COOLING OF NEUTRON STARS D G Yakovlev Ioffe
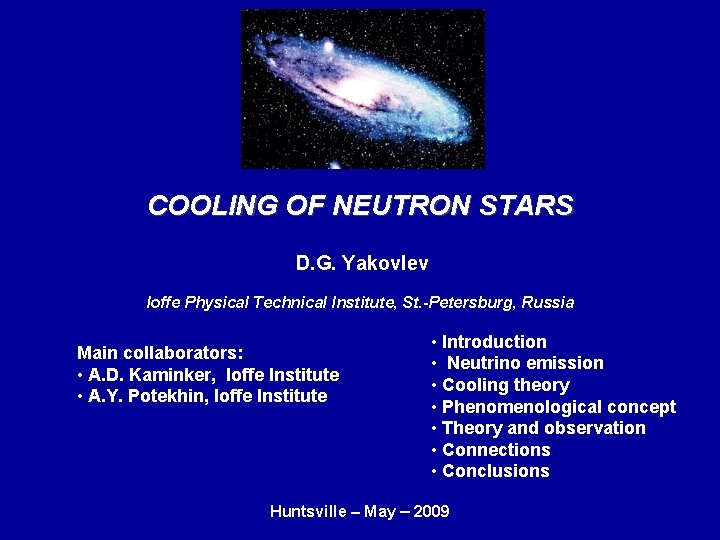
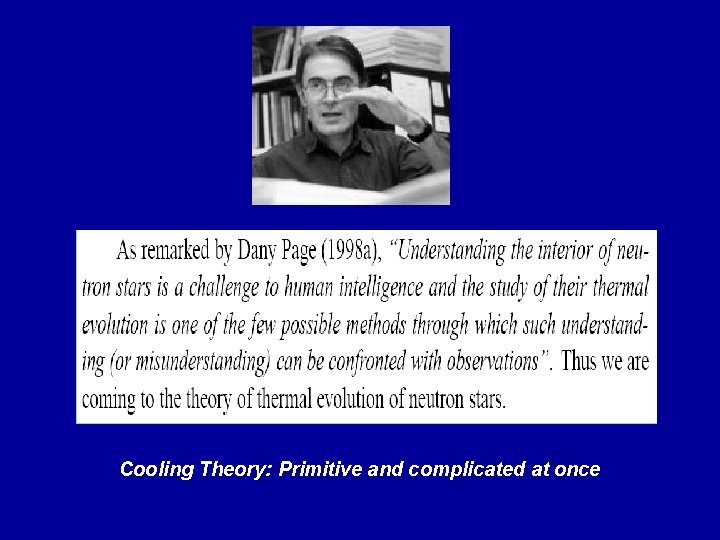
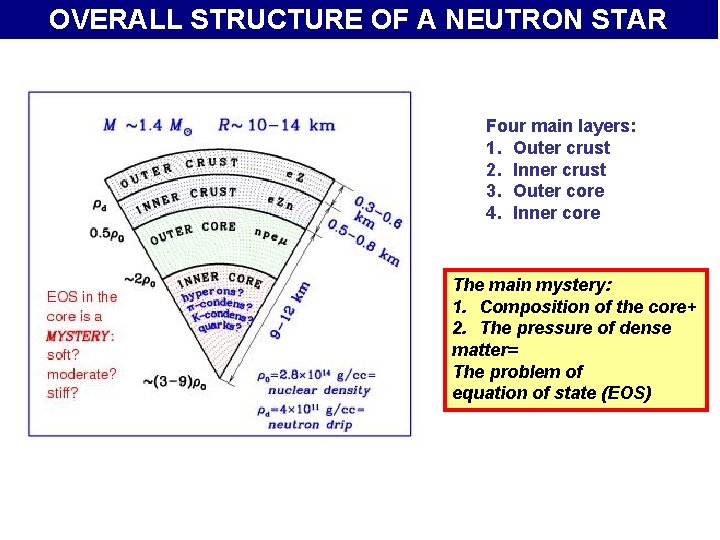
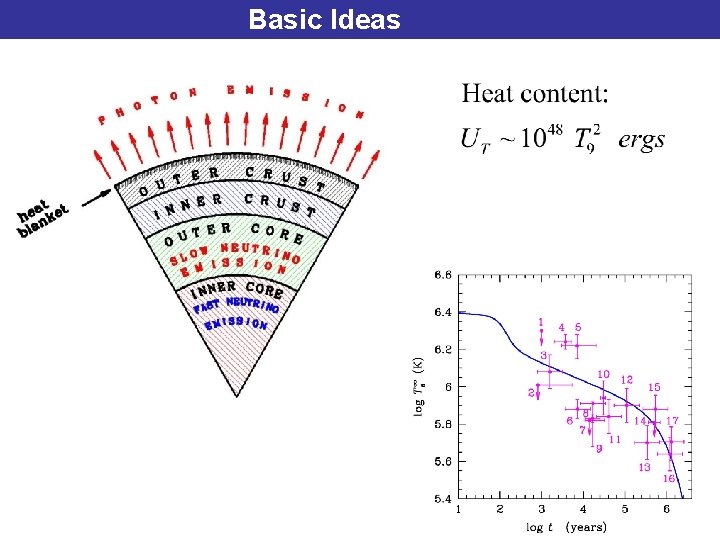
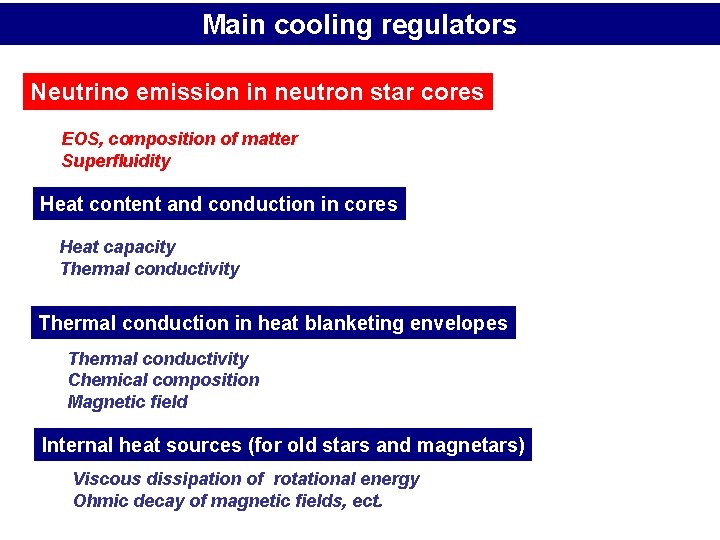
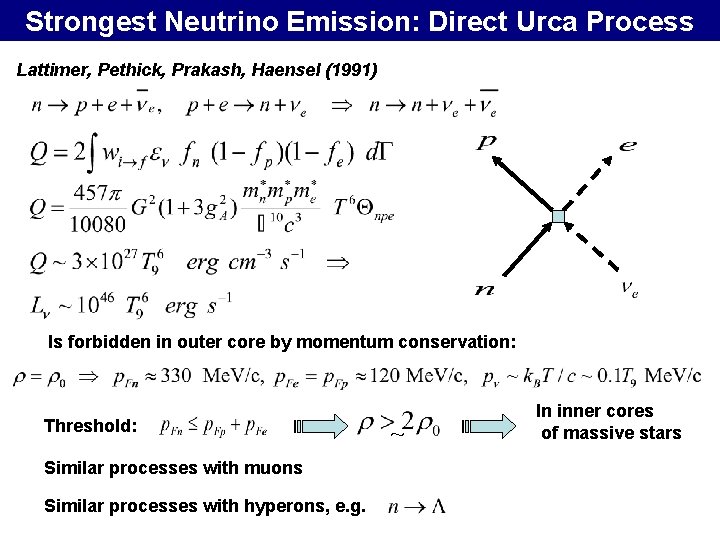
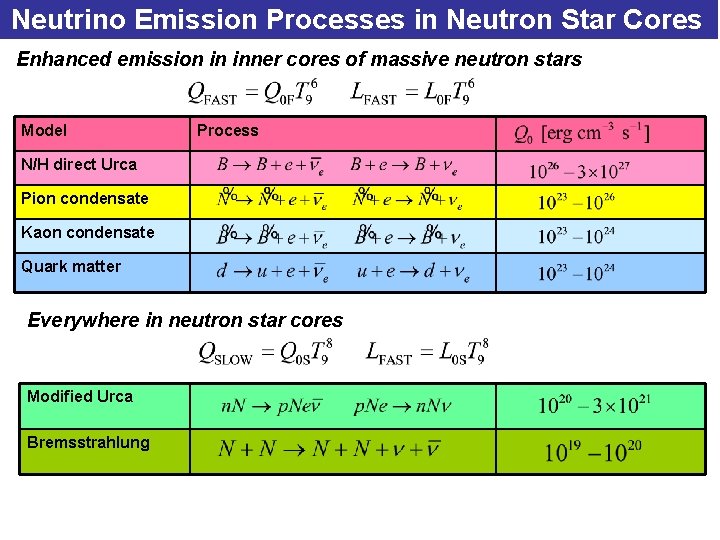
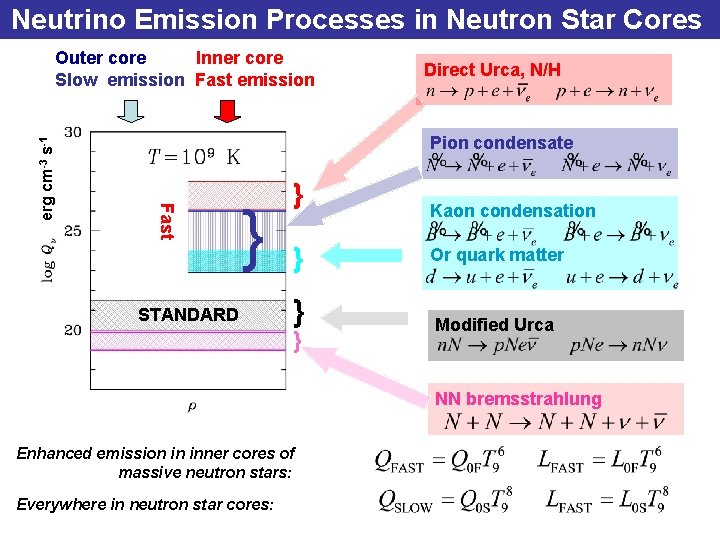
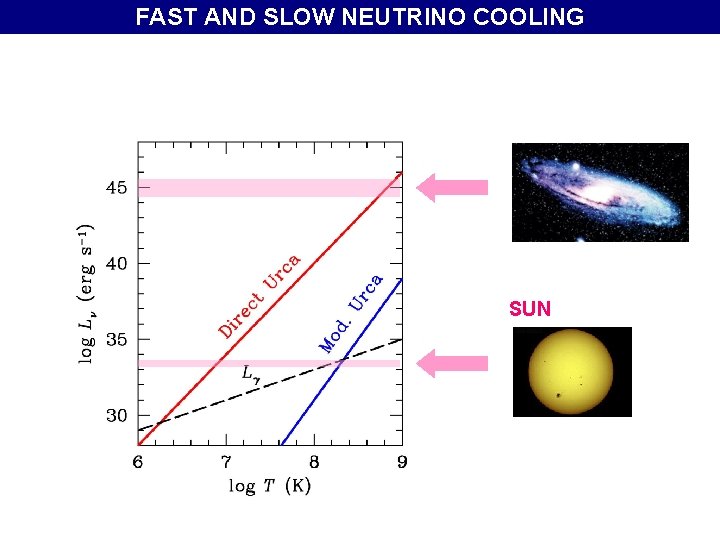
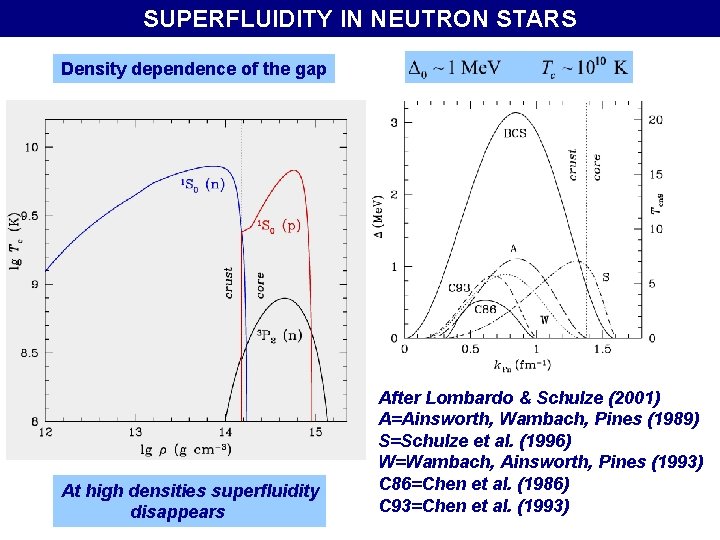
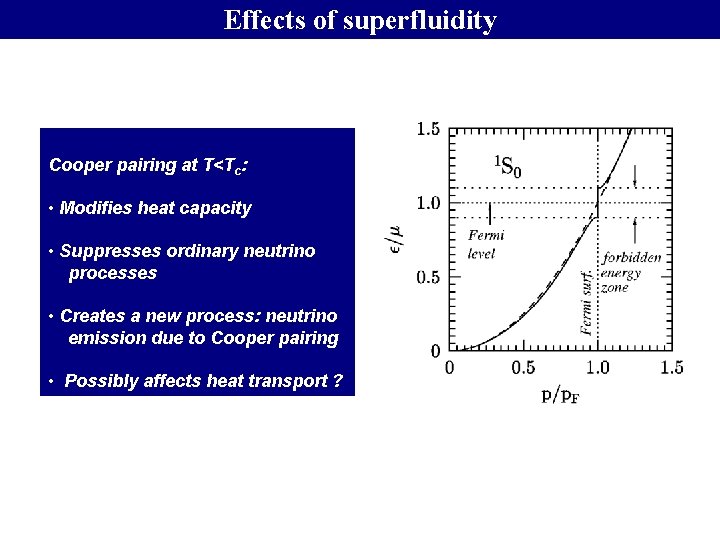
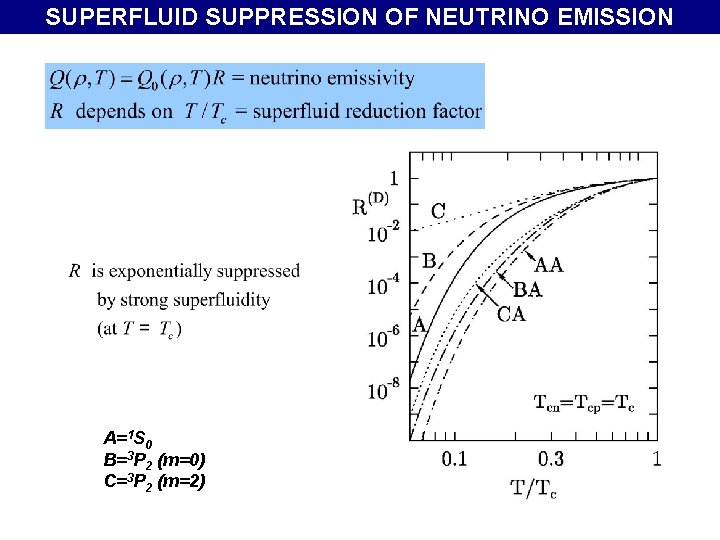
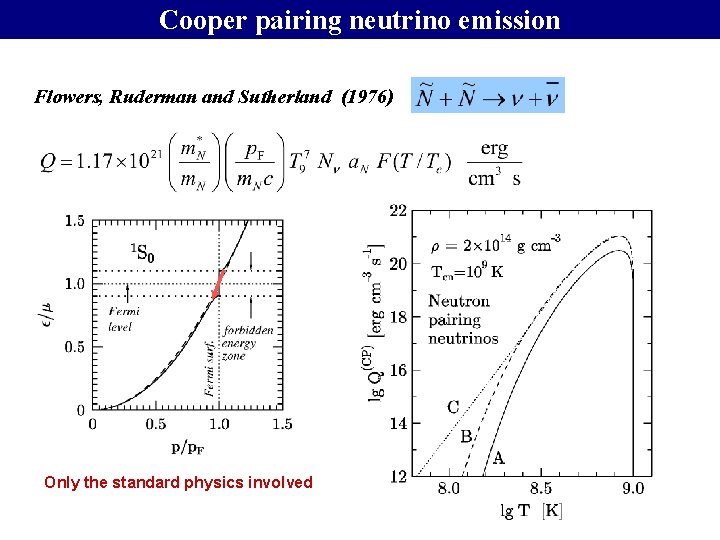
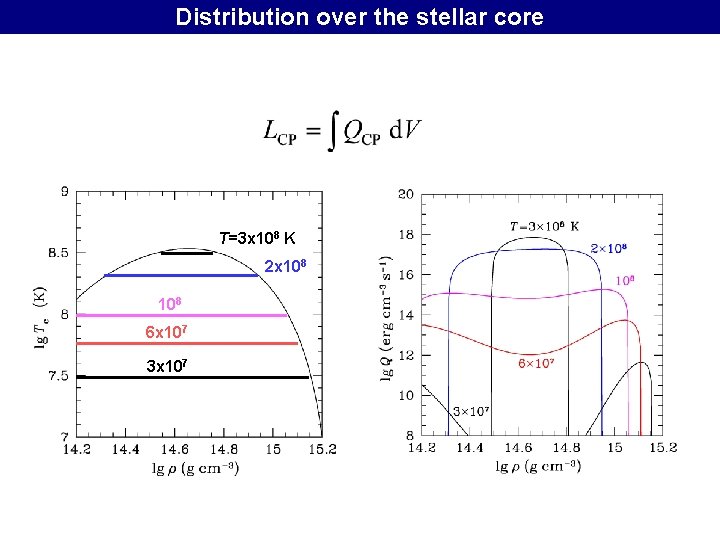
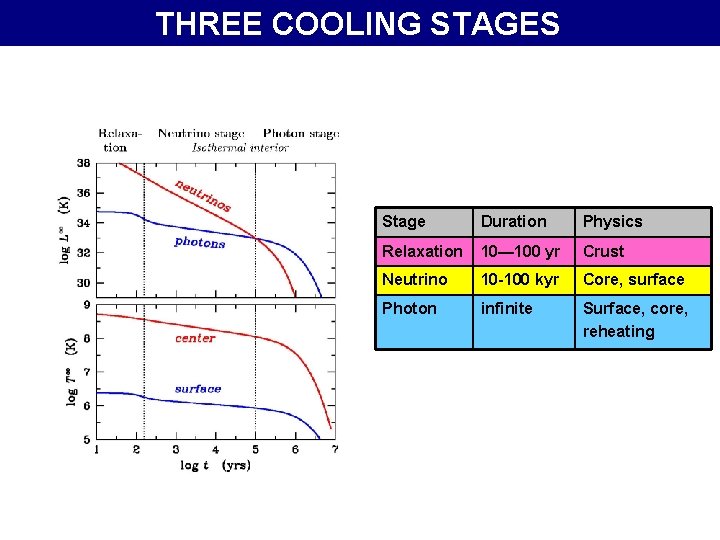
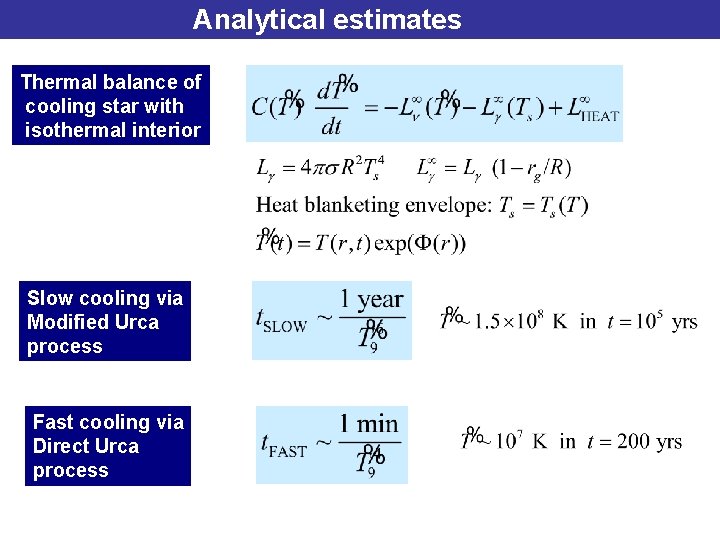
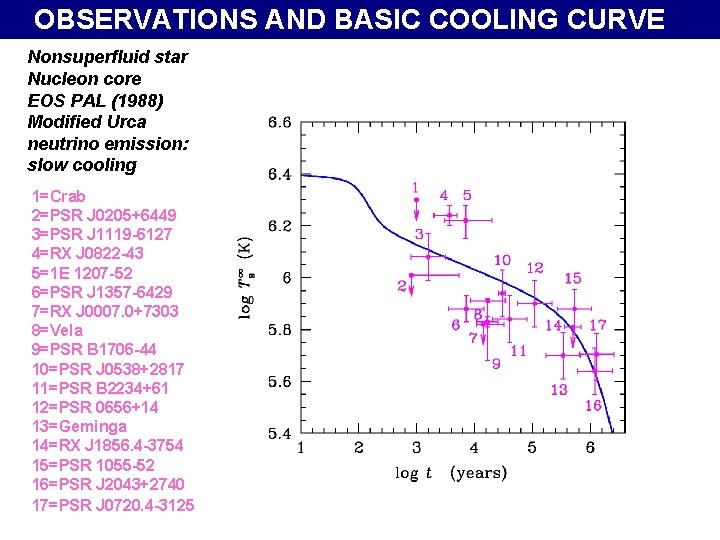
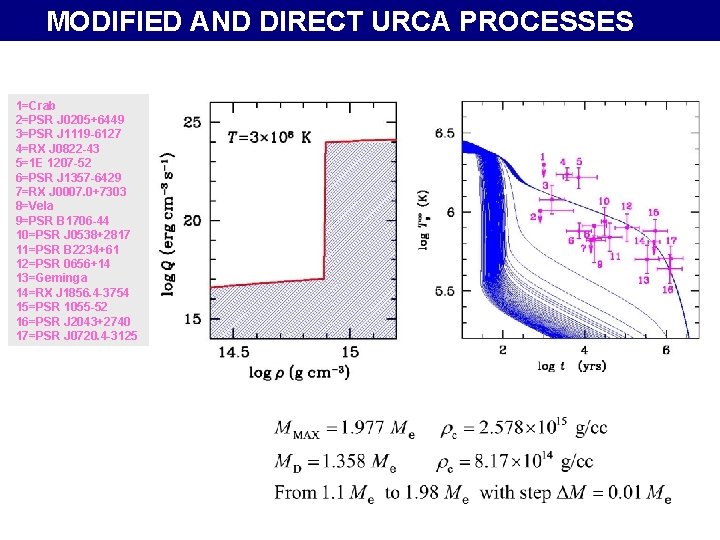
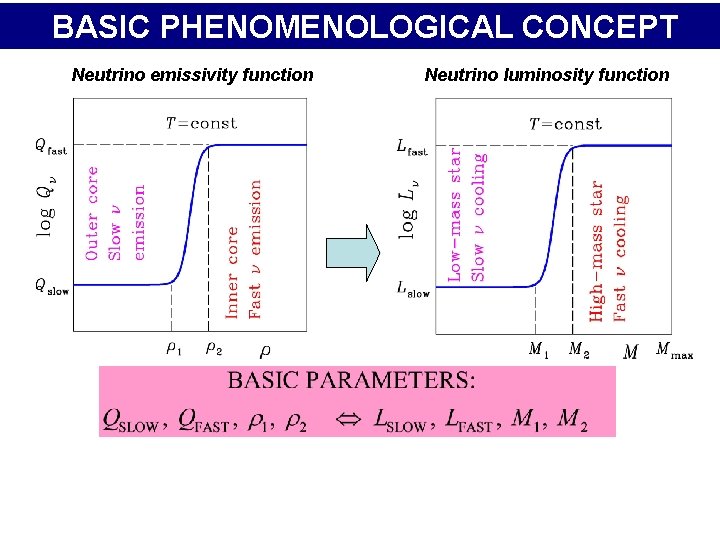
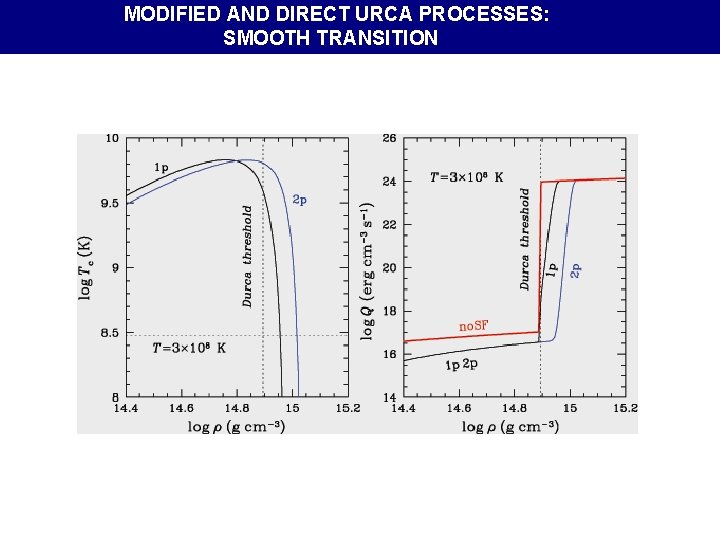
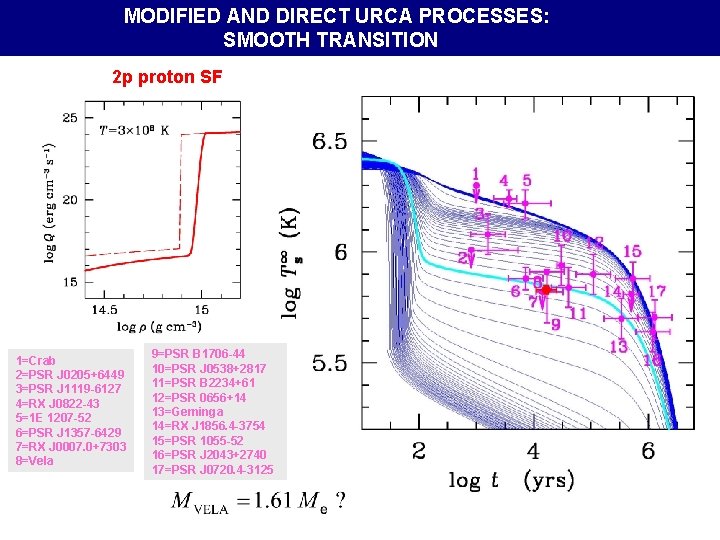
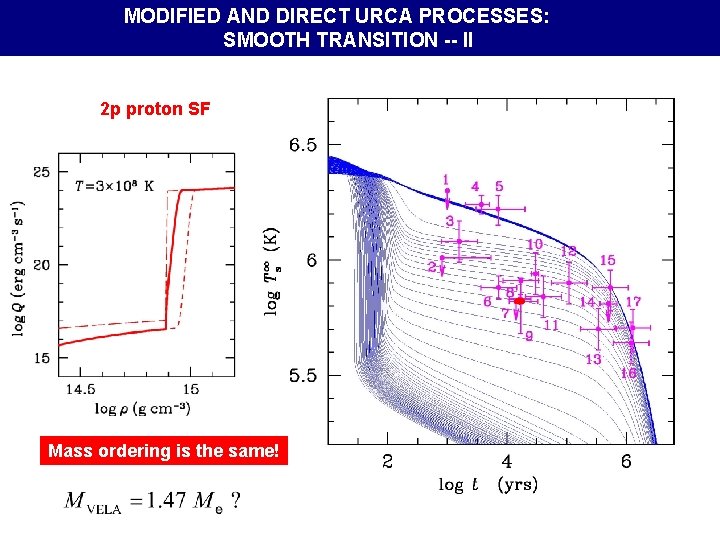
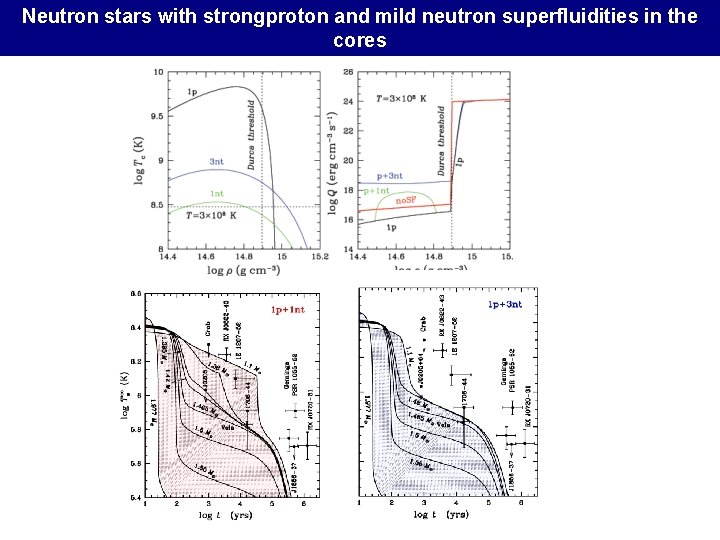
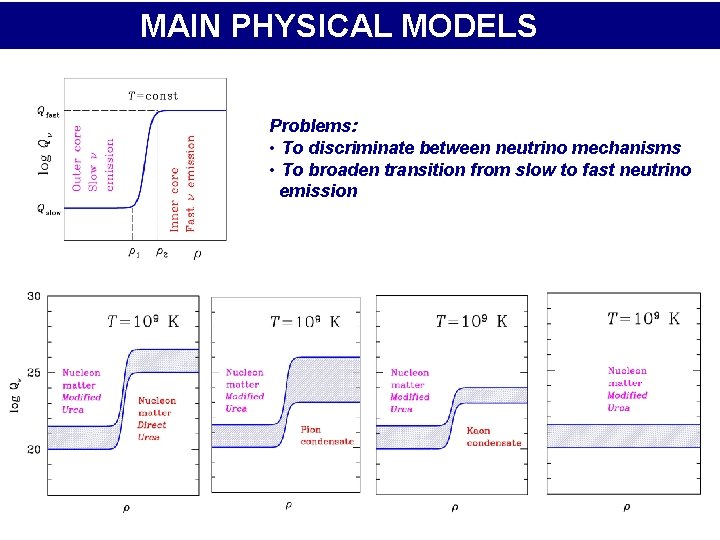
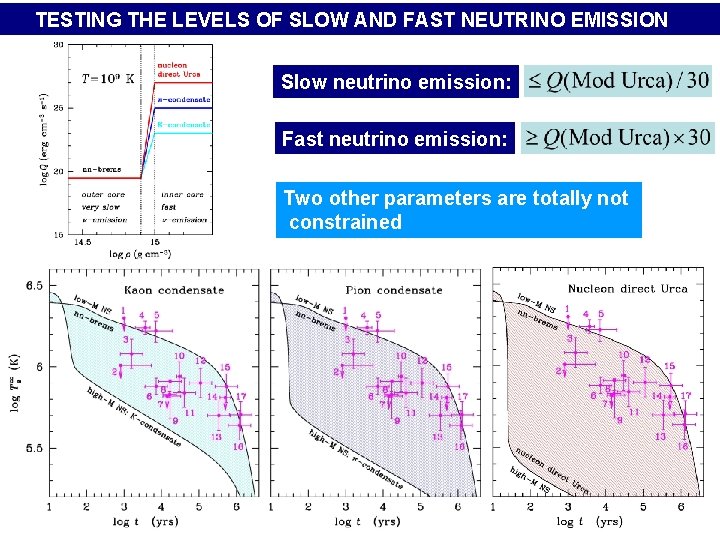
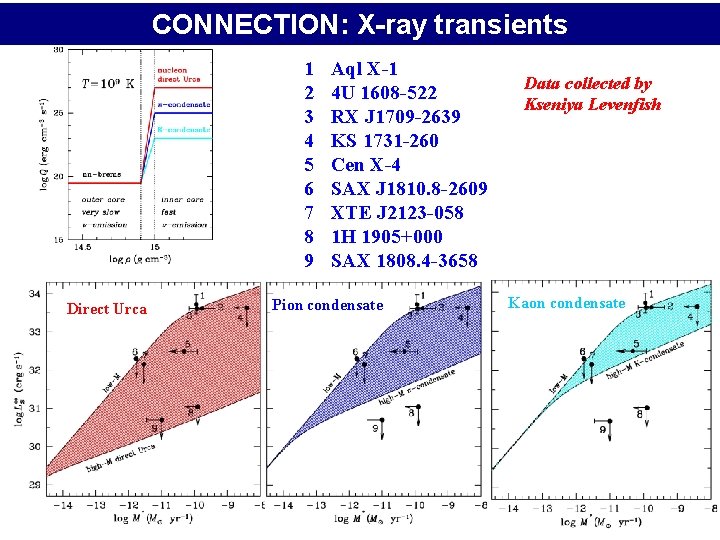
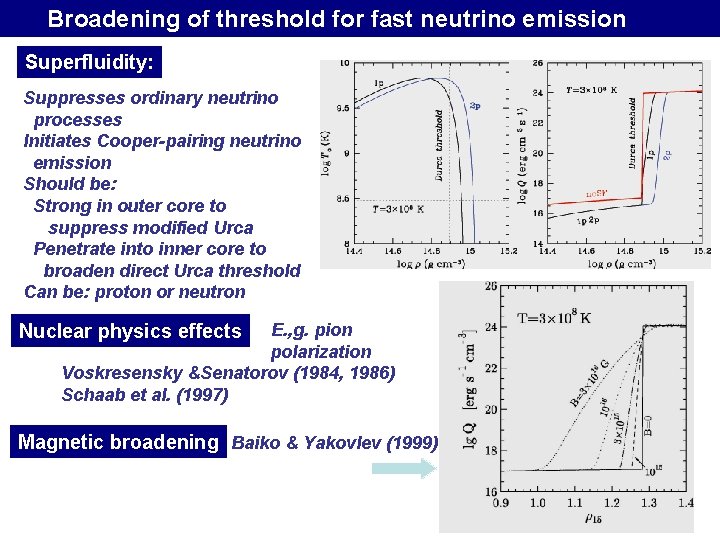
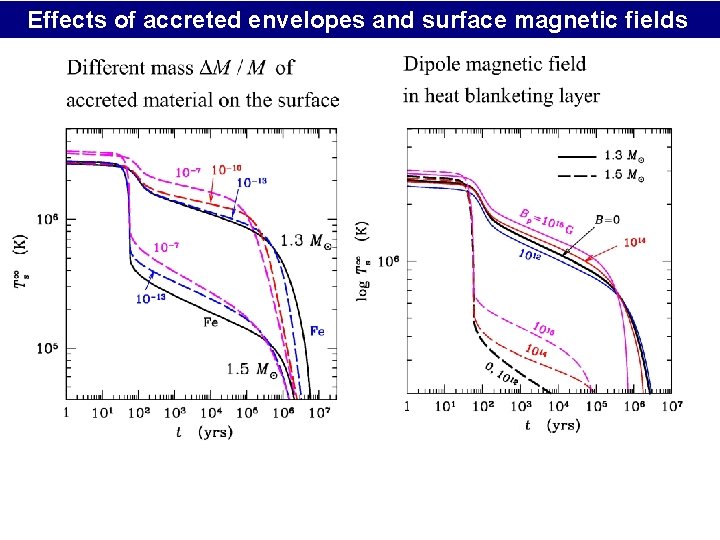
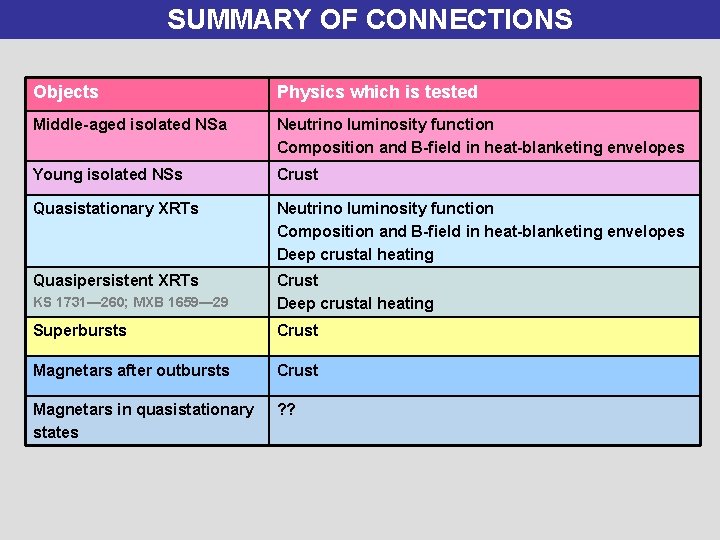
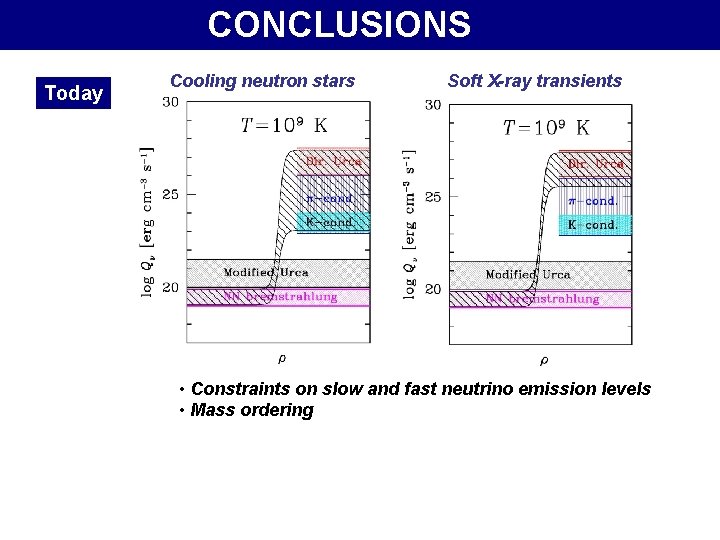
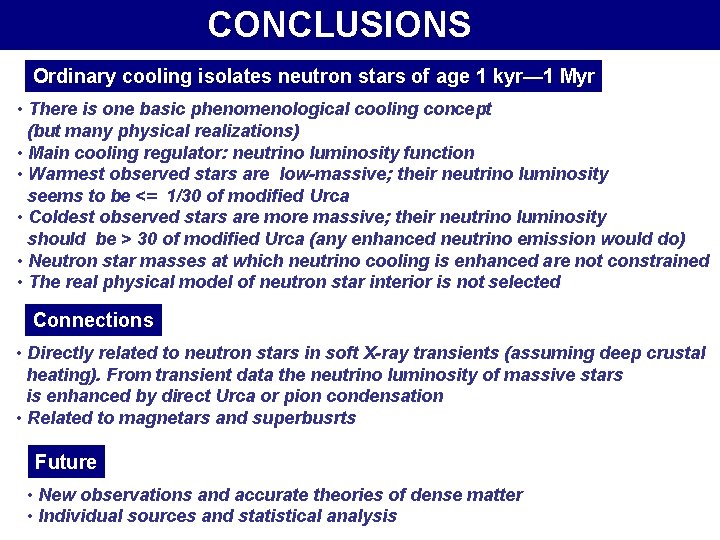
- Slides: 31
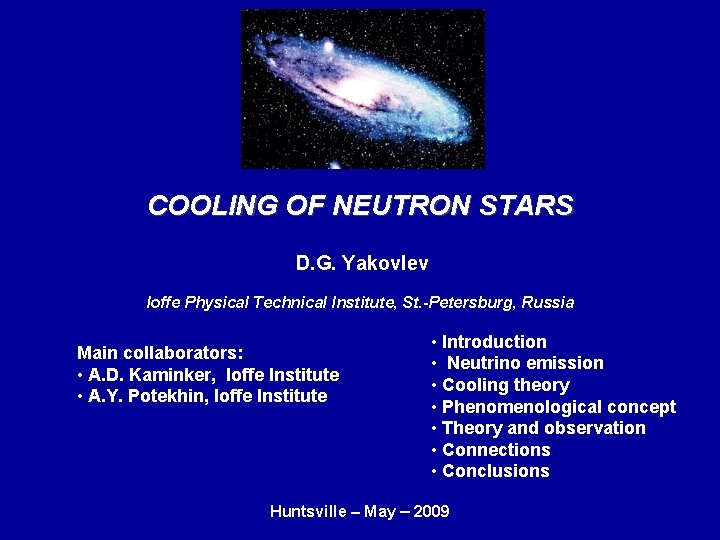
COOLING OF NEUTRON STARS D. G. Yakovlev Ioffe Physical Technical Institute, St. -Petersburg, Russia Main collaborators: • A. D. Kaminker, Ioffe Institute • A. Y. Potekhin, Ioffe Institute • Introduction • Neutrino emission • Cooling theory • Phenomenological concept • Theory and observation • Connections • Conclusions Huntsville – May – 2009
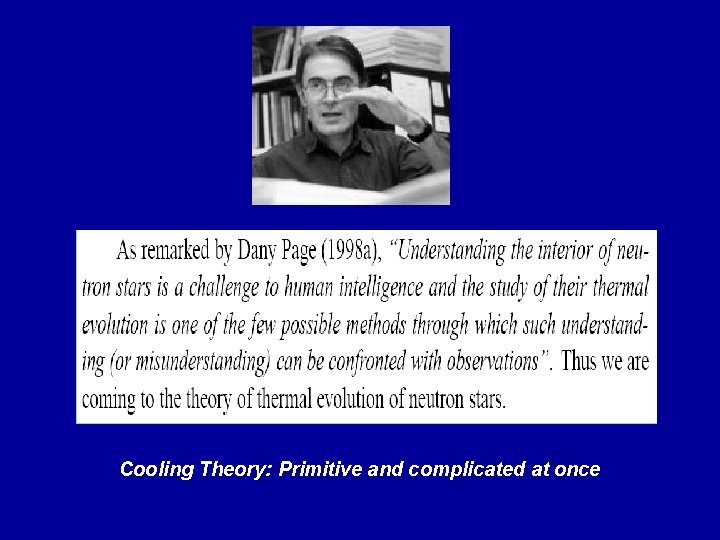
Cooling Theory: Primitive and complicated at once
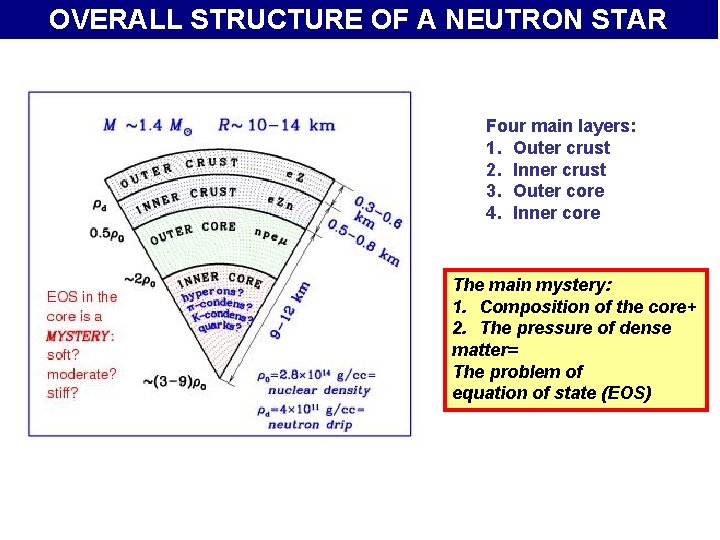
OVERALL STRUCTURE OF A NEUTRON STAR Four main layers: 1. Outer crust 2. Inner crust 3. Outer core 4. Inner core The main mystery: 1. Composition of the core+ 2. The pressure of dense matter= The problem of equation of state (EOS)
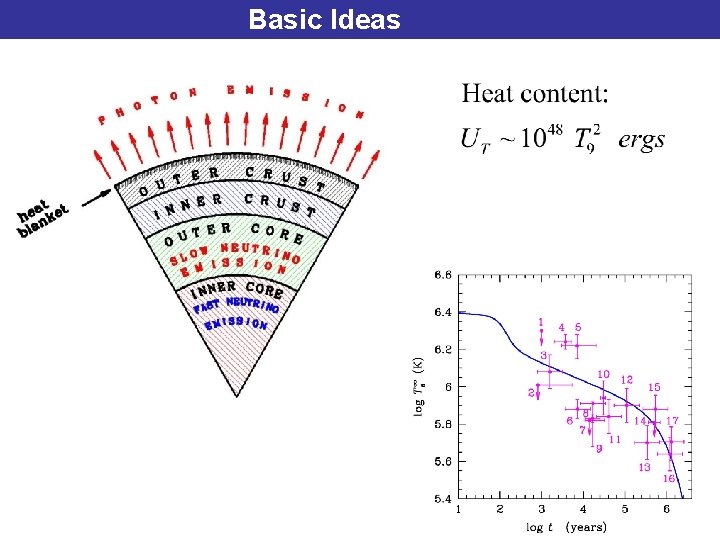
Basic Ideas
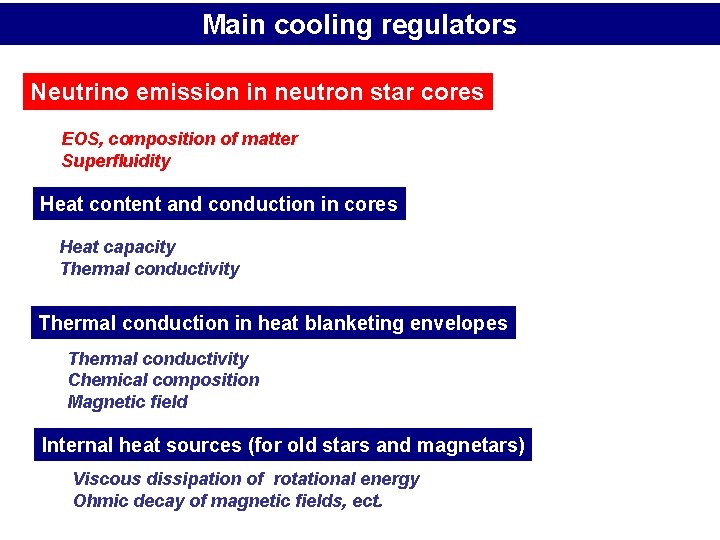
Main cooling regulators Neutrino emission in neutron star cores EOS, composition of matter Superfluidity Heat content and conduction in cores Heat capacity Thermal conductivity Thermal conduction in heat blanketing envelopes Thermal conductivity Chemical composition Magnetic field Internal heat sources (for old stars and magnetars) Viscous dissipation of rotational energy Ohmic decay of magnetic fields, ect.
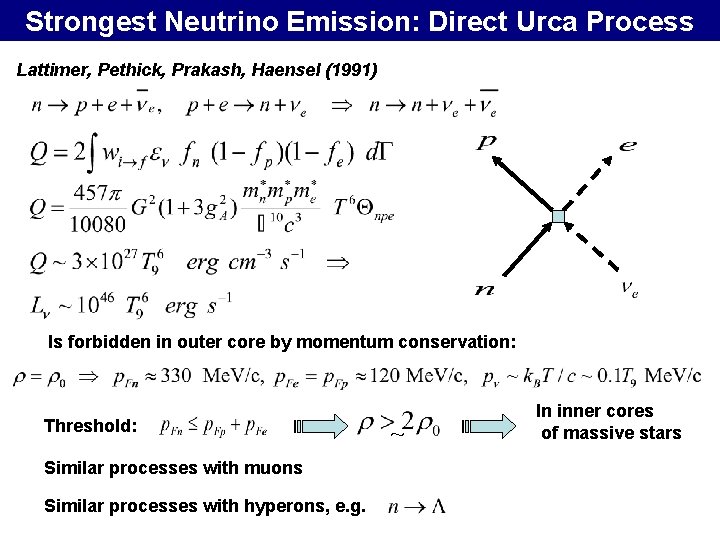
Strongest Neutrino Emission: Direct Urca Process Lattimer, Pethick, Prakash, Haensel (1991) Is forbidden in outer core by momentum conservation: Threshold: Similar processes with muons Similar processes with hyperons, e. g. ~ In inner cores of massive stars
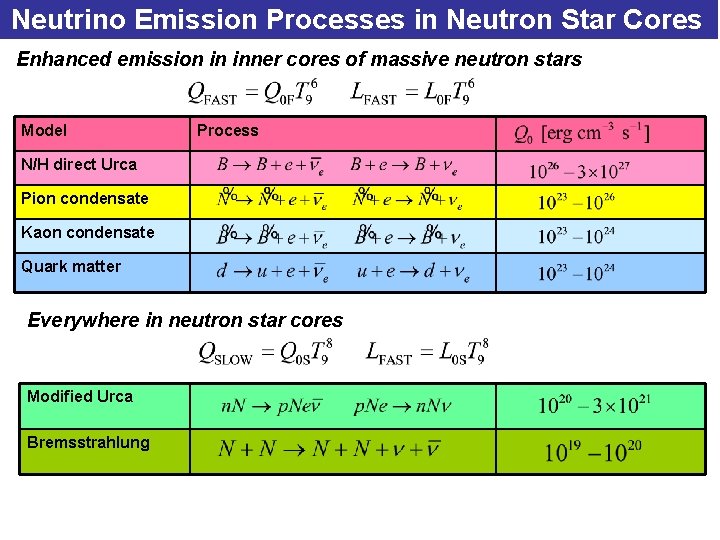
Neutrino Emission Processes in Neutron Star Cores Enhanced emission in inner cores of massive neutron stars Model Process N/H direct Urca Pion condensate Kaon condensate Quark matter Everywhere in neutron star cores Modified Urca Bremsstrahlung
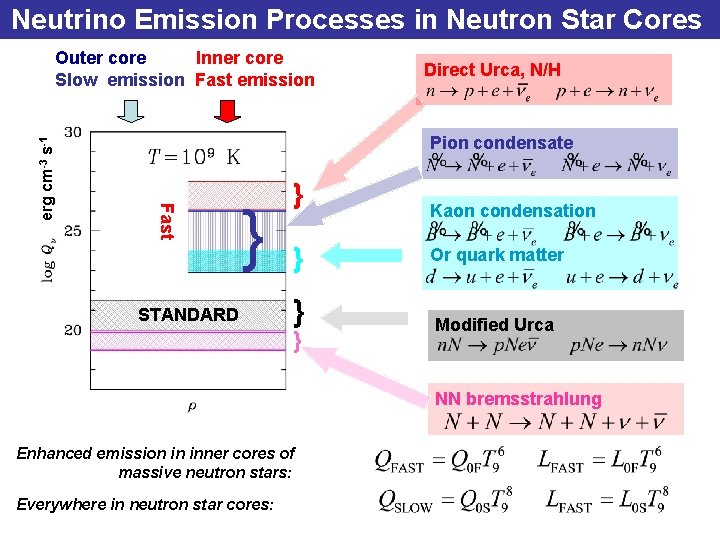
Neutrino Emission Processes in Neutron Star Cores Direct Urca, N/H Pion condensate Fast erg cm-3 s-1 Outer core Inner core Slow emission Fast emission } STANDARD } Kaon condensation } Or quark matter } Modified Urca } NN bremsstrahlung Enhanced emission in inner cores of massive neutron stars: Everywhere in neutron star cores:
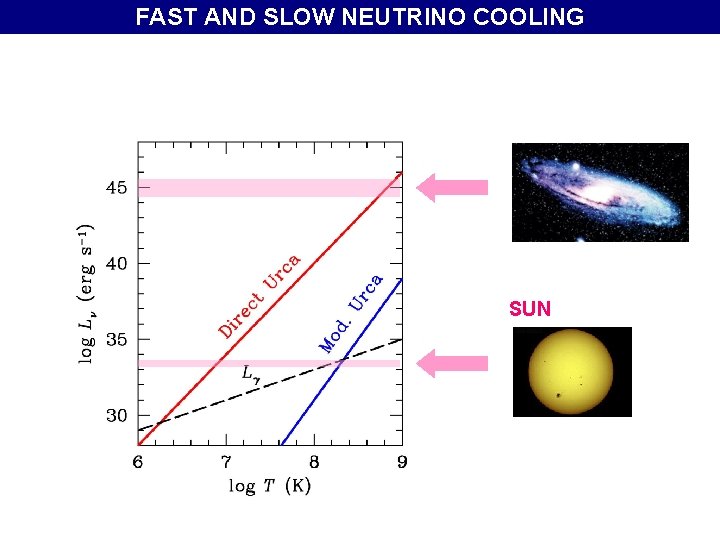
FAST AND SLOW NEUTRINO COOLING SUN
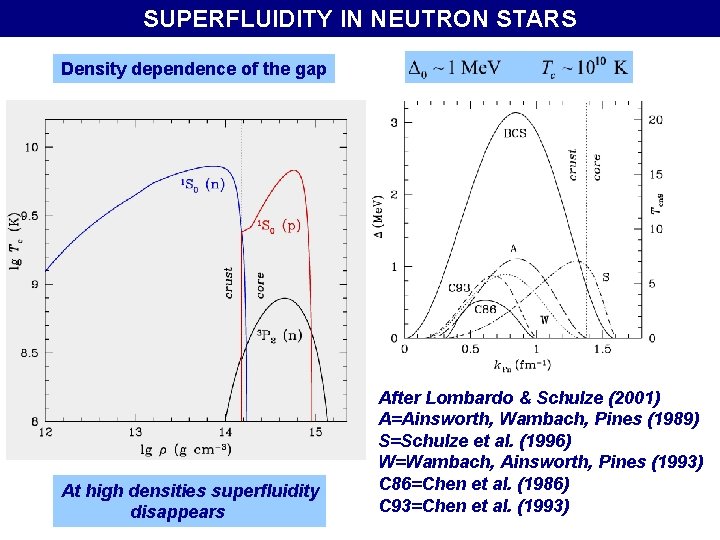
SUPERFLUIDITY IN NEUTRON STARS Density dependence of the gap At high densities superfluidity disappears After Lombardo & Schulze (2001) A=Ainsworth, Wambach, Pines (1989) S=Schulze et al. (1996) W=Wambach, Ainsworth, Pines (1993) C 86=Chen et al. (1986) C 93=Chen et al. (1993)
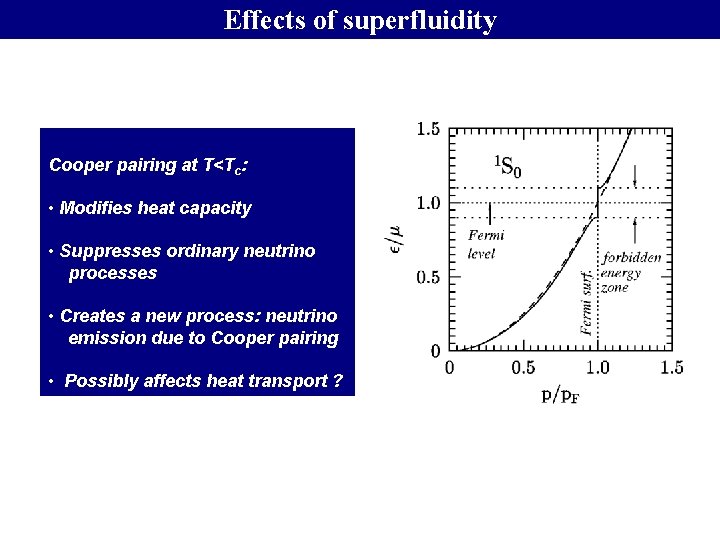
Effects of superfluidity Cooper pairing at T<Tc: • Modifies heat capacity • Suppresses ordinary neutrino processes • Creates a new process: neutrino emission due to Cooper pairing • Possibly affects heat transport ?
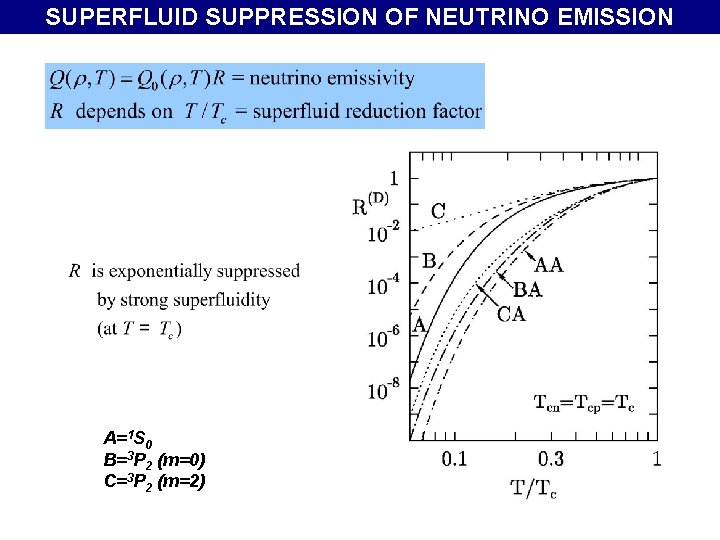
SUPERFLUID SUPPRESSION OF NEUTRINO EMISSION A=1 S 0 B=3 P 2 (m=0) C=3 P 2 (m=2)
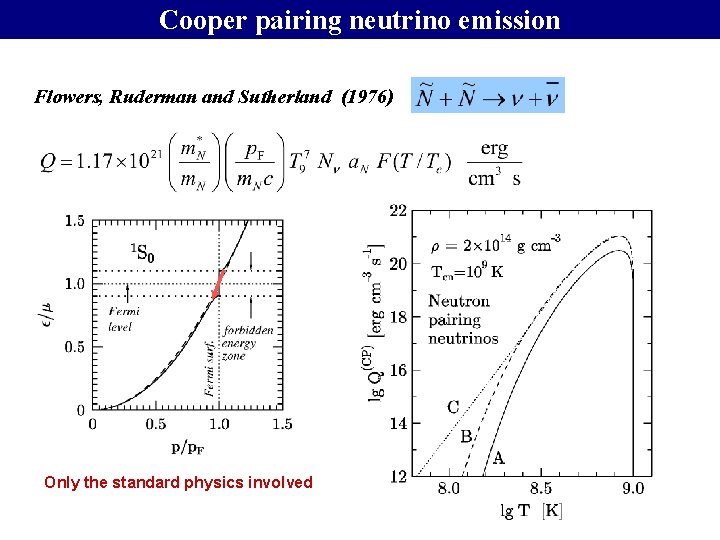
Cooper pairing neutrino emission Flowers, Ruderman and Sutherland (1976) Only the standard physics involved
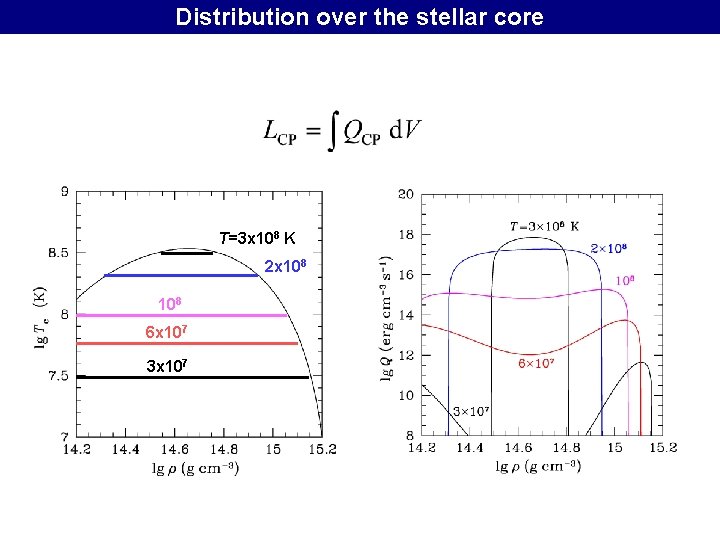
Distribution over the stellar core T=3 x 108 K 2 x 108 6 x 107 3 x 107
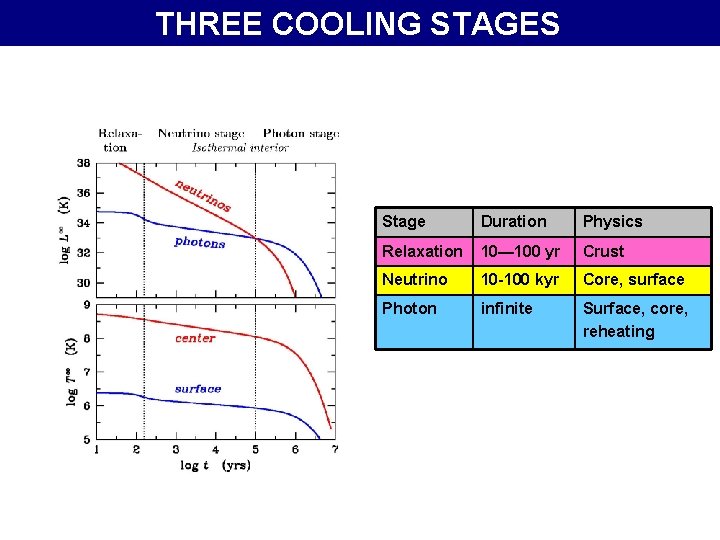
THREE COOLING STAGES Stage Duration Physics Relaxation 10— 100 yr Crust Neutrino 10 -100 kyr Core, surface Photon infinite Surface, core, reheating
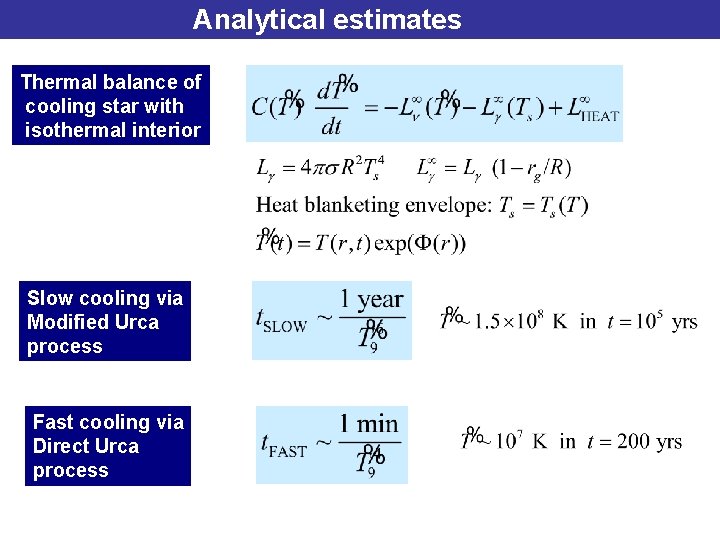
Analytical estimates Thermal balance of cooling star with isothermal interior Slow cooling via Modified Urca process Fast cooling via Direct Urca process
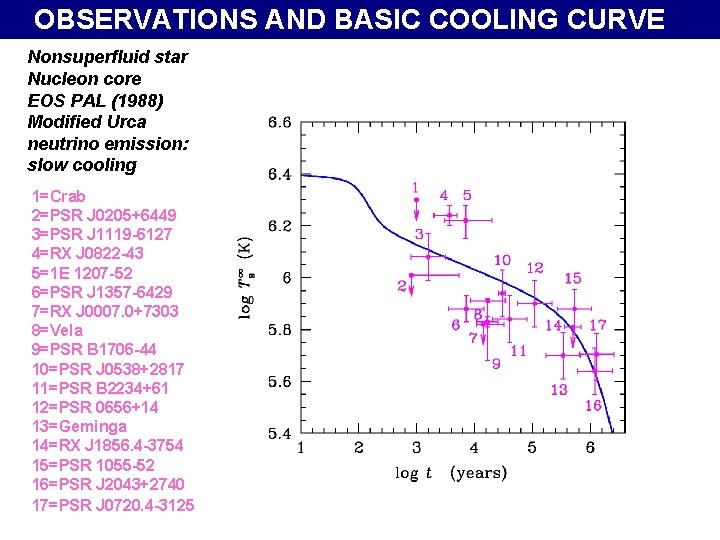
OBSERVATIONS AND BASIC COOLING CURVE Nonsuperfluid star Nucleon core EOS PAL (1988) Modified Urca neutrino emission: slow cooling 1=Crab 2=PSR J 0205+6449 3=PSR J 1119 -6127 4=RX J 0822 -43 5=1 E 1207 -52 6=PSR J 1357 -6429 7=RX J 0007. 0+7303 8=Vela 9=PSR B 1706 -44 10=PSR J 0538+2817 11=PSR B 2234+61 12=PSR 0656+14 13=Geminga 14=RX J 1856. 4 -3754 15=PSR 1055 -52 16=PSR J 2043+2740 17=PSR J 0720. 4 -3125
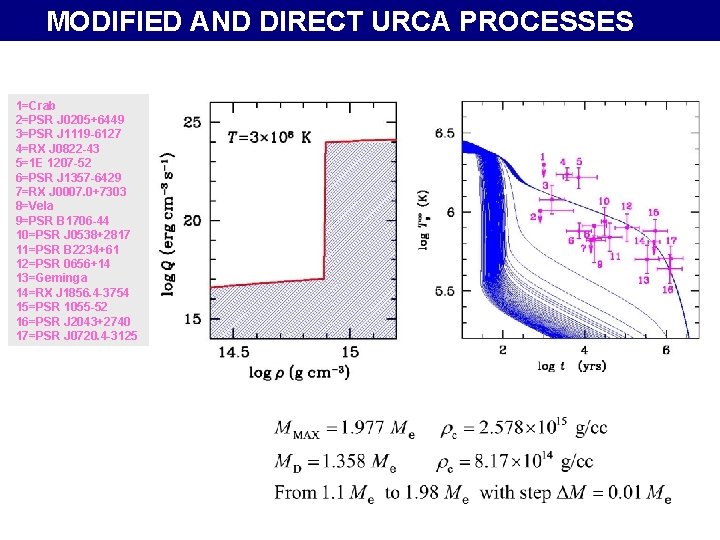
MODIFIED AND DIRECT URCA PROCESSES 1=Crab 2=PSR J 0205+6449 3=PSR J 1119 -6127 4=RX J 0822 -43 5=1 E 1207 -52 6=PSR J 1357 -6429 7=RX J 0007. 0+7303 8=Vela 9=PSR B 1706 -44 10=PSR J 0538+2817 11=PSR B 2234+61 12=PSR 0656+14 13=Geminga 14=RX J 1856. 4 -3754 15=PSR 1055 -52 16=PSR J 2043+2740 17=PSR J 0720. 4 -3125
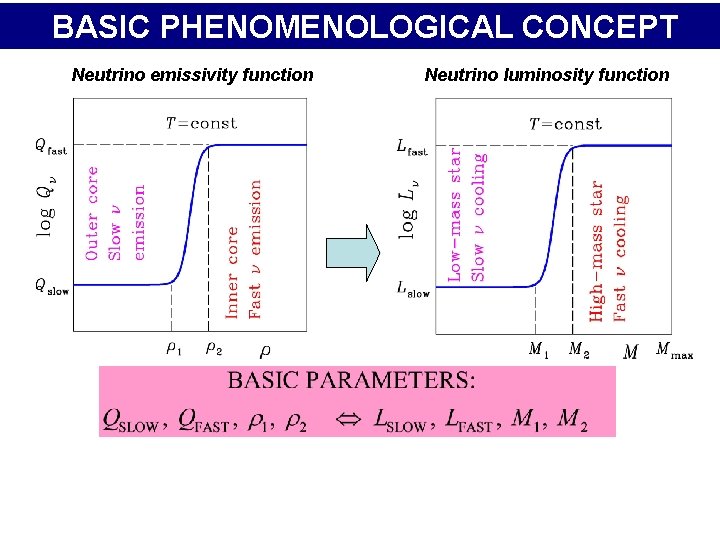
BASIC PHENOMENOLOGICAL CONCEPT Neutrino emissivity function Neutrino luminosity function
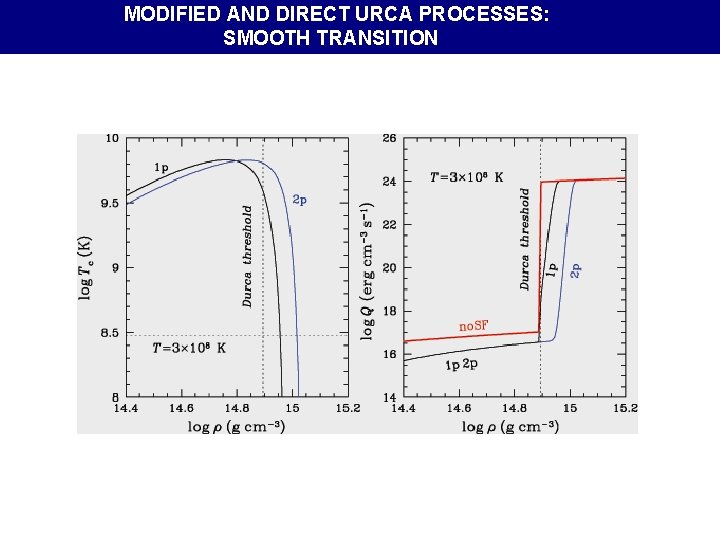
MODIFIED AND DIRECT URCA PROCESSES: SMOOTH TRANSITION
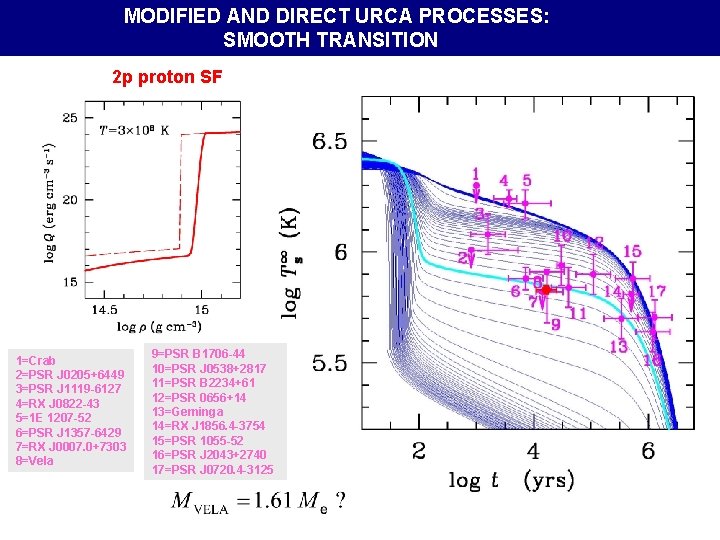
MODIFIED AND DIRECT URCA PROCESSES: SMOOTH TRANSITION 2 p proton SF 1=Crab 2=PSR J 0205+6449 3=PSR J 1119 -6127 4=RX J 0822 -43 5=1 E 1207 -52 6=PSR J 1357 -6429 7=RX J 0007. 0+7303 8=Vela 9=PSR B 1706 -44 10=PSR J 0538+2817 11=PSR B 2234+61 12=PSR 0656+14 13=Geminga 14=RX J 1856. 4 -3754 15=PSR 1055 -52 16=PSR J 2043+2740 17=PSR J 0720. 4 -3125
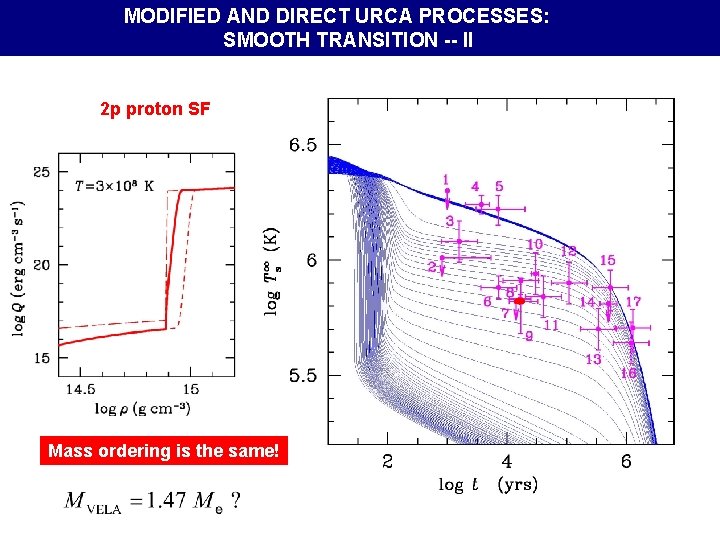
MODIFIED AND DIRECT URCA PROCESSES: SMOOTH TRANSITION -- II 2 p proton SF Mass ordering is the same!
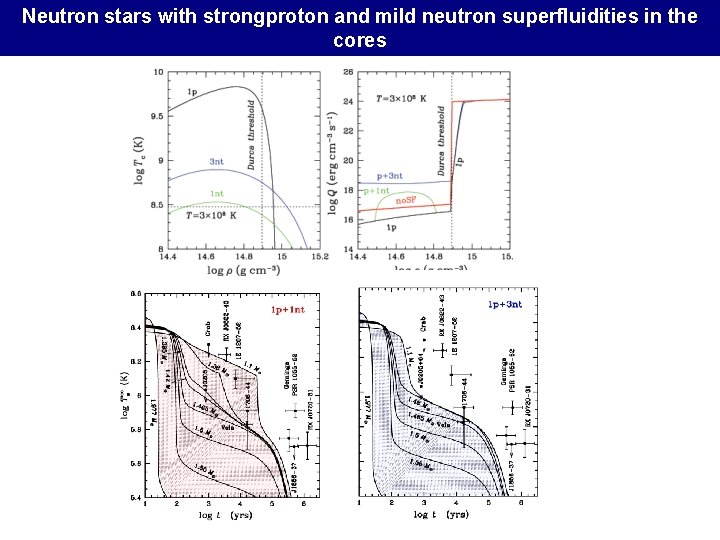
Neutron stars with strongproton and mild neutron superfluidities in the cores
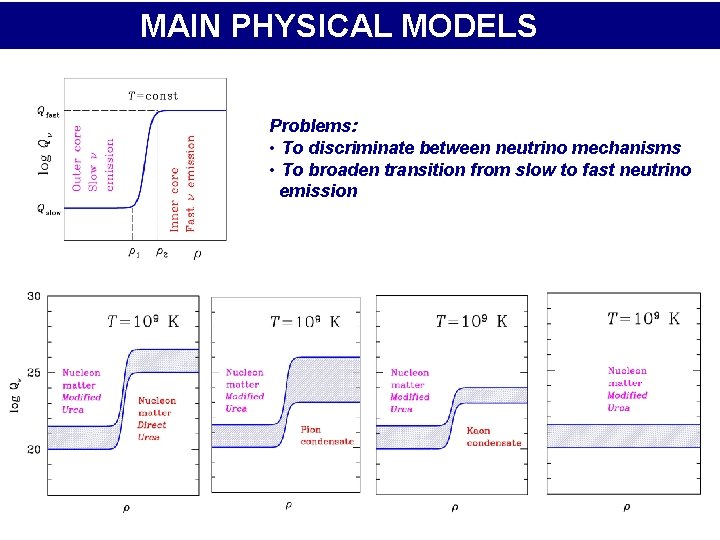
MAIN PHYSICAL MODELS Problems: • To discriminate between neutrino mechanisms • To broaden transition from slow to fast neutrino emission
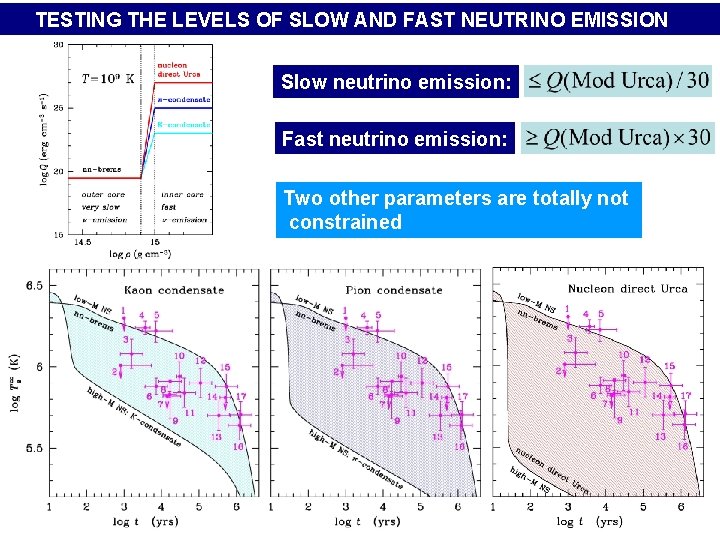
TESTING THE LEVELS OF SLOW AND FAST NEUTRINO EMISSION Slow neutrino emission: Fast neutrino emission: Two other parameters are totally not constrained
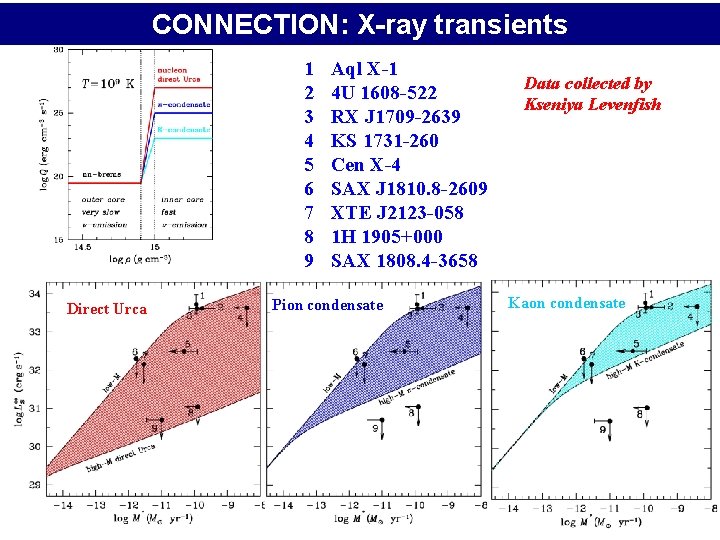
CONNECTION: X-ray transients 1 2 3 4 5 6 7 8 9 Direct Urca Aql X-1 4 U 1608 -522 RX J 1709 -2639 KS 1731 -260 Cen X-4 SAX J 1810. 8 -2609 XTE J 2123 -058 1 H 1905+000 SAX 1808. 4 -3658 Pion condensate Data collected by Kseniya Levenfish Kaon condensate
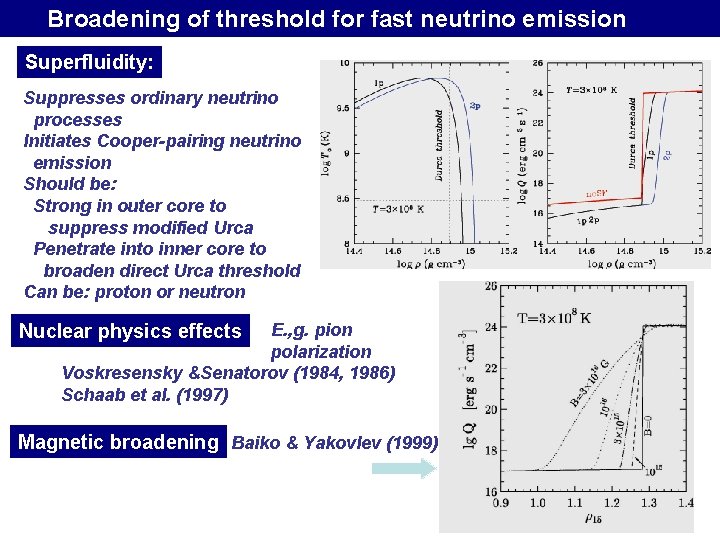
Broadening of threshold for fast neutrino emission Superfluidity: Suppresses ordinary neutrino processes Initiates Cooper-pairing neutrino emission Should be: Strong in outer core to suppress modified Urca Penetrate into inner core to broaden direct Urca threshold Can be: proton or neutron E. , g. pion polarization Voskresensky &Senatorov (1984, 1986) Schaab et al. (1997) Nuclear physics effects Magnetic broadening Baiko & Yakovlev (1999)
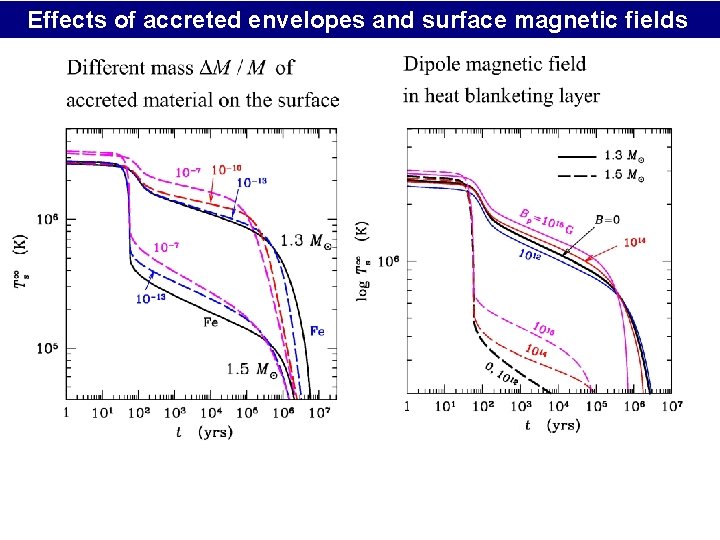
Effects of accreted envelopes and surface magnetic fields
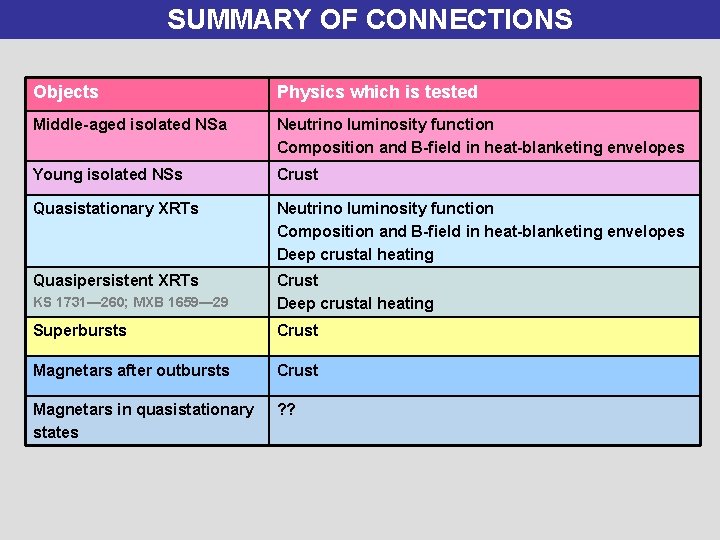
SUMMARY OF CONNECTIONS Objects Physics which is tested Middle-aged isolated NSa Neutrino luminosity function Composition and B-field in heat-blanketing envelopes Young isolated NSs Crust Quasistationary XRTs Neutrino luminosity function Composition and B-field in heat-blanketing envelopes Deep crustal heating Quasipersistent XRTs KS 1731— 260; MXB 1659— 29 Crust Deep crustal heating Superbursts Crust Magnetars after outbursts Crust Magnetars in quasistationary states ? ?
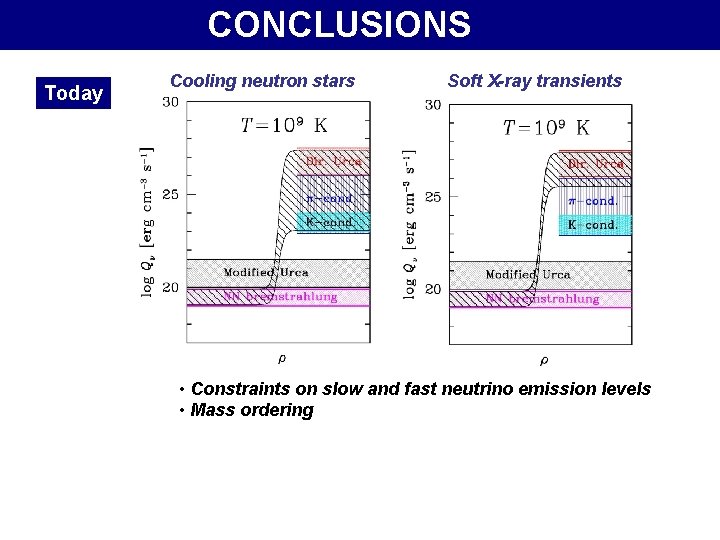
CONCLUSIONS Today Cooling neutron stars Soft X-ray transients • Constraints on slow and fast neutrino emission levels • Mass ordering
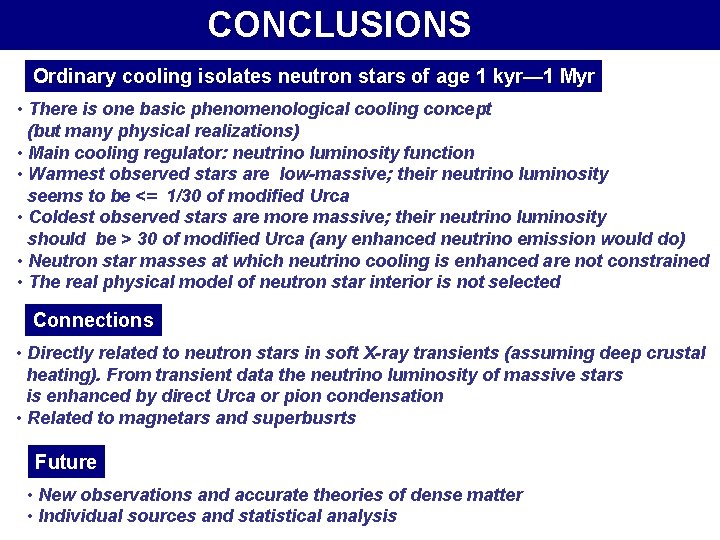
CONCLUSIONS Ordinary cooling isolates neutron stars of age 1 kyr— 1 Myr • There is one basic phenomenological cooling concept (but many physical realizations) • Main cooling regulator: neutrino luminosity function • Warmest observed stars are low-massive; their neutrino luminosity seems to be <= 1/30 of modified Urca • Coldest observed stars are more massive; their neutrino luminosity should be > 30 of modified Urca (any enhanced neutrino emission would do) • Neutron star masses at which neutrino cooling is enhanced are not constrained • The real physical model of neutron star interior is not selected Connections • Directly related to neutron stars in soft X-ray transients (assuming deep crustal heating). From transient data the neutrino luminosity of massive stars is enhanced by direct Urca or pion condensation • Related to magnetars and superbusrts Future • New observations and accurate theories of dense matter • Individual sources and statistical analysis
Venera 14 images
Ioffe
Ioffe
Ioffe
How are neutrons formed
There are millions of stars in
Mirjam van daalen
Goldstein adalah penemu
Neutron activation analysis
Binary neutron star
Masa del neutron
Cns summer school
Cipher neutron
Parity of neutron
Neutron skin
Co 60
Neutron star
What element
Magnets for neutron diffraction
Timi neutron
Chlorine neutron
Which scientist discovered neutron
Symbole proton neutron electron
Neutron star
Neutron labs w07
Neutron star router
Neutron activation analysis
Difference between nuclear reaction and chemical reaction
Lithium number of protons
Symbols of subatomic particles
Neutron fission
Dis off