Simulating Anderson localization via a quantum walk on
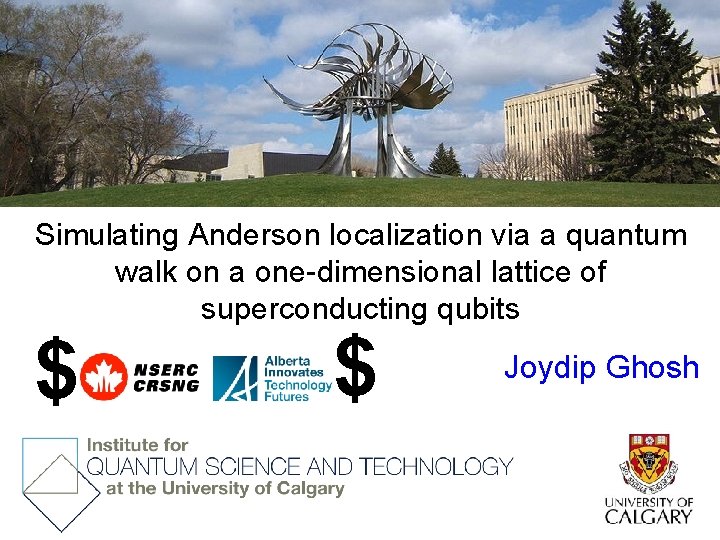
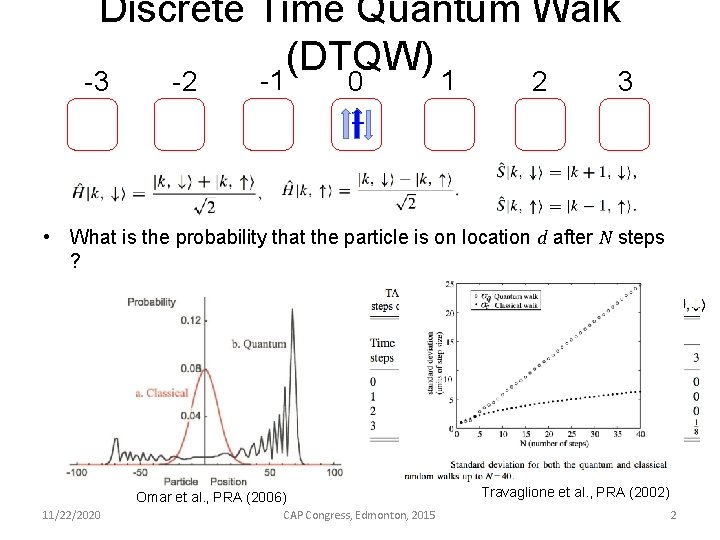
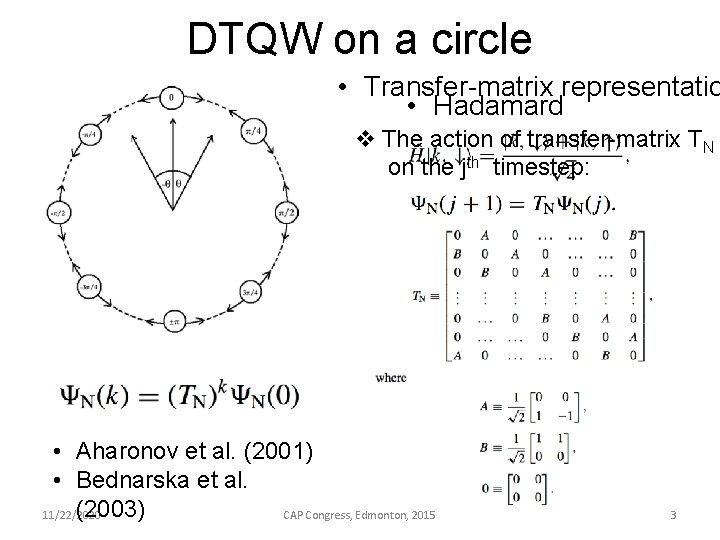
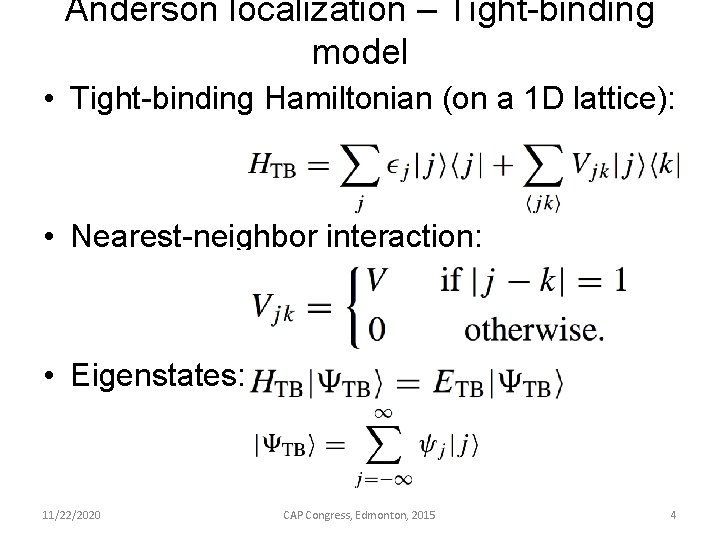
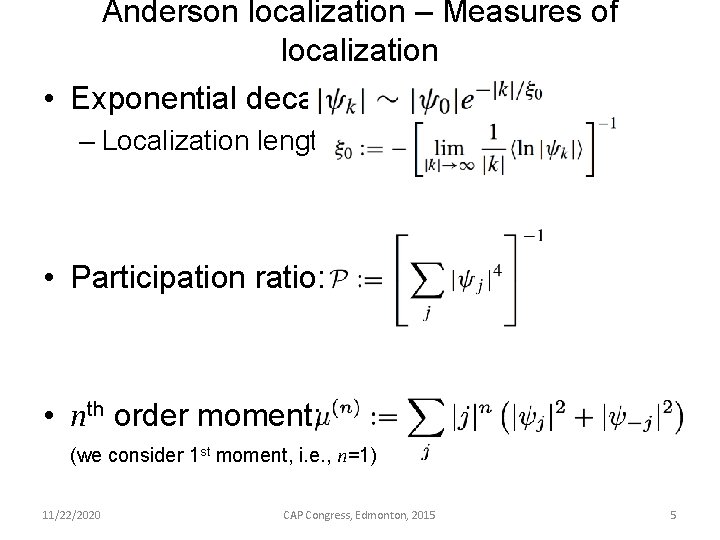
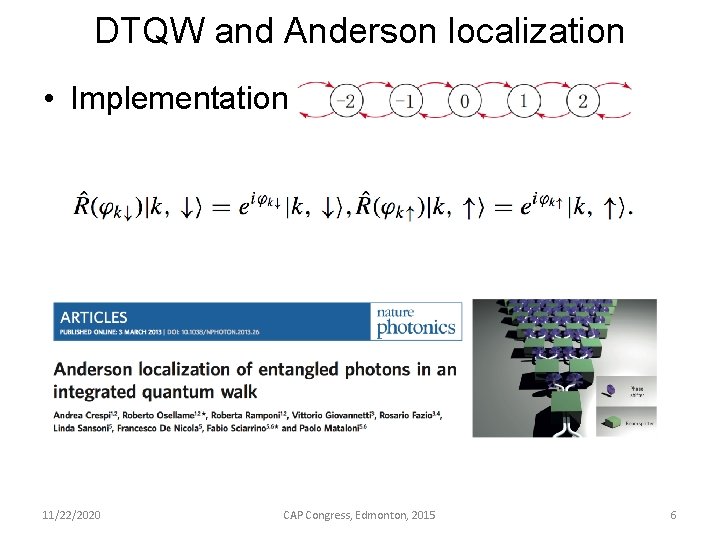
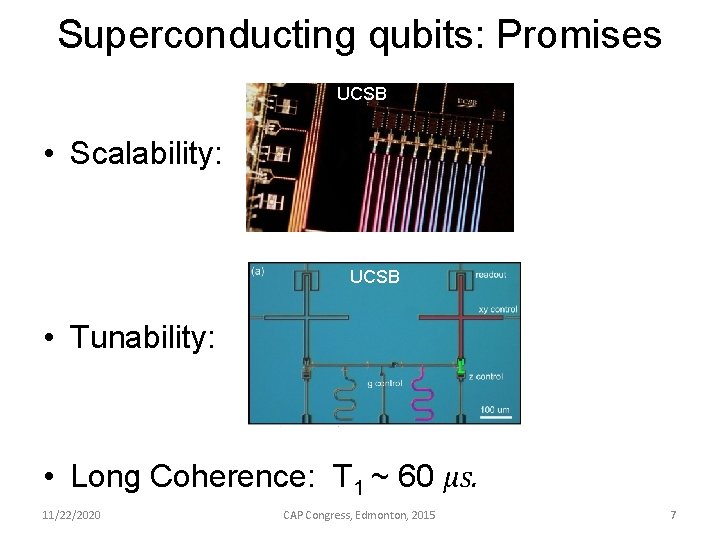
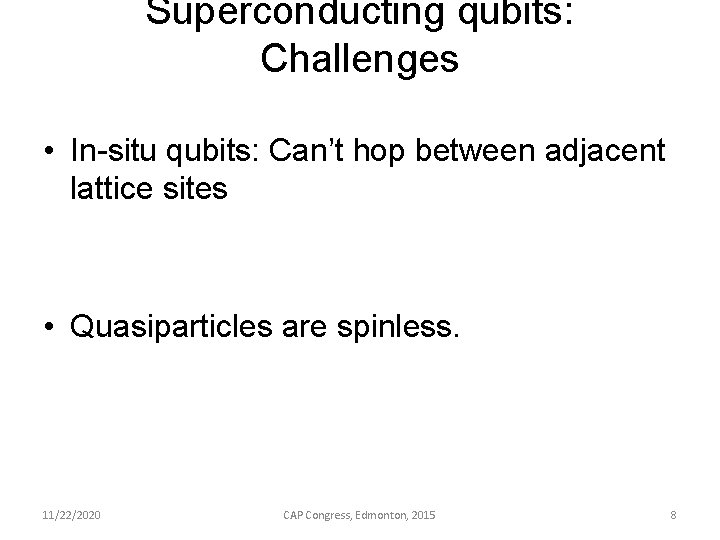
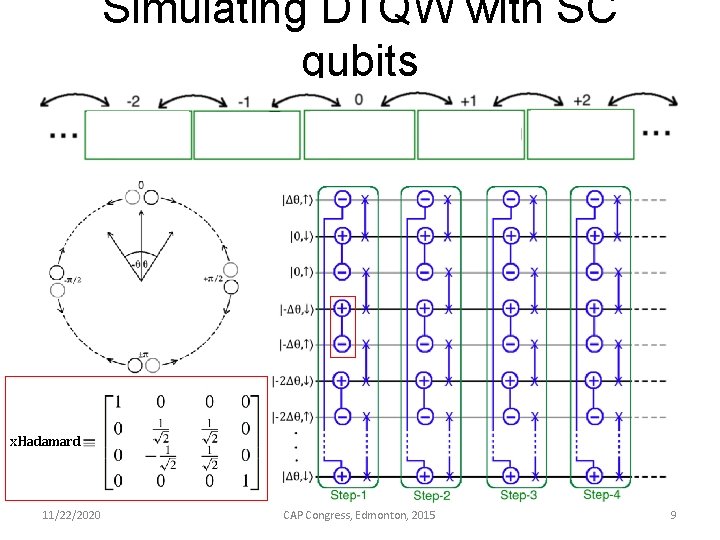
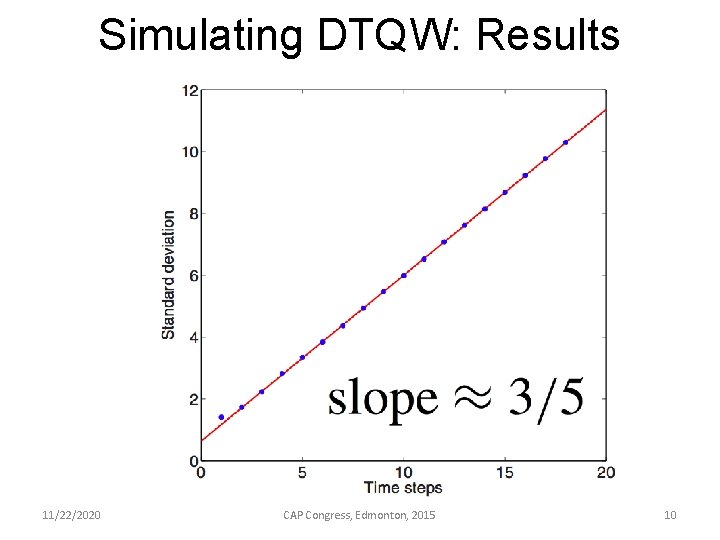
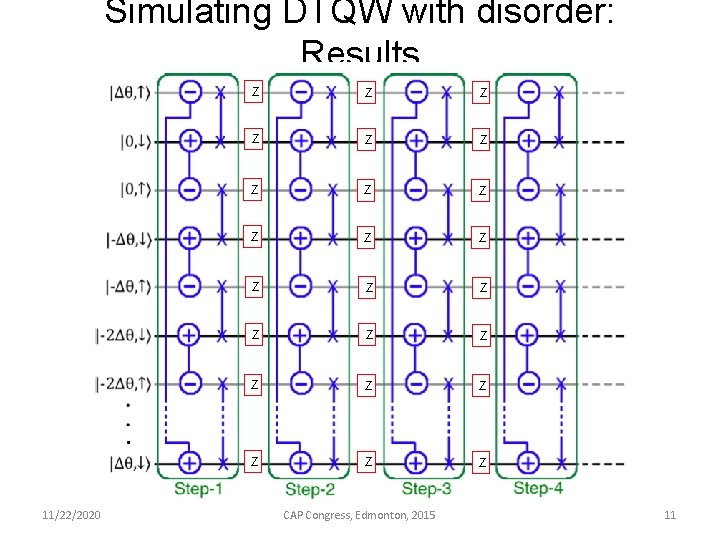
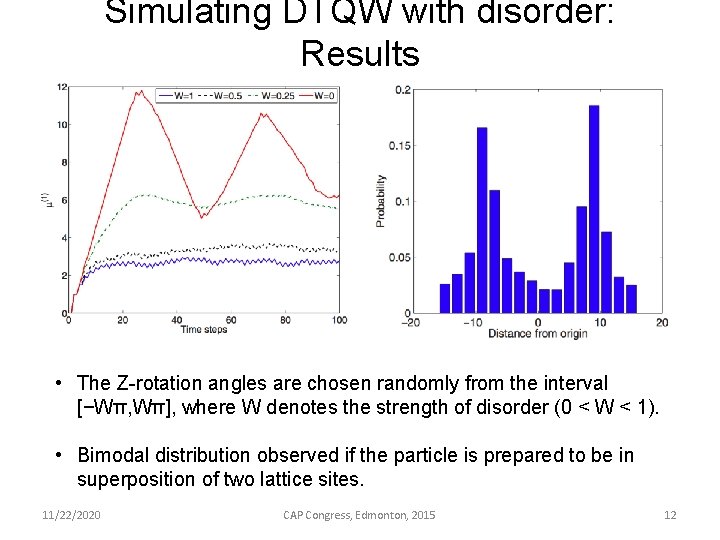
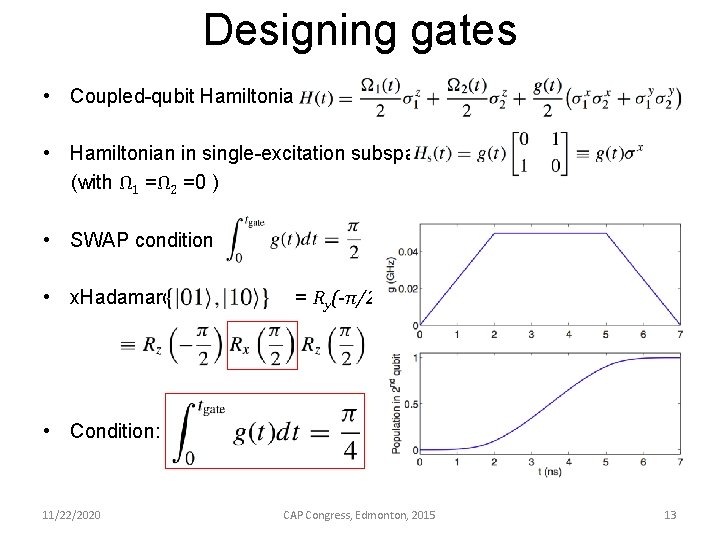
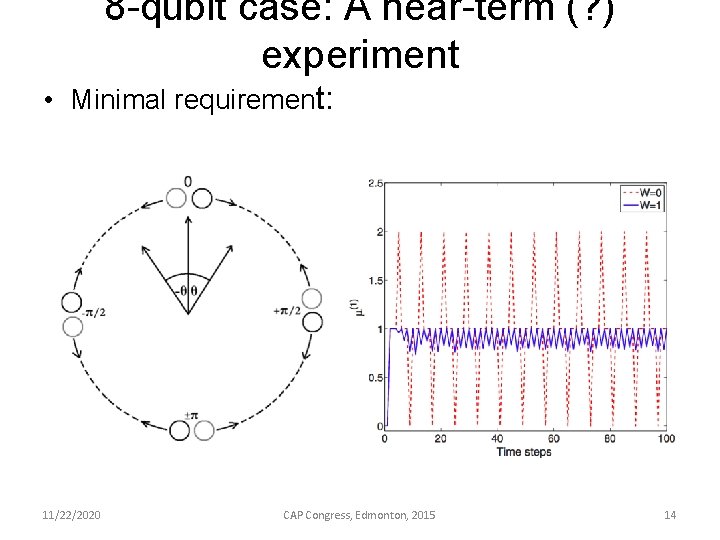
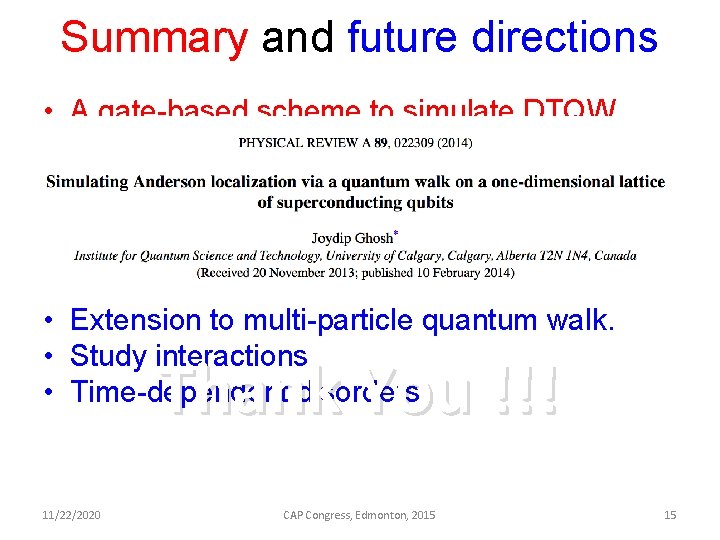
- Slides: 15
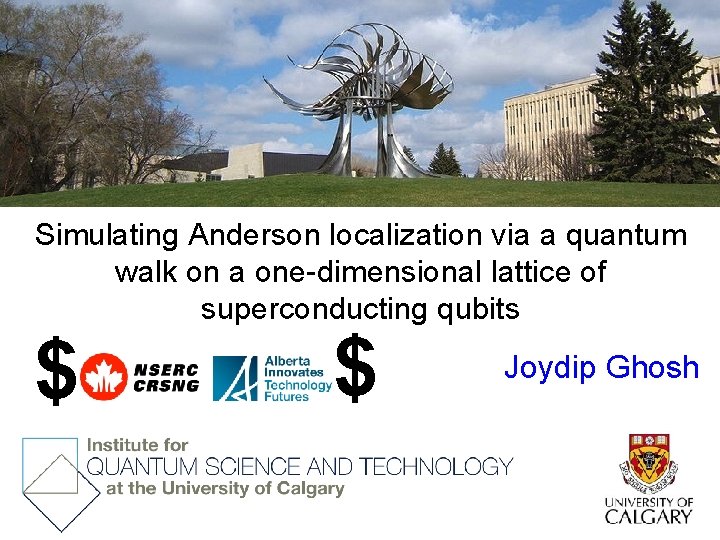
Simulating Anderson localization via a quantum walk on a one-dimensional lattice of superconducting qubits $ $ Joydip Ghosh
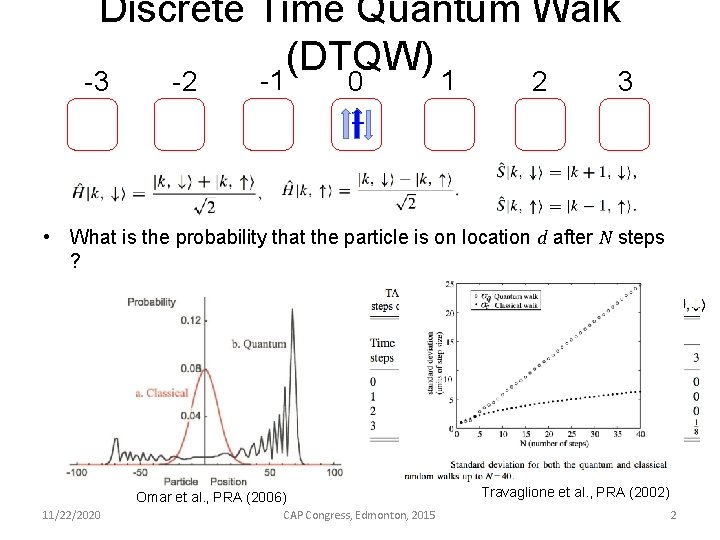
Discrete Time Quantum Walk (DTQW) -3 -2 -1 0 + 1 2 3 • What is the probability that the particle is on location d after N steps ? – No closed form solution Omar et al. , PRA (2006) 11/22/2020 CAP Congress, Edmonton, 2015 Travaglione et al. , PRA (2002) 2
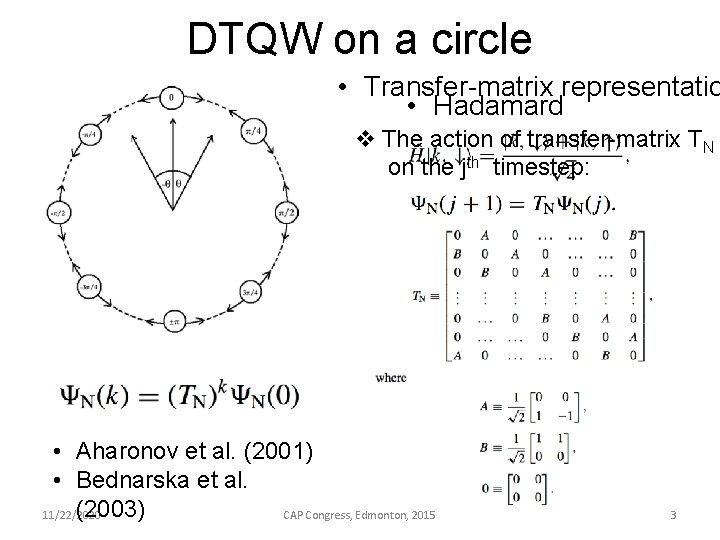
DTQW on a circle • Transfer-matrix representatio • Hadamard v The action of transfer-matrix TN on the jth timestep: • Conditional Shift • Aharonov et al. (2001) • Bednarska et al. (2003) 11/22/2020 CAP Congress, Edmonton, 2015 3
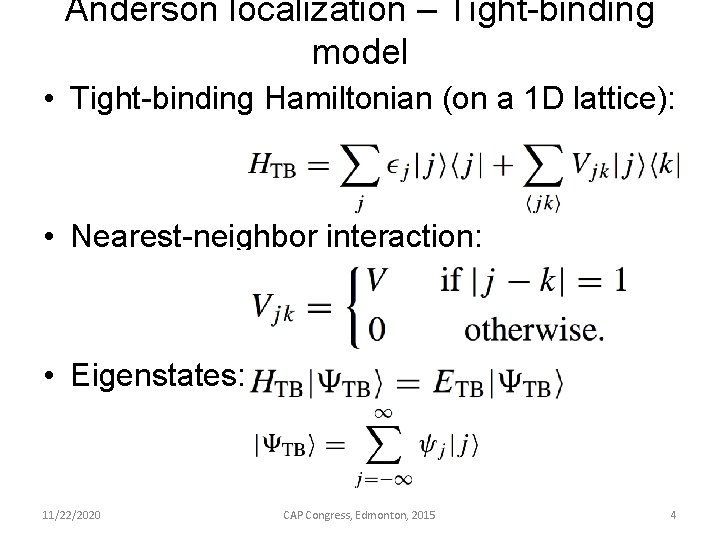
Anderson localization – Tight-binding model • Tight-binding Hamiltonian (on a 1 D lattice): • Nearest-neighbor interaction: • Eigenstates: 11/22/2020 CAP Congress, Edmonton, 2015 4
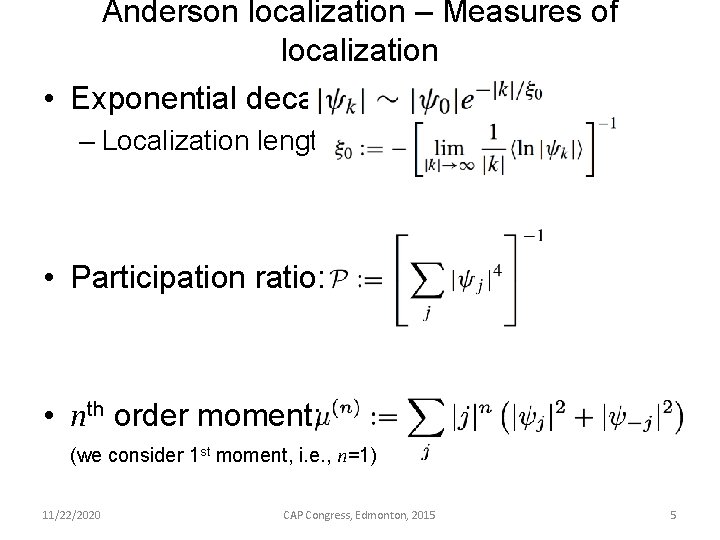
Anderson localization – Measures of localization • Exponential decay: – Localization length: • Participation ratio: • nth order moment: (we consider 1 st moment, i. e. , n=1) 11/22/2020 CAP Congress, Edmonton, 2015 5
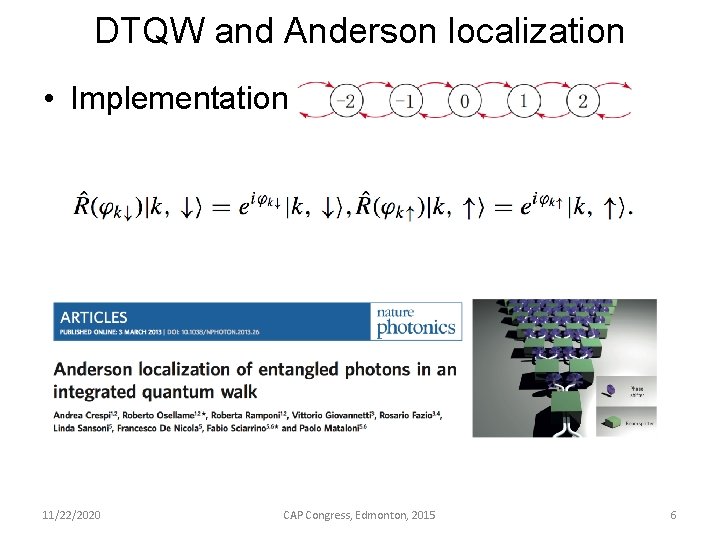
DTQW and Anderson localization • Implementation: 11/22/2020 CAP Congress, Edmonton, 2015 6
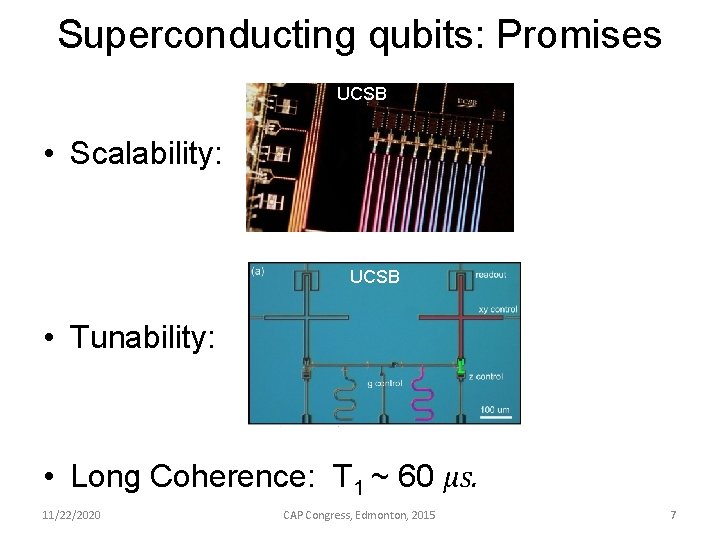
Superconducting qubits: Promises UCSB • Scalability: UCSB • Tunability: • Long Coherence: T 1 ~ 60 μs. 11/22/2020 CAP Congress, Edmonton, 2015 7
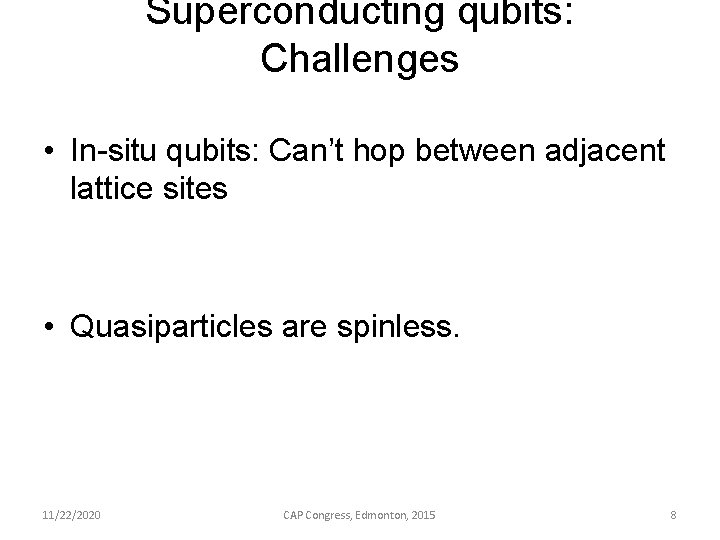
Superconducting qubits: Challenges • In-situ qubits: Can’t hop between adjacent lattice sites • Quasiparticles are spinless. 11/22/2020 CAP Congress, Edmonton, 2015 8
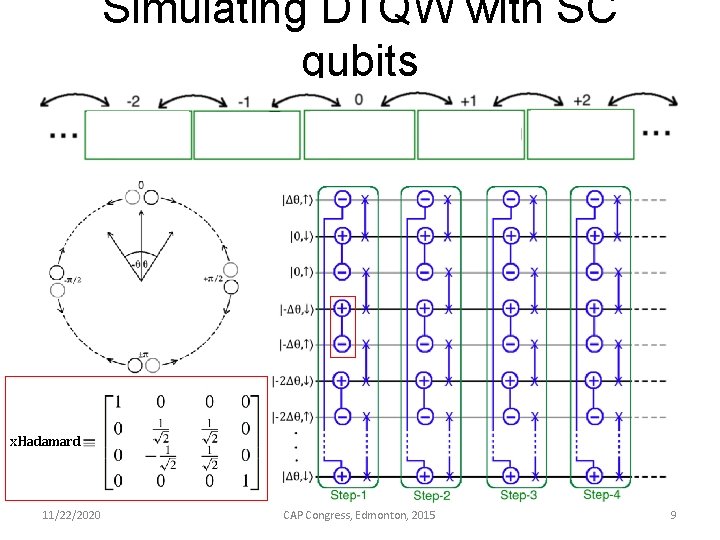
Simulating DTQW with SC qubits x. Hadamard 11/22/2020 CAP Congress, Edmonton, 2015 9
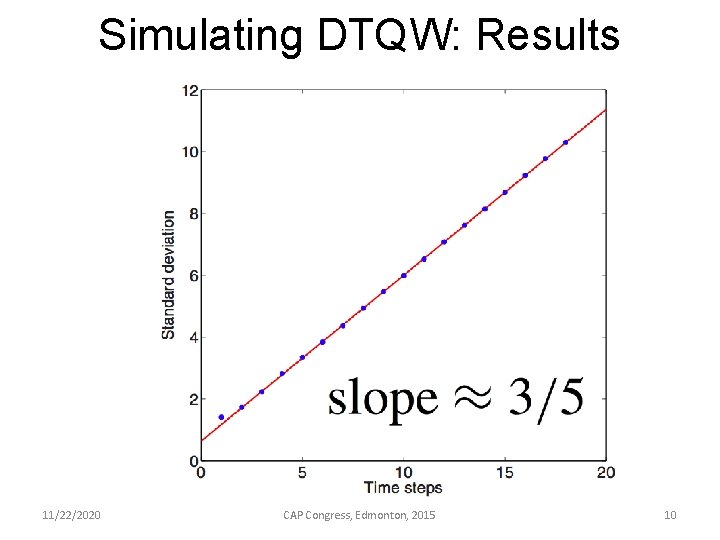
Simulating DTQW: Results 11/22/2020 CAP Congress, Edmonton, 2015 10
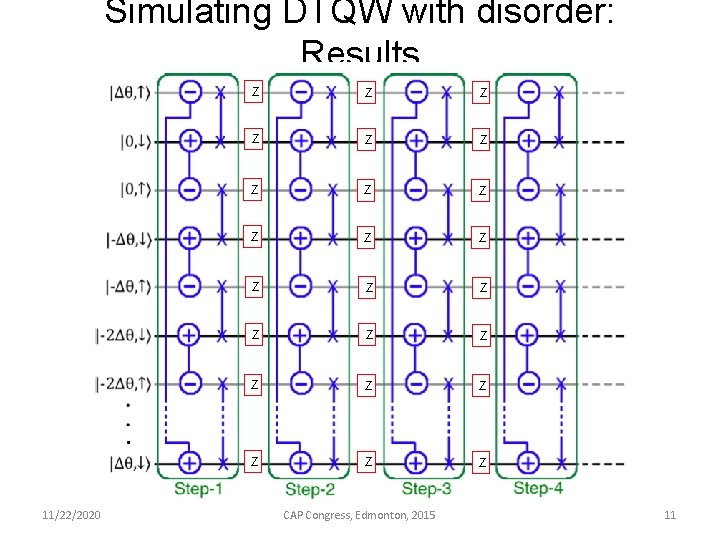
Simulating DTQW with disorder: Results 11/22/2020 Z Z Z Z Z Z CAP Congress, Edmonton, 2015 11
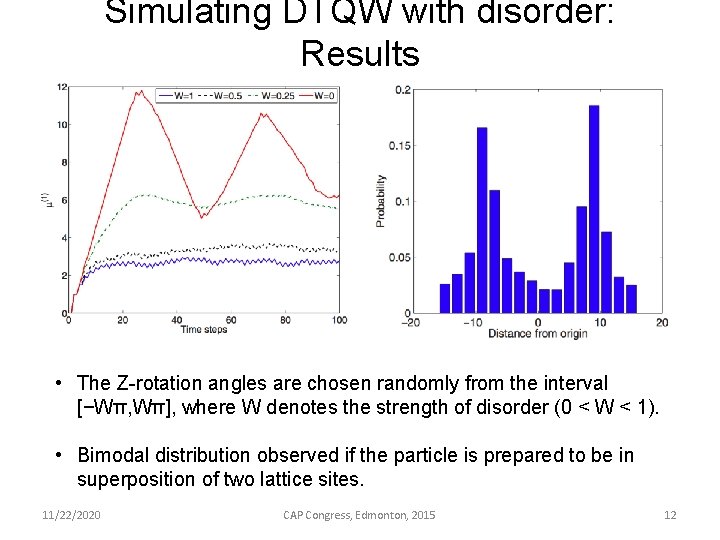
Simulating DTQW with disorder: Results • The Z-rotation angles are chosen randomly from the interval [−Wπ, Wπ], where W denotes the strength of disorder (0 < W < 1). • Bimodal distribution observed if the particle is prepared to be in superposition of two lattice sites. 11/22/2020 CAP Congress, Edmonton, 2015 12
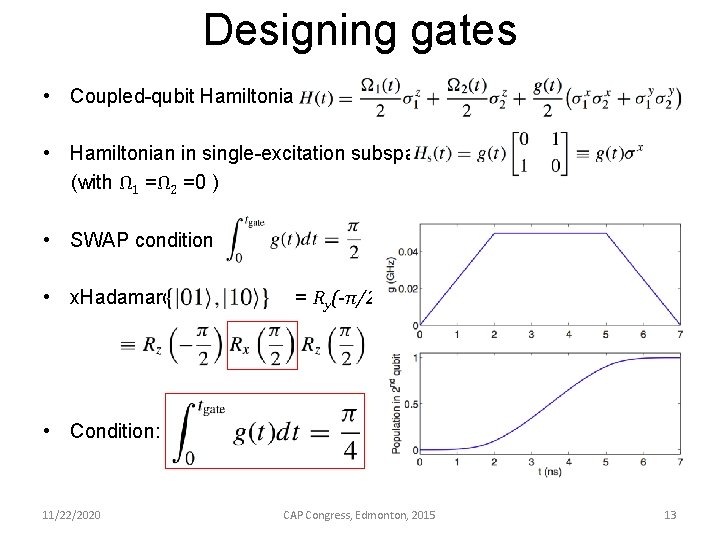
Designing gates • Coupled-qubit Hamiltonian: • Hamiltonian in single-excitation subspace (with Ω 1 =Ω 2 =0 ) • SWAP condition: • x. Hadamard = Ry(-π/2) • Condition: 11/22/2020 CAP Congress, Edmonton, 2015 13
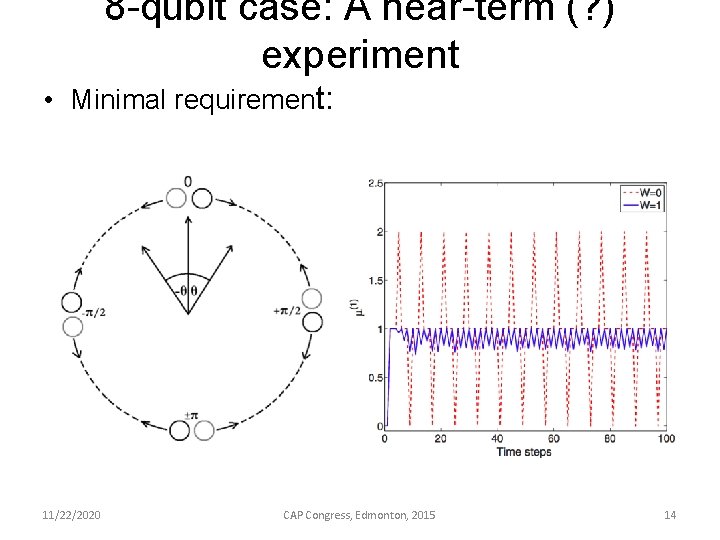
8 -qubit case: A near-term (? ) experiment • Minimal requirement: 11/22/2020 CAP Congress, Edmonton, 2015 14
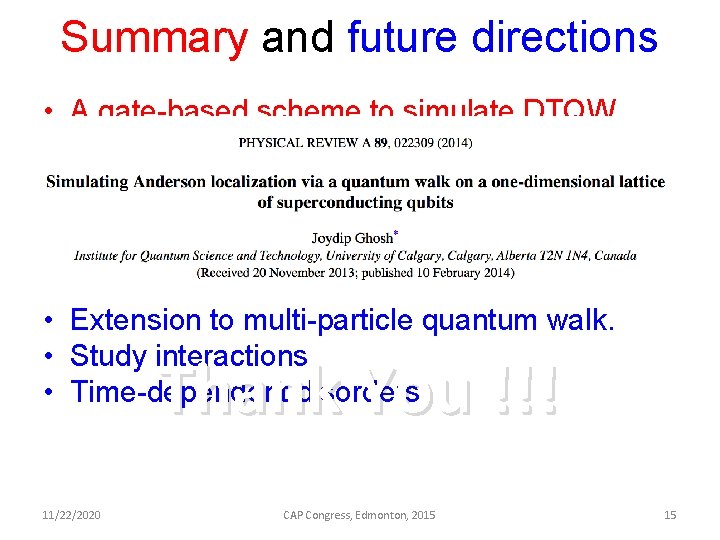
Summary and future directions • A gate-based scheme to simulate DTQW. • Disorders can be introduced and controlled. • Anderson localization observed with SC qubits. • Extension to multi-particle quantum walk. • Study interactions. • Time-dependent disorders. Thank You !!! 11/22/2020 CAP Congress, Edmonton, 2015 15
Anderson localization lecture notes
Cadaveric spasm and rigor mortis difference
Postmortem signs
Quantum walk
Quantum walk
Umesh vazirani
Walk the line
Quantum physics vs quantum mechanics
Quantum physics vs quantum mechanics
Via luces
Rodete piramidal
Decimoquinta estacion del via crucis
Via erudita e via popular
Via positiva and via negativa
Bayes filter
Tactile localization meaning