QuantumDot Lasers Nanoelectronics term project R 91543013 Outline
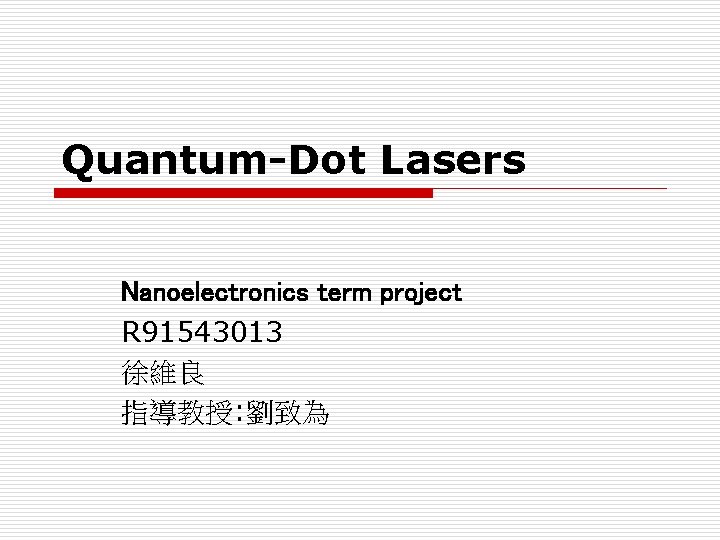
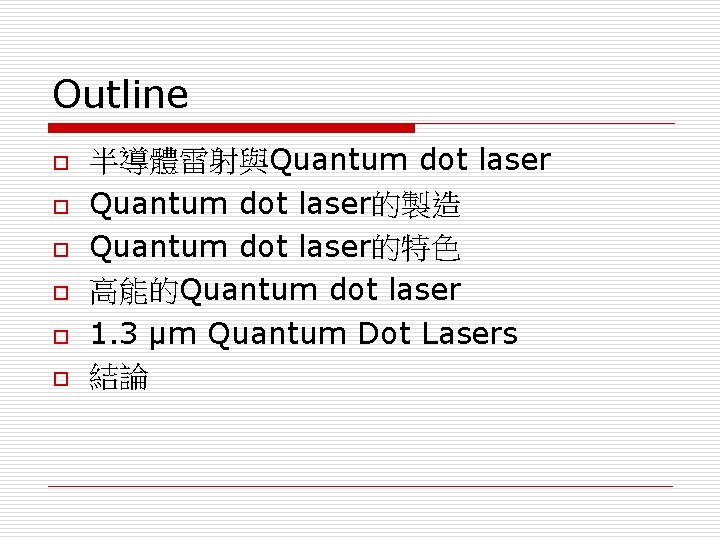
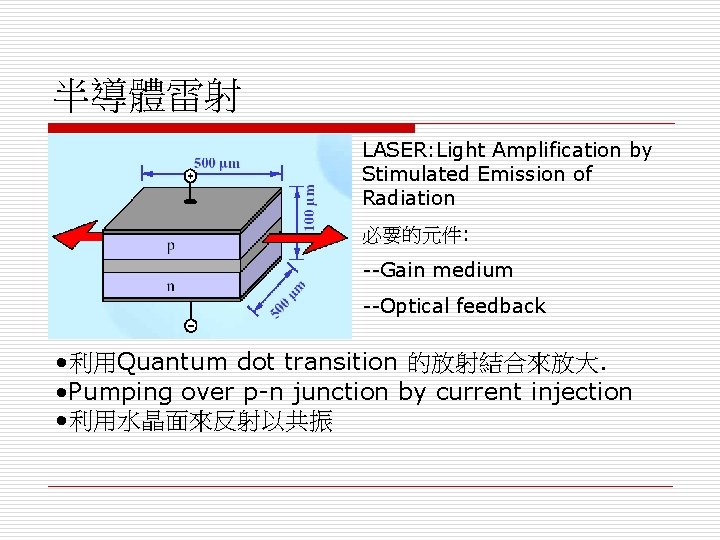
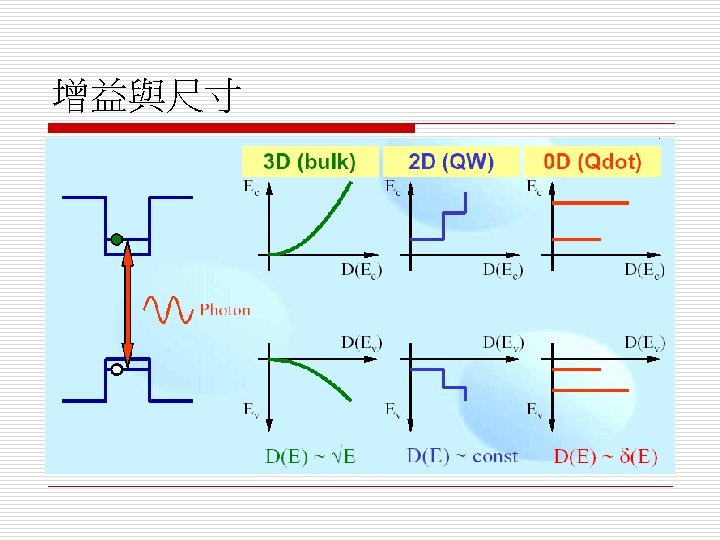
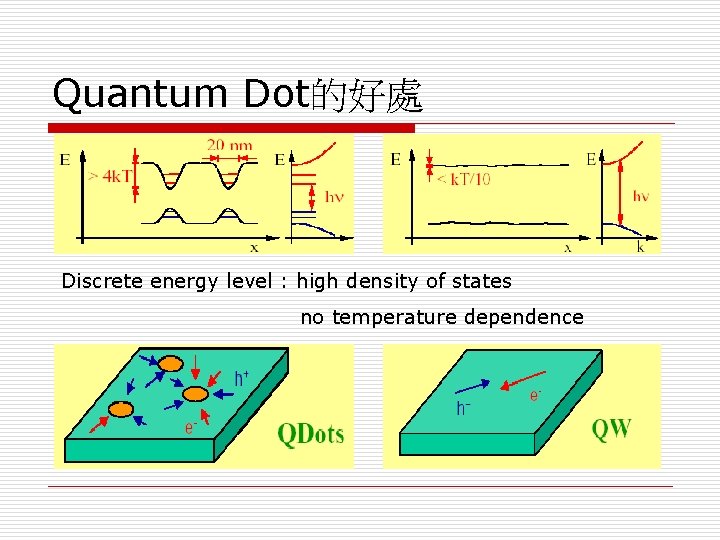
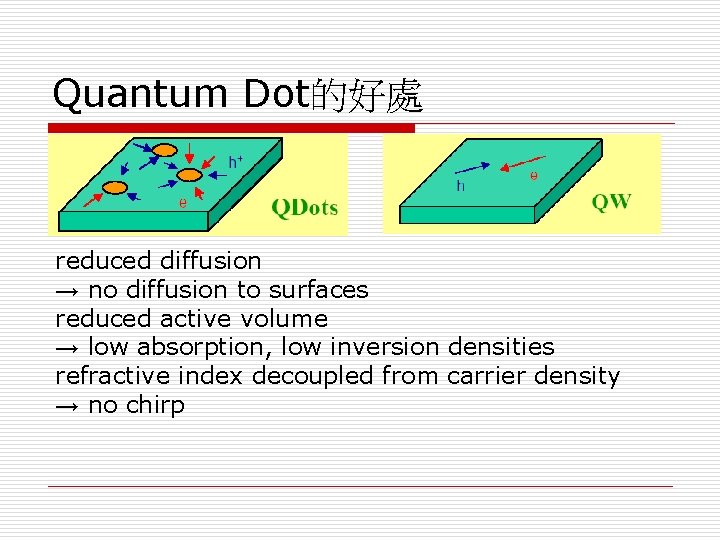
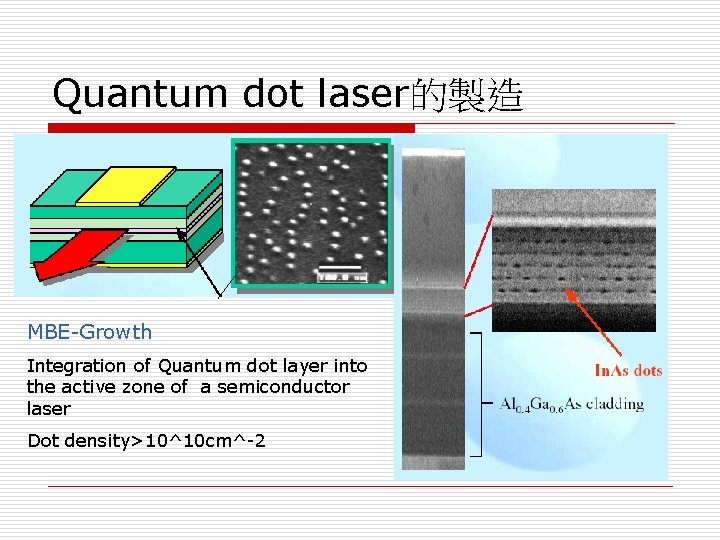
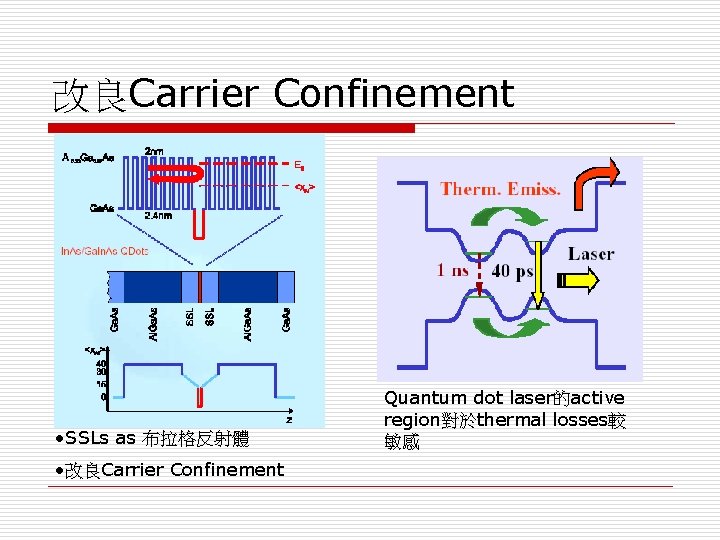
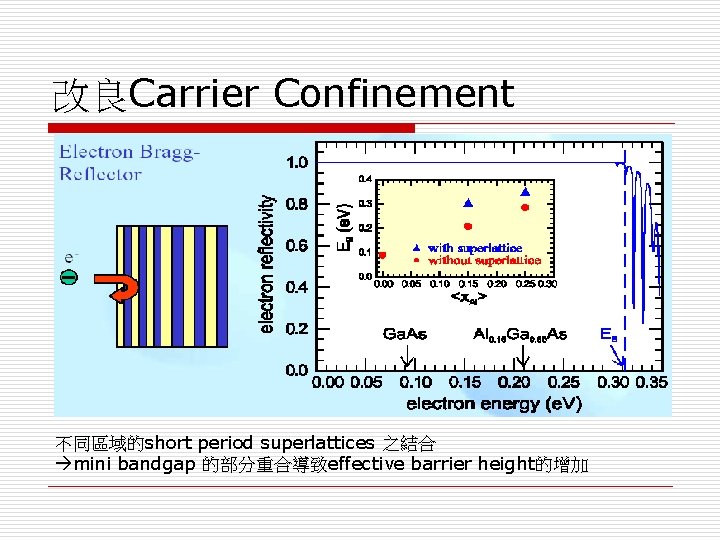
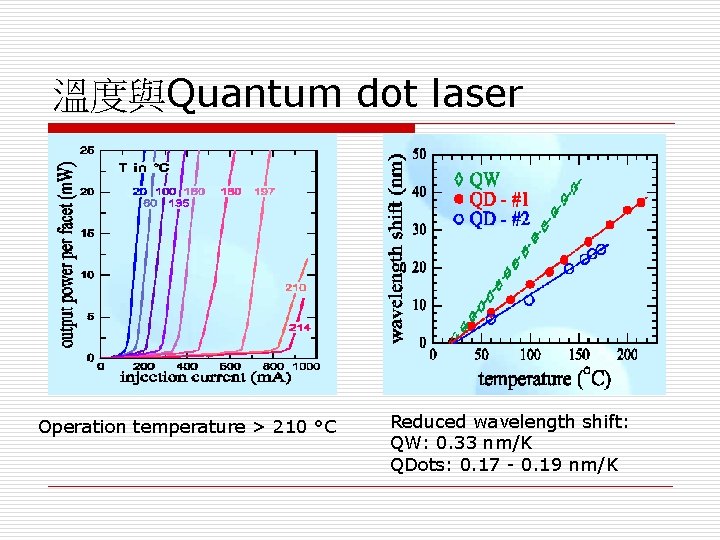
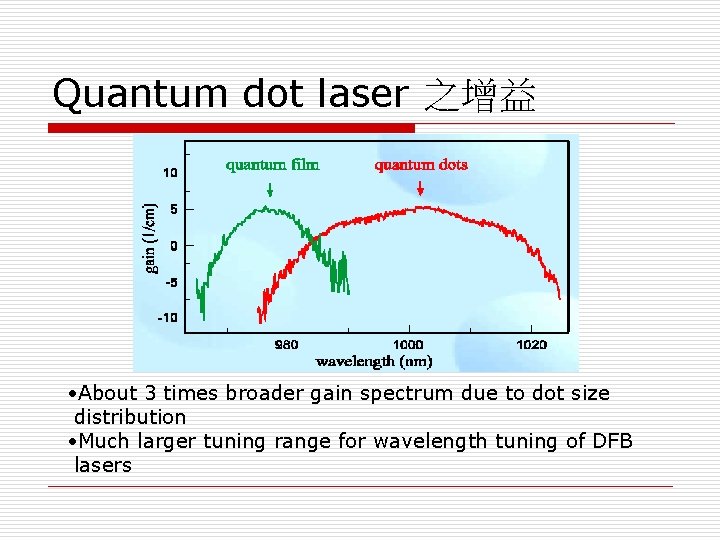
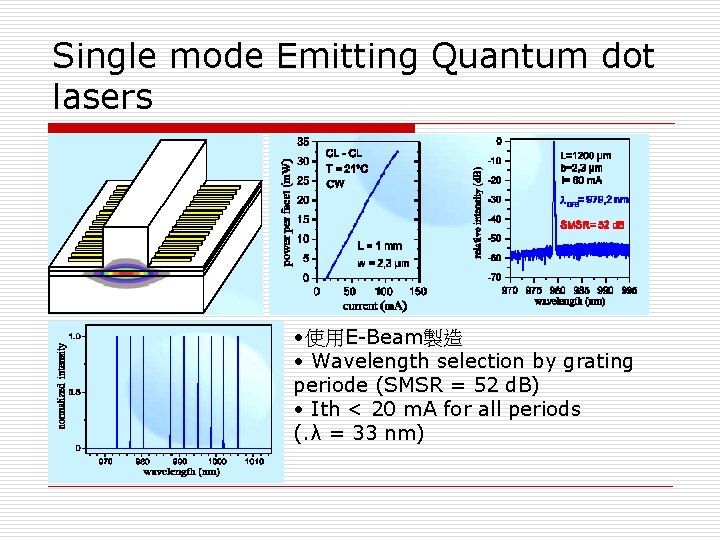
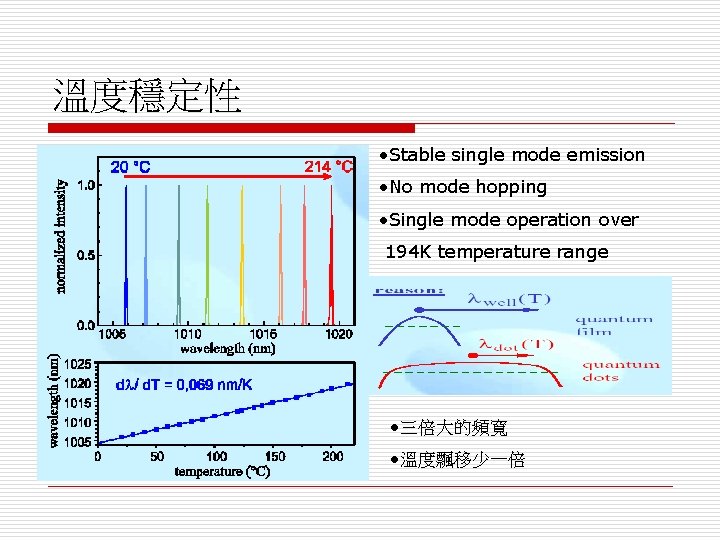
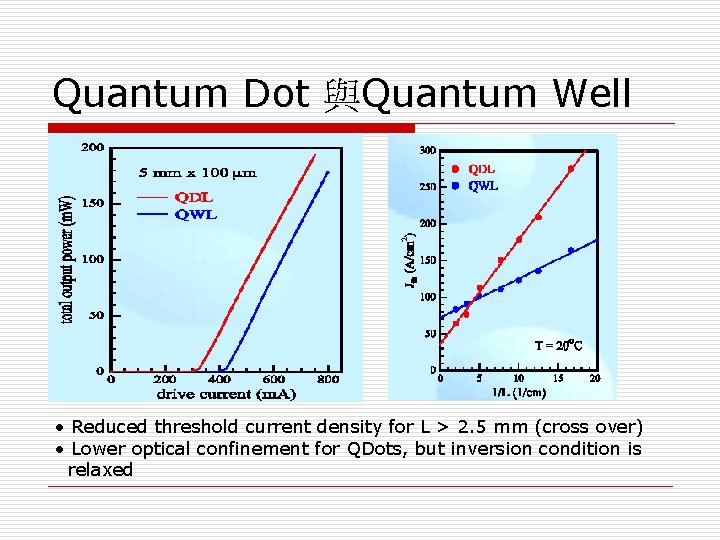
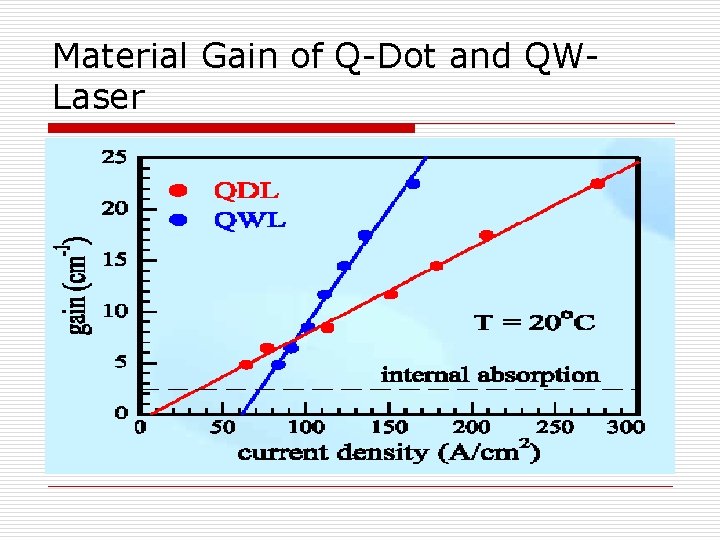
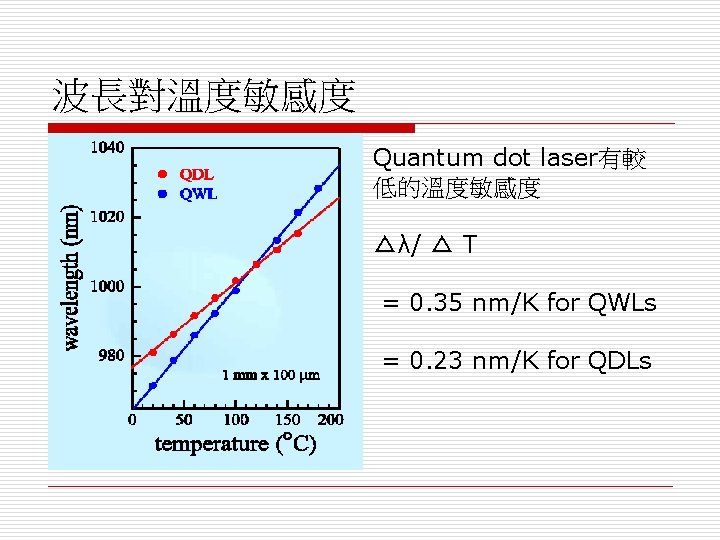
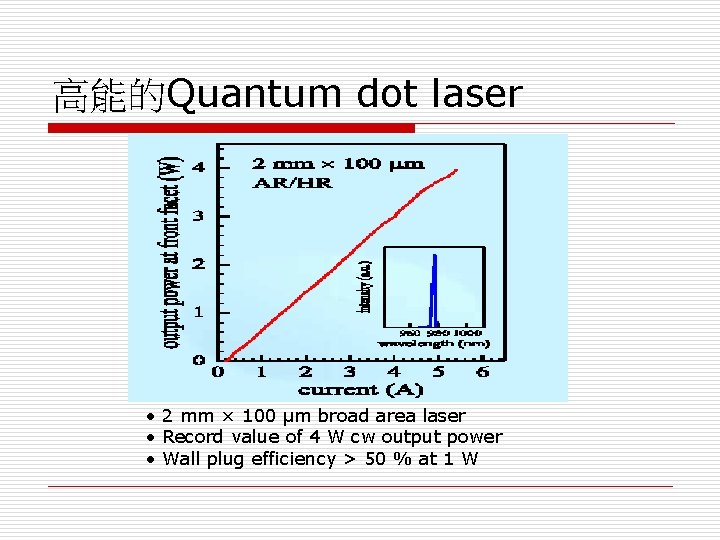
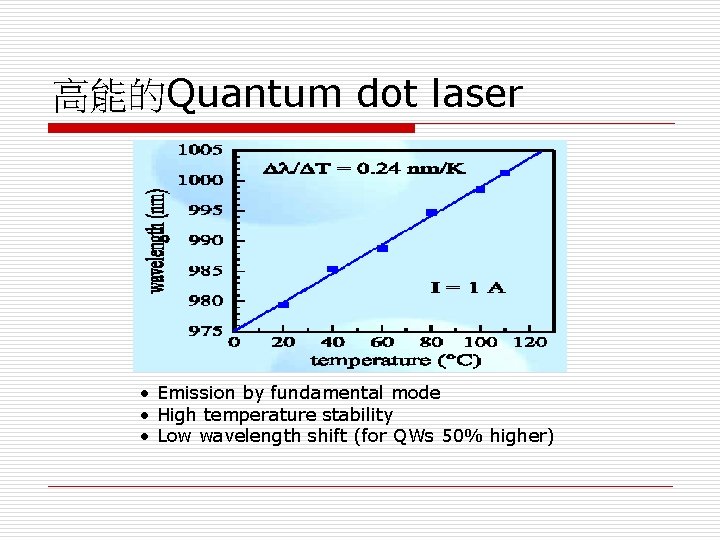
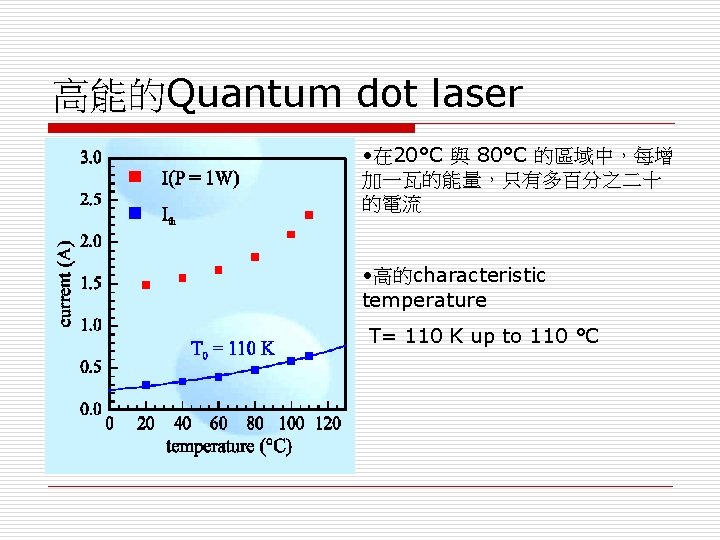
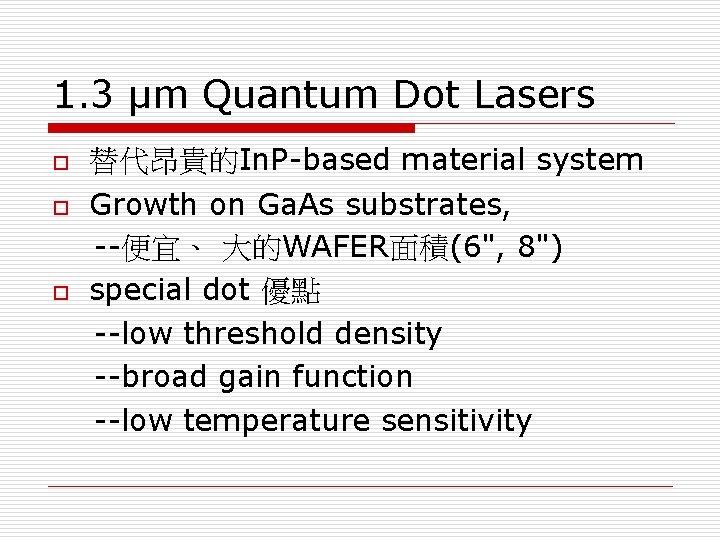
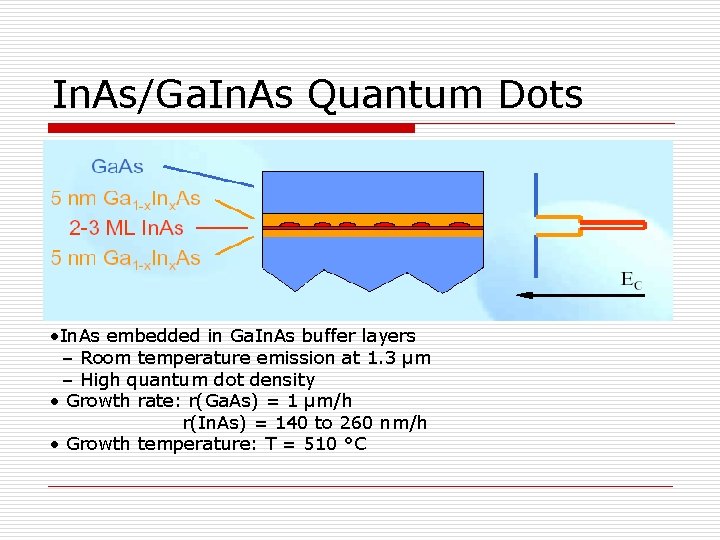
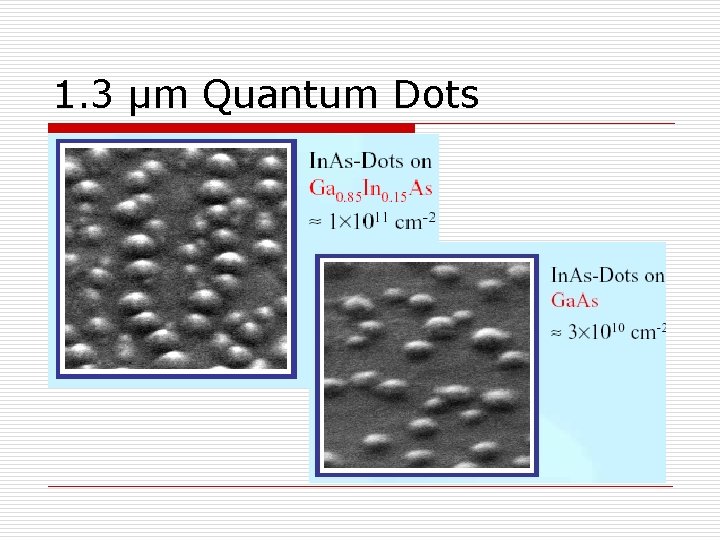
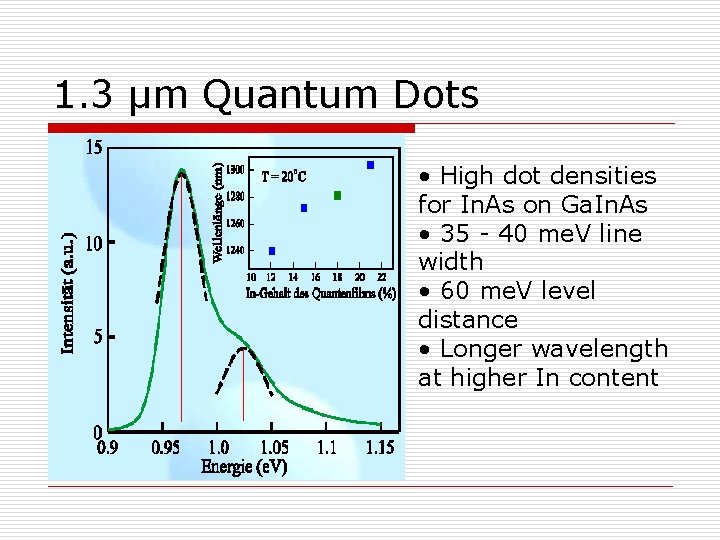
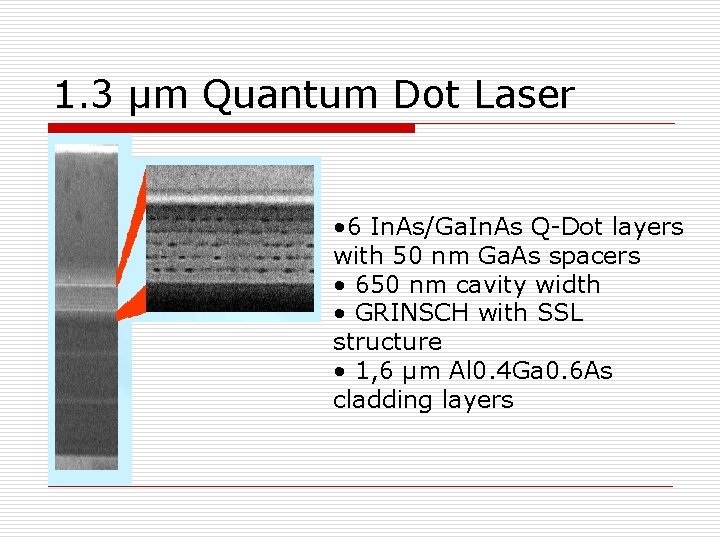
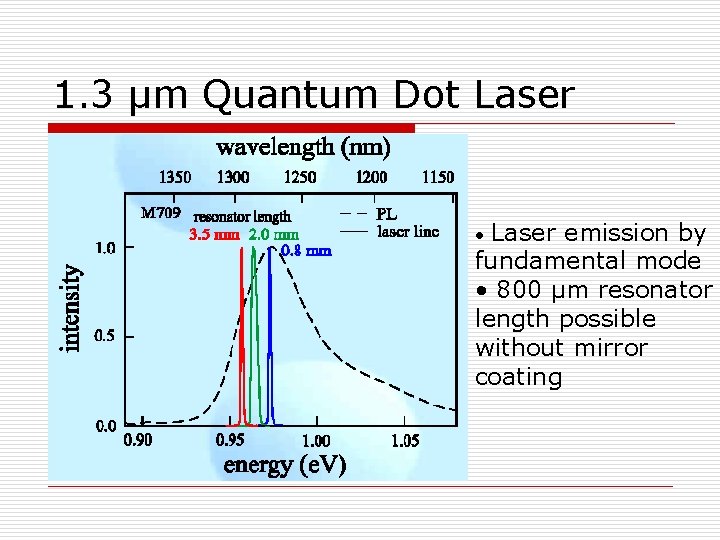
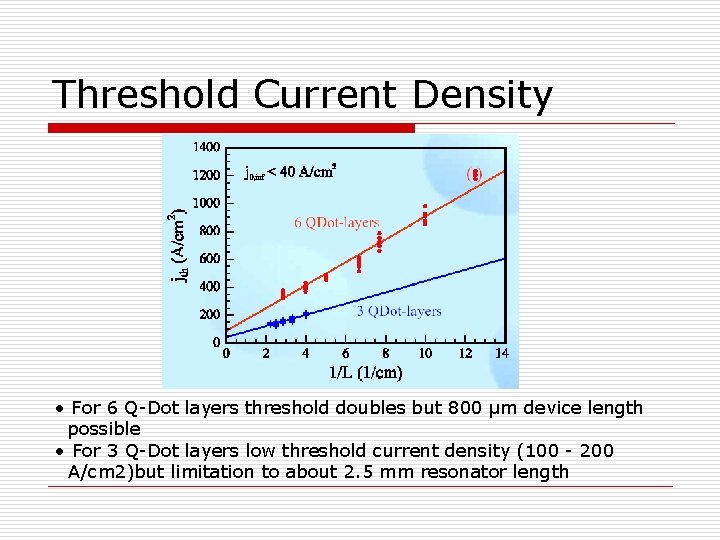
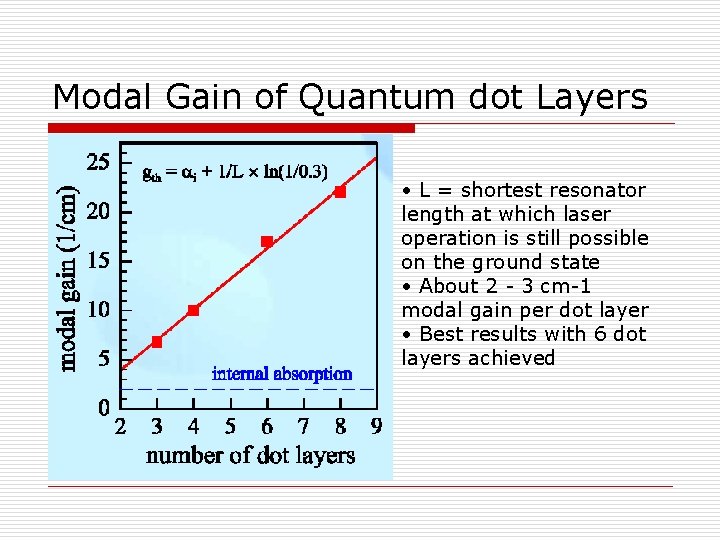
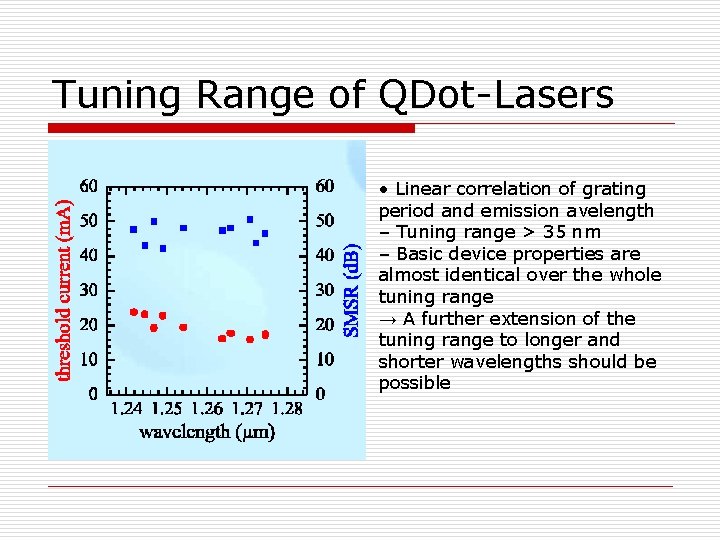
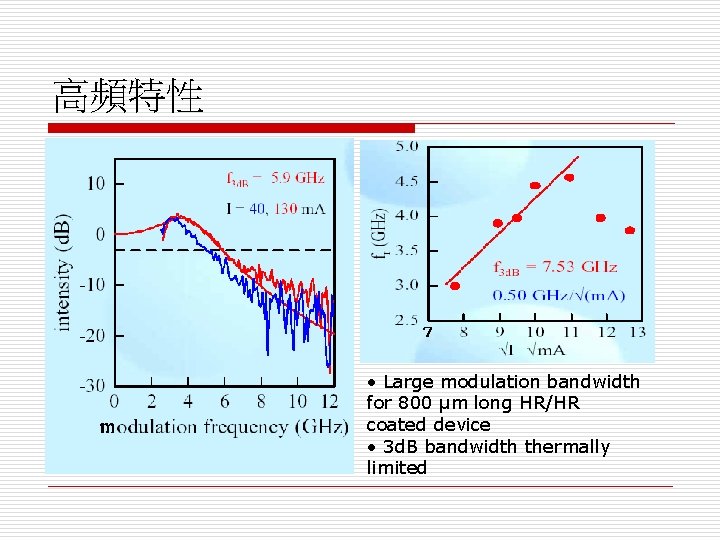
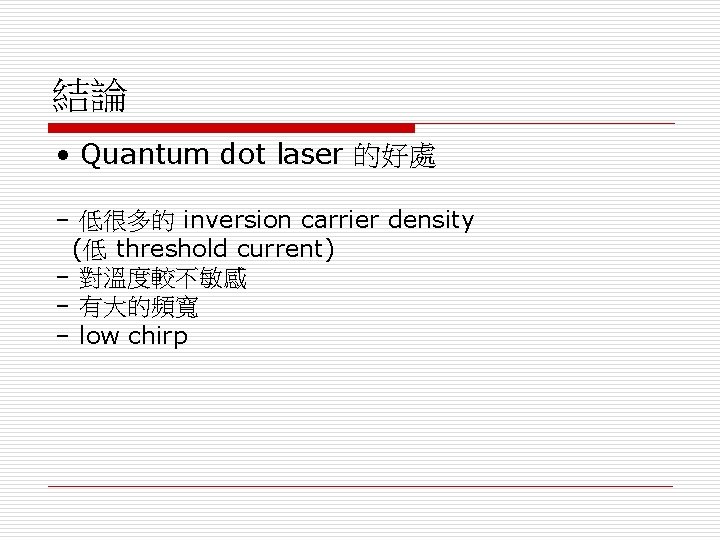
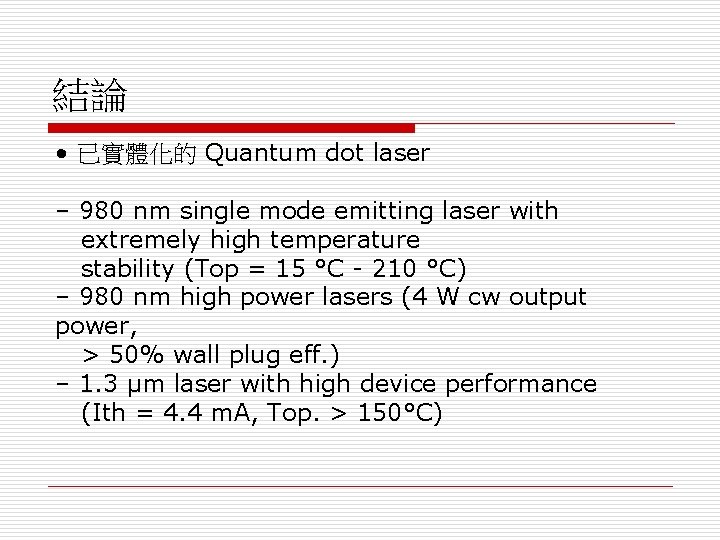
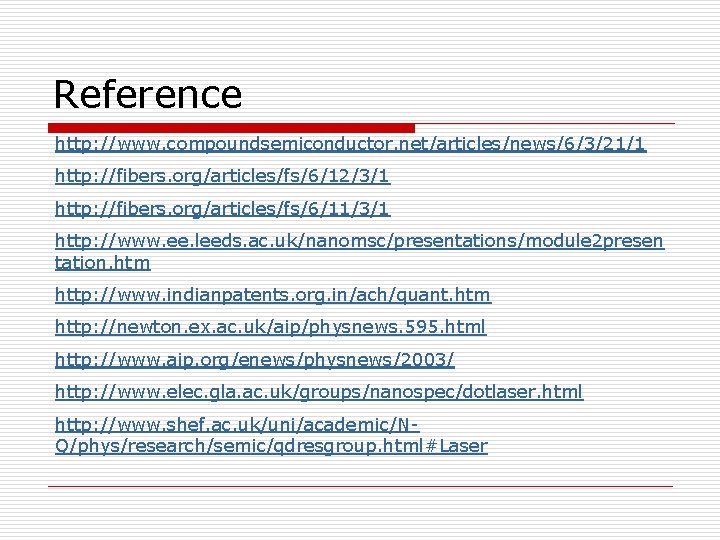
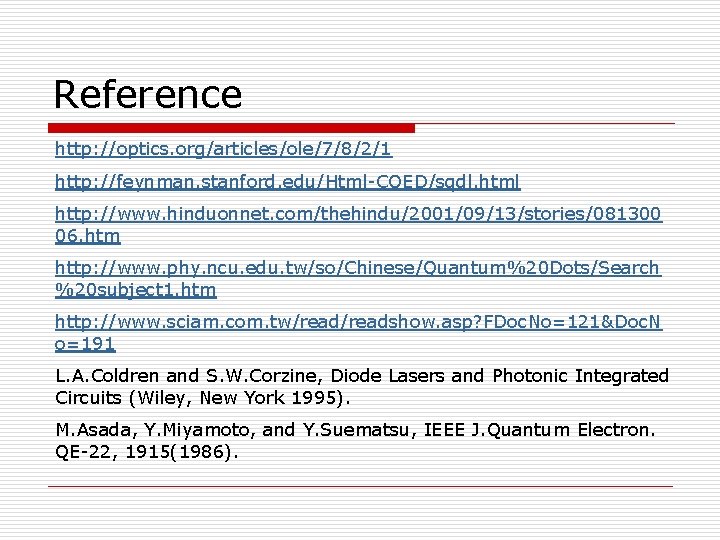
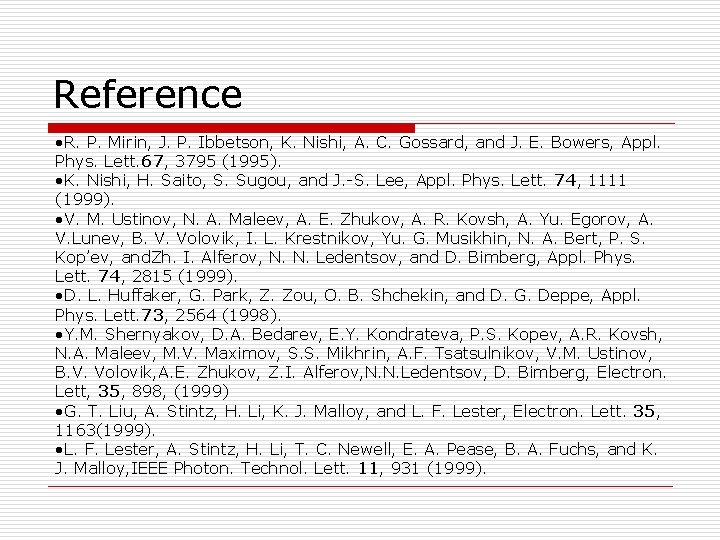
- Slides: 34
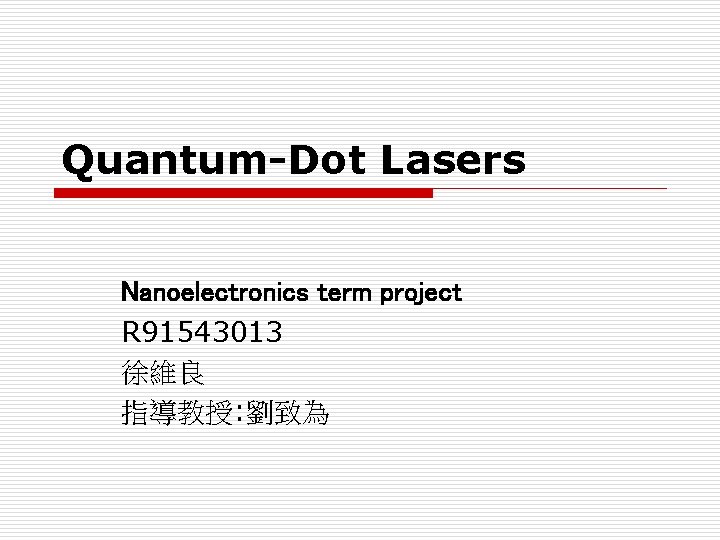
Quantum-Dot Lasers Nanoelectronics term project R 91543013 徐維良 指導教授: 劉致為
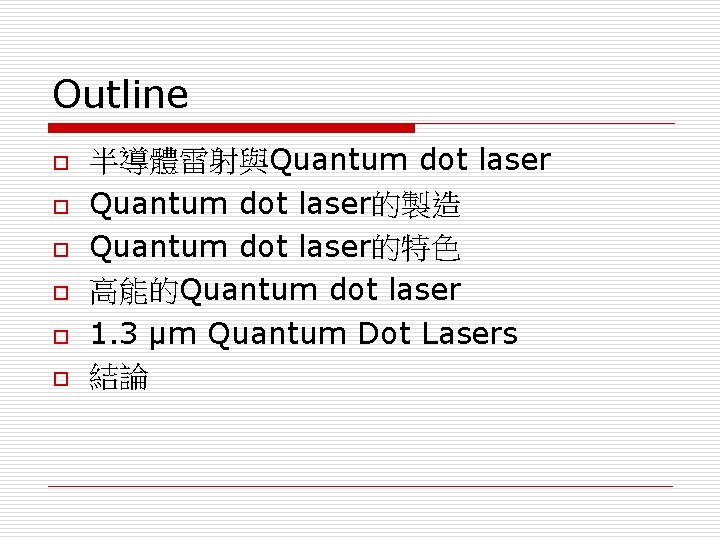
Outline o o o 半導體雷射與Quantum dot laser的製造 Quantum dot laser的特色 高能的Quantum dot laser 1. 3 µm Quantum Dot Lasers 結論
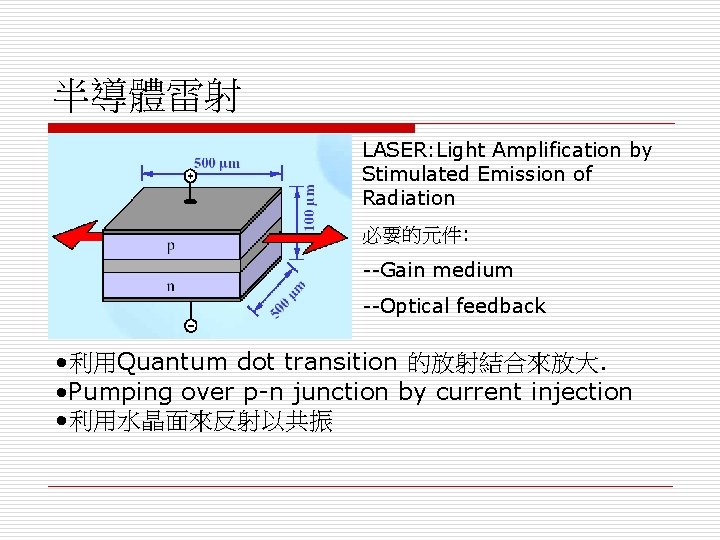
半導體雷射 LASER: Light Amplification by Stimulated Emission of Radiation 必要的元件: --Gain medium --Optical feedback • 利用Quantum dot transition 的放射結合來放大. • Pumping over p-n junction by current injection • 利用水晶面來反射以共振
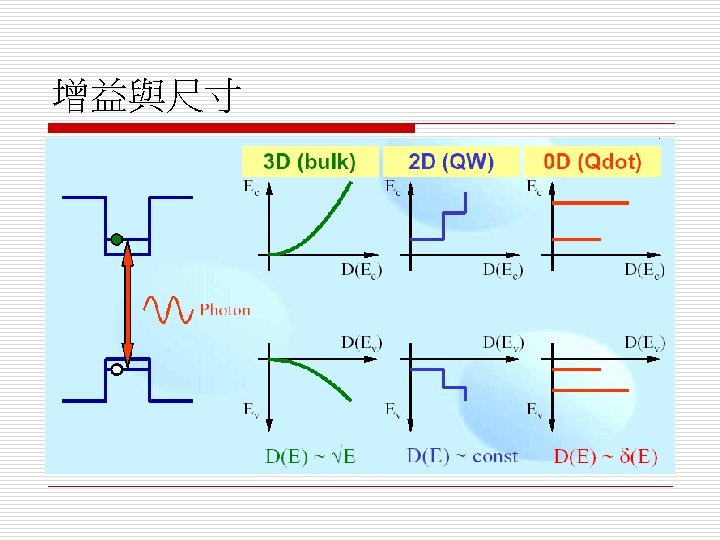
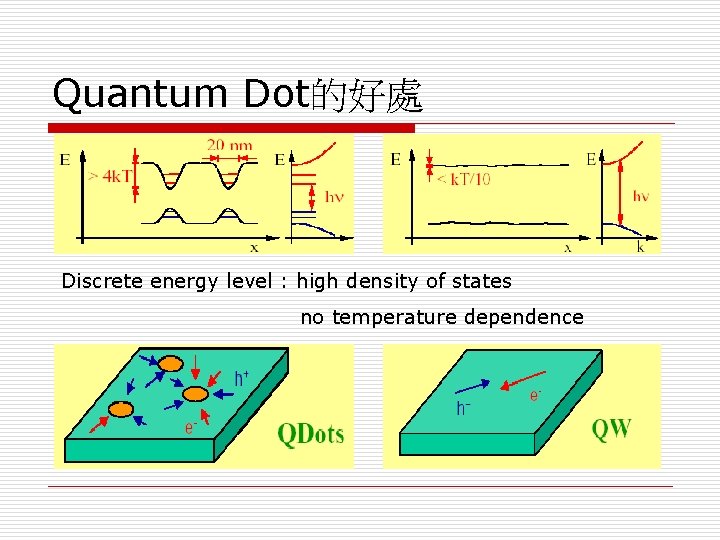
Quantum Dot的好處 Discrete energy level : high density of states no temperature dependence
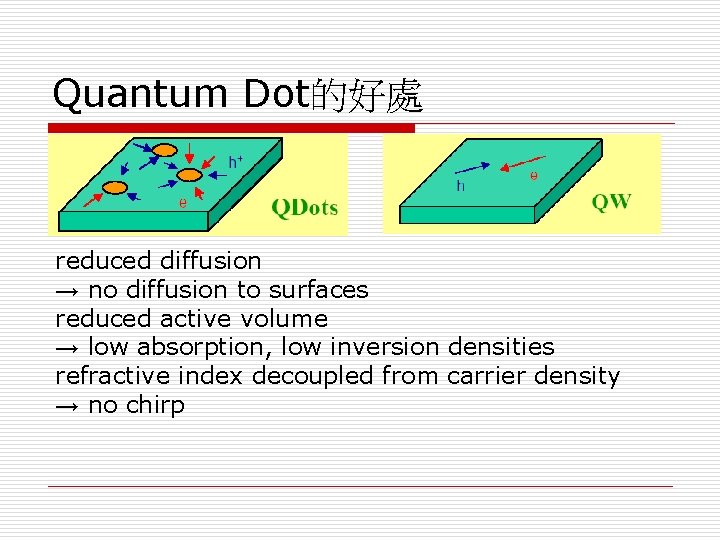
Quantum Dot的好處 reduced diffusion → no diffusion to surfaces reduced active volume → low absorption, low inversion densities refractive index decoupled from carrier density → no chirp
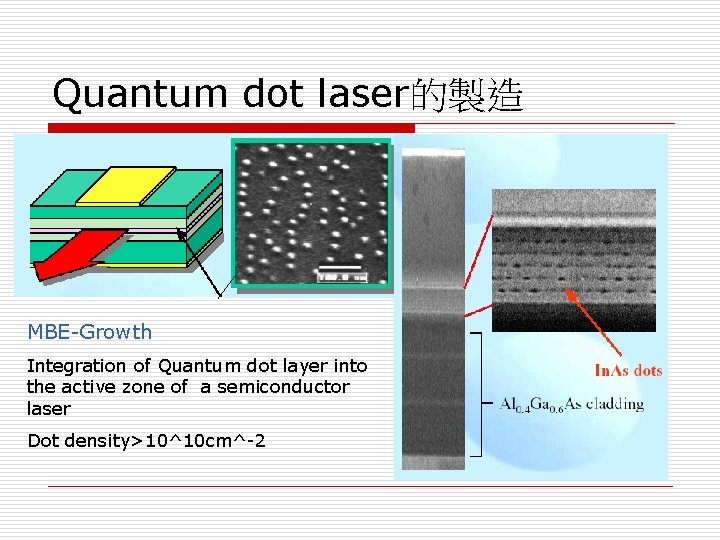
Quantum dot laser的製造 MBE-Growth Integration of Quantum dot layer into the active zone of a semiconductor laser Dot density>10^10 cm^-2
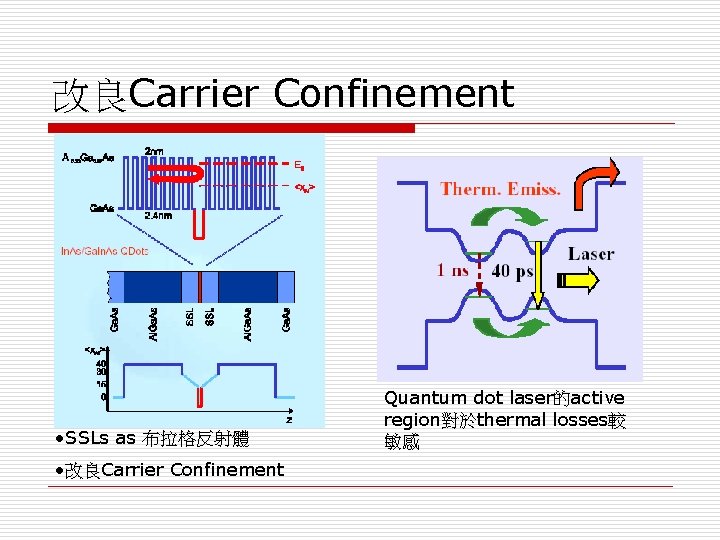
改良Carrier Confinement • SSLs as 布拉格反射體 • 改良Carrier Confinement Quantum dot laser的active region對於thermal losses較 敏感
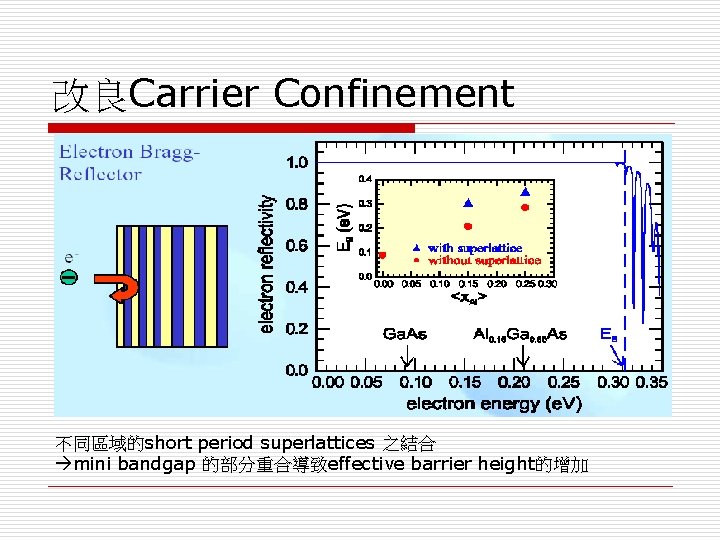
改良Carrier Confinement 不同區域的short period superlattices 之結合 mini bandgap 的部分重合導致effective barrier height的增加
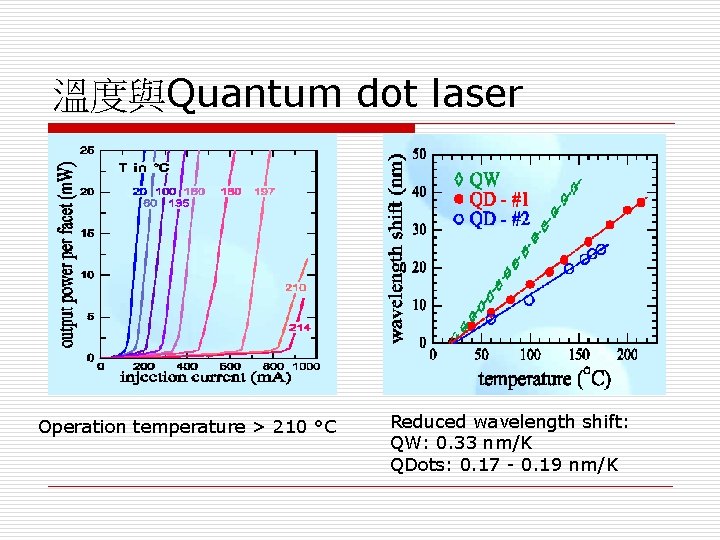
溫度與Quantum dot laser Operation temperature > 210 °C Reduced wavelength shift: QW: 0. 33 nm/K QDots: 0. 17 - 0. 19 nm/K
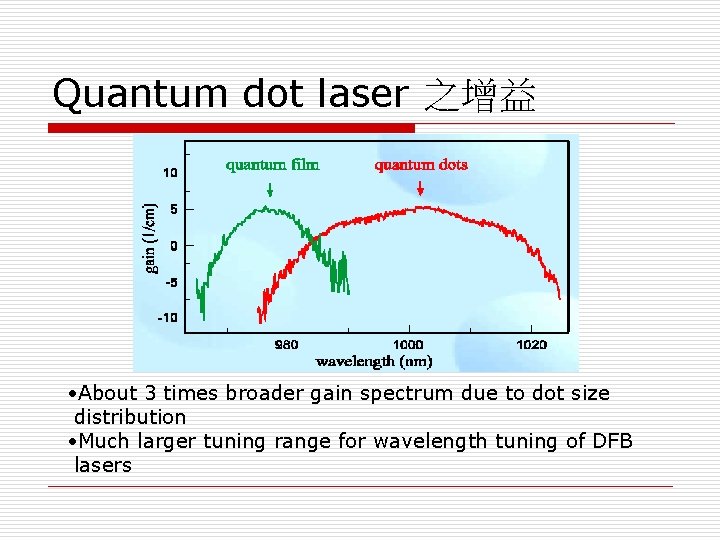
Quantum dot laser 之增益 • About 3 times broader gain spectrum due to dot size distribution • Much larger tuning range for wavelength tuning of DFB lasers
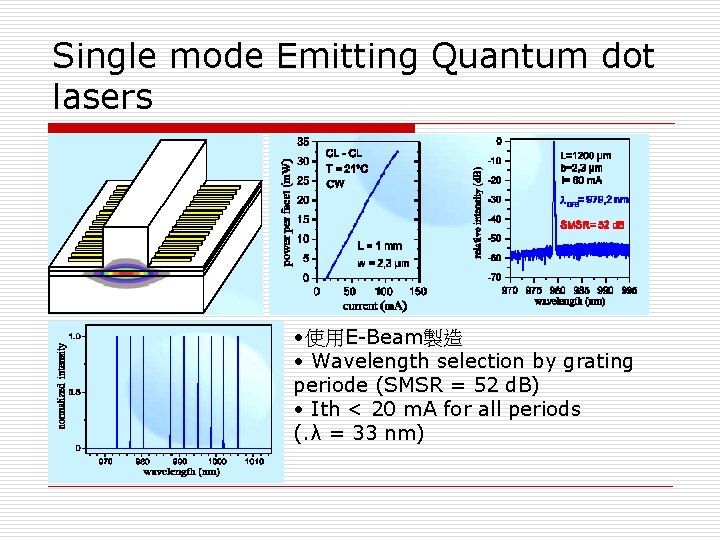
Single mode Emitting Quantum dot lasers • 使用E-Beam製造 • Wavelength selection by grating periode (SMSR = 52 d. B) • Ith < 20 m. A for all periods (. λ = 33 nm)
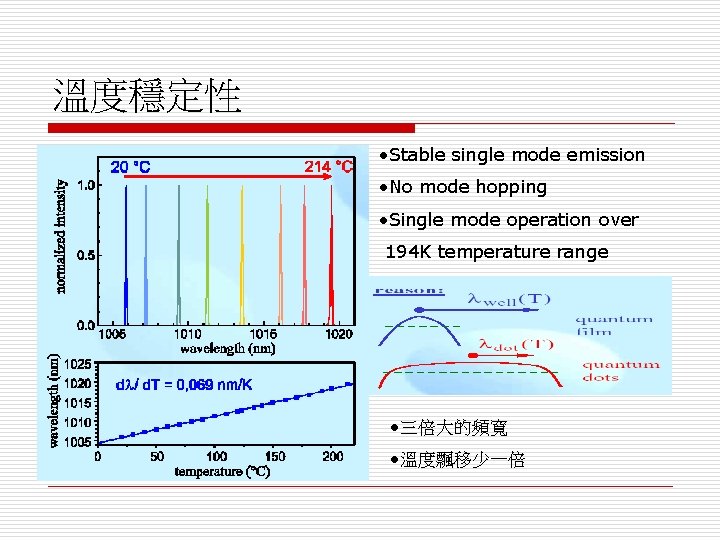
溫度穩定性 • Stable single mode emission • No mode hopping • Single mode operation over 194 K temperature range • 三倍大的頻寬 • 溫度飄移少一倍
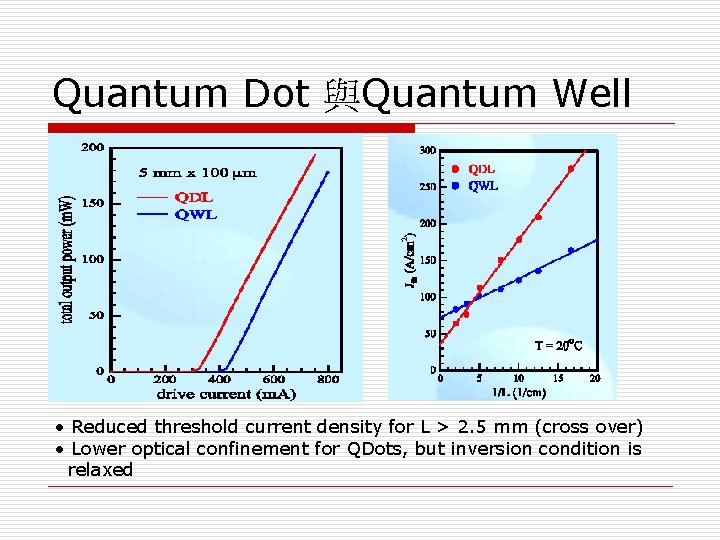
Quantum Dot 與Quantum Well • Reduced threshold current density for L > 2. 5 mm (cross over) • Lower optical confinement for QDots, but inversion condition is relaxed
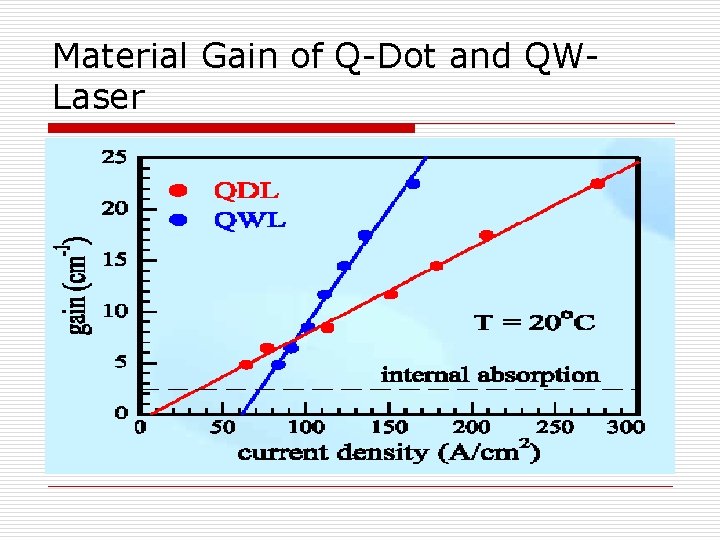
Material Gain of Q-Dot and QWLaser
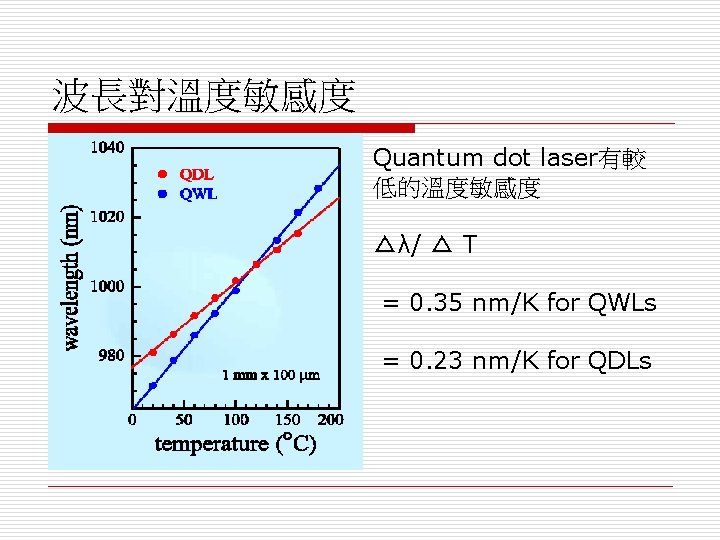
波長對溫度敏感度 Quantum dot laser有較 低的溫度敏感度 △λ/ △ T = 0. 35 nm/K for QWLs = 0. 23 nm/K for QDLs
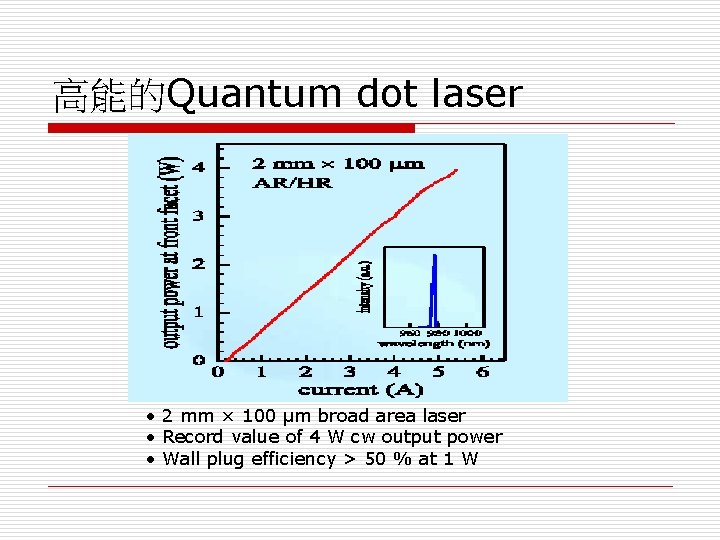
高能的Quantum dot laser • 2 mm × 100 µm broad area laser • Record value of 4 W cw output power • Wall plug efficiency > 50 % at 1 W
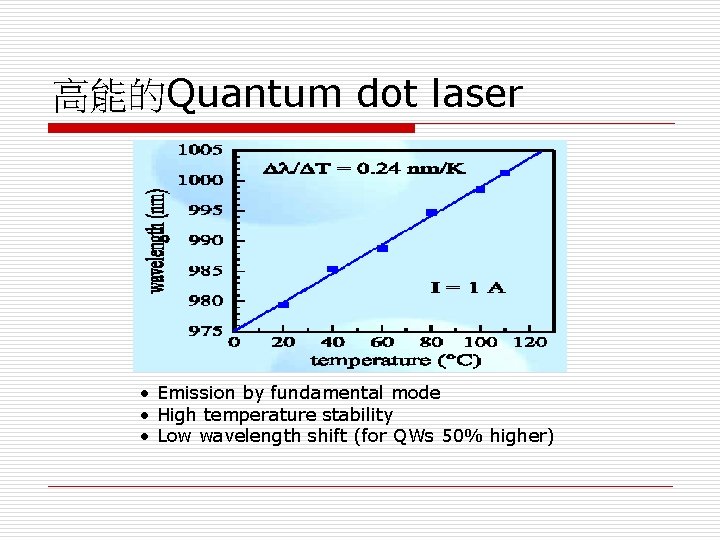
高能的Quantum dot laser • Emission by fundamental mode • High temperature stability • Low wavelength shift (for QWs 50% higher)
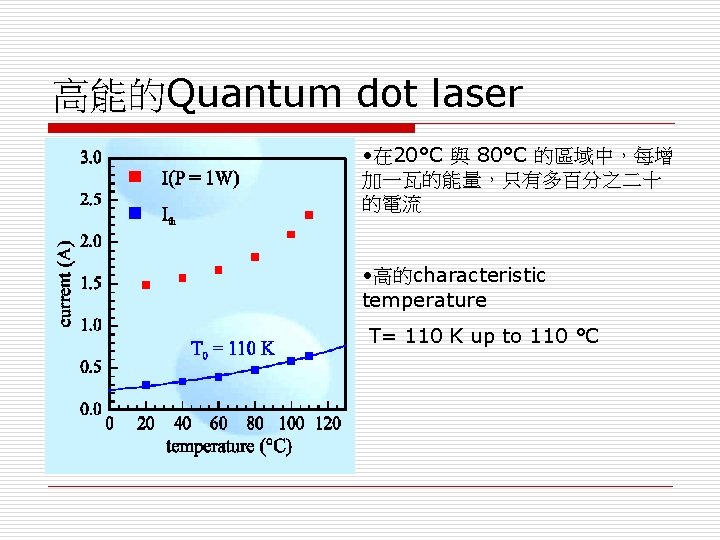
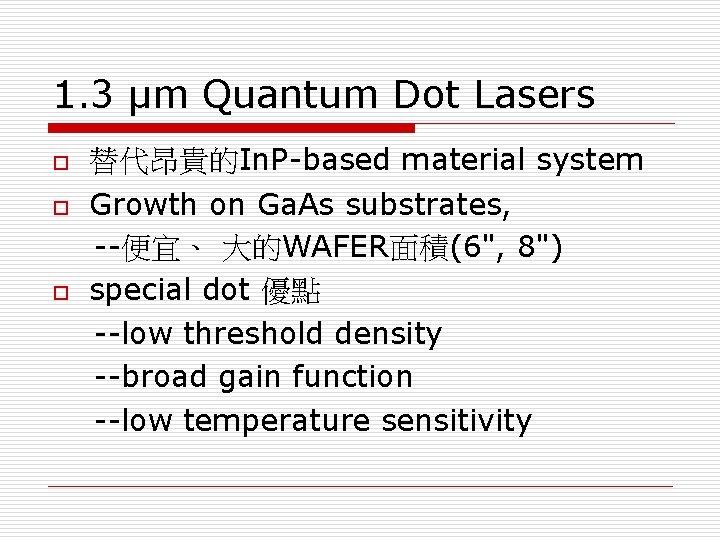
1. 3 µm Quantum Dot Lasers o o o 替代昂貴的In. P-based material system Growth on Ga. As substrates, --便宜、 大的WAFER面積(6", 8") special dot 優點 --low threshold density --broad gain function --low temperature sensitivity
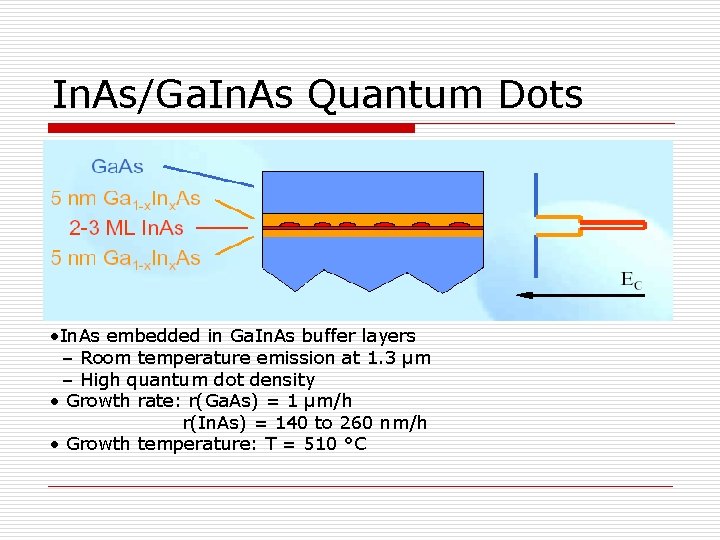
In. As/Ga. In. As Quantum Dots • In. As embedded in Ga. In. As buffer layers – Room temperature emission at 1. 3 µm – High quantum dot density • Growth rate: r(Ga. As) = 1 µm/h r(In. As) = 140 to 260 nm/h • Growth temperature: T = 510 °C
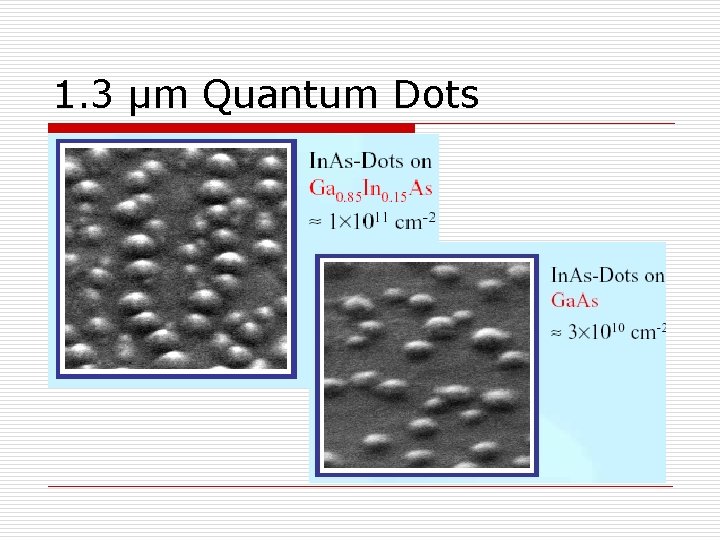
1. 3 µm Quantum Dots
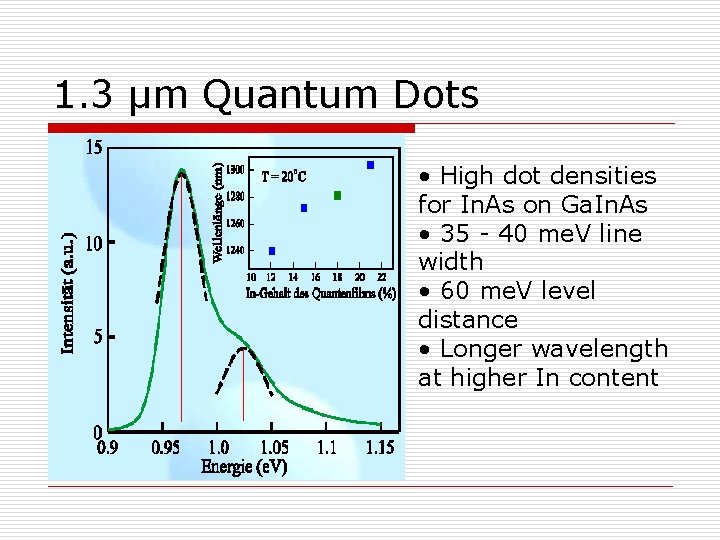
1. 3 µm Quantum Dots • High dot densities for In. As on Ga. In. As • 35 - 40 me. V line width • 60 me. V level distance • Longer wavelength at higher In content
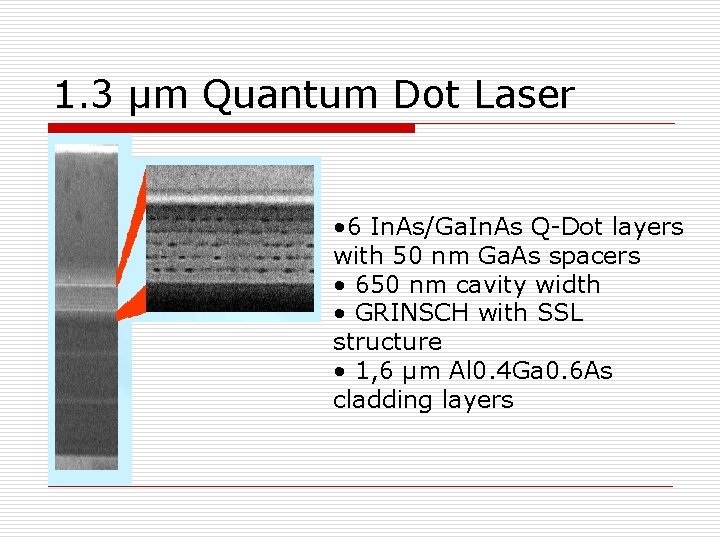
1. 3 µm Quantum Dot Laser • 6 In. As/Ga. In. As Q-Dot layers with 50 nm Ga. As spacers • 650 nm cavity width • GRINSCH with SSL structure • 1, 6 µm Al 0. 4 Ga 0. 6 As cladding layers
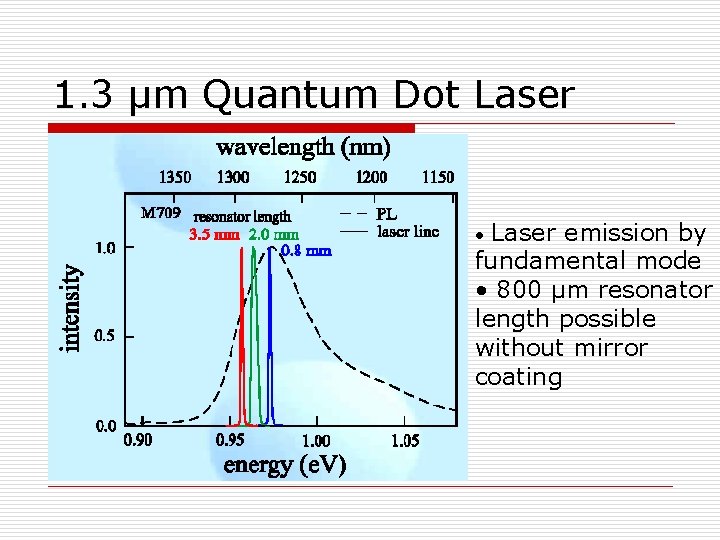
1. 3 µm Quantum Dot Laser emission by fundamental mode • 800 µm resonator length possible without mirror coating •
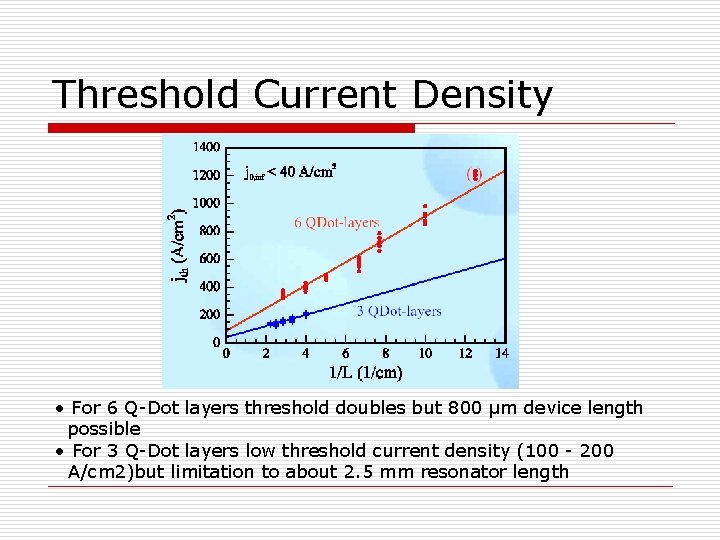
Threshold Current Density • For 6 Q-Dot layers threshold doubles but 800 µm device length possible • For 3 Q-Dot layers low threshold current density (100 - 200 A/cm 2)but limitation to about 2. 5 mm resonator length
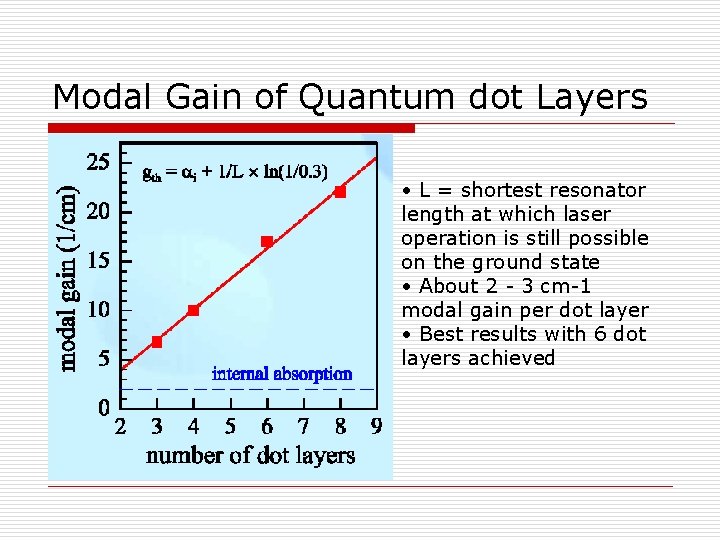
Modal Gain of Quantum dot Layers • L = shortest resonator length at which laser operation is still possible on the ground state • About 2 - 3 cm-1 modal gain per dot layer • Best results with 6 dot layers achieved
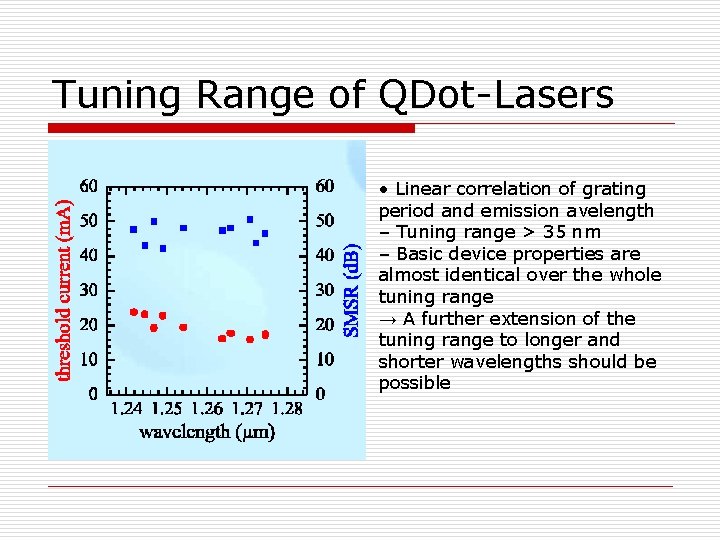
Tuning Range of QDot-Lasers • Linear correlation of grating period and emission avelength – Tuning range > 35 nm – Basic device properties are almost identical over the whole tuning range → A further extension of the tuning range to longer and shorter wavelengths should be possible
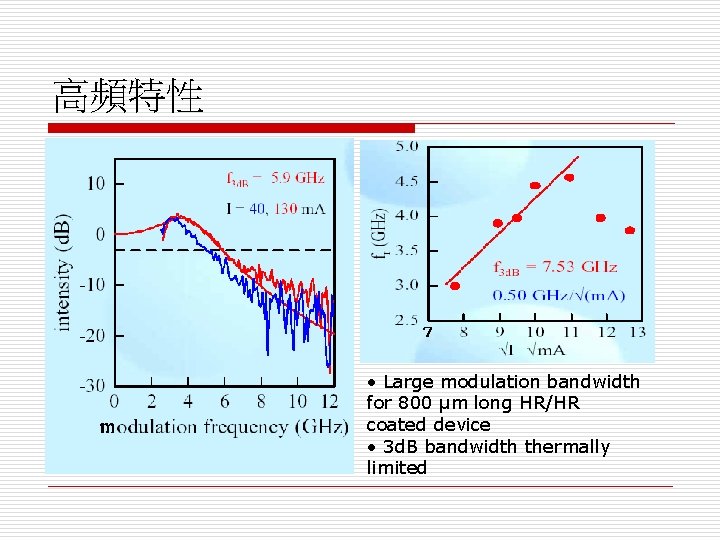
高頻特性 • Large modulation bandwidth for 800 µm long HR/HR coated device • 3 d. B bandwidth thermally limited
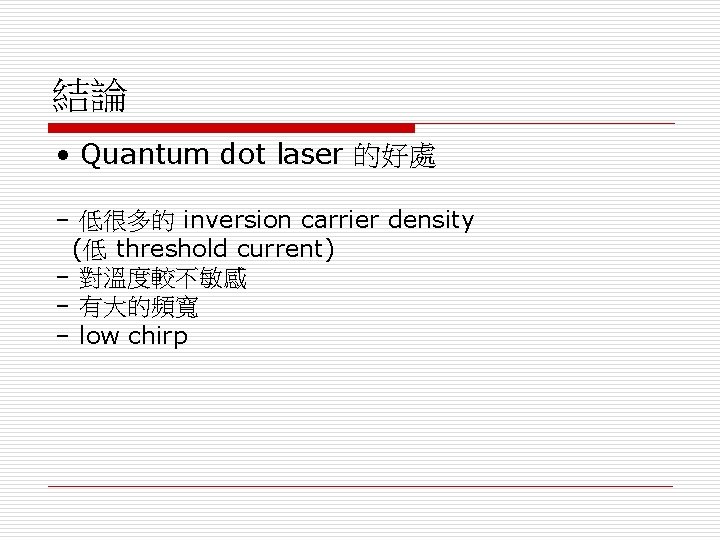
結論 • Quantum dot laser 的好處 – 低很多的 inversion carrier density (低 threshold current) – 對溫度較不敏感 – 有大的頻寬 – low chirp
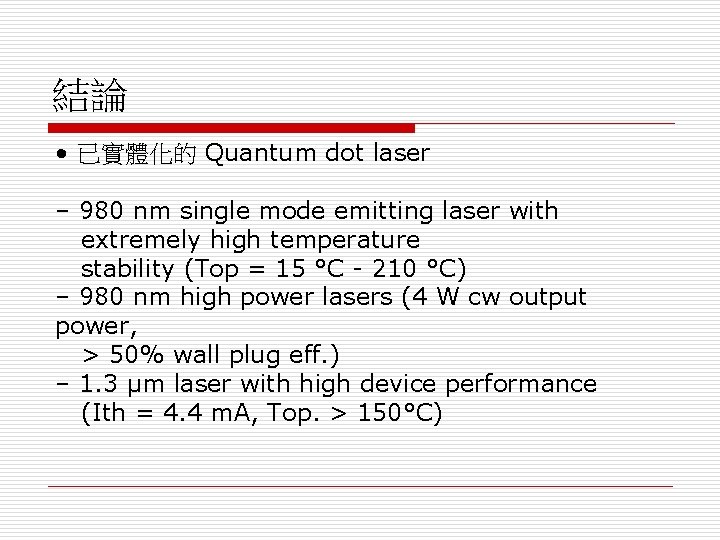
結論 • 已實體化的 Quantum dot laser – 980 nm single mode emitting laser with extremely high temperature stability (Top = 15 °C - 210 °C) – 980 nm high power lasers (4 W cw output power, > 50% wall plug eff. ) – 1. 3 µm laser with high device performance (Ith = 4. 4 m. A, Top. > 150°C)
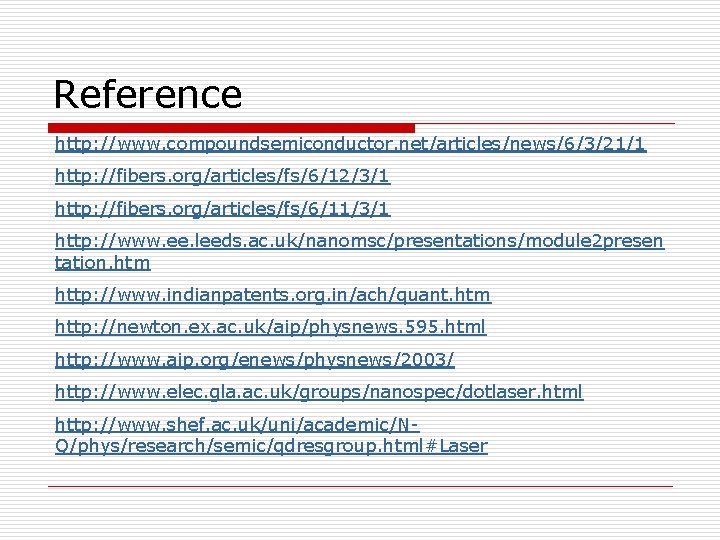
Reference http: //www. compoundsemiconductor. net/articles/news/6/3/21/1 http: //fibers. org/articles/fs/6/12/3/1 http: //fibers. org/articles/fs/6/11/3/1 http: //www. ee. leeds. ac. uk/nanomsc/presentations/module 2 presen tation. htm http: //www. indianpatents. org. in/ach/quant. htm http: //newton. ex. ac. uk/aip/physnews. 595. html http: //www. aip. org/enews/physnews/2003/ http: //www. elec. gla. ac. uk/groups/nanospec/dotlaser. html http: //www. shef. ac. uk/uni/academic/NQ/phys/research/semic/qdresgroup. html#Laser
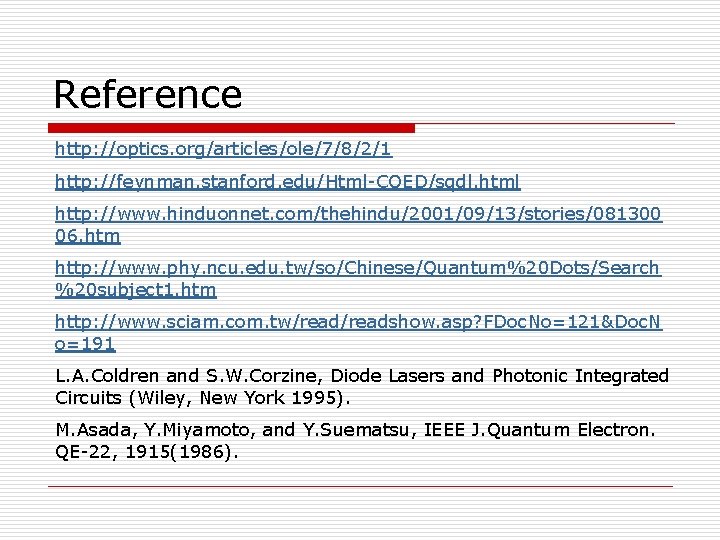
Reference http: //optics. org/articles/ole/7/8/2/1 http: //feynman. stanford. edu/Html-CQED/sqdl. html http: //www. hinduonnet. com/thehindu/2001/09/13/stories/081300 06. htm http: //www. phy. ncu. edu. tw/so/Chinese/Quantum%20 Dots/Search %20 subject 1. htm http: //www. sciam. com. tw/readshow. asp? FDoc. No=121&Doc. N o=191 L. A. Coldren and S. W. Corzine, Diode Lasers and Photonic Integrated Circuits (Wiley, New York 1995). M. Asada, Y. Miyamoto, and Y. Suematsu, IEEE J. Quantum Electron. QE-22, 1915(1986).
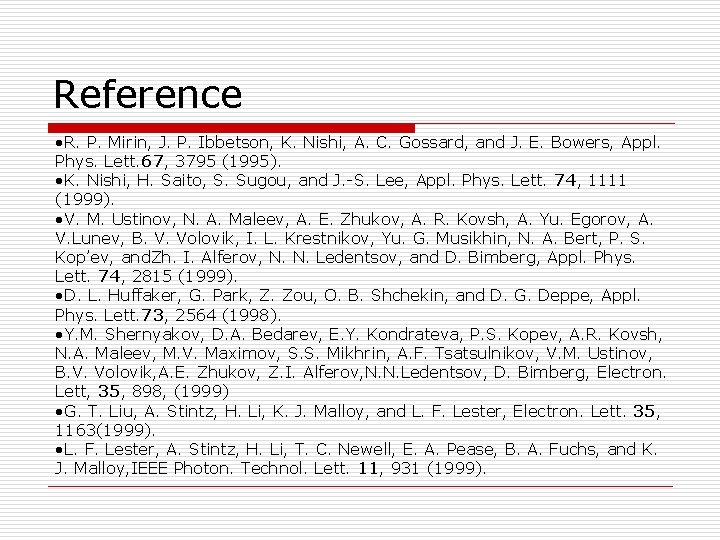
Reference • R. P. Mirin, J. P. Ibbetson, K. Nishi, A. C. Gossard, and J. E. Bowers, Appl. Phys. Lett. 67, 3795 (1995). • K. Nishi, H. Saito, S. Sugou, and J. -S. Lee, Appl. Phys. Lett. 74, 1111 (1999). • V. M. Ustinov, N. A. Maleev, A. E. Zhukov, A. R. Kovsh, A. Yu. Egorov, A. V. Lunev, B. V. Volovik, I. L. Krestnikov, Yu. G. Musikhin, N. A. Bert, P. S. Kop’ev, and. Zh. I. Alferov, N. N. Ledentsov, and D. Bimberg, Appl. Phys. Lett. 74, 2815 (1999). • D. L. Huffaker, G. Park, Z. Zou, O. B. Shchekin, and D. G. Deppe, Appl. Phys. Lett. 73, 2564 (1998). • Y. M. Shernyakov, D. A. Bedarev, E. Y. Kondrateva, P. S. Kopev, A. R. Kovsh, N. A. Maleev, M. V. Maximov, S. S. Mikhrin, A. F. Tsatsulnikov, V. M. Ustinov, B. V. Volovik, A. E. Zhukov, Z. I. Alferov, N. N. Ledentsov, D. Bimberg, Electron. Lett, 35, 898, (1999) • G. T. Liu, A. Stintz, H. Li, K. J. Malloy, and L. F. Lester, Electron. Lett. 35, 1163(1999). • L. F. Lester, A. Stintz, H. Li, T. C. Newell, E. A. Pease, B. A. Fuchs, and K. J. Malloy, IEEE Photon. Technol. Lett. 11, 931 (1999).
Nanoelectronics
Semiconductor examples
Nanocrystal flash memory device
Properties of laser light
Audience scanning lasers
Inertial confinement fusion lasers
Gingivoplasty
A storage device that uses lasers to read data
Machine vision lasers
Audience scanning laser
Audience scanning lasers
Bread sentence
Ib command terms
Ib command terms
Short term planning and long term planning
In my understanding
Long term memory vs short term memory
Term-to-term rule
Short term and long term human resource planning
Term-to-term rule
Difference between long term and short term liabilities
Long-term liabilities examples
Minterms
Nth term rule
Term to term rule
Long term goals examples
Research paper on financial planning and forecasting
Senior project outline
Capstone paper outline
Parts of investigatory project
Project presentation outline
Bsb51407
Project presentation outline
Project outline
Project proposal title ideas