Pulsed Proton Linac Presented at Proton Driver Efficiency
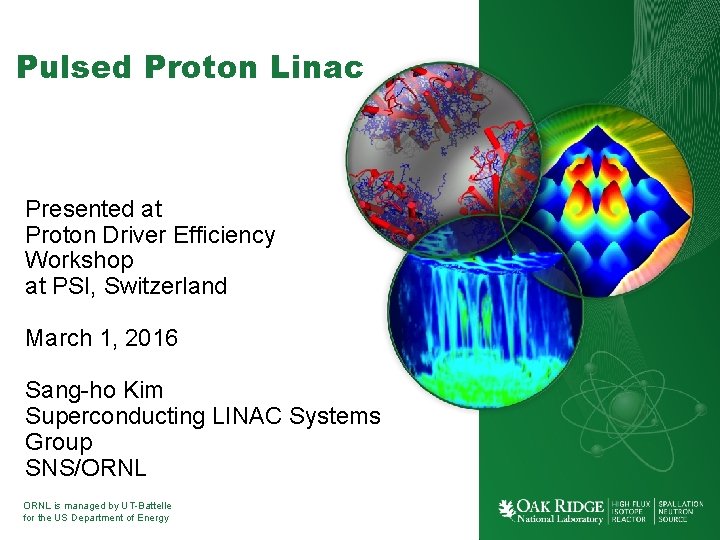
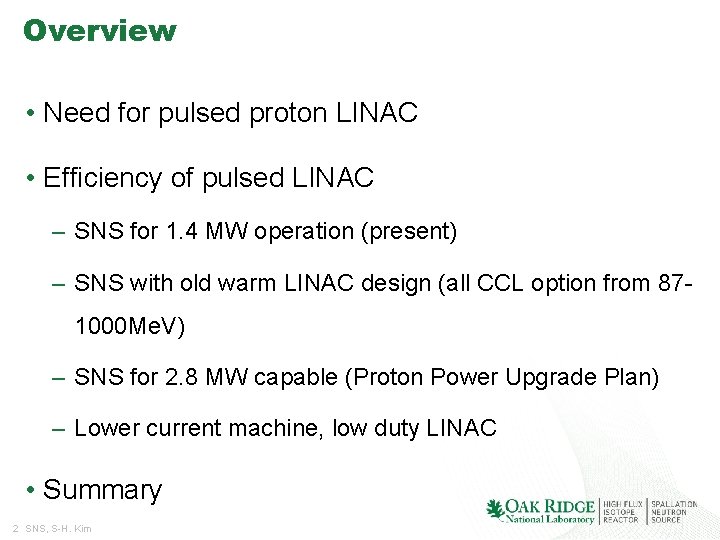
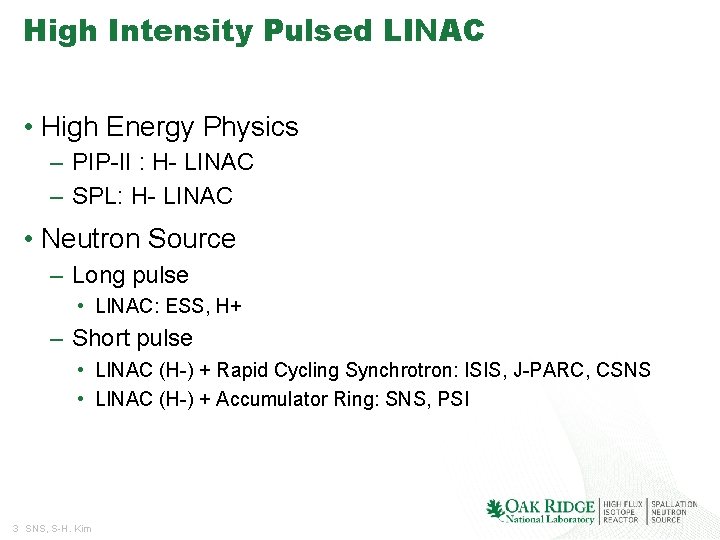
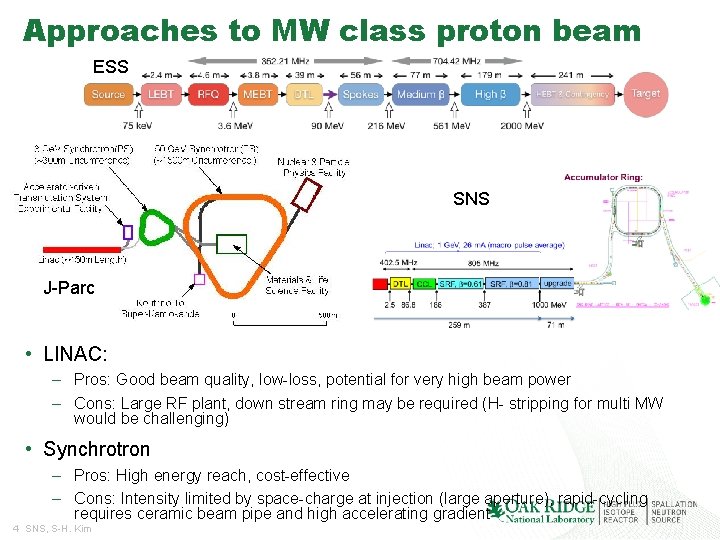
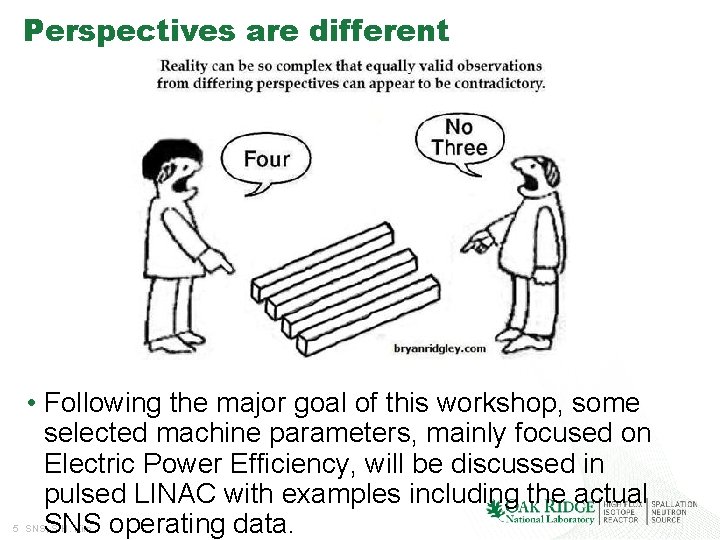
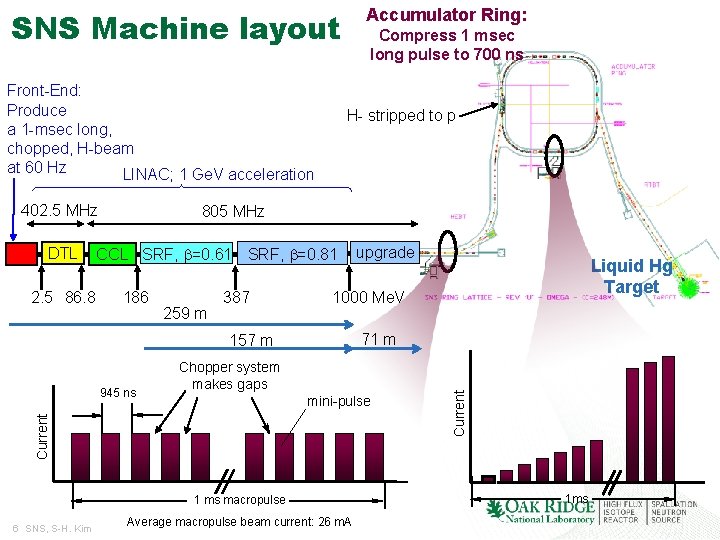
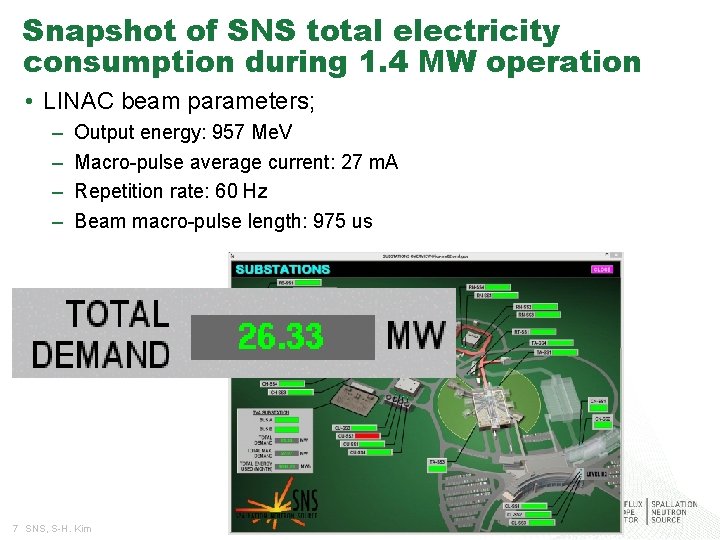
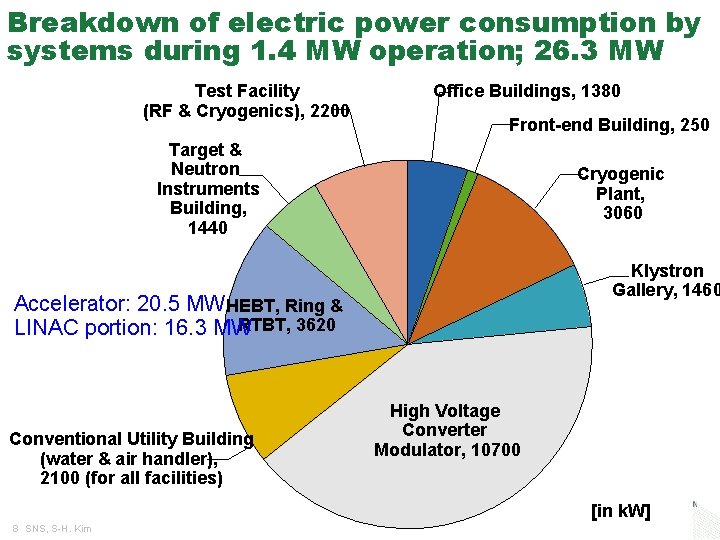
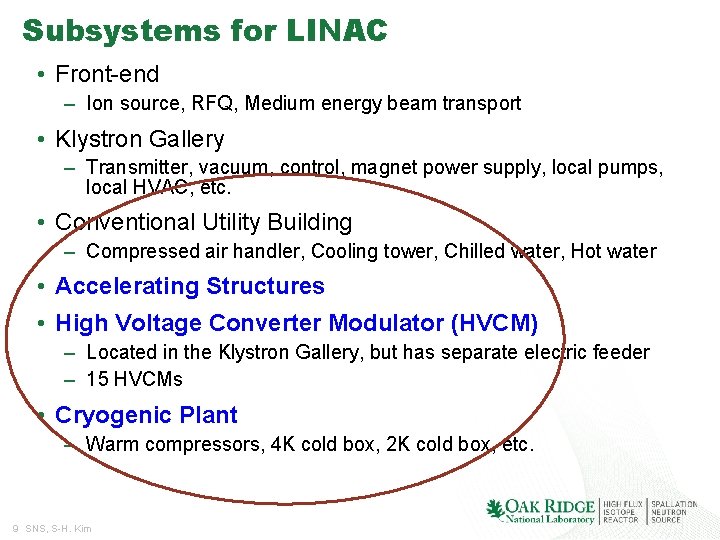
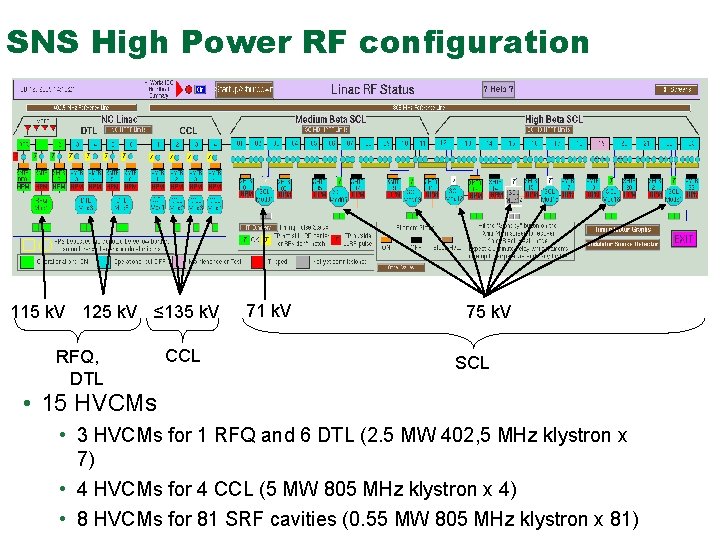
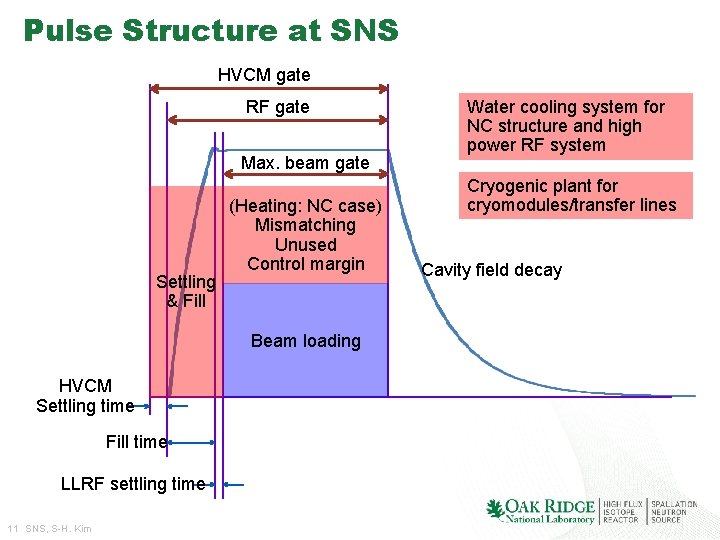
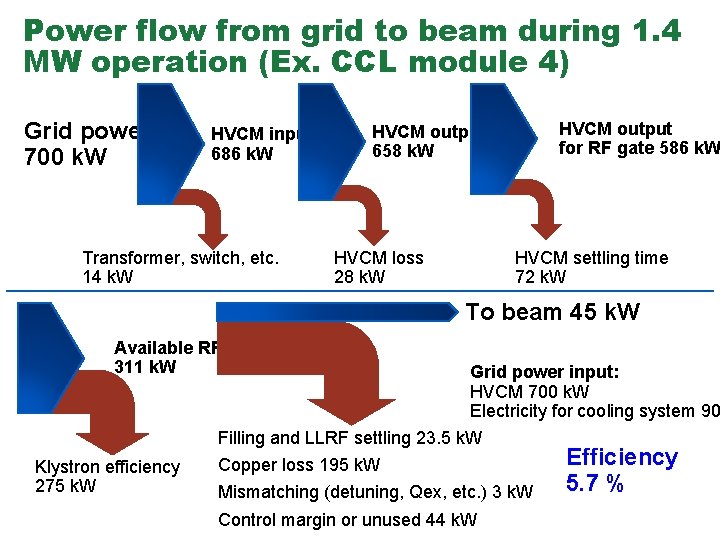
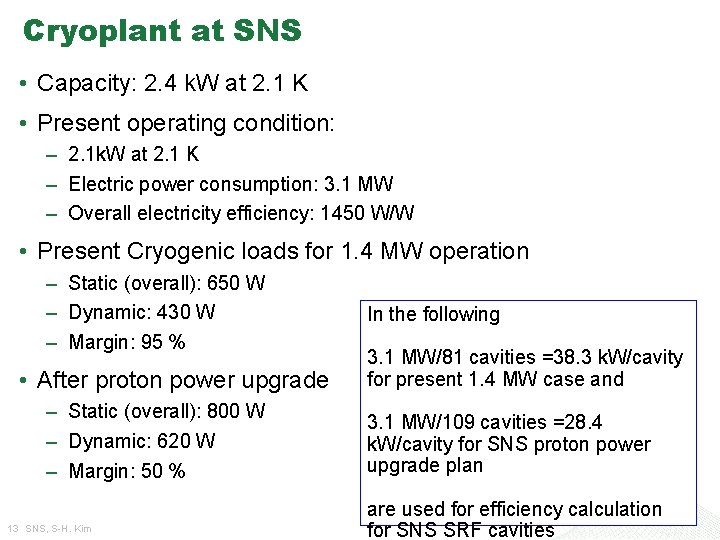
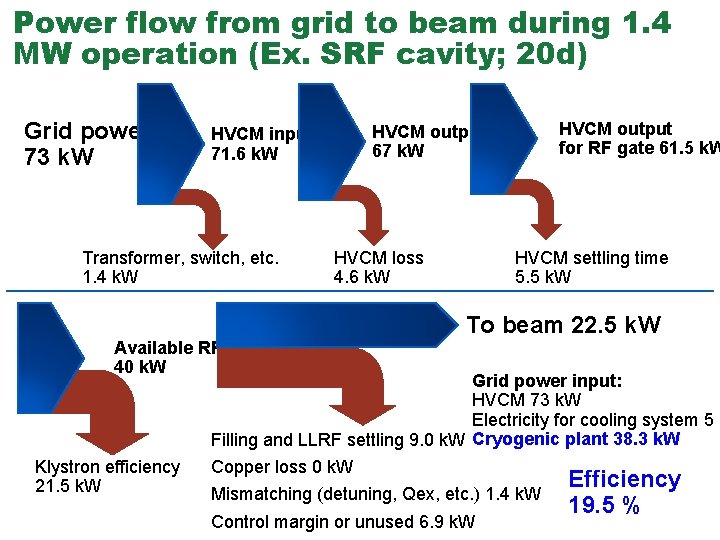
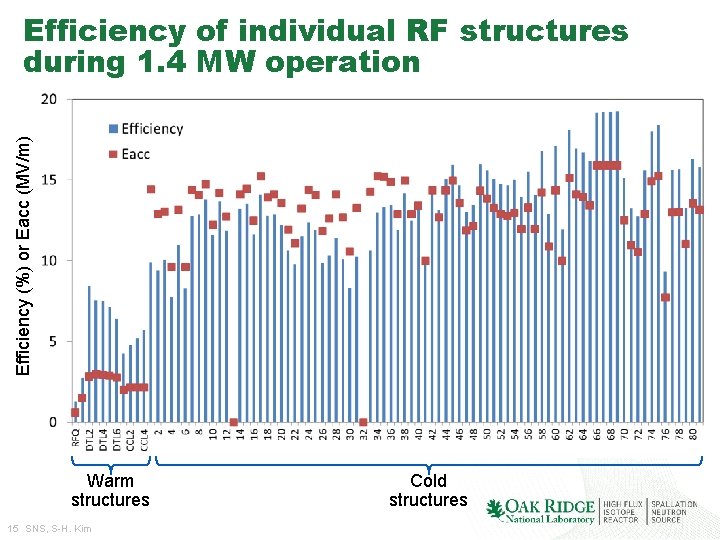
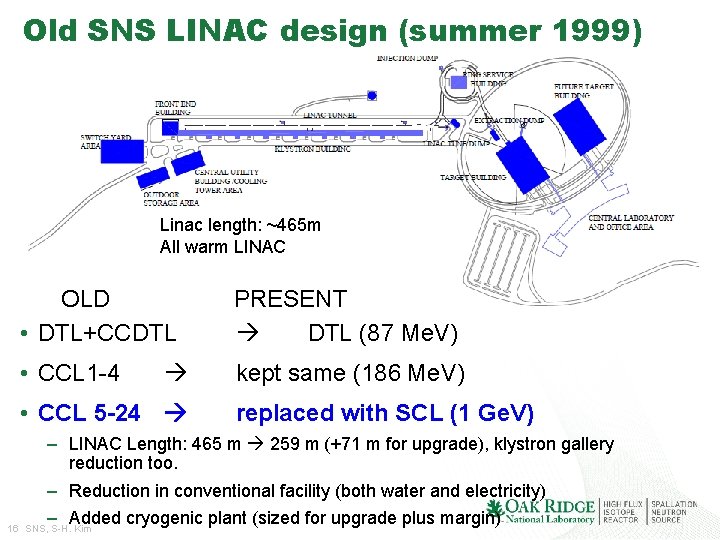
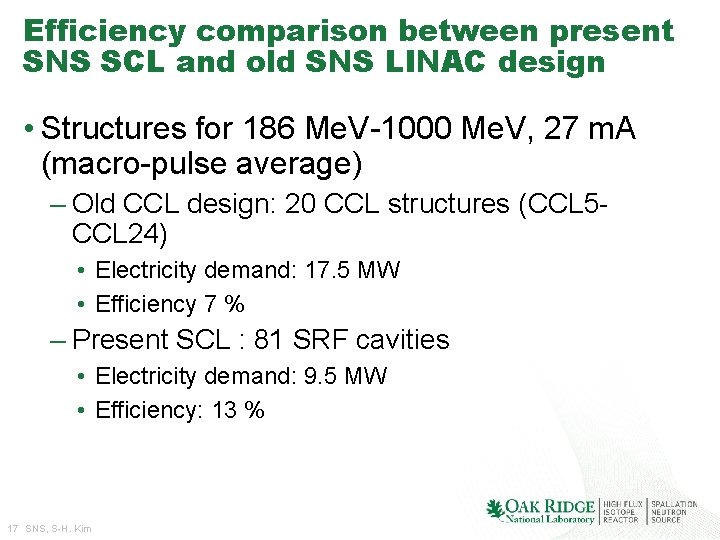
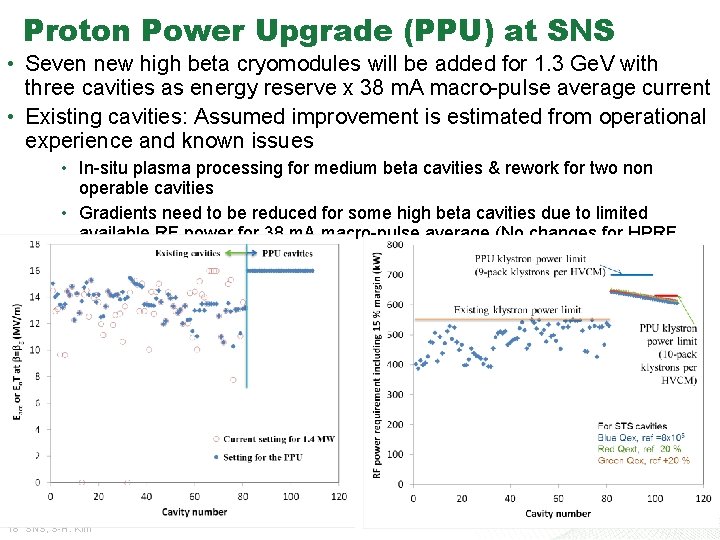
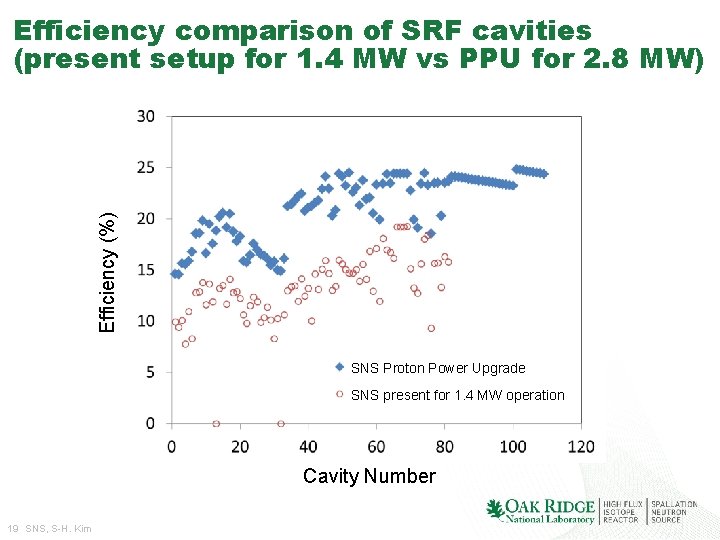
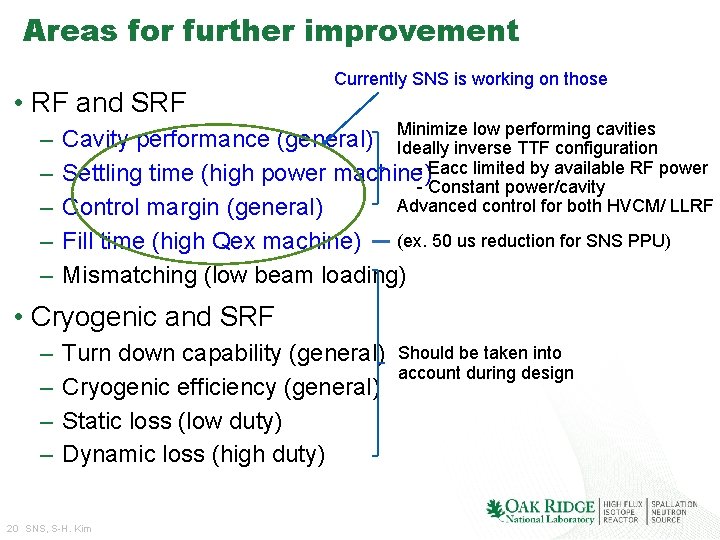
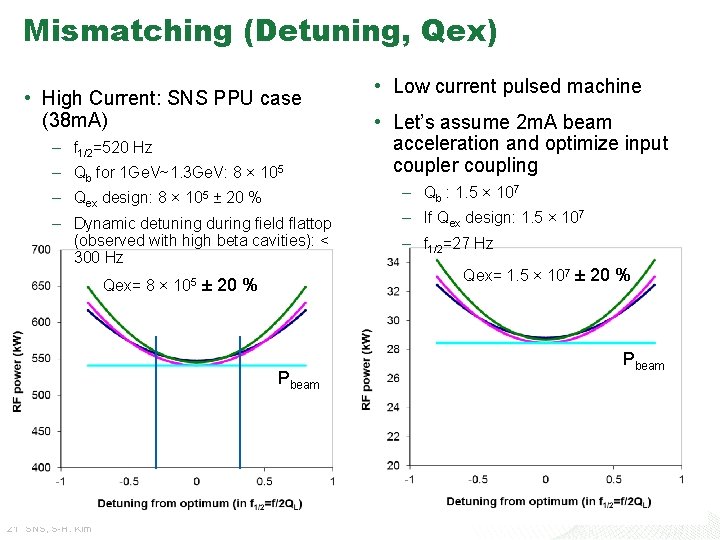
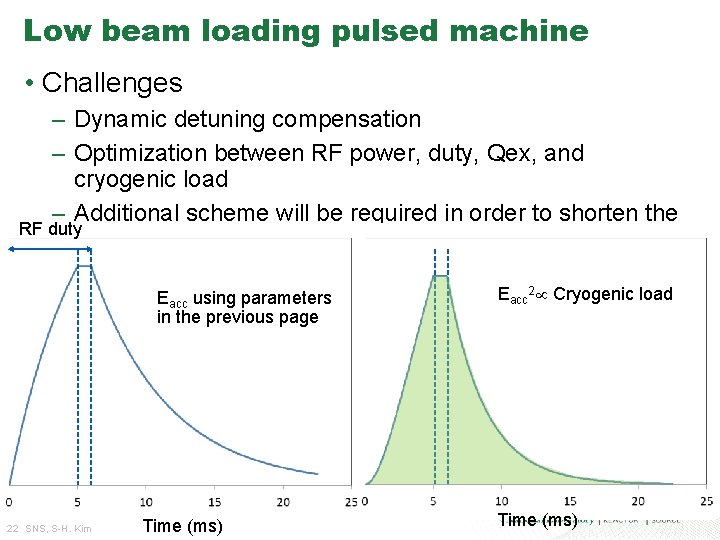
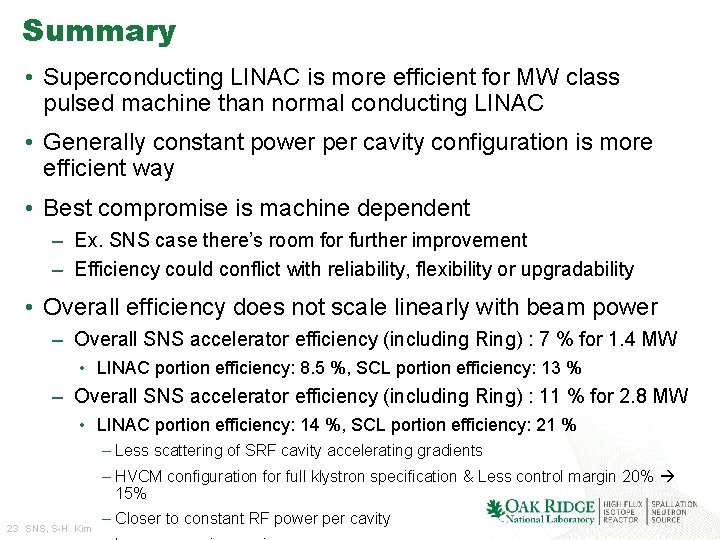
- Slides: 23
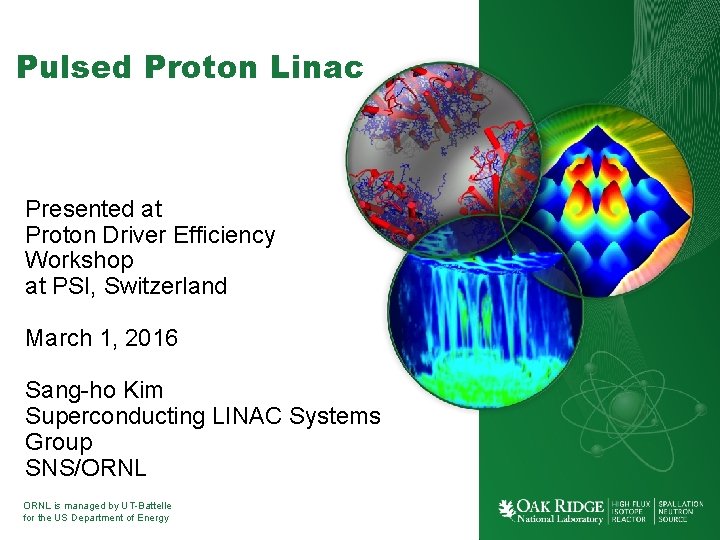
Pulsed Proton Linac Presented at Proton Driver Efficiency Workshop at PSI, Switzerland March 1, 2016 Sang-ho Kim Superconducting LINAC Systems Group SNS/ORNL is managed by UT-Battelle for the US Department of Energy
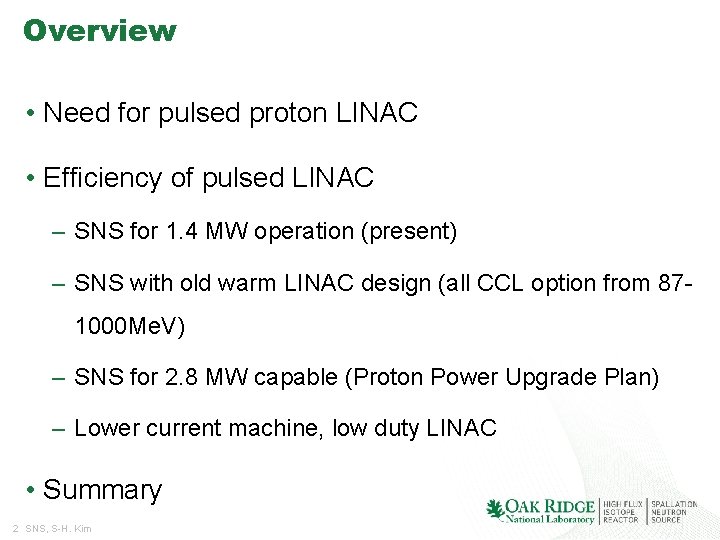
Overview • Need for pulsed proton LINAC • Efficiency of pulsed LINAC – SNS for 1. 4 MW operation (present) – SNS with old warm LINAC design (all CCL option from 871000 Me. V) – SNS for 2. 8 MW capable (Proton Power Upgrade Plan) – Lower current machine, low duty LINAC • Summary 2 SNS, S-H. Kim
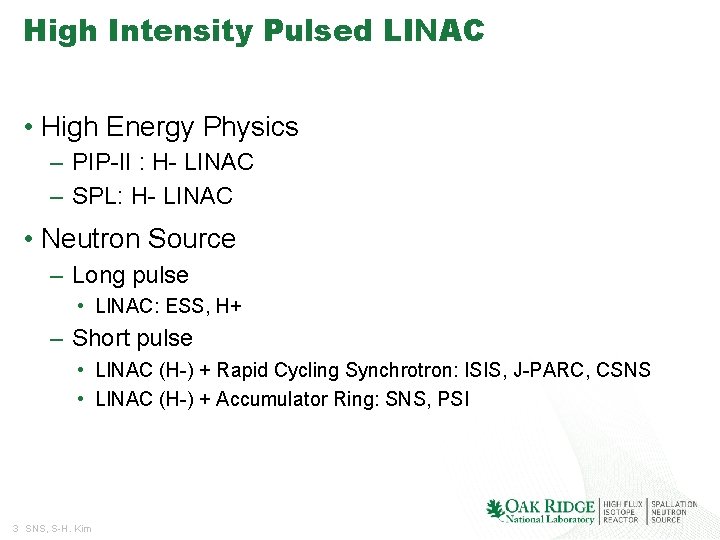
High Intensity Pulsed LINAC • High Energy Physics – PIP-II : H- LINAC – SPL: H- LINAC • Neutron Source – Long pulse • LINAC: ESS, H+ – Short pulse • LINAC (H-) + Rapid Cycling Synchrotron: ISIS, J-PARC, CSNS • LINAC (H-) + Accumulator Ring: SNS, PSI 3 SNS, S-H. Kim
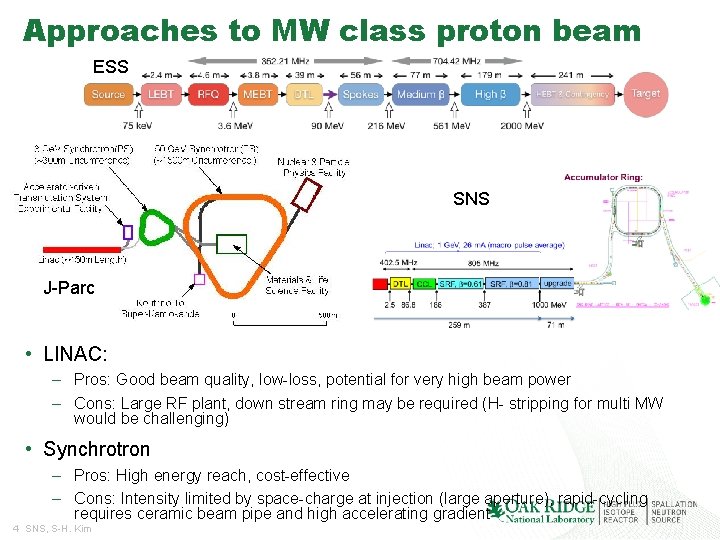
Approaches to MW class proton beam ESS SNS J-Parc • LINAC: – Pros: Good beam quality, low-loss, potential for very high beam power – Cons: Large RF plant, down stream ring may be required (H- stripping for multi MW would be challenging) • Synchrotron – Pros: High energy reach, cost-effective – Cons: Intensity limited by space-charge at injection (large aperture), rapid-cycling requires ceramic beam pipe and high accelerating gradient 4 SNS, S-H. Kim
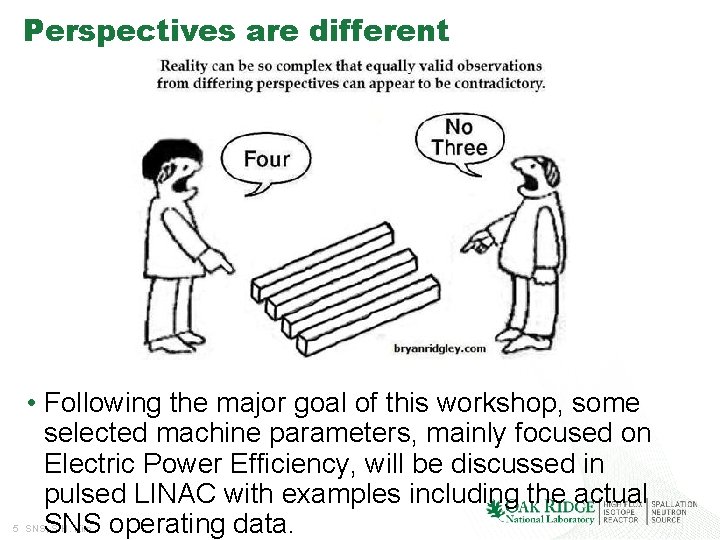
Perspectives are different • Following the major goal of this workshop, some selected machine parameters, mainly focused on Electric Power Efficiency, will be discussed in pulsed LINAC with examples including the actual SNS operating data. 5 SNS, S-H. Kim
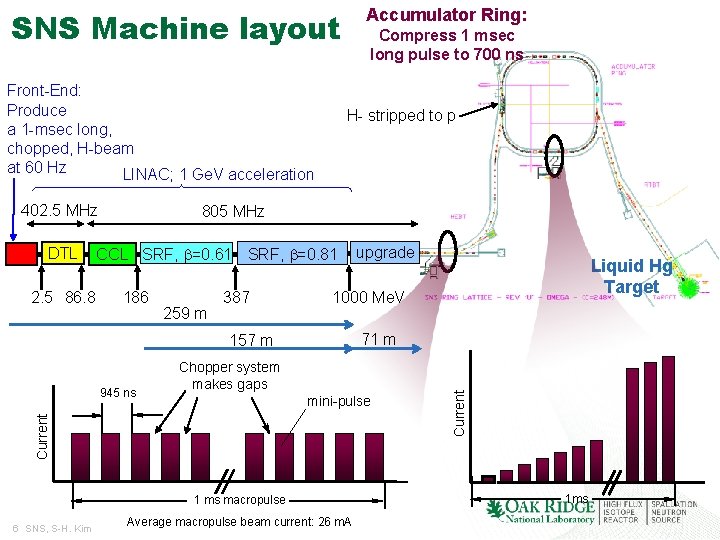
Accumulator Ring: SNS Machine layout Front-End: Produce a 1 -msec long, chopped, H-beam at 60 Hz LINAC; 1 Ge. V acceleration 402. 5 MHz 2. 5 86. 8 H- stripped to p 805 MHz CCL SRF, b=0. 61 SRF, b=0. 81 186 259 m 387 71 m Chopper system makes gaps Current mini-pulse 1 ms macropulse 6 SNS, S-H. Kim Liquid Hg Target 1000 Me. V 157 m 945 ns upgrade Average macropulse beam current: 26 m. A Current DTL Compress 1 msec long pulse to 700 ns 1 ms
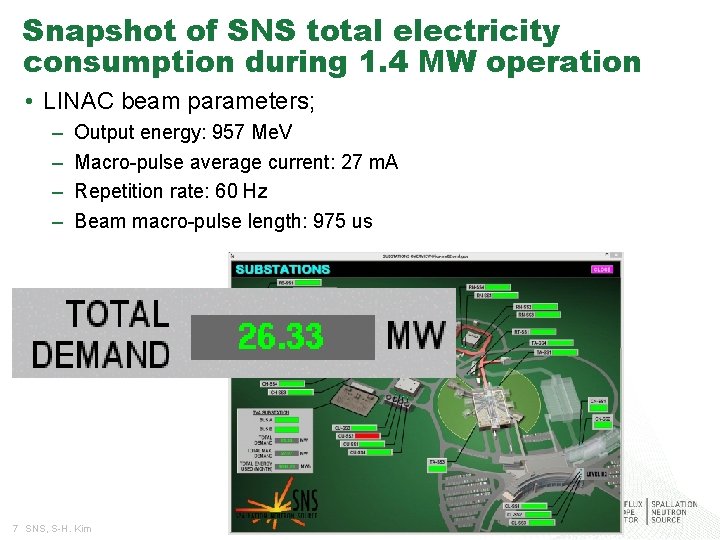
Snapshot of SNS total electricity consumption during 1. 4 MW operation • LINAC beam parameters; – – Output energy: 957 Me. V Macro-pulse average current: 27 m. A Repetition rate: 60 Hz Beam macro-pulse length: 975 us 7 SNS, S-H. Kim
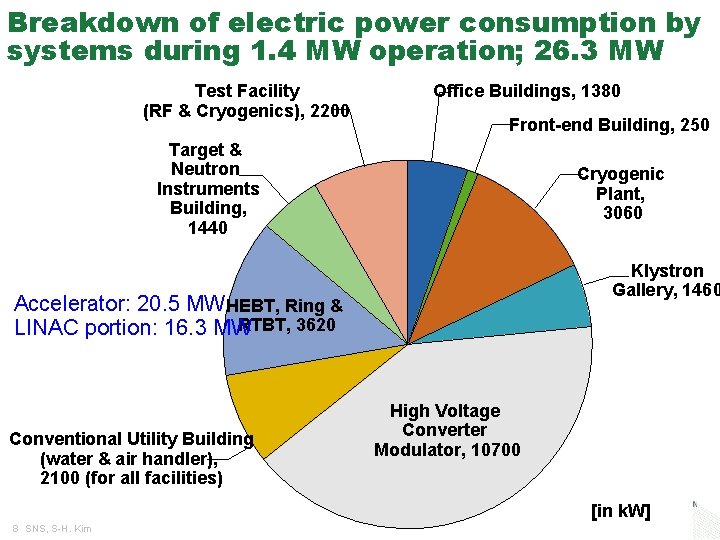
Breakdown of electric power consumption by systems during 1. 4 MW operation; 26. 3 MW Test Facility (RF & Cryogenics), 2200 Office Buildings, 1380 Front-end Building, 250 Target & Neutron Instruments Building, 1440 Cryogenic Plant, 3060 Klystron Gallery, 1460 Accelerator: 20. 5 MW HEBT, Ring & RTBT, 3620 LINAC portion: 16. 3 MW Conventional Utility Building (water & air handler), 2100 (for all facilities) High Voltage Converter Modulator, 10700 [in k. W] 8 SNS, S-H. Kim
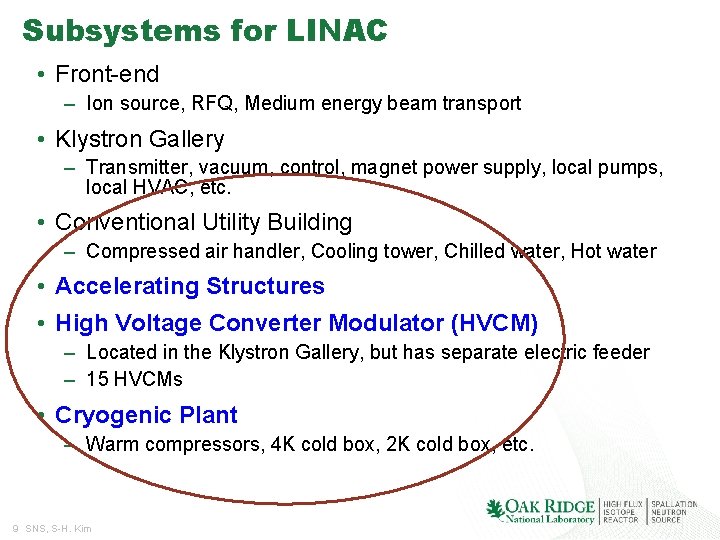
Subsystems for LINAC • Front-end – Ion source, RFQ, Medium energy beam transport • Klystron Gallery – Transmitter, vacuum, control, magnet power supply, local pumps, local HVAC, etc. • Conventional Utility Building – Compressed air handler, Cooling tower, Chilled water, Hot water • Accelerating Structures • High Voltage Converter Modulator (HVCM) – Located in the Klystron Gallery, but has separate electric feeder – 15 HVCMs • Cryogenic Plant – Warm compressors, 4 K cold box, 2 K cold box, etc. 9 SNS, S-H. Kim
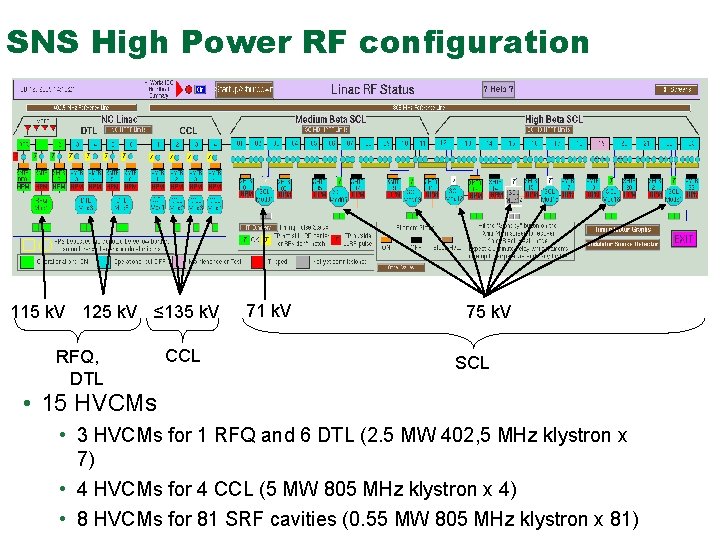
SNS High Power RF configuration 115 k. V 125 k. V ≤ 135 k. V RFQ, DTL CCL 71 k. V 75 k. V SCL • 15 HVCMs • 3 HVCMs for 1 RFQ and 6 DTL (2. 5 MW 402, 5 MHz klystron x 7) • 4 HVCMs for 4 CCL (5 MW 805 MHz klystron x 4) • 8 HVCMs for 81 SRF cavities (0. 55 MW 805 MHz klystron x 81)
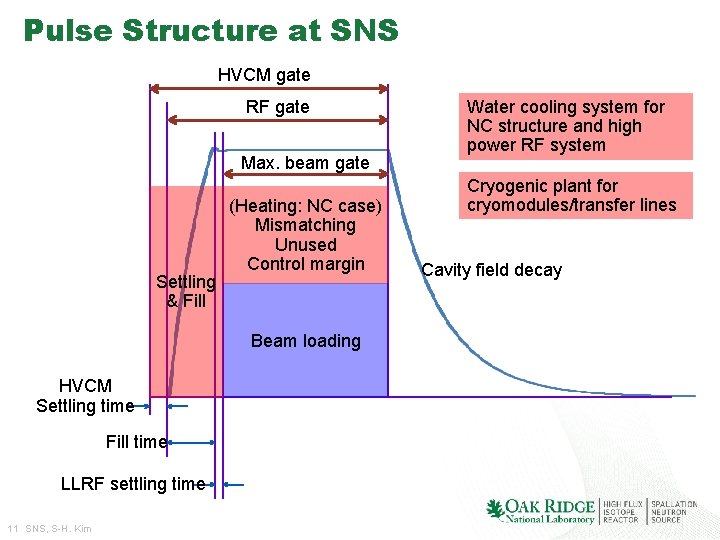
Pulse Structure at SNS HVCM gate RF gate Max. beam gate Settling & Fill (Heating: NC case) Mismatching Unused Control margin Beam loading HVCM Settling time Fill time LLRF settling time 11 SNS, S-H. Kim Water cooling system for NC structure and high power RF system Cryogenic plant for cryomodules/transfer lines Cavity field decay
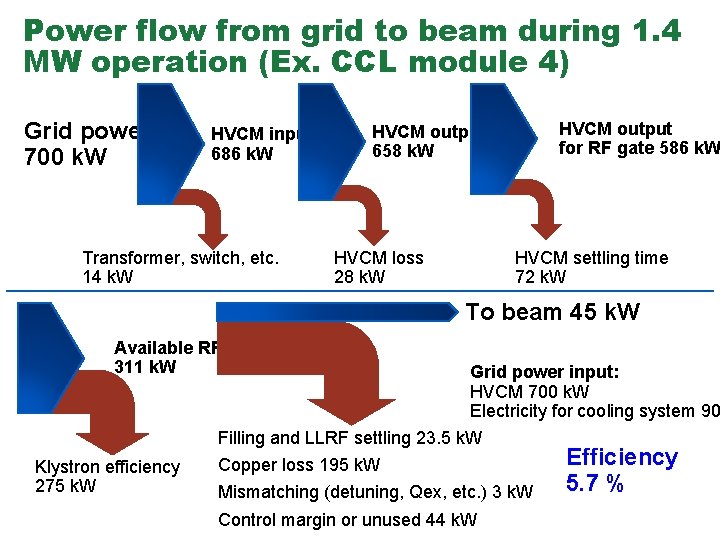
Power flow from grid to beam during 1. 4 MW operation (Ex. CCL module 4) Grid power 700 k. W HVCM input 686 k. W Transformer, switch, etc. 14 k. W HVCM output for RF gate 586 k. W HVCM output 658 k. W HVCM settling time 72 k. W HVCM loss 28 k. W To beam 45 k. W Available RF 311 k. W Klystron efficiency 275 k. W Grid power input: HVCM 700 k. W Electricity for cooling system 90 Filling and LLRF settling 23. 5 k. W Copper loss 195 k. W Mismatching (detuning, Qex, etc. ) 3 k. W Control margin or unused 44 k. W Efficiency 5. 7 %
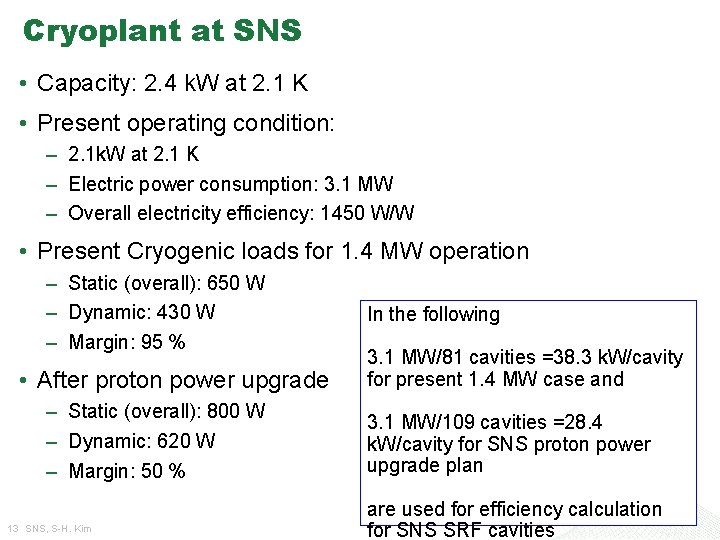
Cryoplant at SNS • Capacity: 2. 4 k. W at 2. 1 K • Present operating condition: – 2. 1 k. W at 2. 1 K – Electric power consumption: 3. 1 MW – Overall electricity efficiency: 1450 W/W • Present Cryogenic loads for 1. 4 MW operation – Static (overall): 650 W – Dynamic: 430 W – Margin: 95 % • After proton power upgrade – Static (overall): 800 W – Dynamic: 620 W – Margin: 50 % 13 SNS, S-H. Kim In the following 3. 1 MW/81 cavities =38. 3 k. W/cavity for present 1. 4 MW case and 3. 1 MW/109 cavities =28. 4 k. W/cavity for SNS proton power upgrade plan are used for efficiency calculation for SNS SRF cavities
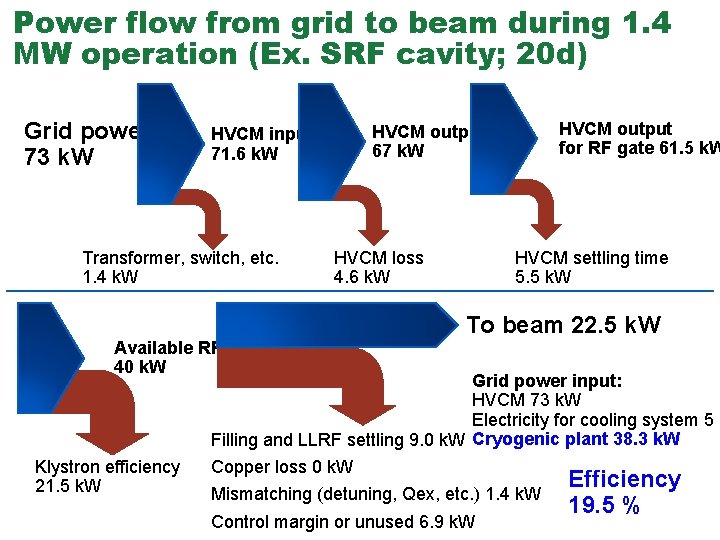
Power flow from grid to beam during 1. 4 MW operation (Ex. SRF cavity; 20 d) Grid power 73 k. W HVCM input 71. 6 k. W Transformer, switch, etc. 1. 4 k. W Available RF 40 k. W Klystron efficiency 21. 5 k. W HVCM output 67 k. W HVCM output for RF gate 61. 5 k. W HVCM settling time 5. 5 k. W HVCM loss 4. 6 k. W To beam 22. 5 k. W Grid power input: HVCM 73 k. W Electricity for cooling system 5 k Filling and LLRF settling 9. 0 k. W Cryogenic plant 38. 3 k. W Copper loss 0 k. W Efficiency Mismatching (detuning, Qex, etc. ) 1. 4 k. W Control margin or unused 6. 9 k. W 19. 5 %
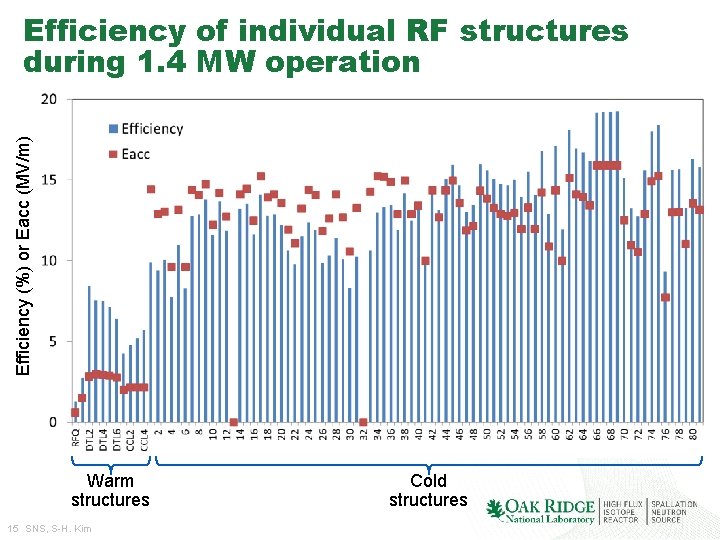
Efficiency (%) or Eacc (MV/m) Efficiency of individual RF structures during 1. 4 MW operation Warm structures 15 SNS, S-H. Kim Cold structures
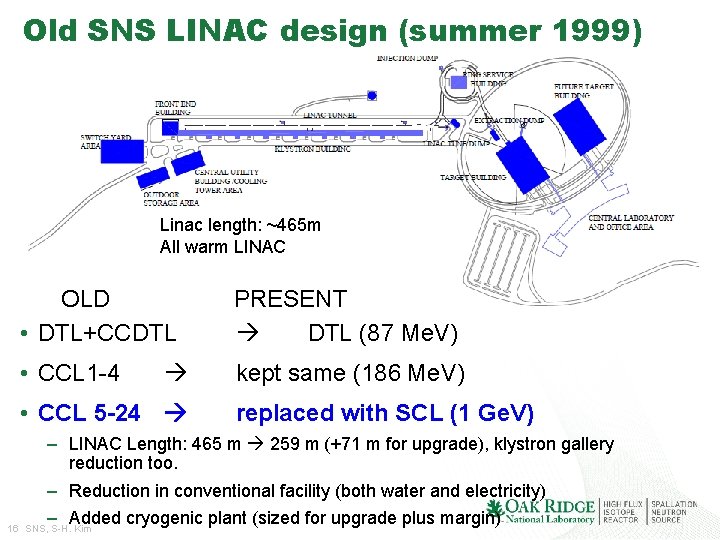
Old SNS LINAC design (summer 1999) Linac length: ~465 m All warm LINAC OLD • DTL+CCDTL PRESENT DTL (87 Me. V) • CCL 1 -4 kept same (186 Me. V) • CCL 5 -24 16 replaced with SCL (1 Ge. V) – LINAC Length: 465 m 259 m (+71 m for upgrade), klystron gallery reduction too. – Reduction in conventional facility (both water and electricity) – Added cryogenic plant (sized for upgrade plus margin) SNS, S-H. Kim
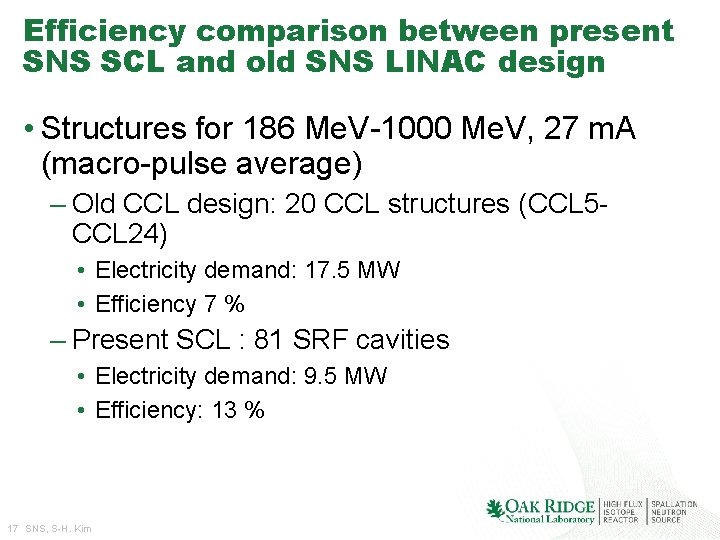
Efficiency comparison between present SNS SCL and old SNS LINAC design • Structures for 186 Me. V-1000 Me. V, 27 m. A (macro-pulse average) – Old CCL design: 20 CCL structures (CCL 5 CCL 24) • Electricity demand: 17. 5 MW • Efficiency 7 % – Present SCL : 81 SRF cavities • Electricity demand: 9. 5 MW • Efficiency: 13 % 17 SNS, S-H. Kim
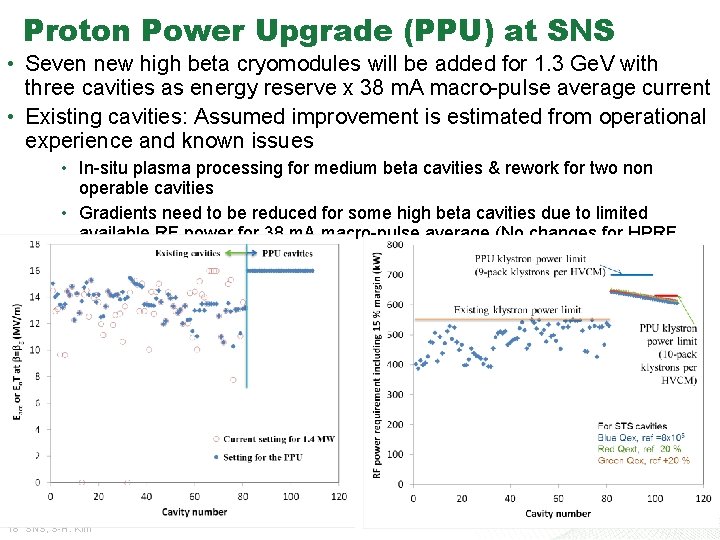
Proton Power Upgrade (PPU) at SNS • Seven new high beta cryomodules will be added for 1. 3 Ge. V with three cavities as energy reserve x 38 m. A macro-pulse average current • Existing cavities: Assumed improvement is estimated from operational experience and known issues • In-situ plasma processing for medium beta cavities & rework for two non operable cavities • Gradients need to be reduced for some high beta cavities due to limited available RF power for 38 m. A macro-pulse average (No changes for HPRF systems) 18 SNS, S-H. Kim
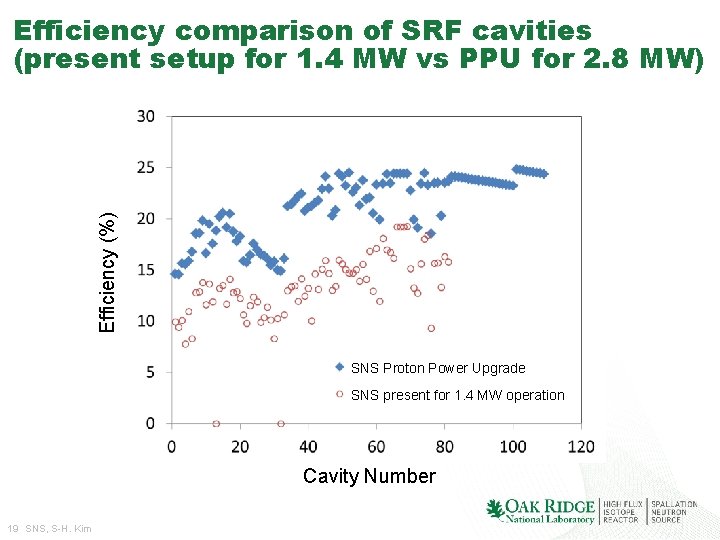
Efficiency (%) Efficiency comparison of SRF cavities (present setup for 1. 4 MW vs PPU for 2. 8 MW) SNS Proton Power Upgrade SNS present for 1. 4 MW operation Cavity Number 19 SNS, S-H. Kim
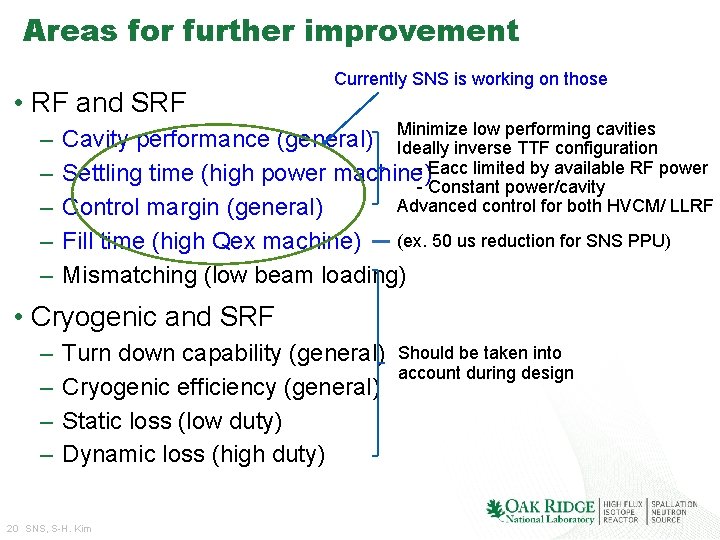
Areas for further improvement • RF and SRF – – – Currently SNS is working on those Minimize low performing cavities Cavity performance (general) Ideally inverse TTF configuration - Eacc limited by available RF power Settling time (high power machine) - Constant power/cavity Advanced control for both HVCM/ LLRF Control margin (general) (ex. 50 us reduction for SNS PPU) Fill time (high Qex machine) Mismatching (low beam loading) • Cryogenic and SRF – – Turn down capability (general) Cryogenic efficiency (general) Static loss (low duty) Dynamic loss (high duty) 20 SNS, S-H. Kim Should be taken into account during design
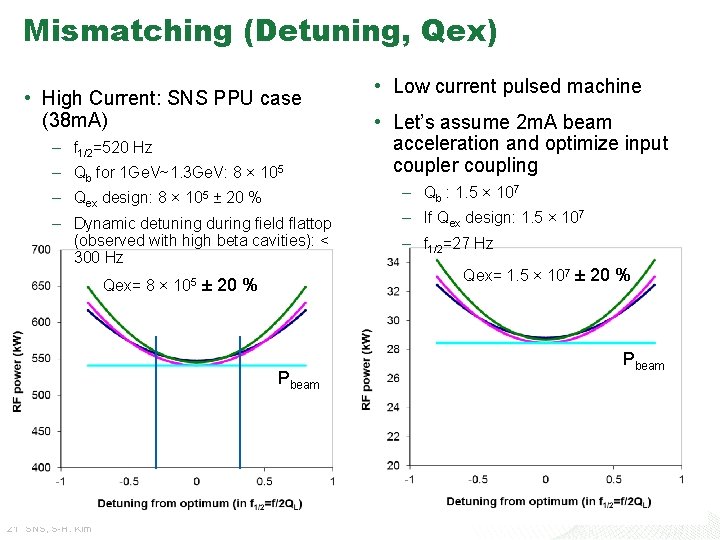
Mismatching (Detuning, Qex) • High Current: SNS PPU case (38 m. A) – f 1/2=520 Hz – Qb for 1 Ge. V~1. 3 Ge. V: 8 × 105 • Low current pulsed machine • Let’s assume 2 m. A beam acceleration and optimize input coupler coupling – Qex design: 8 × 105 ± 20 % – Qb : 1. 5 × 107 – Dynamic detuning during field flattop (observed with high beta cavities): < 300 Hz – f 1/2=27 Hz Qex= 8 × 105 ± 20 % Pbeam 21 SNS, S-H. Kim – If Qex design: 1. 5 × 107 Qex= 1. 5 × 107 ± 20 % Pbeam
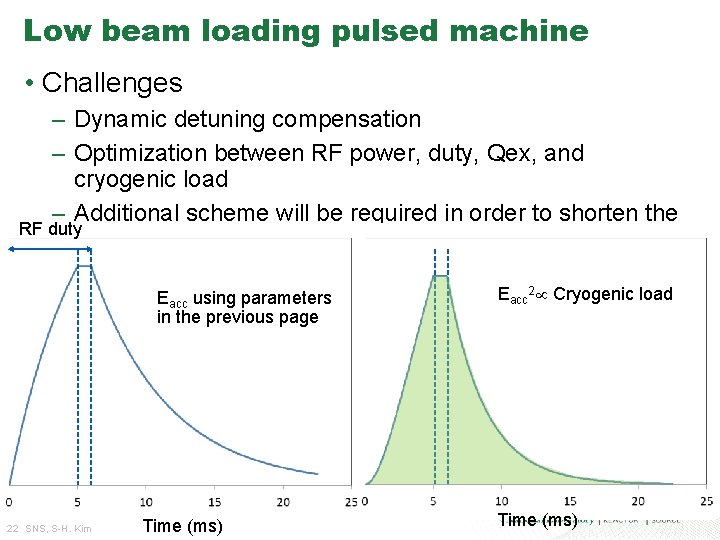
Low beam loading pulsed machine • Challenges – Dynamic detuning compensation – Optimization between RF power, duty, Qex, and cryogenic load – Additional scheme will be required in order to shorten the RF duty decay time Eacc using parameters in the previous page 22 SNS, S-H. Kim Time (ms) Eacc 2 Cryogenic load Time (ms)
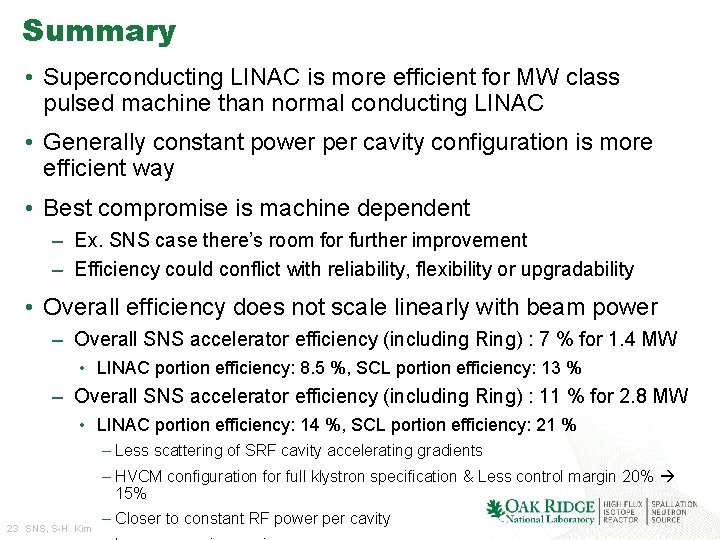
Summary • Superconducting LINAC is more efficient for MW class pulsed machine than normal conducting LINAC • Generally constant power per cavity configuration is more efficient way • Best compromise is machine dependent – Ex. SNS case there’s room for further improvement – Efficiency could conflict with reliability, flexibility or upgradability • Overall efficiency does not scale linearly with beam power – Overall SNS accelerator efficiency (including Ring) : 7 % for 1. 4 MW • LINAC portion efficiency: 8. 5 %, SCL portion efficiency: 13 % – Overall SNS accelerator efficiency (including Ring) : 11 % for 2. 8 MW • LINAC portion efficiency: 14 %, SCL portion efficiency: 21 % – Less scattering of SRF cavity accelerating gradients – HVCM configuration for full klystron specification & Less control margin 20% 15% 23 SNS, S-H. Kim – Closer to constant RF power per cavity
Pulsemaster pulsed electric field
Pulsed coherent radar
Hf ionization energy
Proton proton chain
Proton proton chain
Fission reaction
Productive inefficiency and allocative inefficiency
Allocative efficiency vs productive efficiency
Productively efficient vs allocatively efficient
Windows driver foundation - user-mode driver framework
Flatining
Linac
Linac
Neltun
Linac
Linac 4
Linac
Maurizio vretenar
Linac
Linac
Linac 4
Linac
Linac treatment head
Linac 4