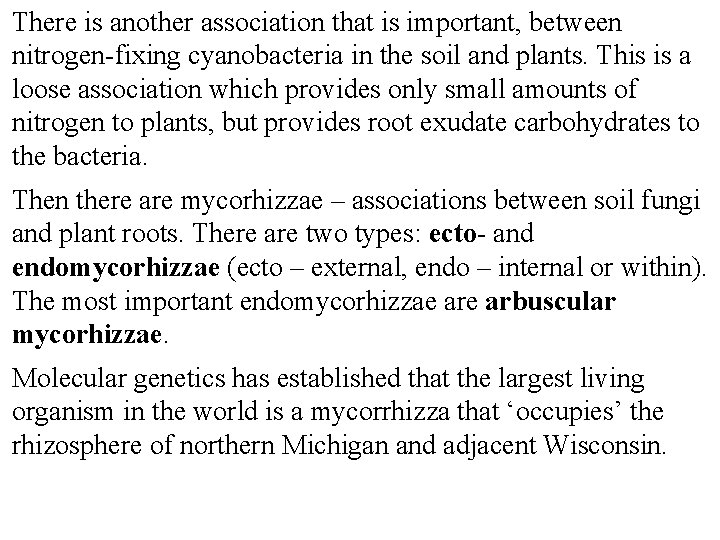
There is another association that is important, between nitrogen-fixing cyanobacteria in the soil and plants. This is a loose association which provides only small amounts of nitrogen to plants, but provides root exudate carbohydrates to the bacteria. Then there are mycorhizzae – associations between soil fungi and plant roots. There are two types: ecto- and endomycorhizzae (ecto – external, endo – internal or within). The most important endomycorhizzae arbuscular mycorhizzae. Molecular genetics has established that the largest living organism in the world is a mycorrhizza that ‘occupies’ the rhizosphere of northern Michigan and adjacent Wisconsin.
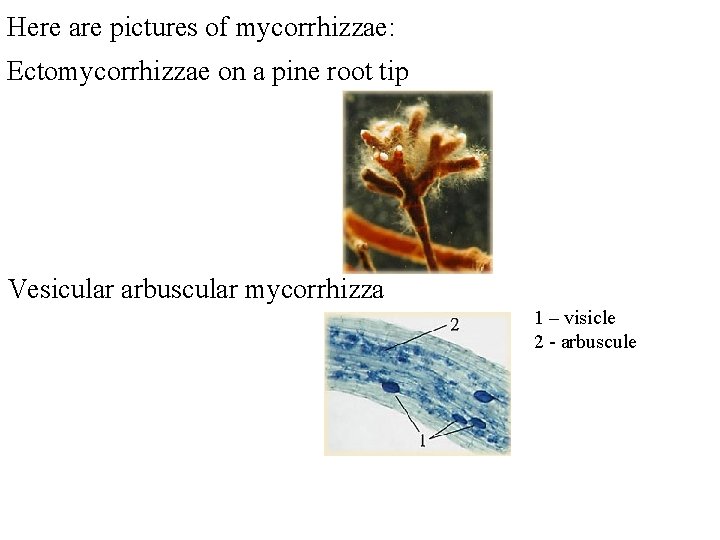
Here are pictures of mycorrhizzae: Ectomycorrhizzae on a pine root tip Vesicular arbuscular mycorrhizza 1 – visicle 2 - arbuscule
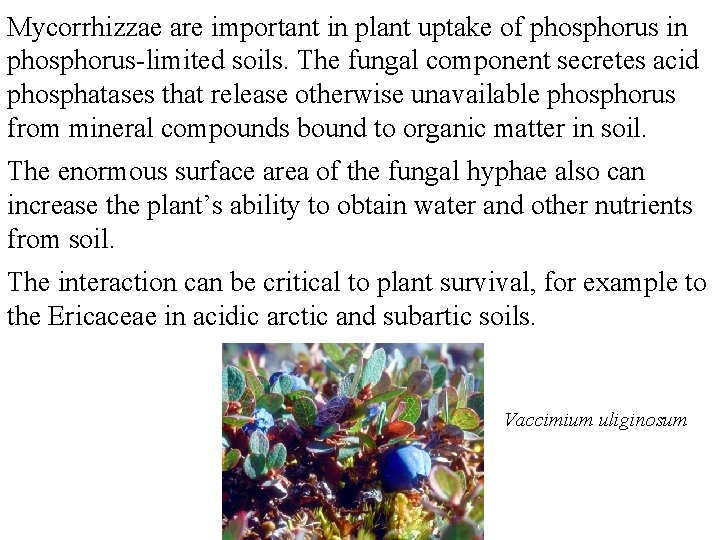
Mycorrhizzae are important in plant uptake of phosphorus in phosphorus-limited soils. The fungal component secretes acid phosphatases that release otherwise unavailable phosphorus from mineral compounds bound to organic matter in soil. The enormous surface area of the fungal hyphae also can increase the plant’s ability to obtain water and other nutrients from soil. The interaction can be critical to plant survival, for example to the Ericaceae in acidic arctic and subartic soils. Vaccimium uliginosum
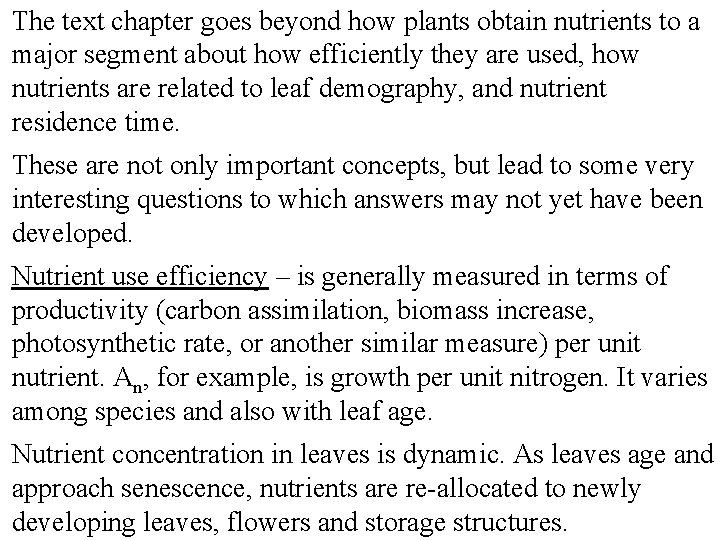
The text chapter goes beyond how plants obtain nutrients to a major segment about how efficiently they are used, how nutrients are related to leaf demography, and nutrient residence time. These are not only important concepts, but lead to some very interesting questions to which answers may not yet have been developed. Nutrient use efficiency – is generally measured in terms of productivity (carbon assimilation, biomass increase, photosynthetic rate, or another similar measure) per unit nutrient. An, for example, is growth per unit nitrogen. It varies among species and also with leaf age. Nutrient concentration in leaves is dynamic. As leaves age and approach senescence, nutrients are re-allocated to newly developing leaves, flowers and storage structures.
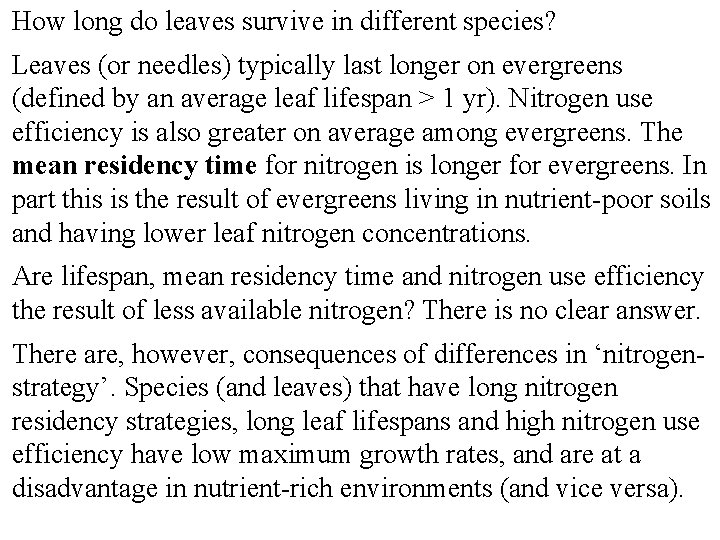
How long do leaves survive in different species? Leaves (or needles) typically last longer on evergreens (defined by an average leaf lifespan > 1 yr). Nitrogen use efficiency is also greater on average among evergreens. The mean residency time for nitrogen is longer for evergreens. In part this is the result of evergreens living in nutrient-poor soils and having lower leaf nitrogen concentrations. Are lifespan, mean residency time and nitrogen use efficiency the result of less available nitrogen? There is no clear answer. There are, however, consequences of differences in ‘nitrogenstrategy’. Species (and leaves) that have long nitrogen residency strategies, long leaf lifespans and high nitrogen use efficiency have low maximum growth rates, and are at a disadvantage in nutrient-rich environments (and vice versa).
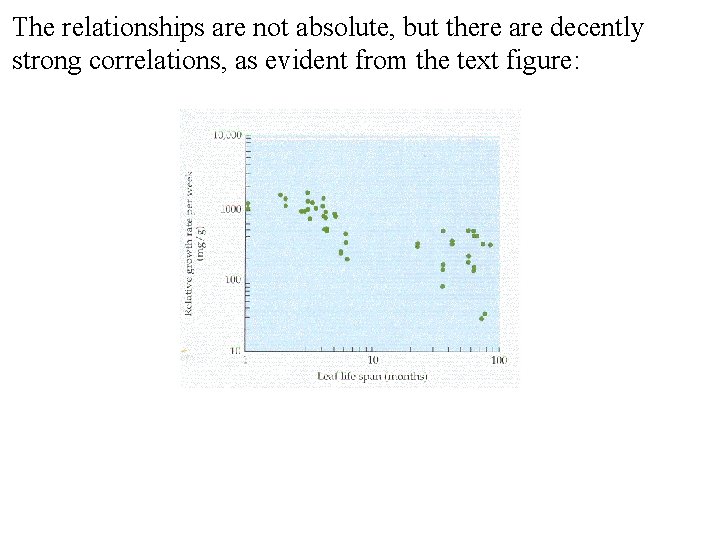
The relationships are not absolute, but there are decently strong correlations, as evident from the text figure:
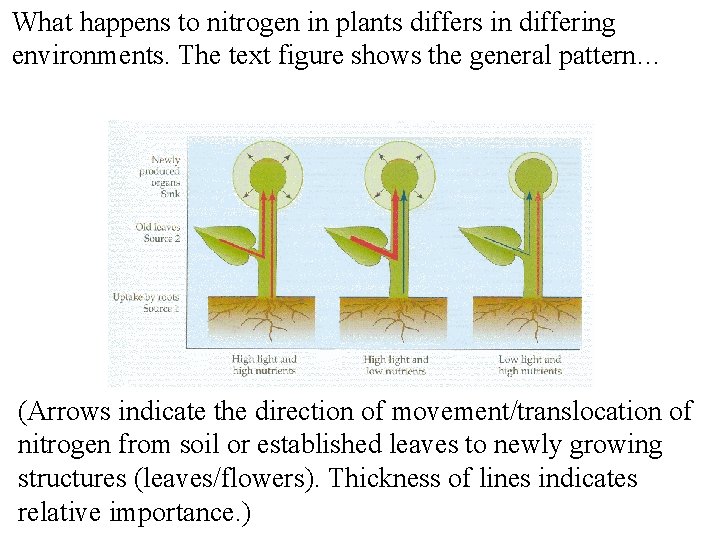
What happens to nitrogen in plants differs in differing environments. The text figure shows the general pattern… (Arrows indicate the direction of movement/translocation of nitrogen from soil or established leaves to newly growing structures (leaves/flowers). Thickness of lines indicates relative importance. )
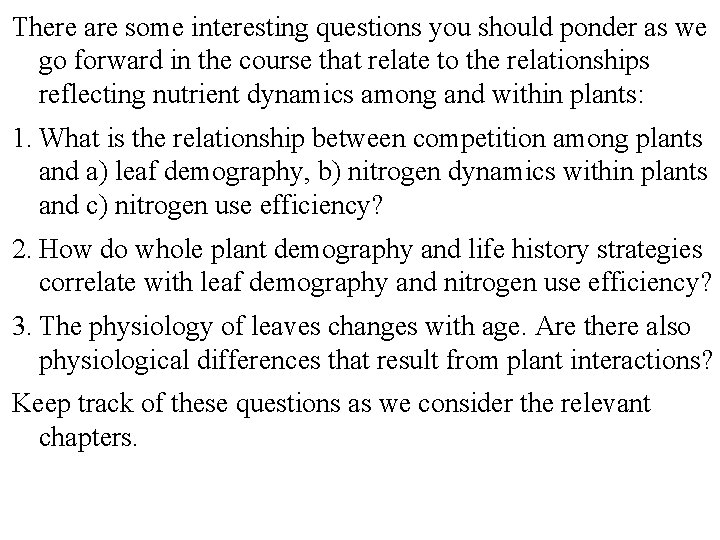
There are some interesting questions you should ponder as we go forward in the course that relate to the relationships reflecting nutrient dynamics among and within plants: 1. What is the relationship between competition among plants and a) leaf demography, b) nitrogen dynamics within plants and c) nitrogen use efficiency? 2. How do whole plant demography and life history strategies correlate with leaf demography and nitrogen use efficiency? 3. The physiology of leaves changes with age. Are there also physiological differences that result from plant interactions? Keep track of these questions as we consider the relevant chapters.
Employee relations in public relations
What non vascular plants
Non vascular plants
Characteristic of non flowering plants
C3 plants vs c4 plants
Living soil vs dead soil
Four major spheres of the earth
Male reproductive structure in plants
Structure of vascular plants
Shear strength of soil ppt
Angular blocky soil structure
Soil structure
Soil structures
Vertical response
What is a covalent bond simple definition
Union myunion structure my structure integer m
Giant molecular structure vs simple molecular structure
@prof._jane_blendes:https://semawur.com/xujhhf16p
Transformational grammar
Surface structure and deep structure
Zinc oxide + nitric acid → zinc nitrate + water
Static data structure and dynamic data structure
Rekaman data
Surface structure
Derivational relations stage
Visual spatial relations
La passe miroir mbti
Think public relations
Todays public relations departments