Radiation from Poynting Jets and Collisionless Shocks Edison
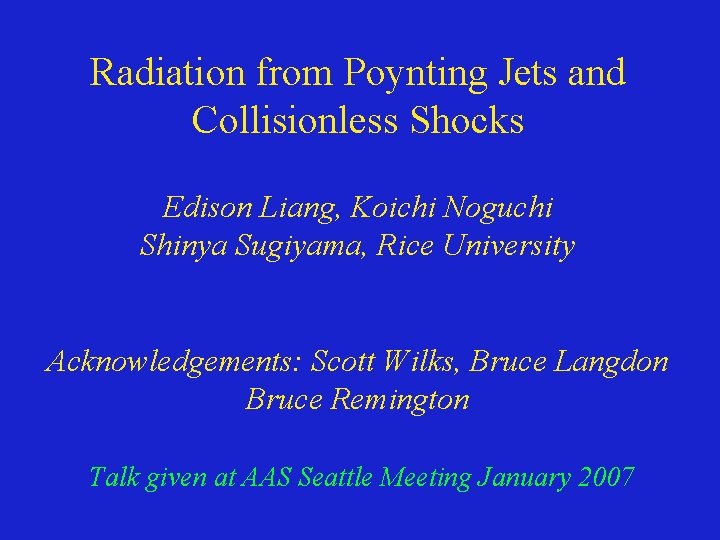
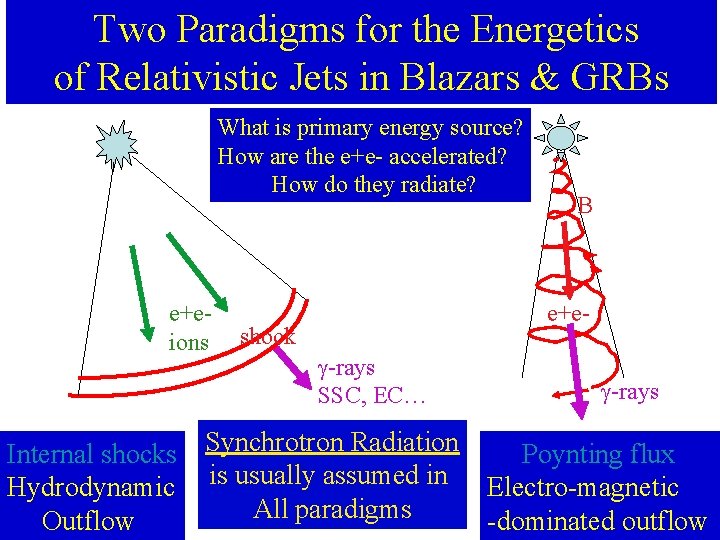
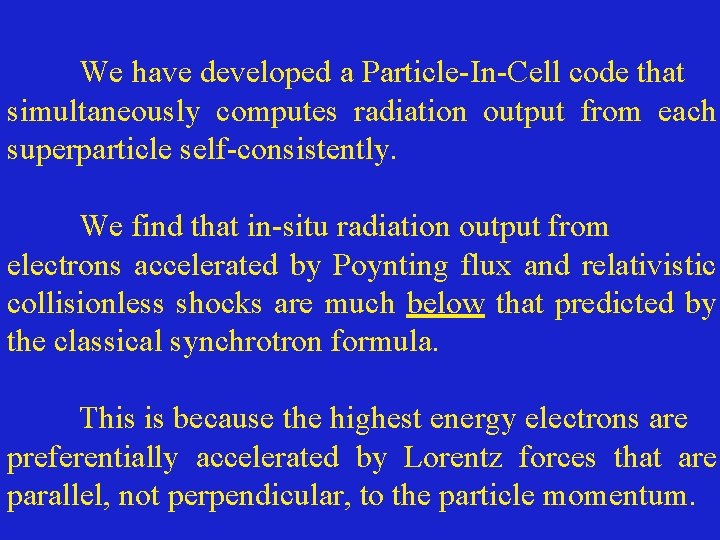
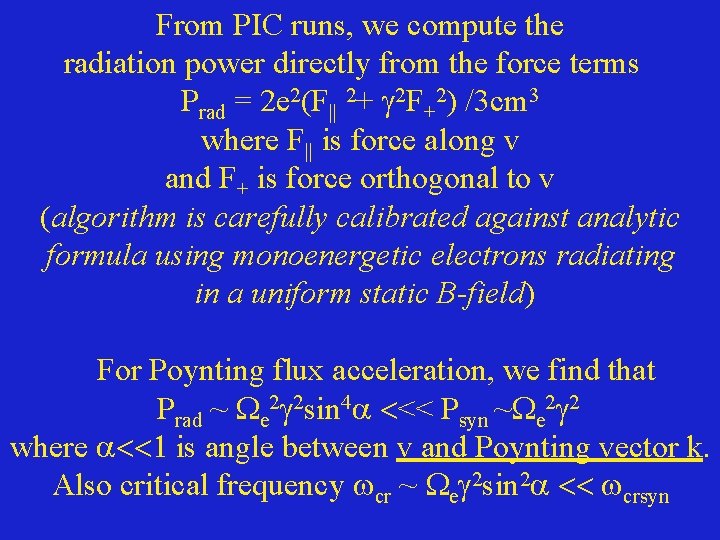
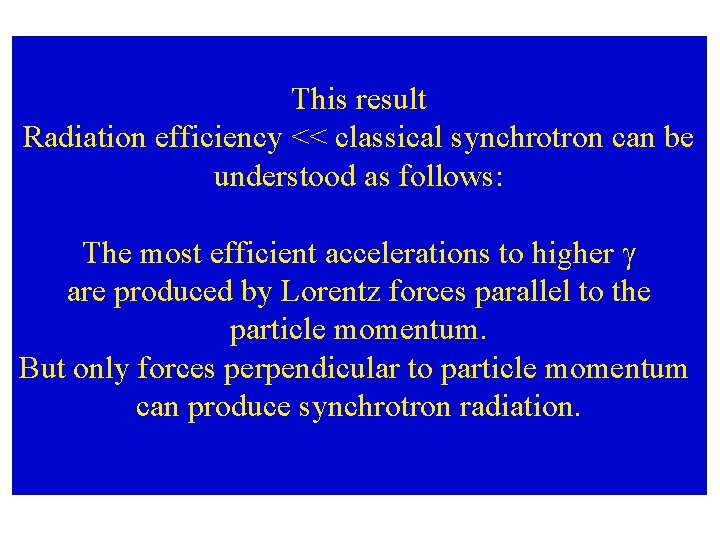
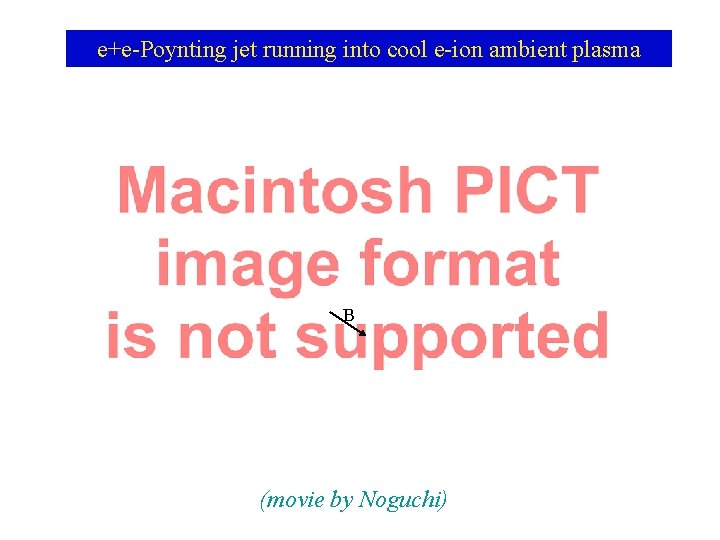
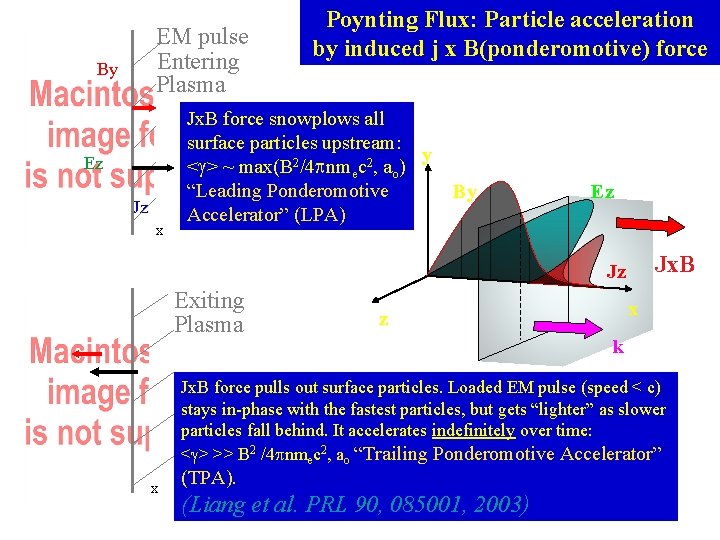
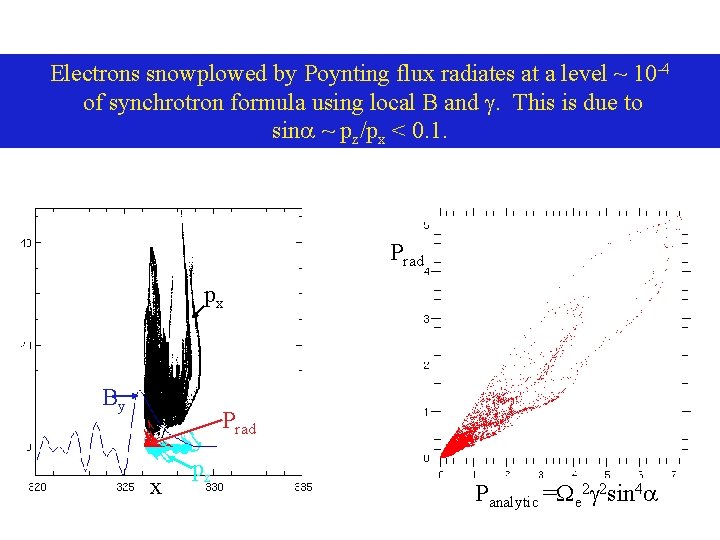
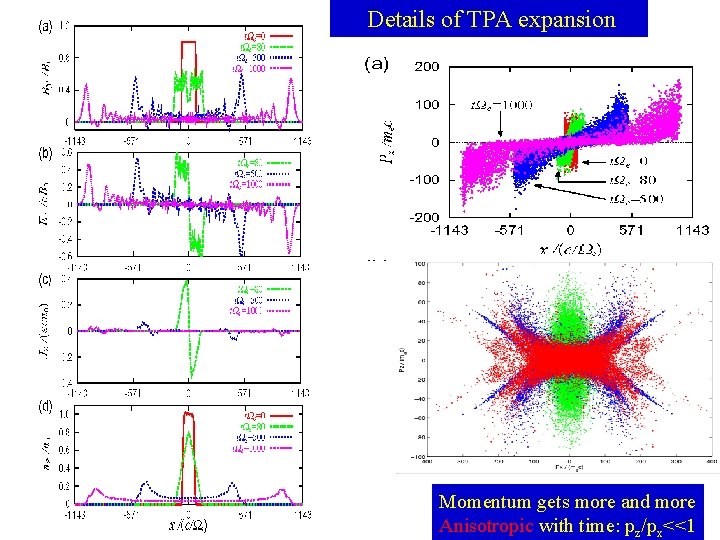
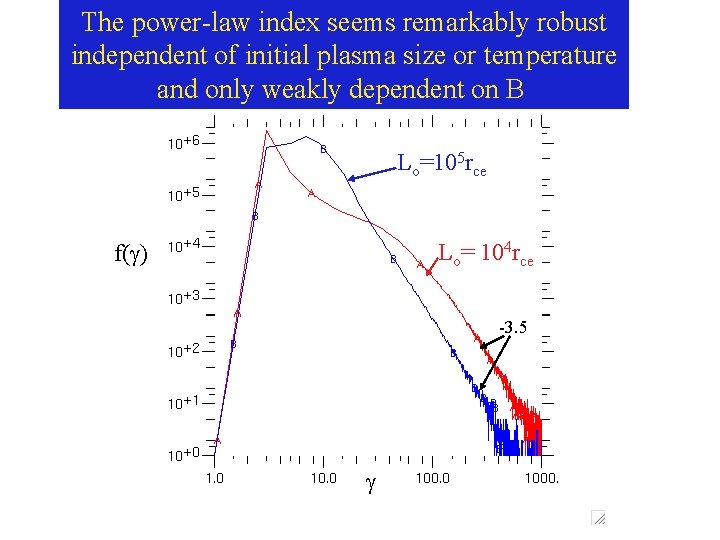
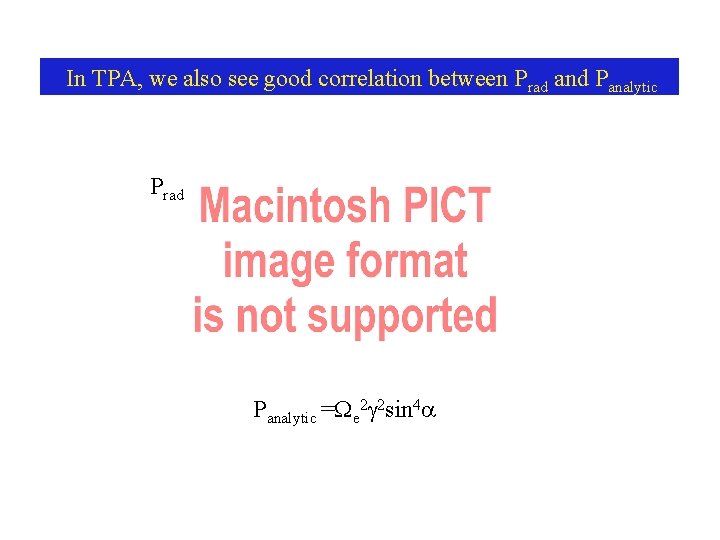
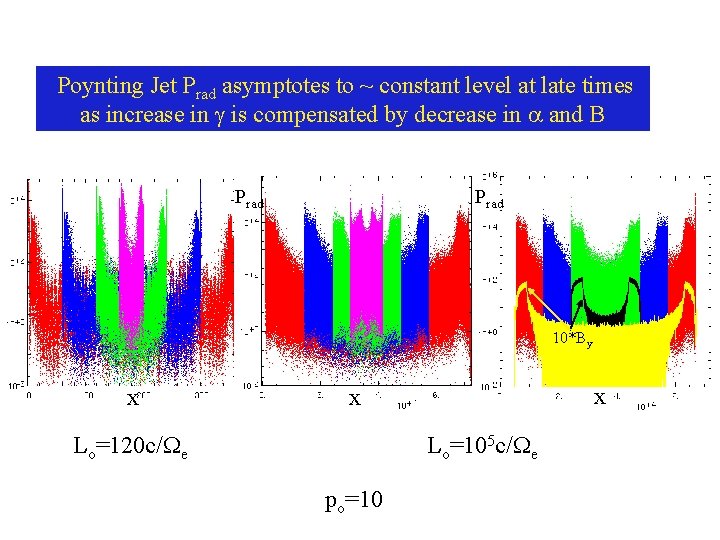
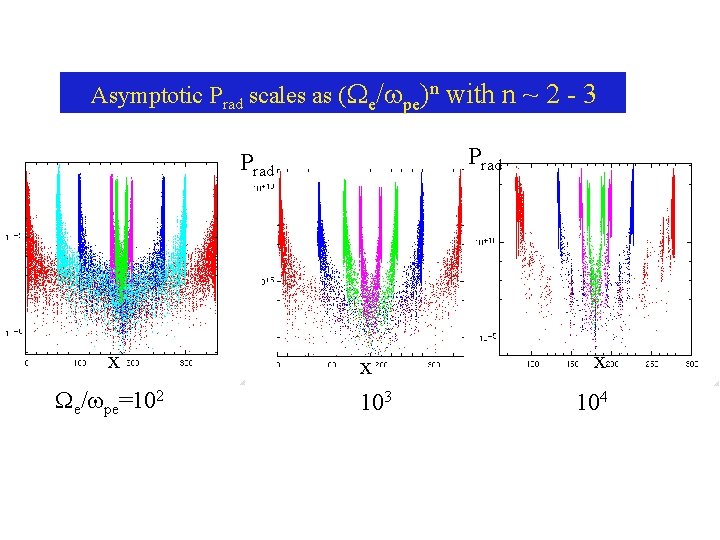
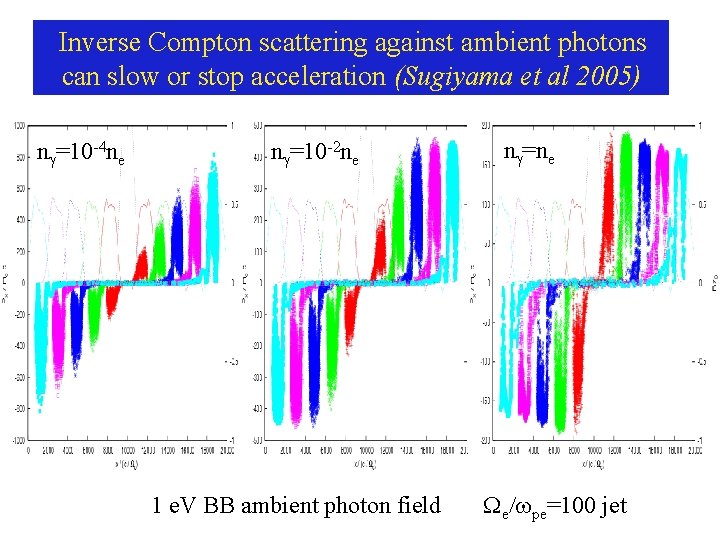
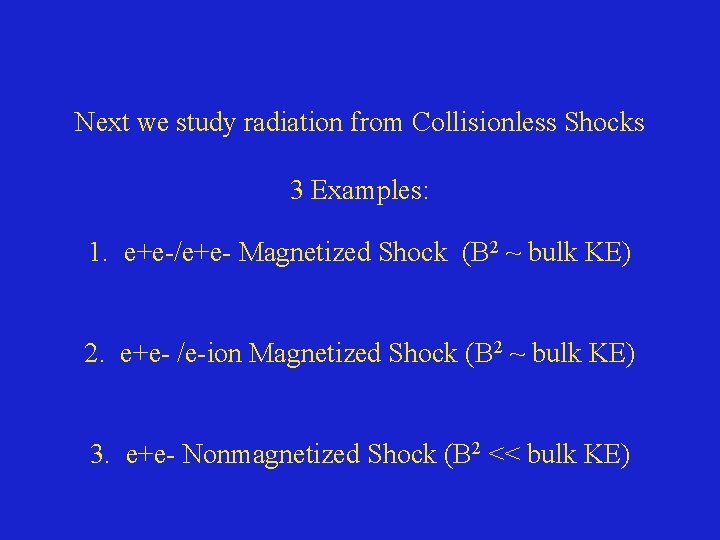
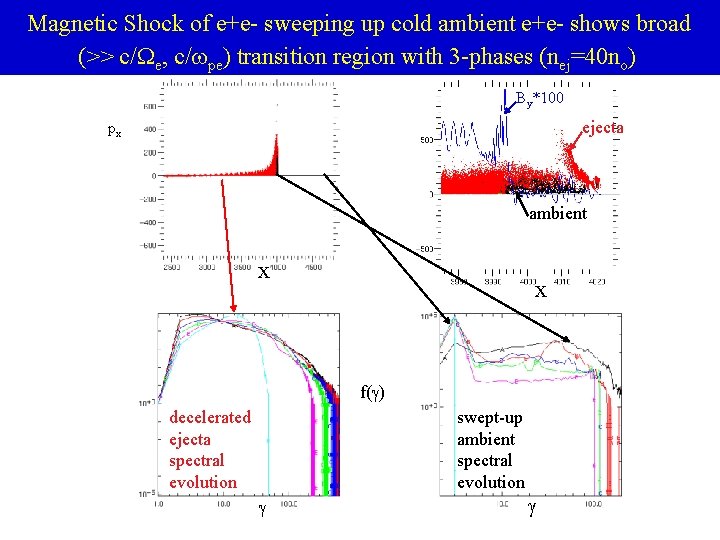
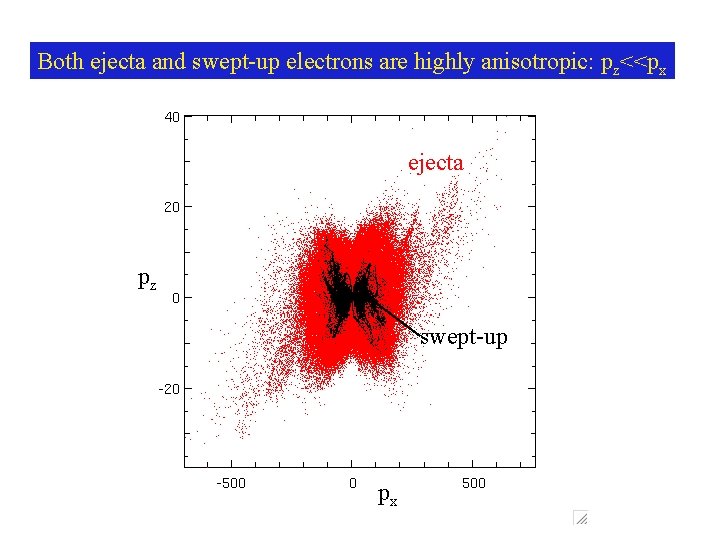
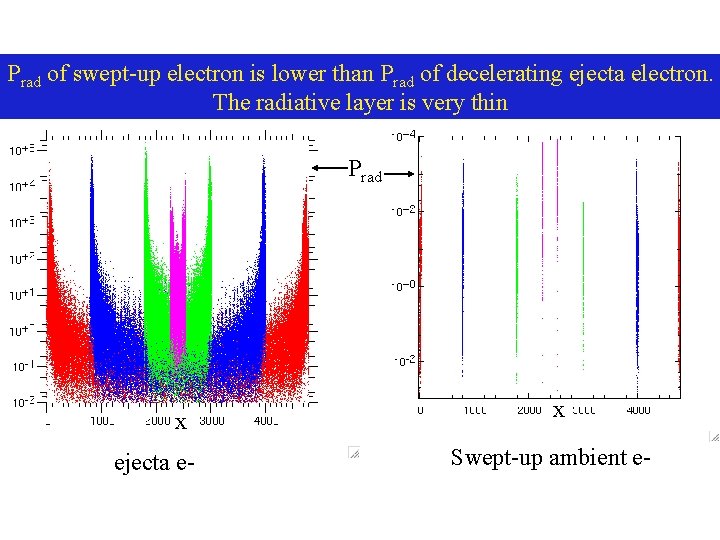
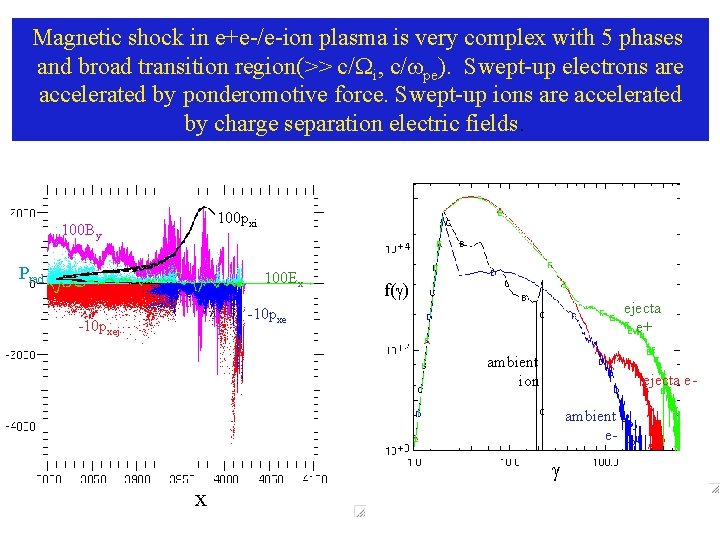
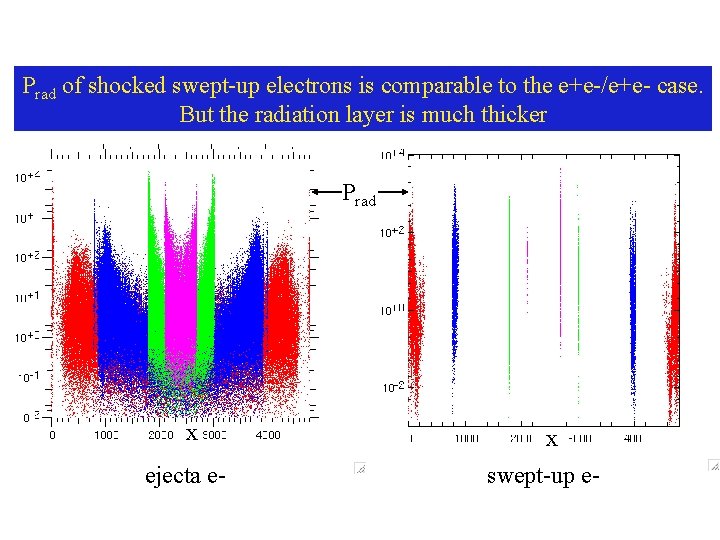
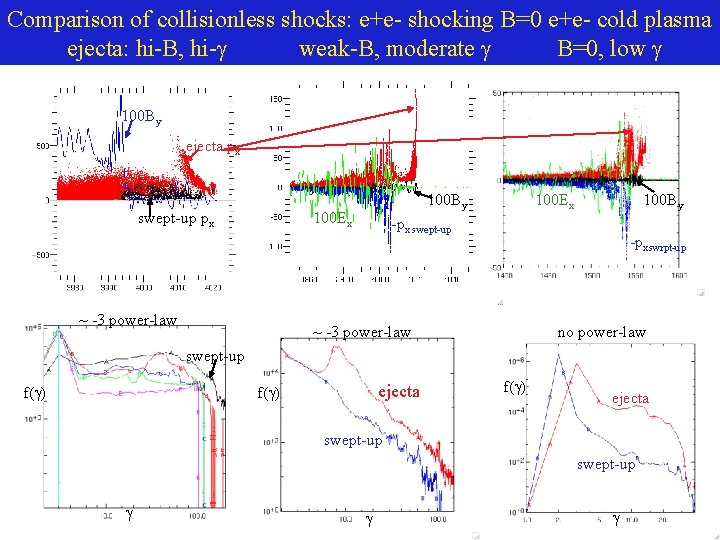
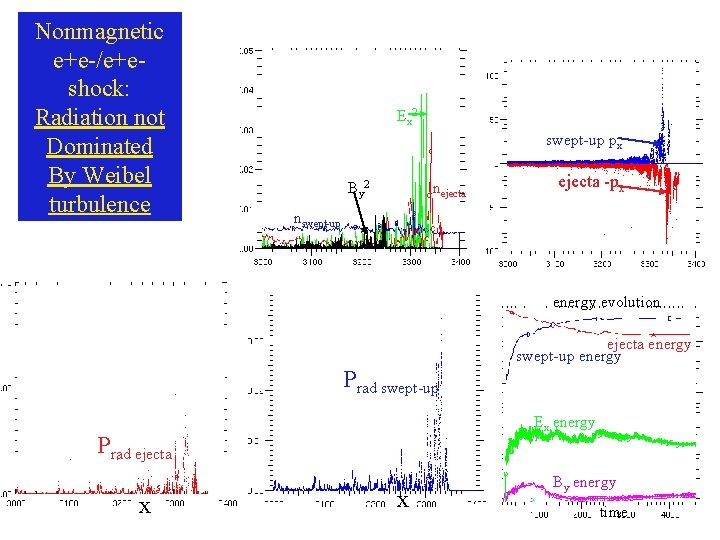
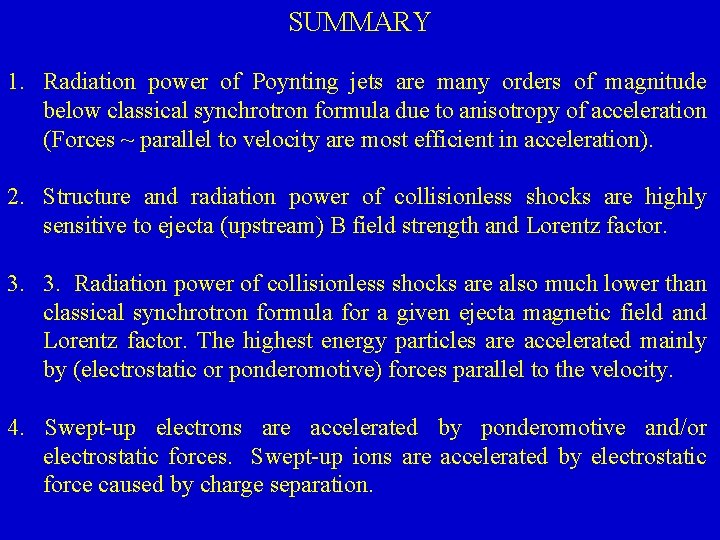
- Slides: 23
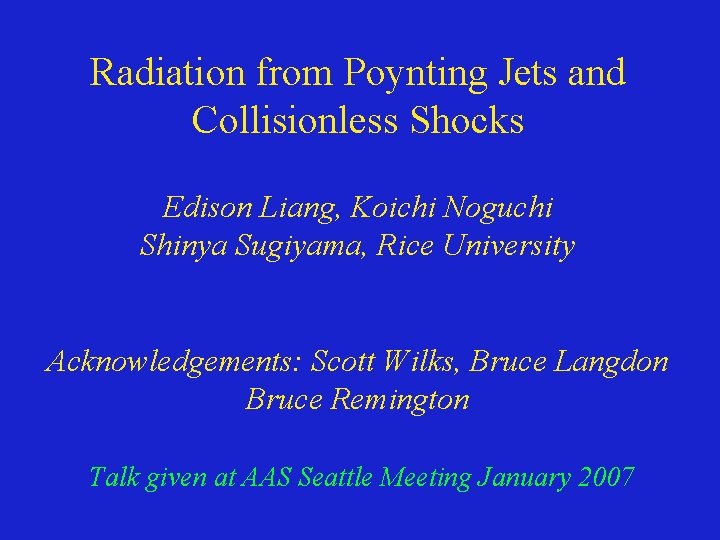
Radiation from Poynting Jets and Collisionless Shocks Edison Liang, Koichi Noguchi Shinya Sugiyama, Rice University Acknowledgements: Scott Wilks, Bruce Langdon Bruce Remington Talk given at AAS Seattle Meeting January 2007
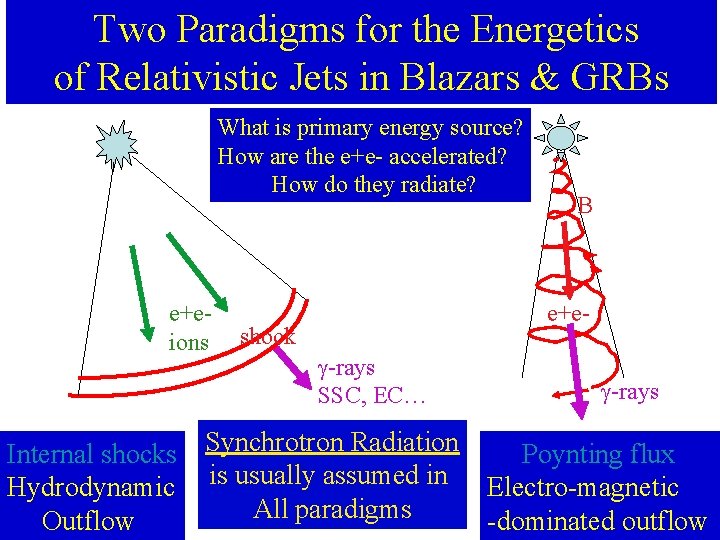
Two Paradigms for the Energetics of Relativistic Jets in Blazars & GRBs What is primary energy source? How are the e+e- accelerated? How do they radiate? e+eions B e+e- shock -rays SSC, EC… -rays Internal shocks Synchrotron Radiation Poynting flux Hydrodynamic is usually assumed in Electro-magnetic All paradigms Outflow -dominated outflow
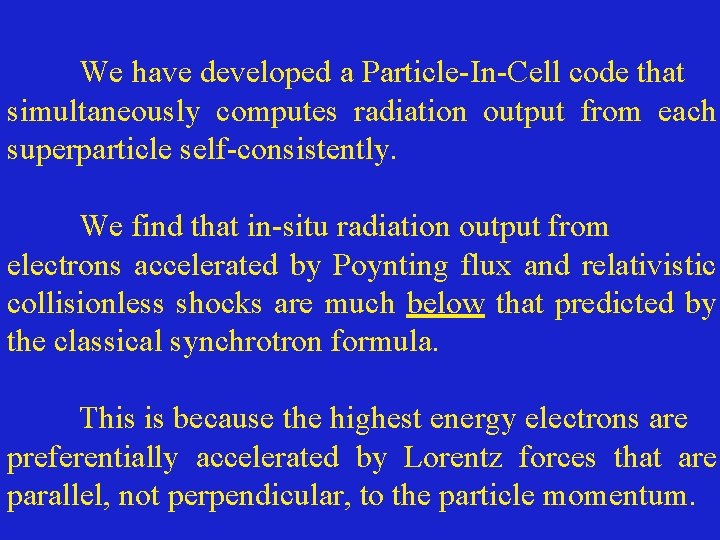
We have developed a Particle-In-Cell code that simultaneously computes radiation output from each superparticle self-consistently. We find that in-situ radiation output from electrons accelerated by Poynting flux and relativistic collisionless shocks are much below that predicted by the classical synchrotron formula. This is because the highest energy electrons are preferentially accelerated by Lorentz forces that are parallel, not perpendicular, to the particle momentum.
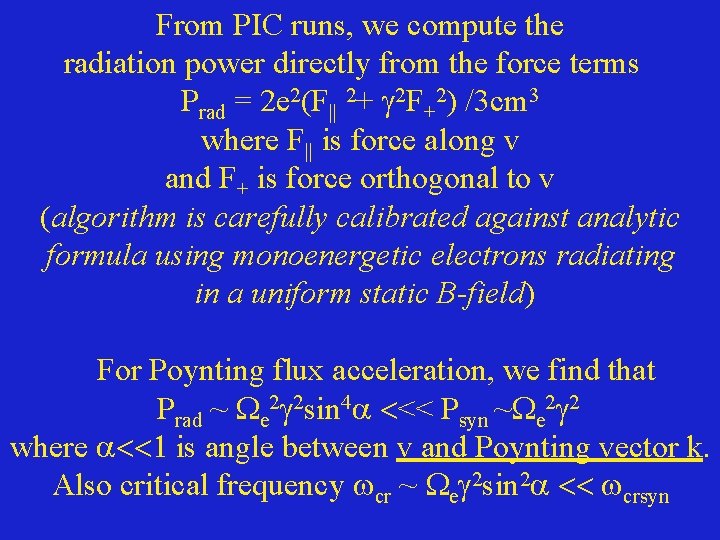
From PIC runs, we compute the radiation power directly from the force terms Prad = 2 e 2(F|| 2+ 2 F+2) /3 cm 3 where F|| is force along v and F+ is force orthogonal to v (algorithm is carefully calibrated against analytic formula using monoenergetic electrons radiating in a uniform static B-field) For Poynting flux acceleration, we find that Prad ~ We 2 2 sin 4 a <<< Psyn ~We 2 2 where a<<1 is angle between v and Poynting vector k. Also critical frequency wcr ~ We 2 sin 2 a << wcrsyn
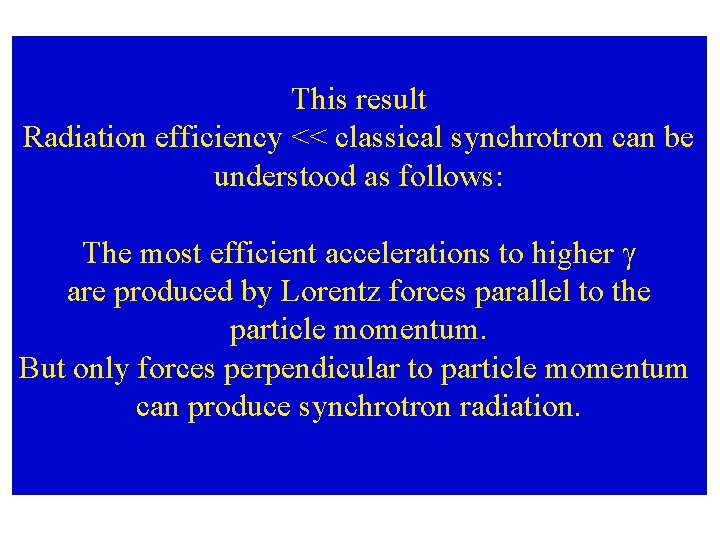
This result Radiation efficiency << classical synchrotron can be understood as follows: The most efficient accelerations to higher are produced by Lorentz forces parallel to the particle momentum. But only forces perpendicular to particle momentum can produce synchrotron radiation.
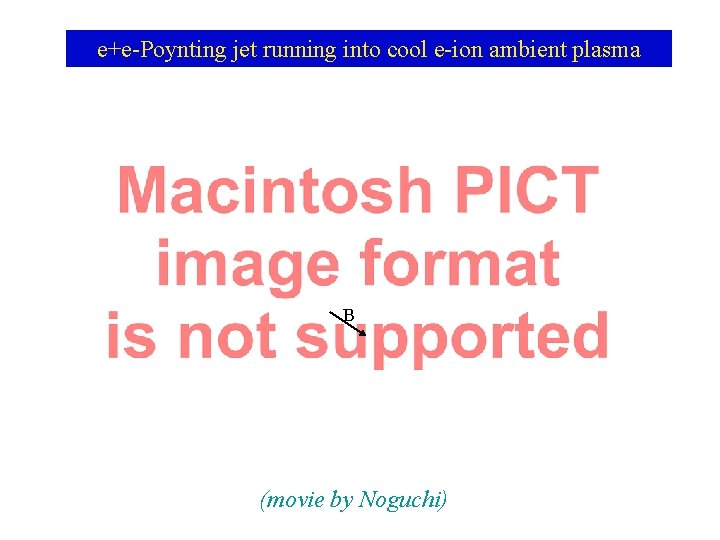
e+e-Poynting jet running into cool e-ion ambient plasma B (movie by Noguchi)
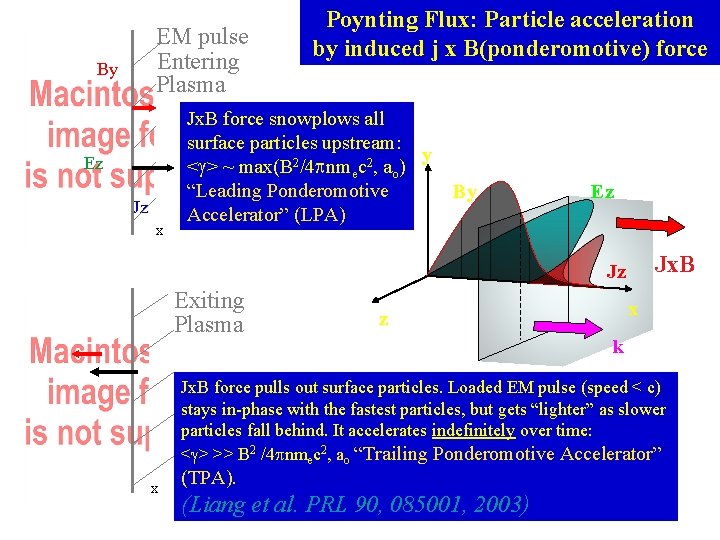
EM pulse Entering Plasma By Ez Jz x Poynting Flux: Particle acceleration by induced j x B(ponderomotive) force Jx. B force snowplows all surface particles upstream: y < > ~ max(B 2/4 pnmec 2, ao) “Leading Ponderomotive Accelerator” (LPA) By Ez Jx. B Jz Exiting Plasma x x z k Jx. B force pulls out surface particles. Loaded EM pulse (speed < c) stays in-phase with the fastest particles, but gets “lighter” as slower particles fall behind. It accelerates indefinitely over time: < > >> B 2 /4 pnmec 2, ao “Trailing Ponderomotive Accelerator” (TPA). (Liang et al. PRL 90, 085001, 2003)
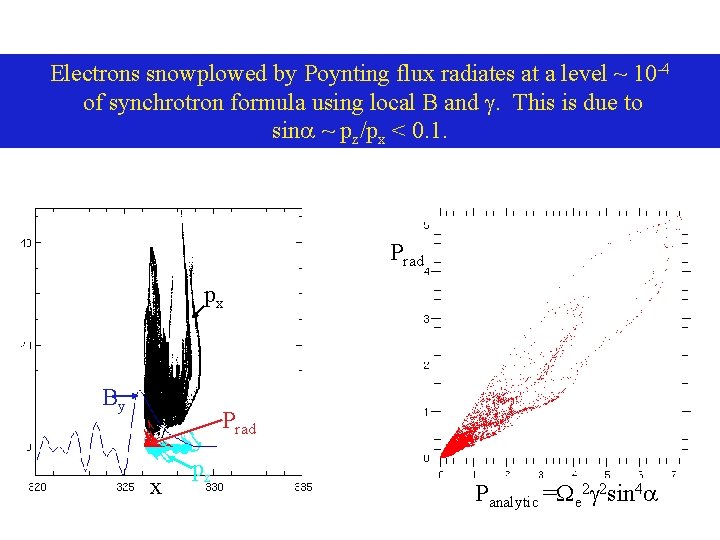
Electrons snowplowed by Poynting flux radiates at a level ~ 10 -4 of synchrotron formula using local B and . This is due to sina ~ pz/px < 0. 1. Prad px By Prad x pz Panalytic =We 2 2 sin 4 a
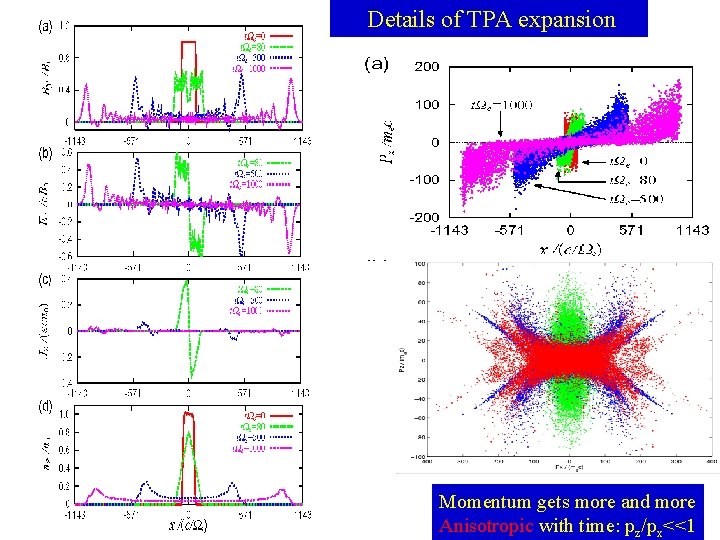
Details of TPA expansion Momentum gets more and more Anisotropic with time: pz/px<<1
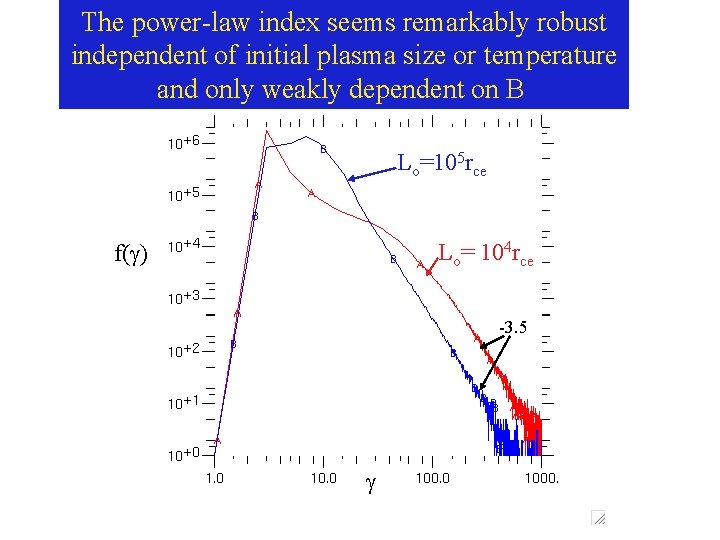
The power-law index seems remarkably robust independent of initial plasma size or temperature and only weakly dependent on B Lo=105 rce Lo= 104 rce f( ) -3. 5
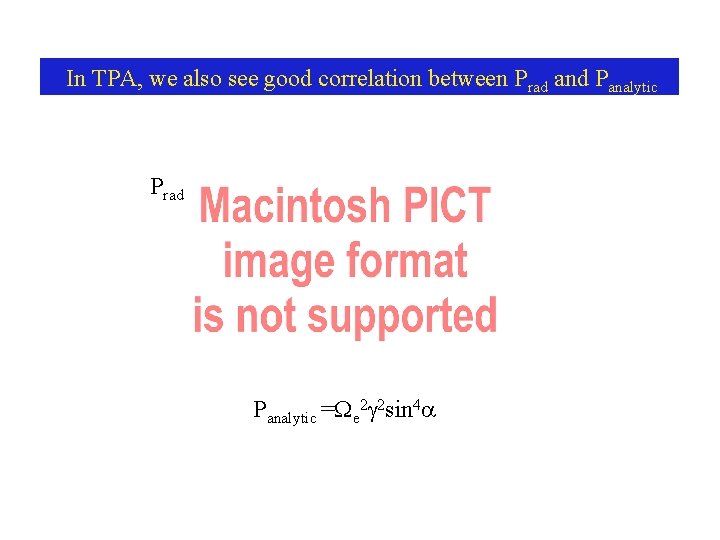
In TPA, we also see good correlation between Prad and Panalytic Prad Panalytic =We 2 2 sin 4 a
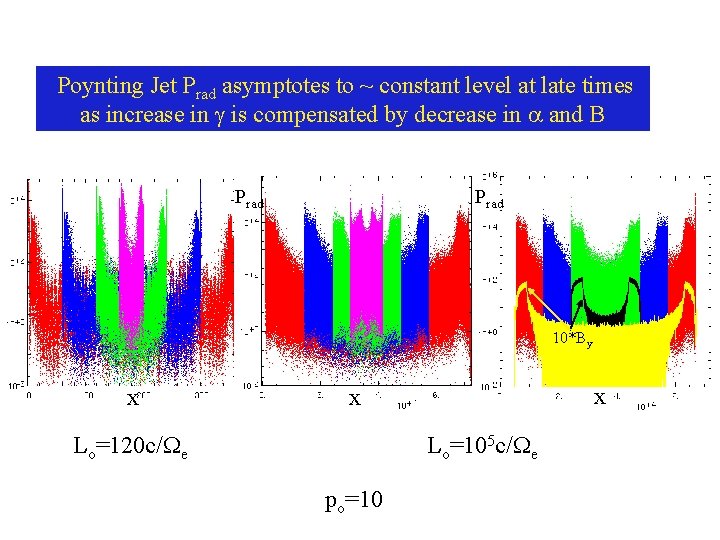
Poynting Jet Prad asymptotes to ~ constant level at late times as increase in is compensated by decrease in a and B Prad 10*By x x x Lo=120 c/We Lo=105 c/We po=10
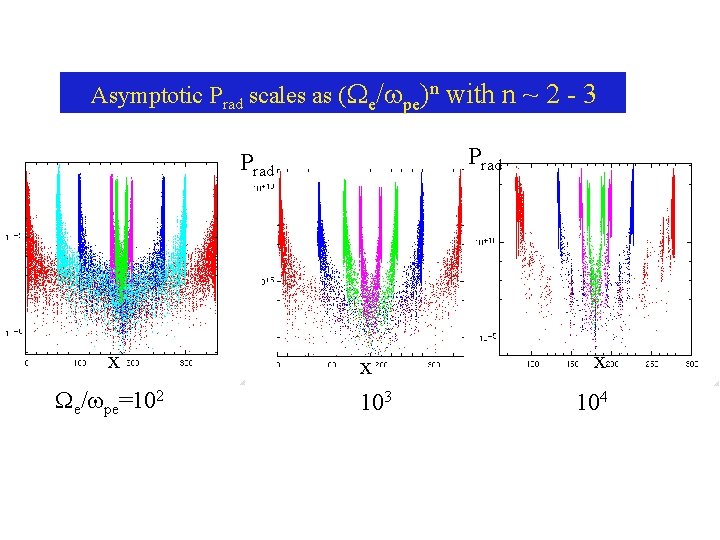
Asymptotic Prad scales as (We/wpe)n with n ~ 2 - 3 Prad x We/wpe=102 x 103 x 104
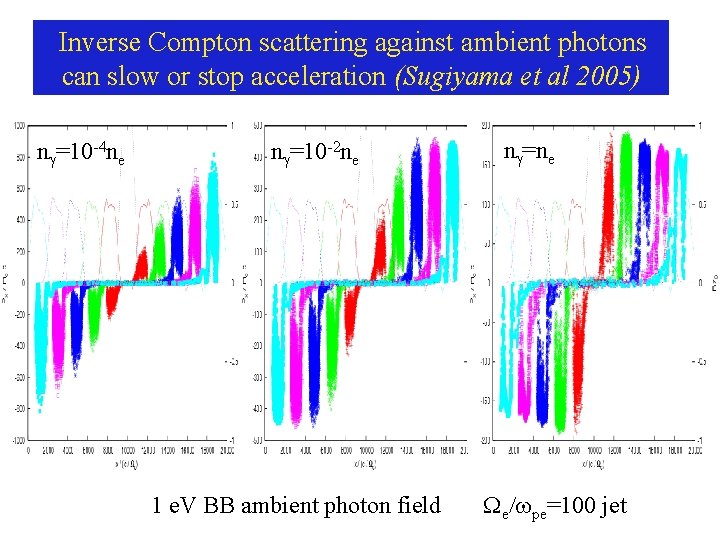
Inverse Compton scattering against ambient photons can slow or stop acceleration (Sugiyama et al 2005) n =10 -4 ne n =10 -2 ne 1 e. V BB ambient photon field n =ne We/wpe=100 jet
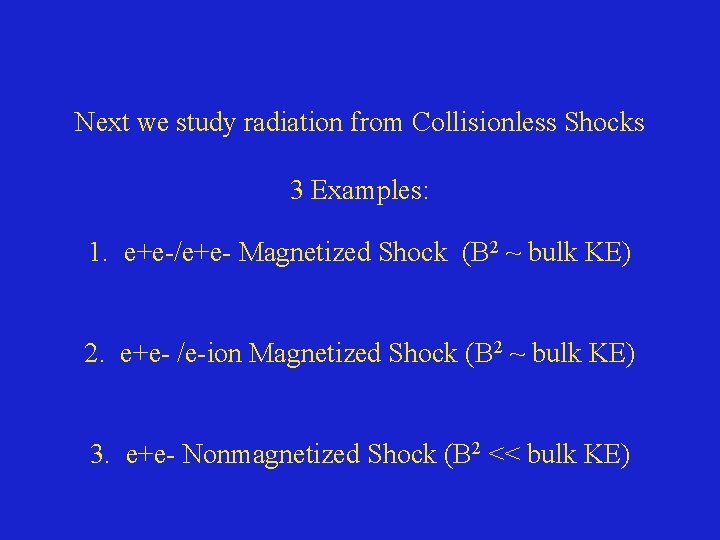
Next we study radiation from Collisionless Shocks 3 Examples: 1. e+e-/e+e- Magnetized Shock (B 2 ~ bulk KE) 2. e+e- /e-ion Magnetized Shock (B 2 ~ bulk KE) 3. e+e- Nonmagnetized Shock (B 2 << bulk KE)
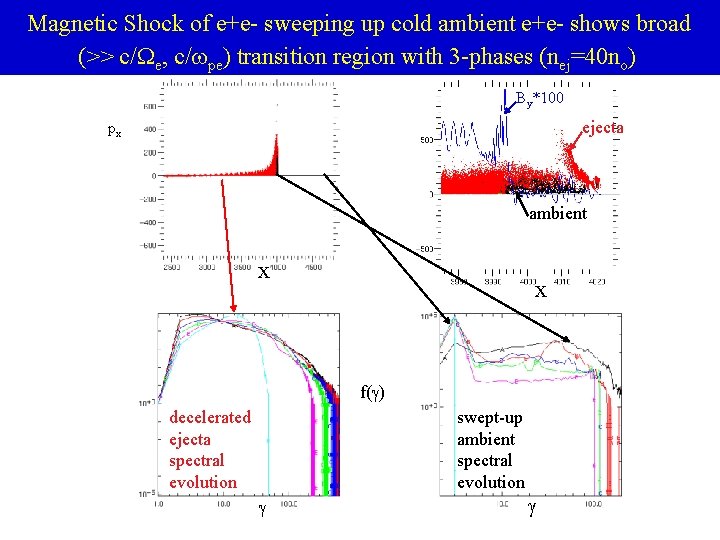
Magnetic Shock of e+e- sweeping up cold ambient e+e- shows broad (>> c/We, c/wpe) transition region with 3 -phases (nej=40 no) By*100 ejecta px ambient x x f( ) decelerated ejecta spectral evolution swept-up ambient spectral evolution
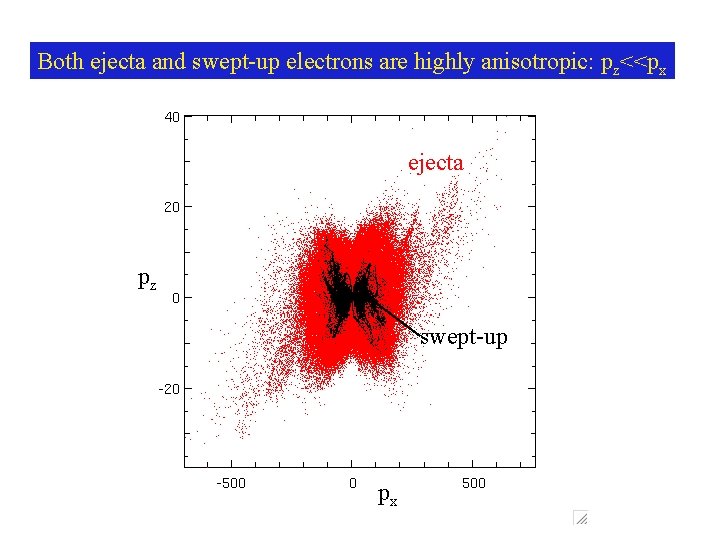
Both ejecta and swept-up electrons are highly anisotropic: pz<<px ejecta pz swept-up px
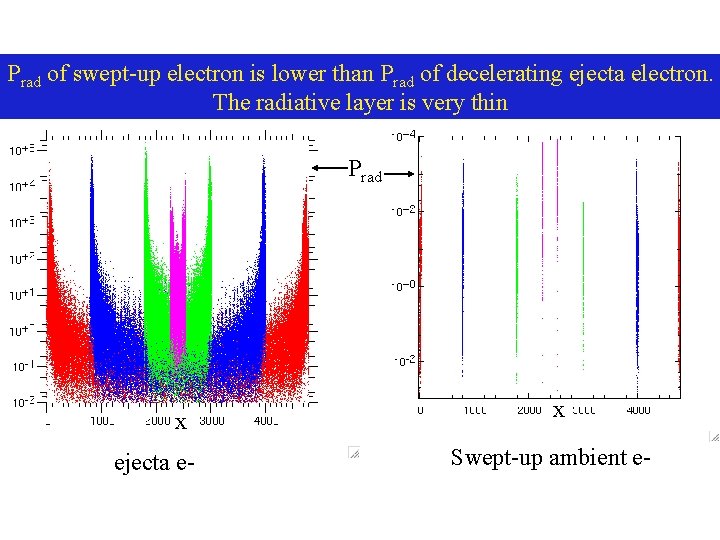
Prad of swept-up electron is lower than Prad of decelerating ejecta electron. The radiative layer is very thin Prad x ejecta e- x Swept-up ambient e-
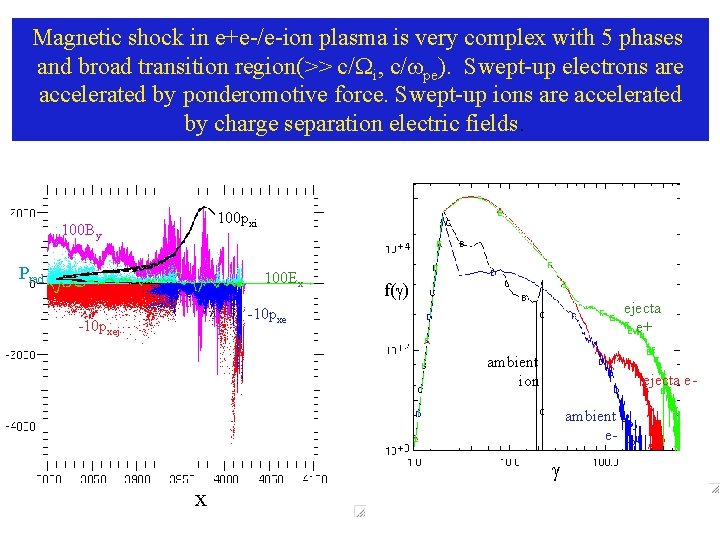
Magnetic shock in e+e-/e-ion plasma is very complex with 5 phases and broad transition region(>> c/Wi, c/wpe). Swept-up electrons are accelerated by ponderomotive force. Swept-up ions are accelerated by charge separation electric fields. 100 pxi 100 By Prad 100 Ex f( ) ejecta e+ -10 pxej ambient ion ejecta eambient e- x
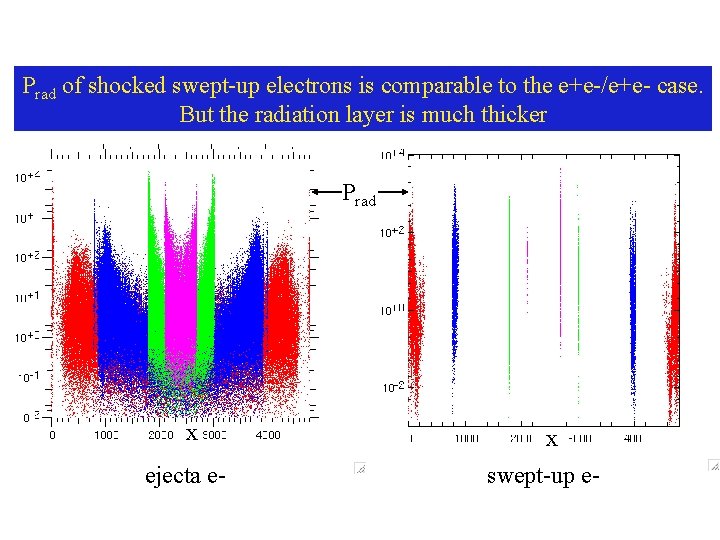
Prad of shocked swept-up electrons is comparable to the e+e-/e+e- case. But the radiation layer is much thicker Prad x ejecta e- x swept-up e-
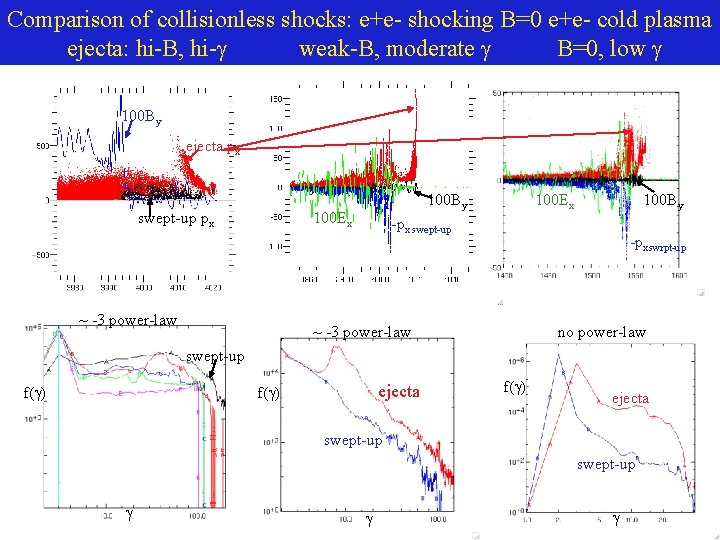
Comparison of collisionless shocks: e+e- shocking B=0 e+e- cold plasma ejecta: hi-B, hi- weak-B, moderate B=0, low 100 By ejecta px swept-up px 100 By 100 Ex ~ -3 power-law 100 Ex 100 By -px swept-up -pxswrpt-up no power-law ~ -3 power-law swept-up f( ) ejecta swept-up
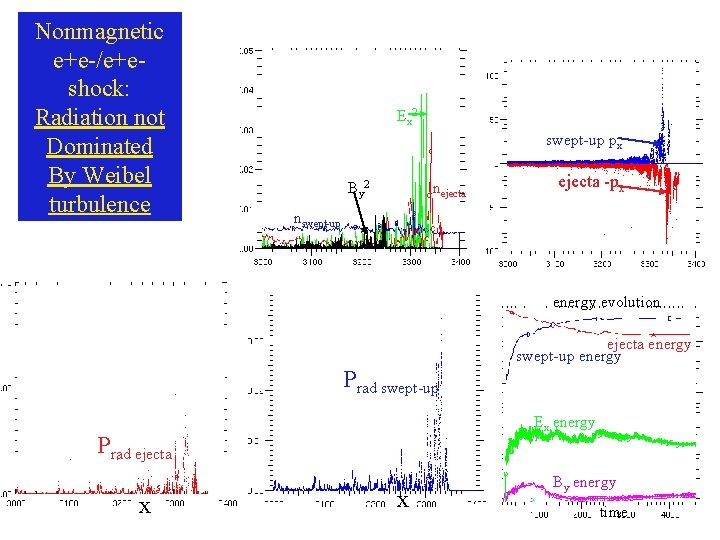
Nonmagnetic e+e-/e+eshock: Radiation not Dominated By Weibel turbulence Ex 2 swept-up px By 2 nejecta -px ejecta nswept-up energy evolution ejecta energy swept-up energy Prad swept-up Ex energy Prad ejecta x x By energy time
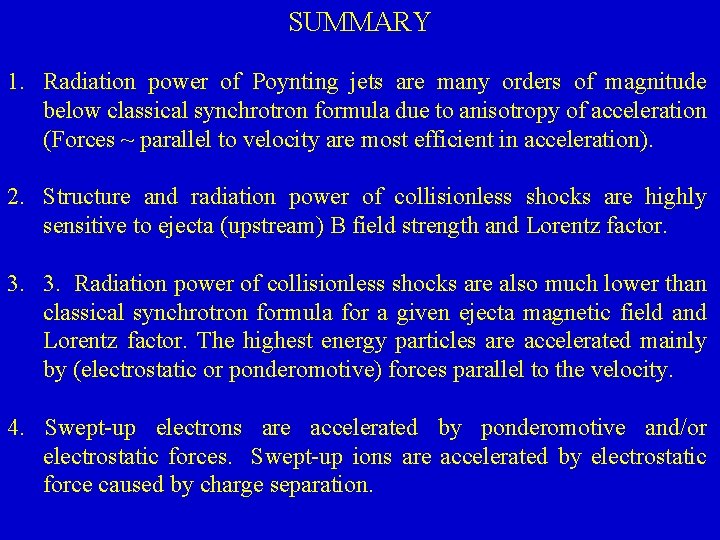
SUMMARY 1. Radiation power of Poynting jets are many orders of magnitude below classical synchrotron formula due to anisotropy of acceleration (Forces ~ parallel to velocity are most efficient in acceleration). 2. Structure and radiation power of collisionless shocks are highly sensitive to ejecta (upstream) B field strength and Lorentz factor. 3. 3. Radiation power of collisionless shocks are also much lower than classical synchrotron formula for a given ejecta magnetic field and Lorentz factor. The highest energy particles are accelerated mainly by (electrostatic or ponderomotive) forces parallel to the velocity. 4. Swept-up electrons are accelerated by ponderomotive and/or electrostatic forces. Swept-up ions are accelerated by electrostatic force caused by charge separation.
Who are the mrunas
Fsae shocks
Aggregate of chapter 6
Black hole jets
Microquasars
Jets
Jets
Parton distribution functions
Jets
Spice jets
Spice jets
Maxwell's equations
Derive the electromagnetic wave equation
Poynting vector and intensity
Polarization ellipse equation
Poynting vector
Maxwell's equations
Poynting vector formula
Poynting vector dimensional formula
Esperimento di hertz onde elettromagnetiche zanichelli
Poynting theorem
Poynting effect anesthesia
Fator de poynting
Optics poynting's theorem