Quantitative Modelling of the Shifts and Splitting in
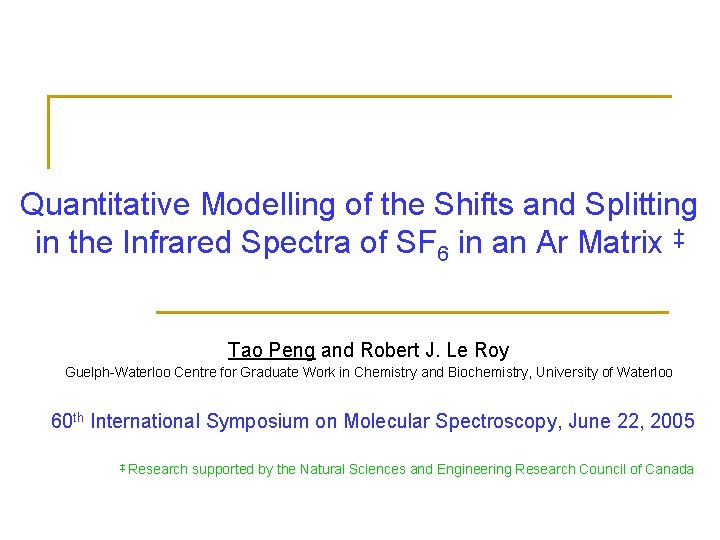
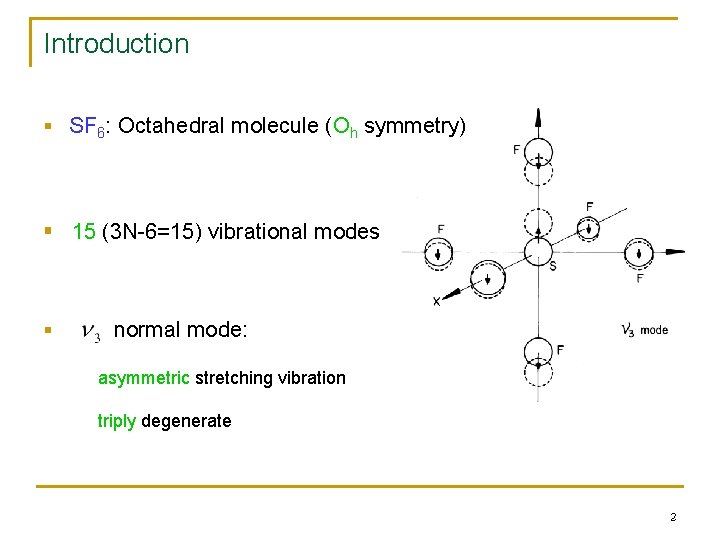
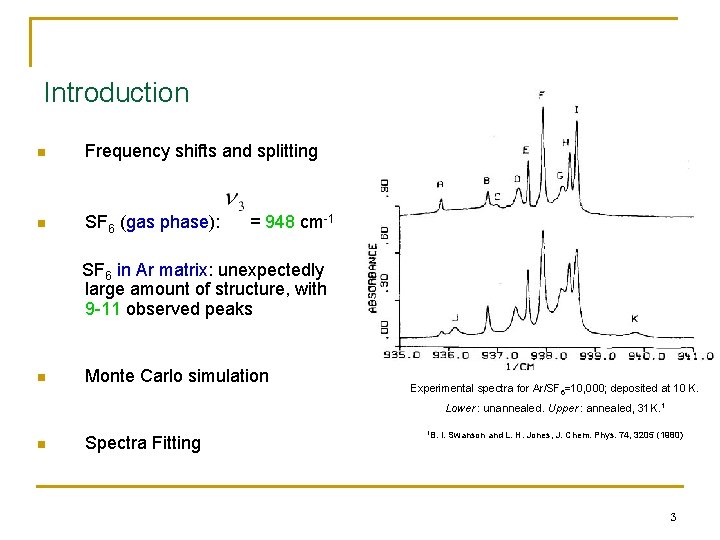
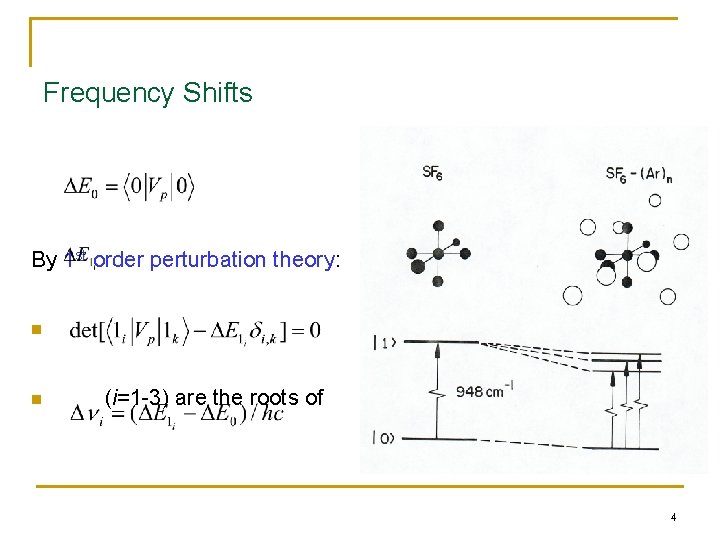
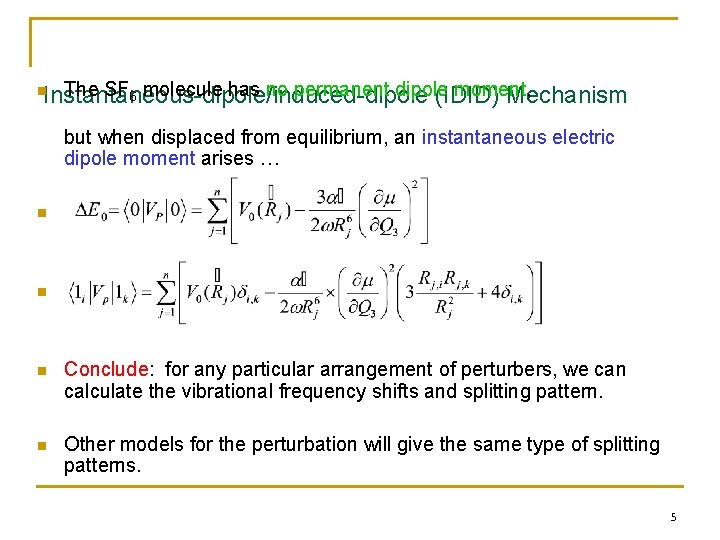
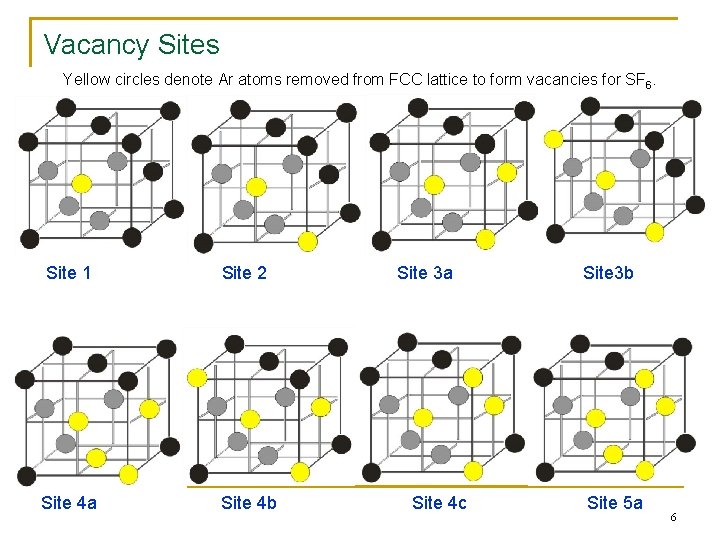
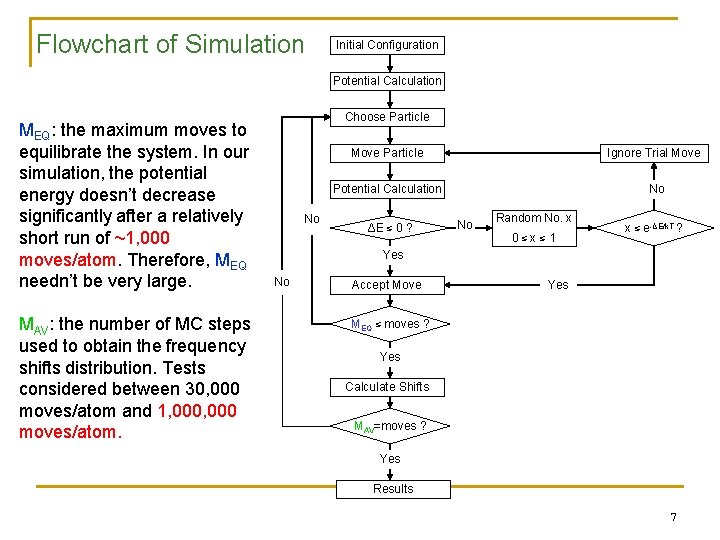
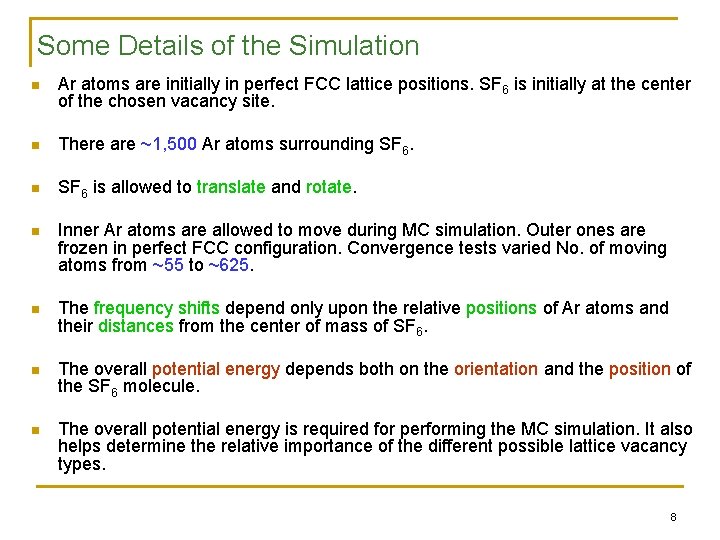
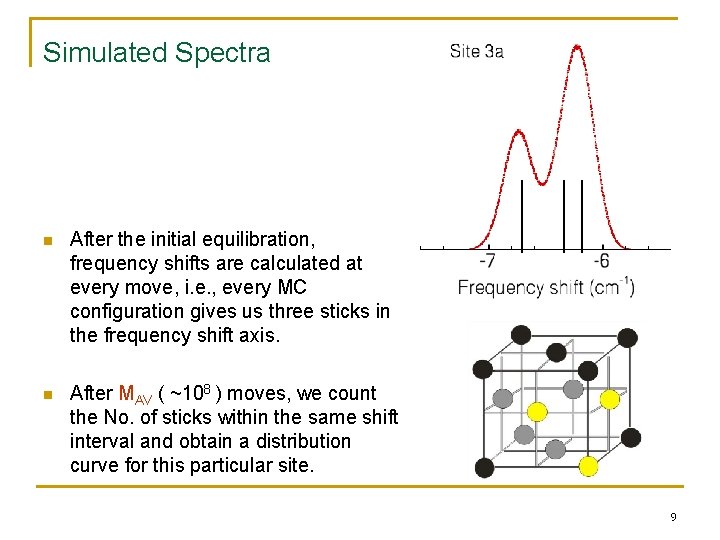
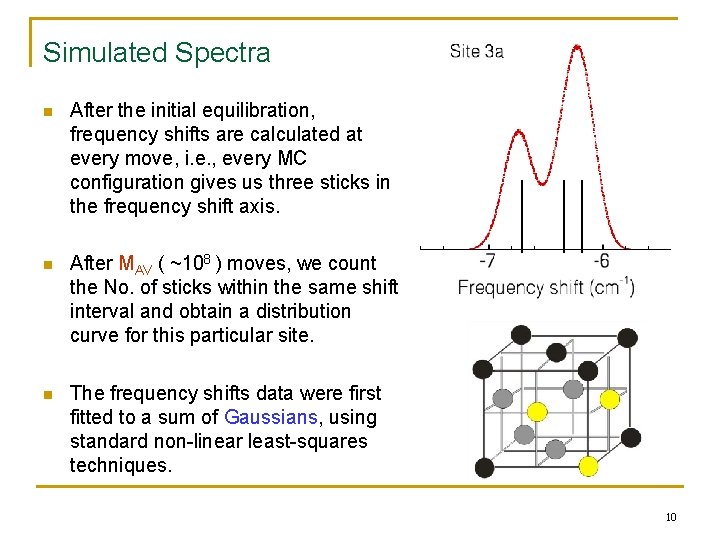
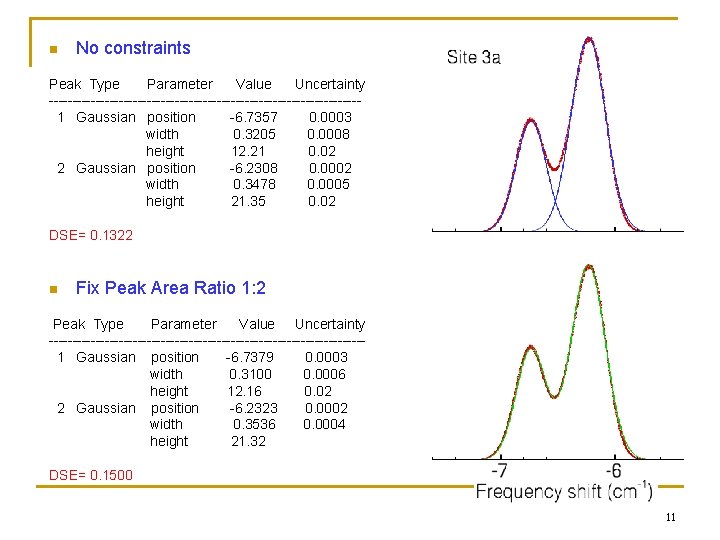
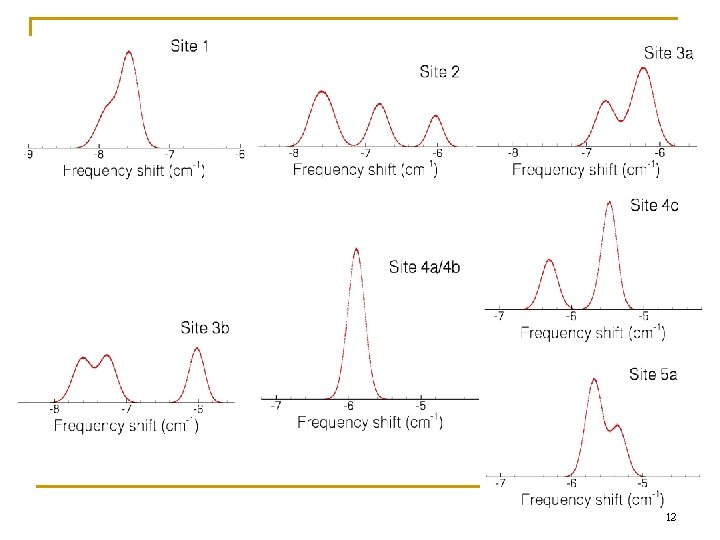
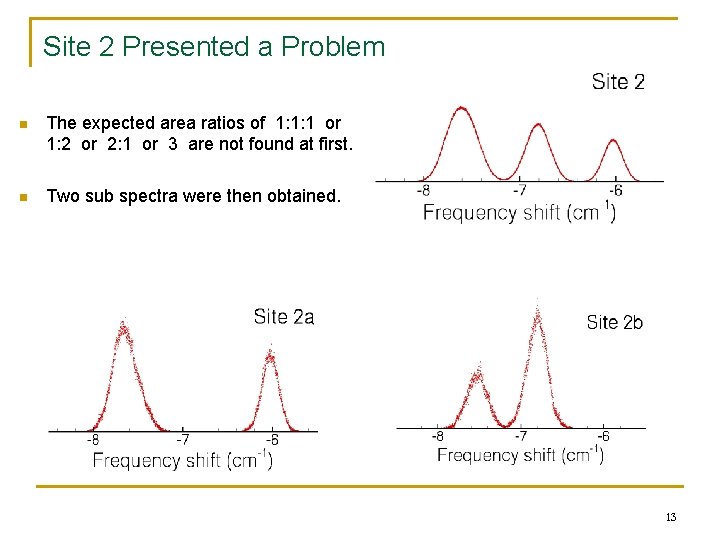
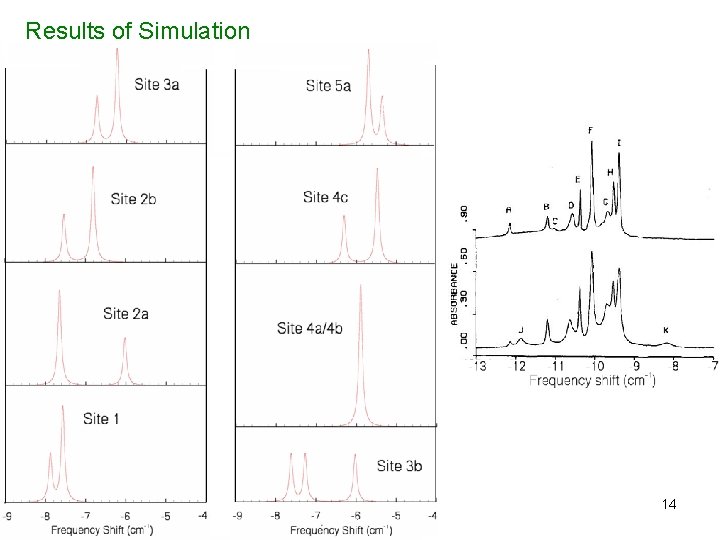
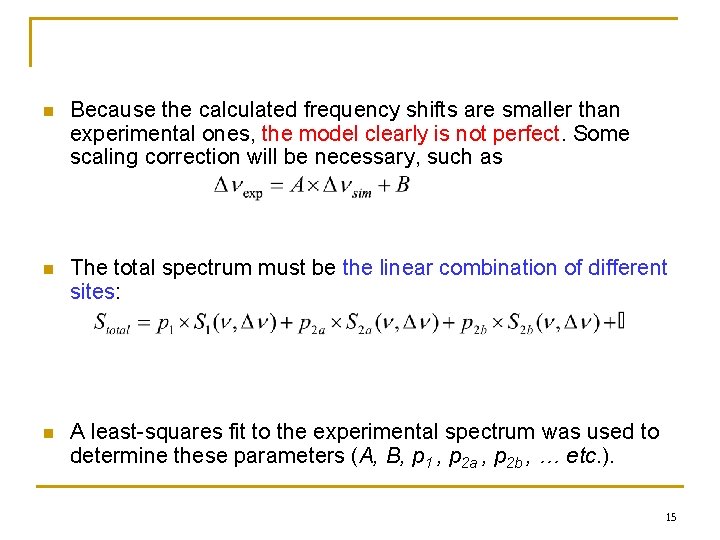
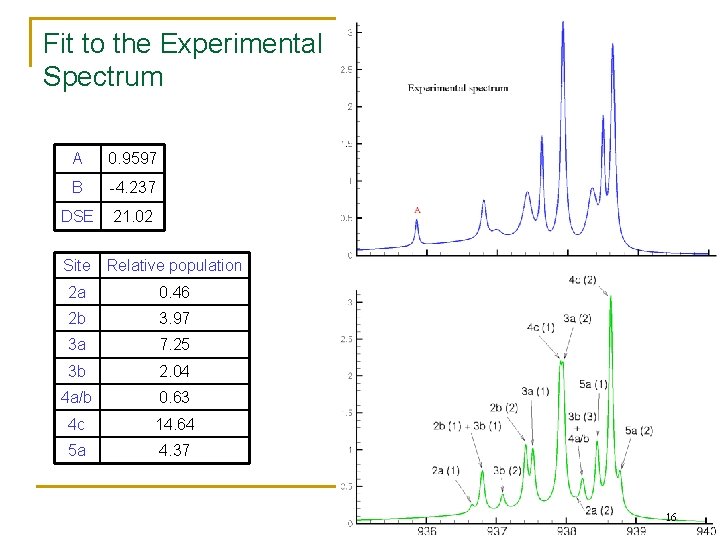
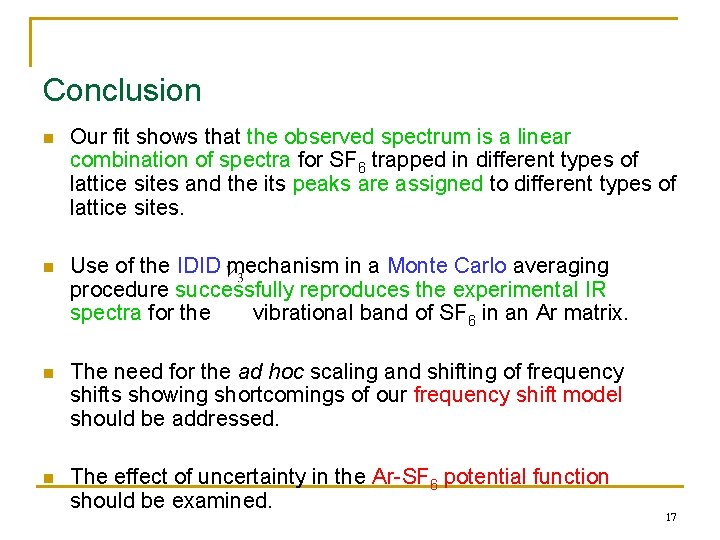
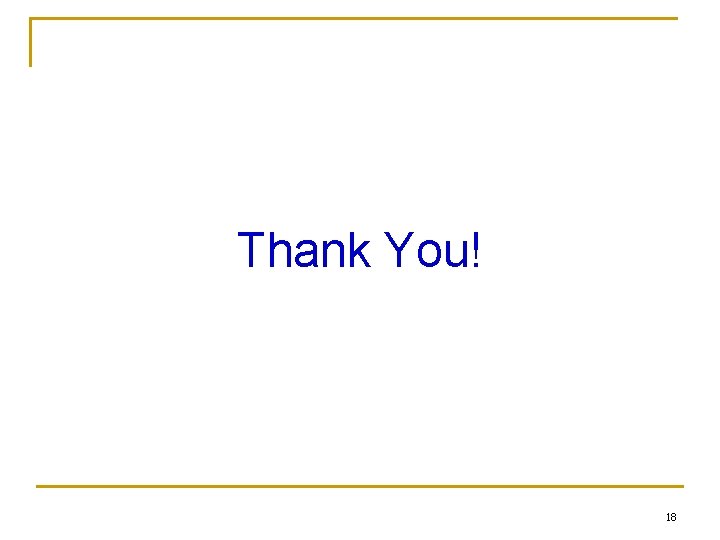
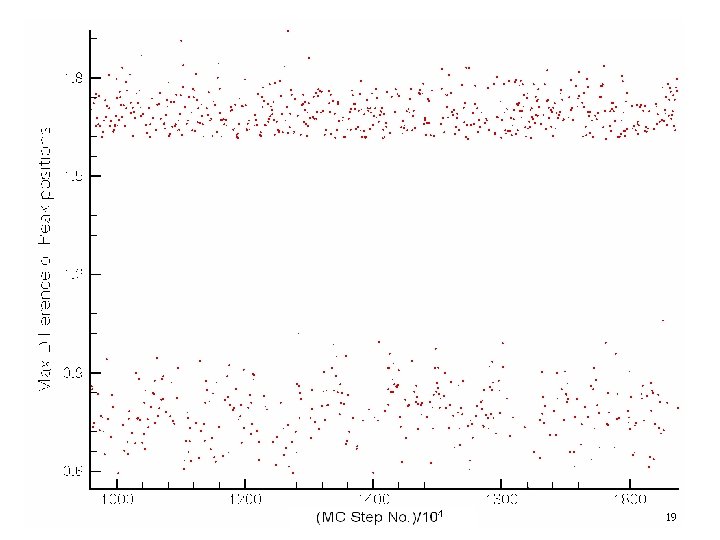
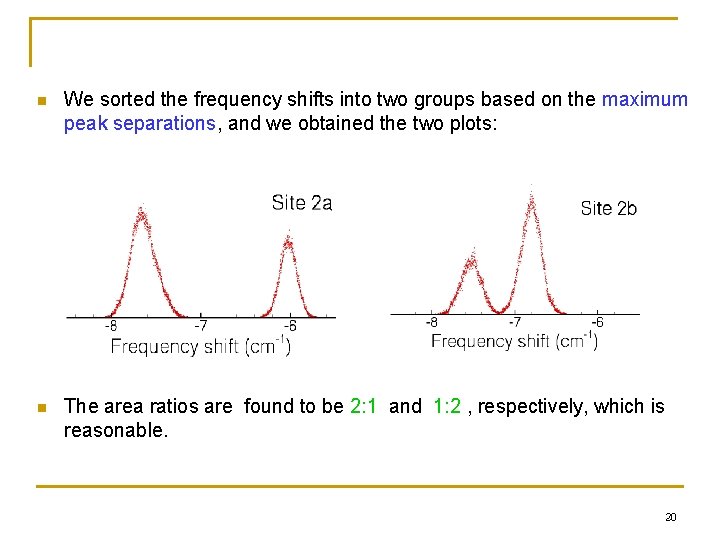
- Slides: 20
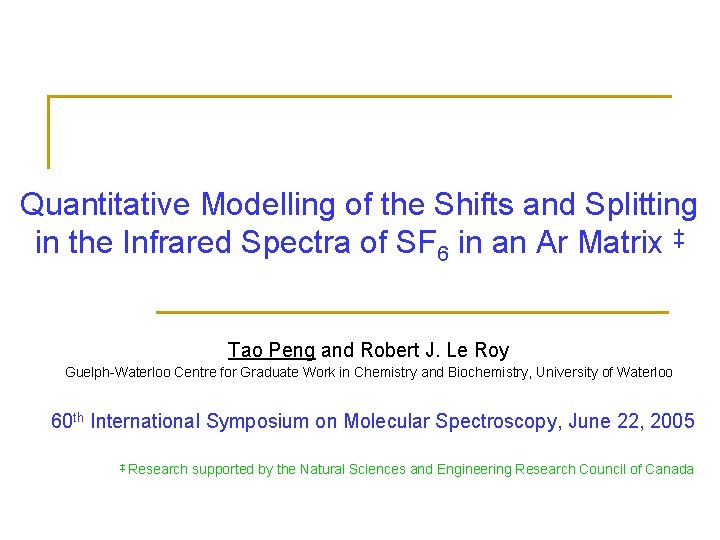
Quantitative Modelling of the Shifts and Splitting in the Infrared Spectra of SF 6 in an Ar Matrix ‡ Tao Peng and Robert J. Le Roy Guelph-Waterloo Centre for Graduate Work in Chemistry and Biochemistry, University of Waterloo 60 th International Symposium on Molecular Spectroscopy, June 22, 2005 ‡ Research supported by the Natural Sciences and Engineering Research Council of Canada
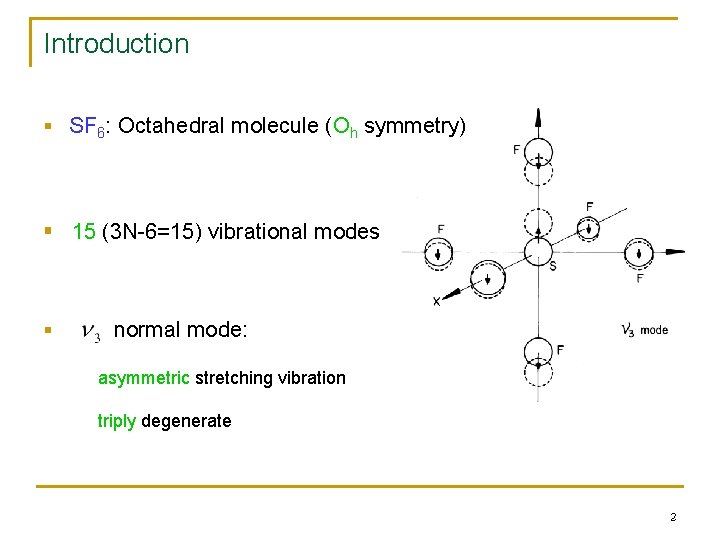
Introduction § SF 6: Octahedral molecule (Oh symmetry) § 15 (3 N-6=15) vibrational modes § normal mode: asymmetric stretching vibration triply degenerate 2
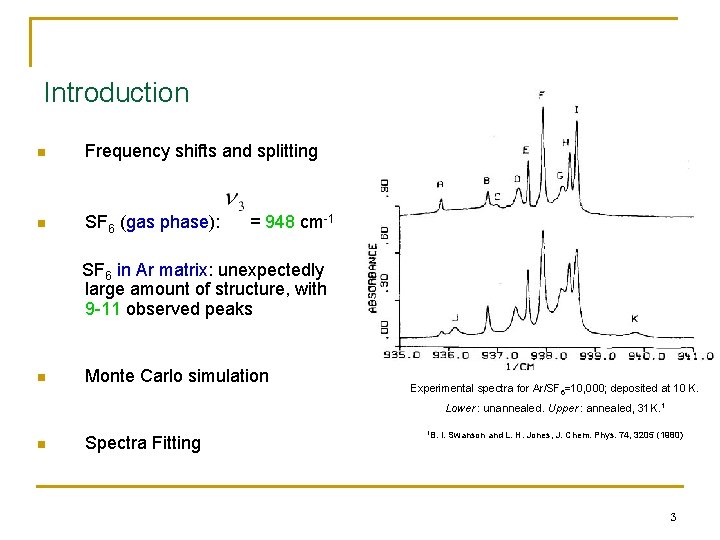
Introduction n Frequency shifts and splitting n SF 6 (gas phase): = 948 cm-1 SF 6 in Ar matrix: unexpectedly large amount of structure, with 9 -11 observed peaks n Monte Carlo simulation Experimental spectra for Ar/SF 6=10, 000; deposited at 10 K. Lower : unannealed. Upper : annealed, 31 K. 1 n Spectra Fitting 1 B. I. Swanson and L. H. Jones, J. Chem. Phys. 74, 3205 (1980) 3
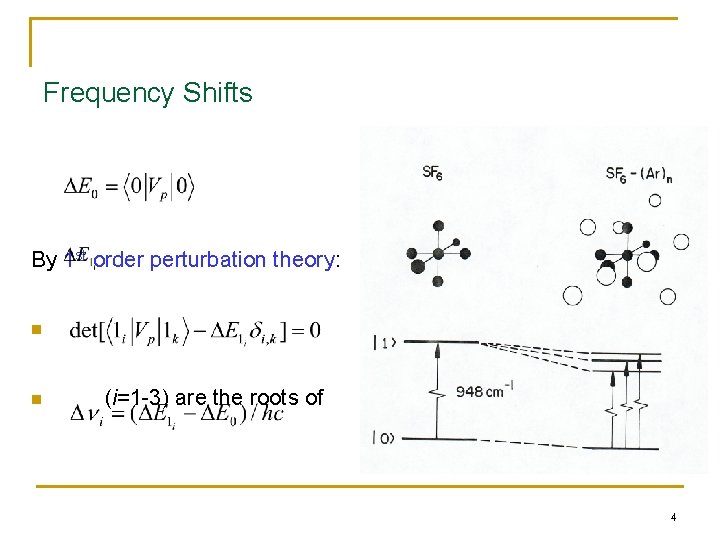
Frequency Shifts By 1 st order perturbation theory: n n (i=1 -3) are the roots of 4
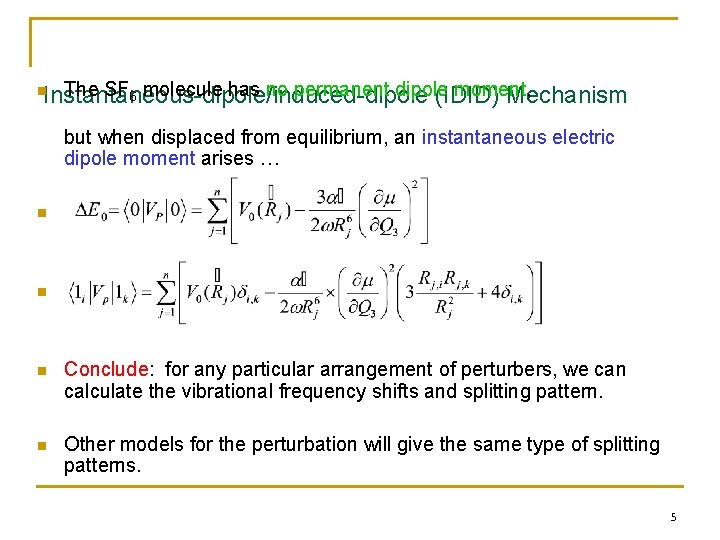
The SF 6 molecule has no permanent dipole moment, Instantaneous-dipole/induced-dipole (IDID) Mechanism n but when displaced from equilibrium, an instantaneous electric dipole moment arises … n n n Conclude: for any particular arrangement of perturbers, we can calculate the vibrational frequency shifts and splitting pattern. n Other models for the perturbation will give the same type of splitting patterns. 5
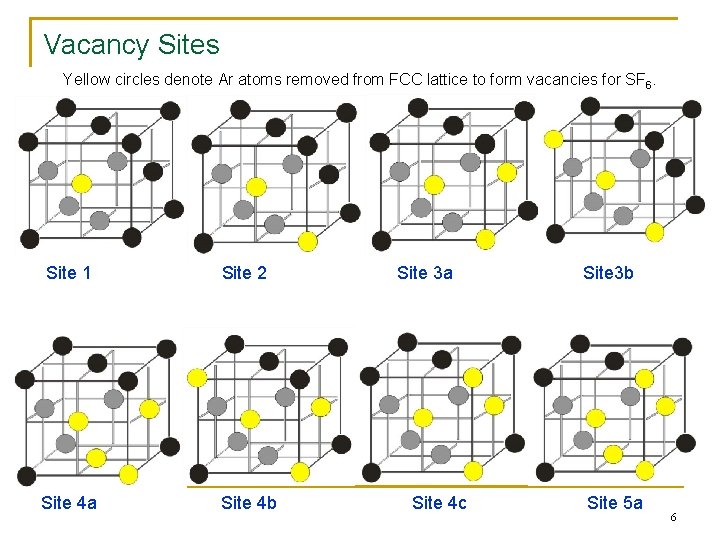
Vacancy Sites Yellow circles denote Ar atoms removed from FCC lattice to form vacancies for SF 6. Site 1 Site 2 Site 4 a Site 4 b Site 3 a Site 4 c Site 3 b Site 5 a 6
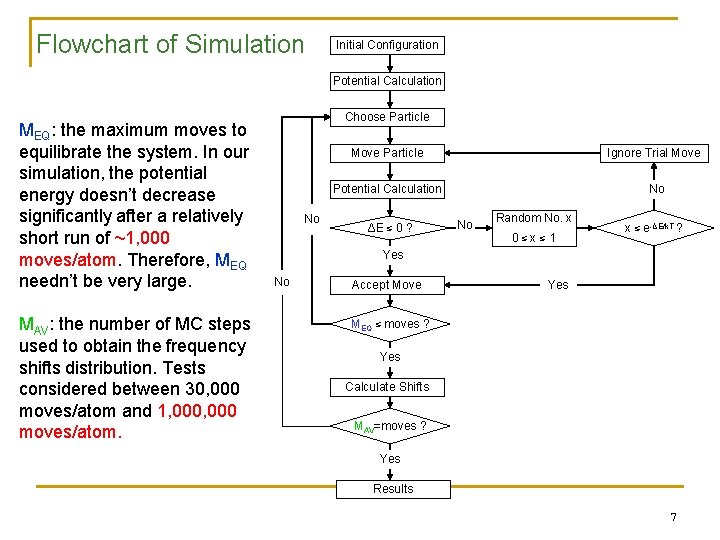
Flowchart of Simulation Initial Configuration Potential Calculation MEQ: the maximum moves to equilibrate the system. In our simulation, the potential energy doesn’t decrease significantly after a relatively short run of ~1, 000 moves/atom. Therefore, MEQ needn’t be very large. MAV: the number of MC steps used to obtain the frequency shifts distribution. Tests considered between 30, 000 moves/atom and 1, 000 moves/atom. Choose Particle No Move Particle Ignore Trial Move Potential Calculation No ΔE ≤ 0 ? No Random No. x 0≤x≤ 1 x ≤ e-ΔE/k. T ? Yes No Accept Move Yes MEQ ≤ moves ? Yes Calculate Shifts MAV=moves ? Yes Results 7
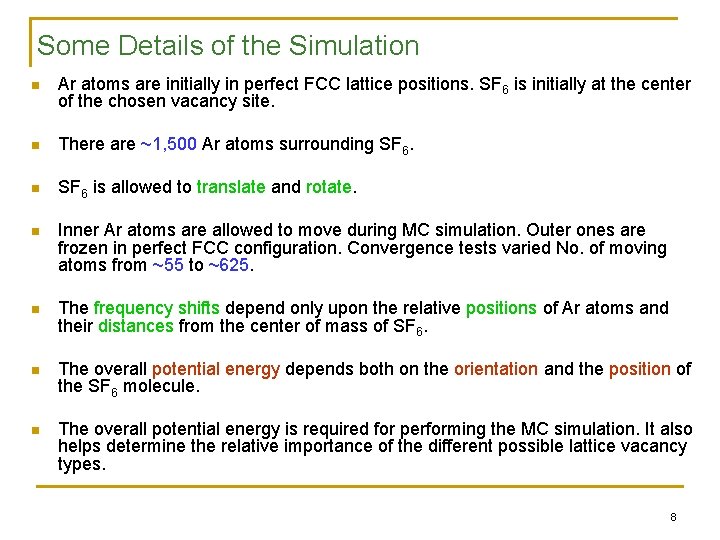
Some Details of the Simulation n Ar atoms are initially in perfect FCC lattice positions. SF 6 is initially at the center of the chosen vacancy site. n There are ~1, 500 Ar atoms surrounding SF 6. n SF 6 is allowed to translate and rotate. n Inner Ar atoms are allowed to move during MC simulation. Outer ones are frozen in perfect FCC configuration. Convergence tests varied No. of moving atoms from ~55 to ~625. n The frequency shifts depend only upon the relative positions of Ar atoms and their distances from the center of mass of SF 6. n The overall potential energy depends both on the orientation and the position of the SF 6 molecule. n The overall potential energy is required for performing the MC simulation. It also helps determine the relative importance of the different possible lattice vacancy types. 8
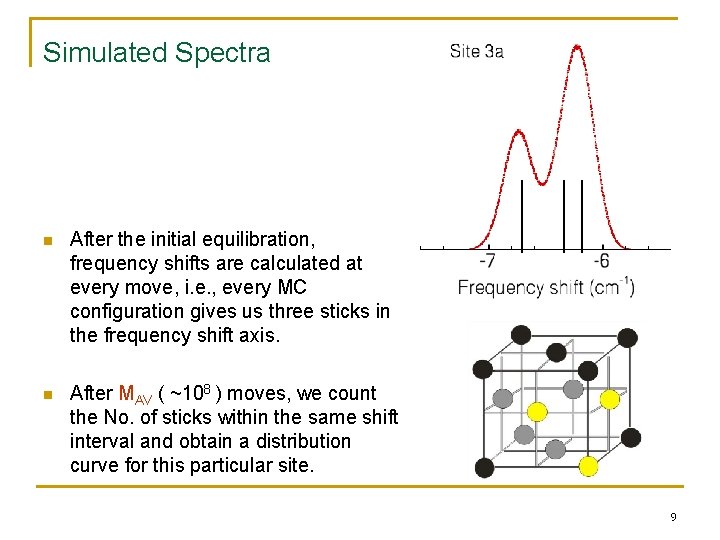
Simulated Spectra n After the initial equilibration, frequency shifts are calculated at every move, i. e. , every MC configuration gives us three sticks in the frequency shift axis. n After MAV ( ~108 ) moves, we count the No. of sticks within the same shift interval and obtain a distribution curve for this particular site. 9
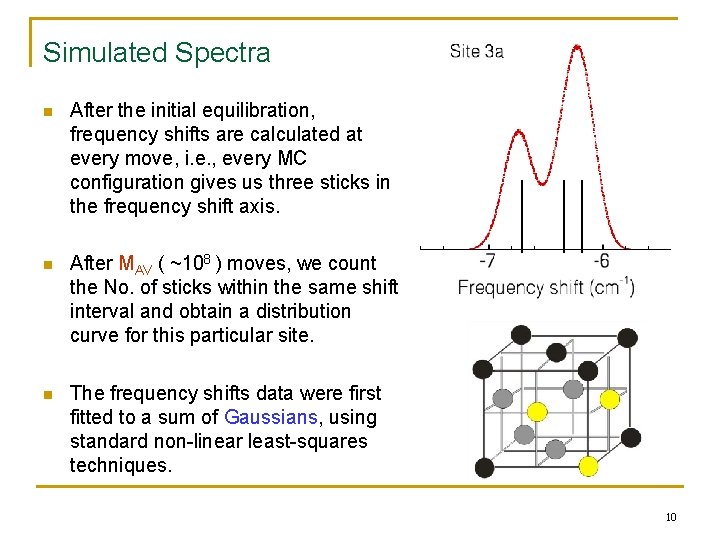
Simulated Spectra n After the initial equilibration, frequency shifts are calculated at every move, i. e. , every MC configuration gives us three sticks in the frequency shift axis. n After MAV ( ~108 ) moves, we count the No. of sticks within the same shift interval and obtain a distribution curve for this particular site. n The frequency shifts data were first fitted to a sum of Gaussians, using standard non-linear least-squares techniques. 10
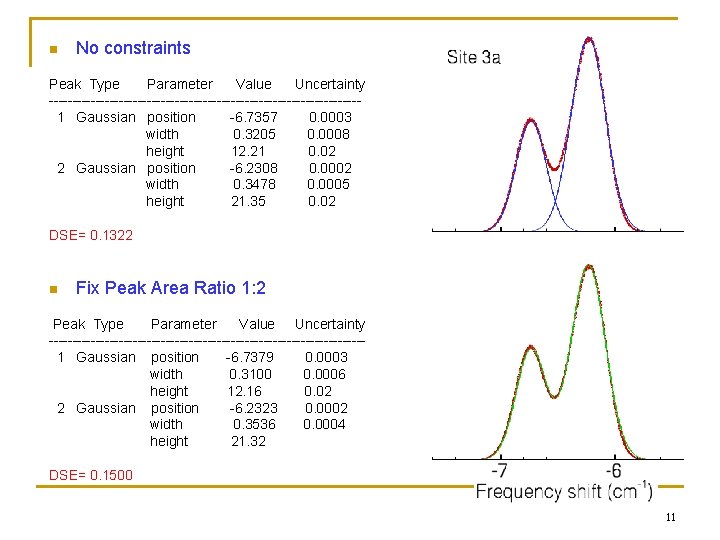
n No constraints Peak Type Parameter Value Uncertainty ---------------------------------1 Gaussian position -6. 7357 0. 0003 width 0. 3205 0. 0008 height 12. 21 0. 02 2 Gaussian position -6. 2308 0. 0002 width 0. 3478 0. 0005 height 21. 35 0. 02 DSE= 0. 1322 n Fix Peak Area Ratio 1: 2 Peak Type Parameter Value Uncertainty ----------------------------------1 Gaussian position -6. 7379 0. 0003 width 0. 3100 0. 0006 height 12. 16 0. 02 2 Gaussian position -6. 2323 0. 0002 width 0. 3536 0. 0004 height 21. 32 DSE= 0. 1500 11
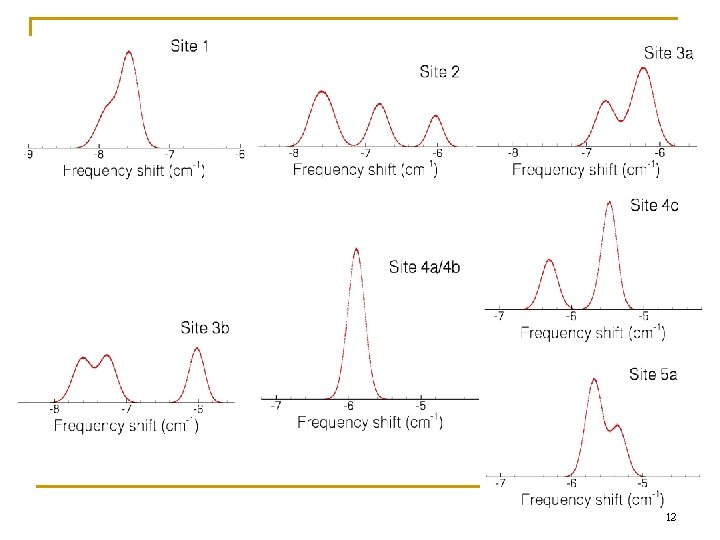
12
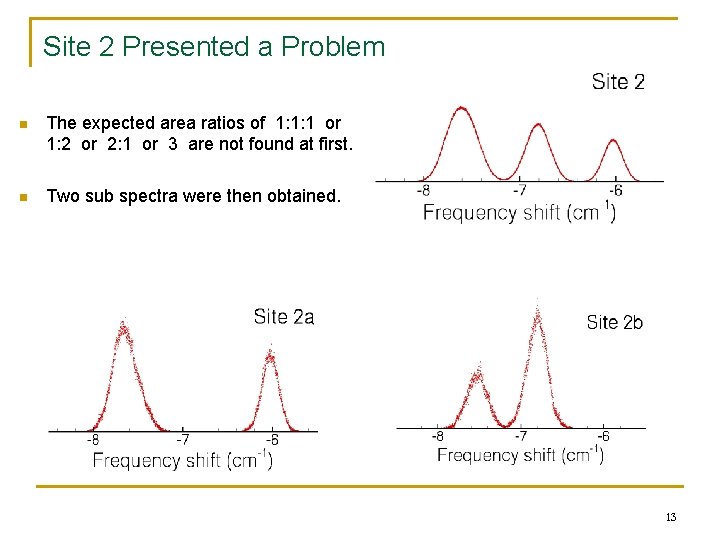
Site 2 Presented a Problem n The expected area ratios of 1: 1: 1 or 1: 2 or 2: 1 or 3 are not found at first. n Two sub spectra were then obtained. 13
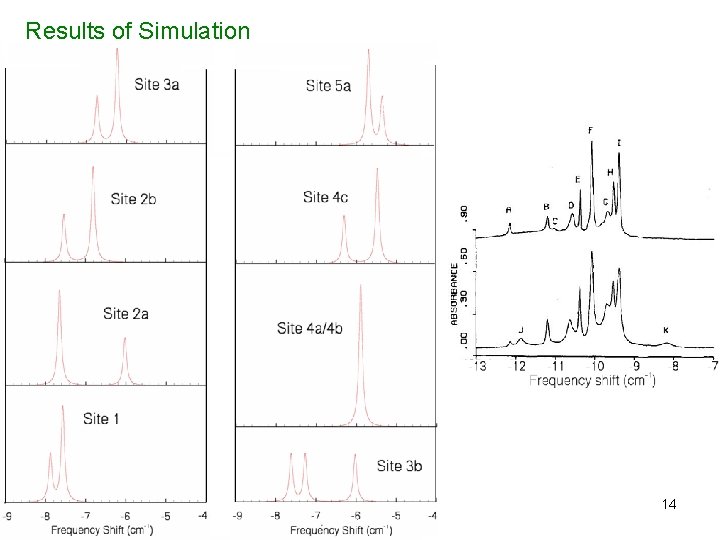
Results of Simulation 14
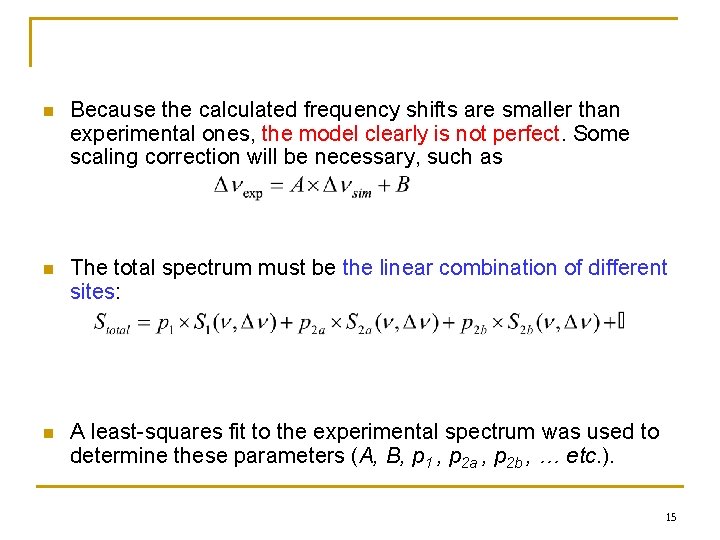
n Because the calculated frequency shifts are smaller than experimental ones, the model clearly is not perfect. Some scaling correction will be necessary, such as n The total spectrum must be the linear combination of different sites: n A least-squares fit to the experimental spectrum was used to determine these parameters (A, B, p 1 , p 2 a , p 2 b , … etc. ). 15
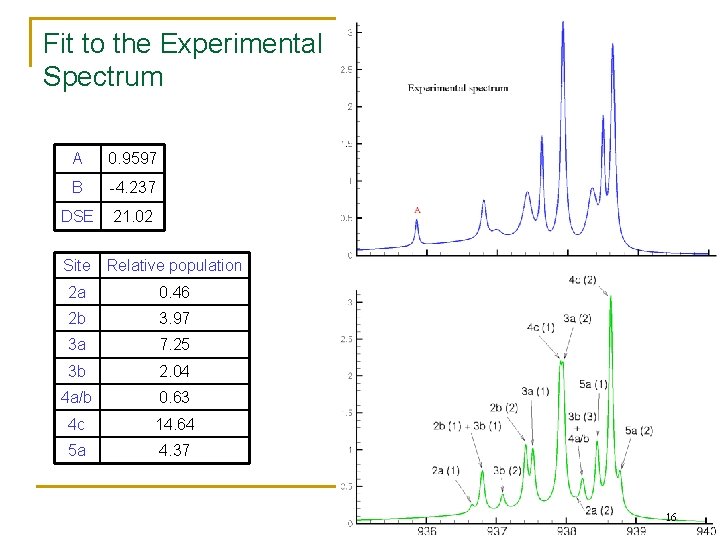
Fit to the Experimental Spectrum A 0. 9597 B -4. 237 DSE 21. 02 Site Relative population 2 a 0. 46 2 b 3. 97 3 a 7. 25 3 b 2. 04 4 a/b 0. 63 4 c 14. 64 5 a 4. 37 16
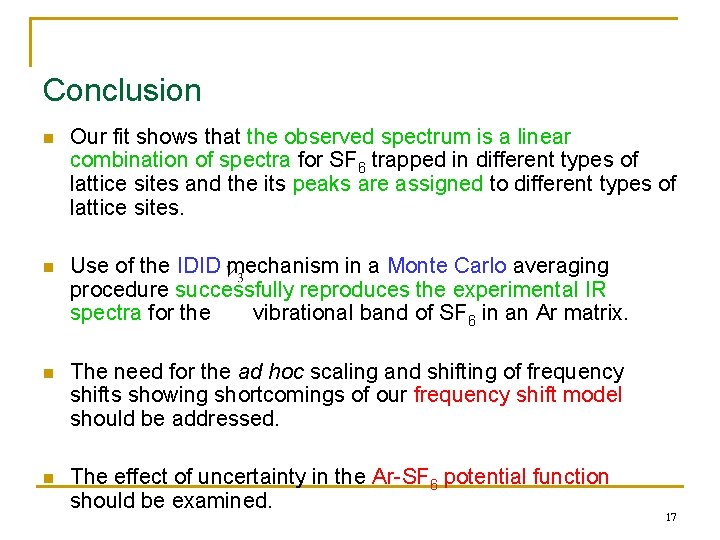
Conclusion n Our fit shows that the observed spectrum is a linear combination of spectra for SF 6 trapped in different types of lattice sites and the its peaks are assigned to different types of lattice sites. n Use of the IDID mechanism in a Monte Carlo averaging procedure successfully reproduces the experimental IR spectra for the vibrational band of SF 6 in an Ar matrix. n The need for the ad hoc scaling and shifting of frequency shifts showing shortcomings of our frequency shift model should be addressed. n The effect of uncertainty in the Ar-SF 6 potential function should be examined. 17
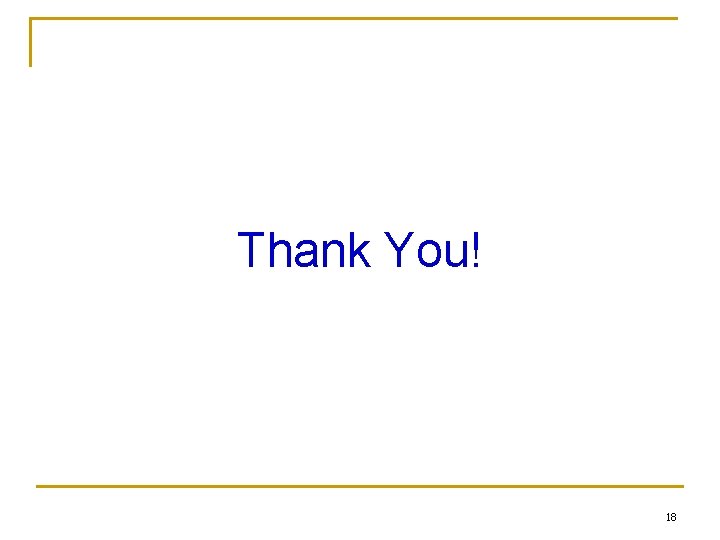
Thank You! 18
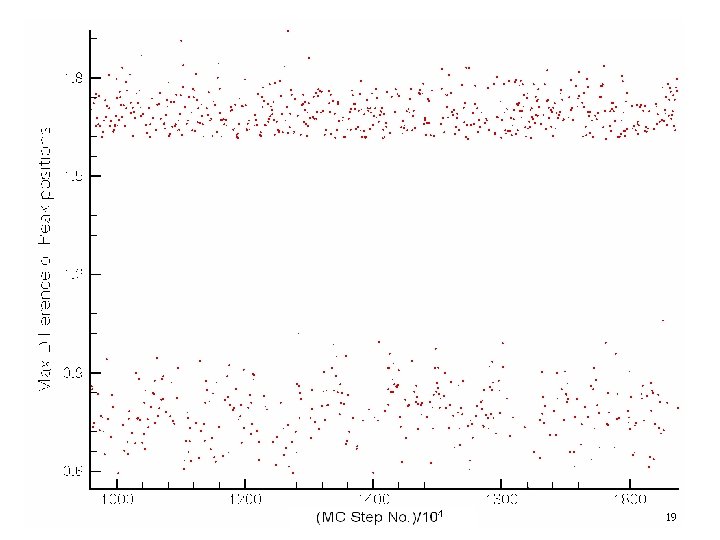
19
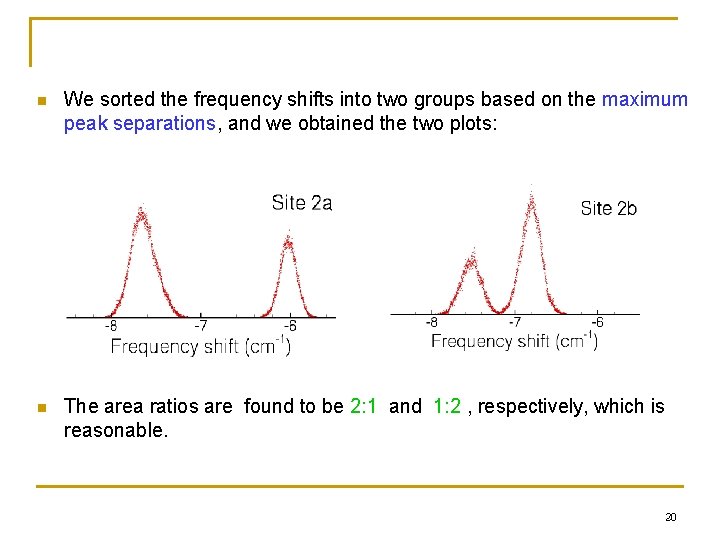
n We sorted the frequency shifts into two groups based on the maximum peak separations, and we obtained the two plots: n The area ratios are found to be 2: 1 and 1: 2 , respectively, which is reasonable. 20
Horizontal and vertical shifts
Vehicle balance is obtained by:
Change in supply and change in quantity supplied
Double shifts in demand and supply
Bpd splitting
Trauma bond
Application of crystal field theory
Pulmonic component of second heart sound
Wide fixed split s2 in asd
What are the ego defense mechanisms
Examples of passive-aggressive
Dbt accepts
Pole splitting miller compensation
Http response splitting example
Splitting heart sounds
Mica splitting test room
Autistic fantasy defense mechanism example
Crystal field theory for tetrahedral complexes
Crystal field theory
Splitting of square planar complex
Clean edge razor