Collective phenomena in condensed matter systems Outline Lectures
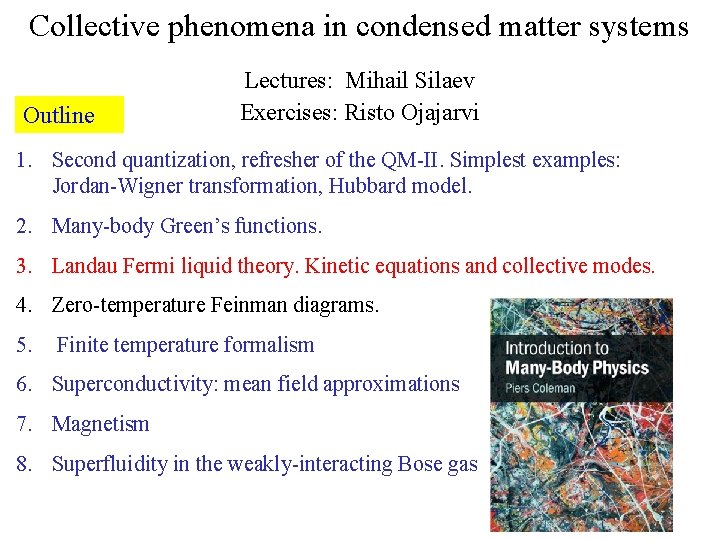
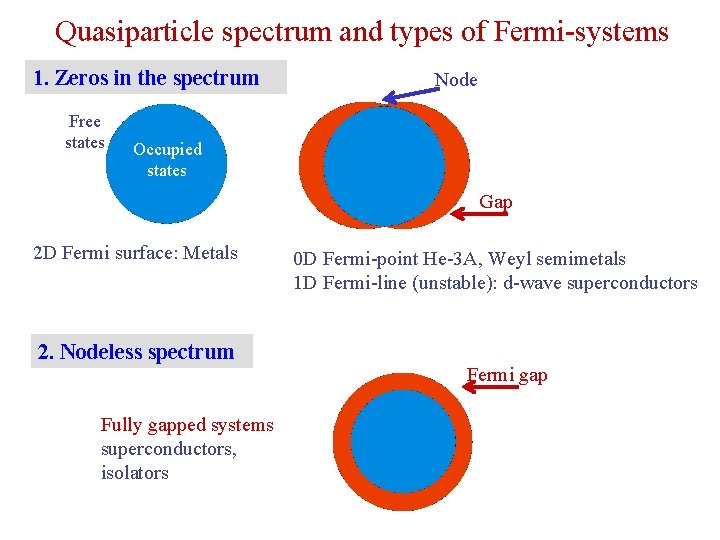
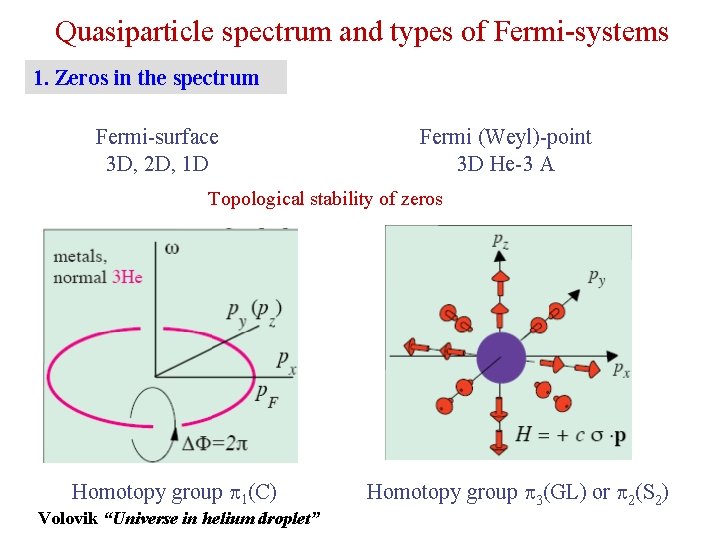
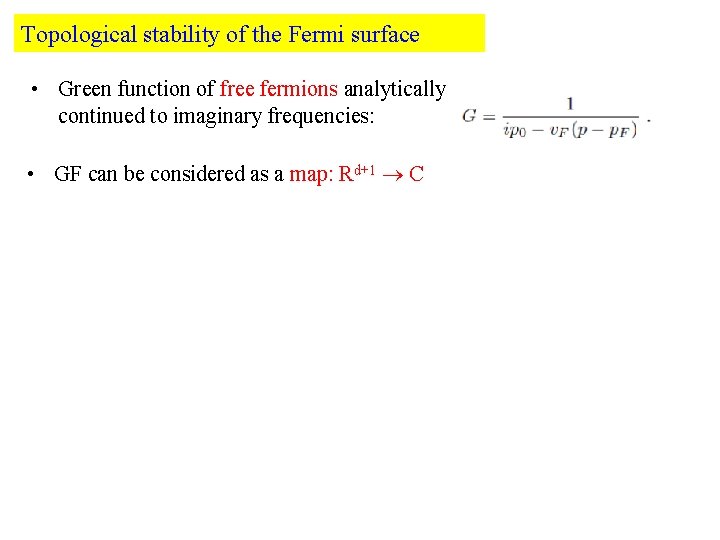
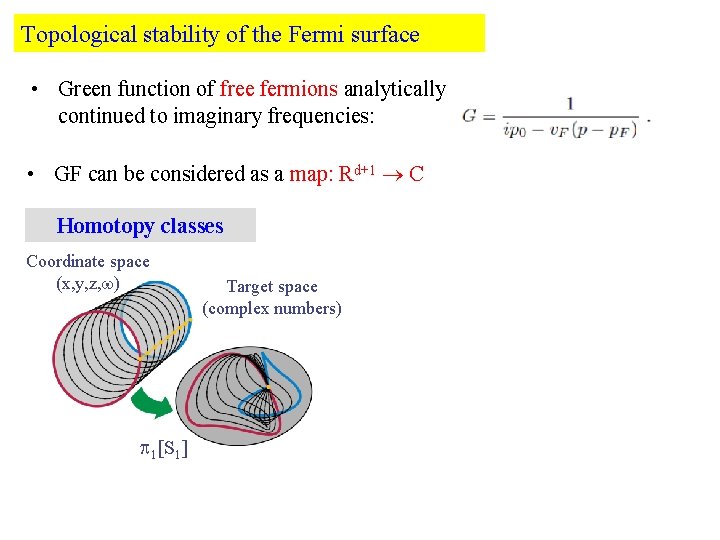
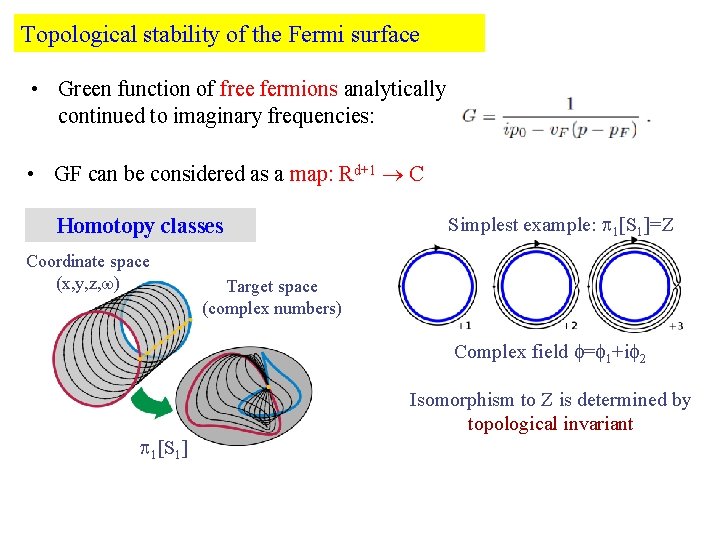
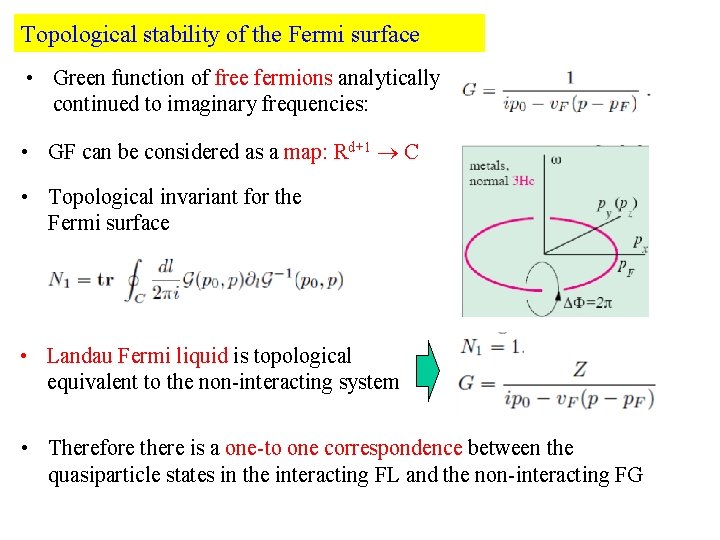
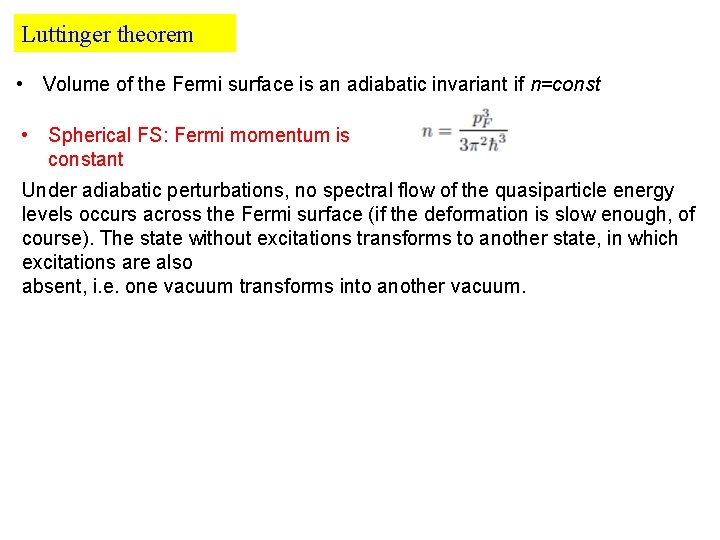
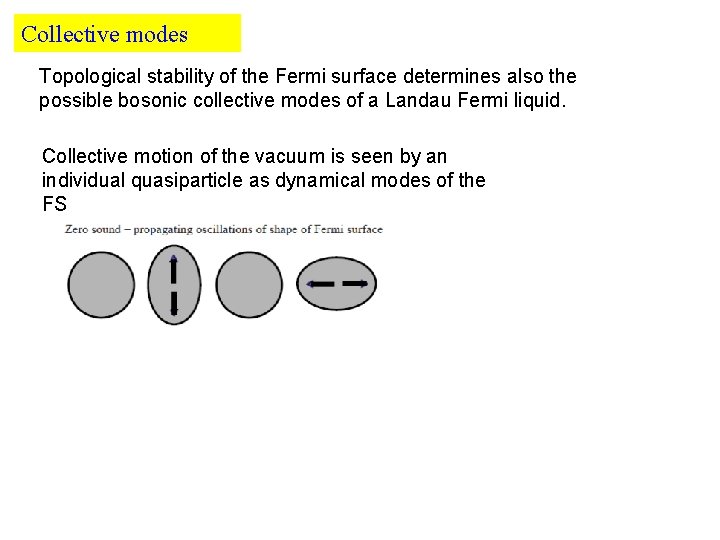
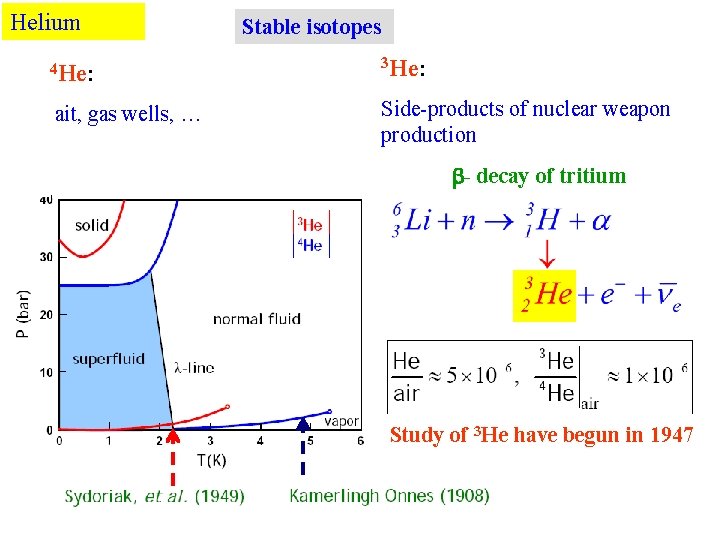
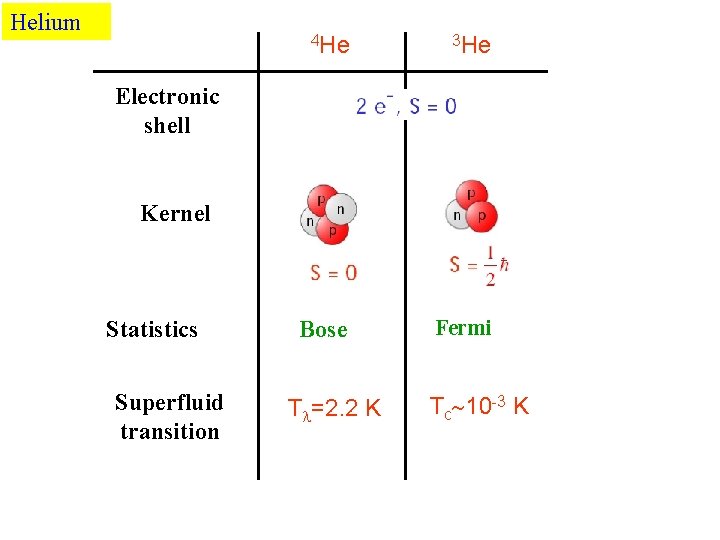
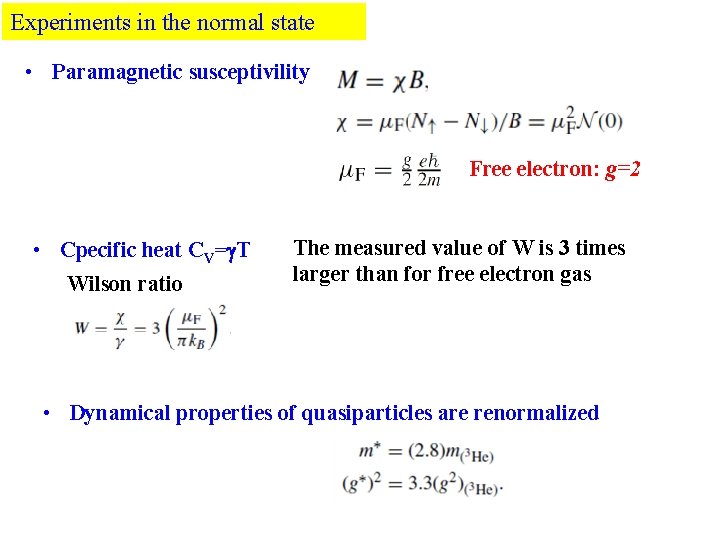
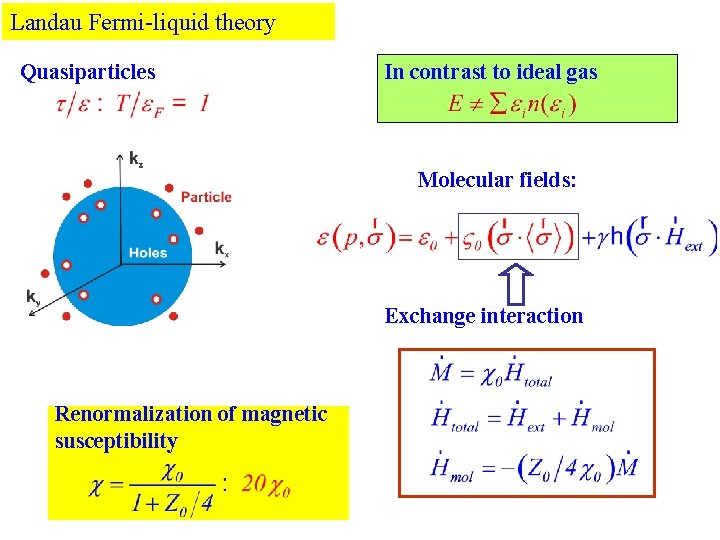
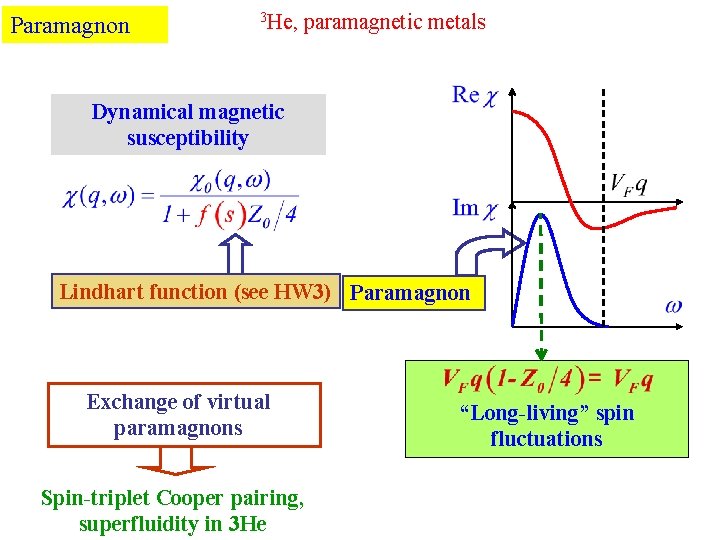
- Slides: 14
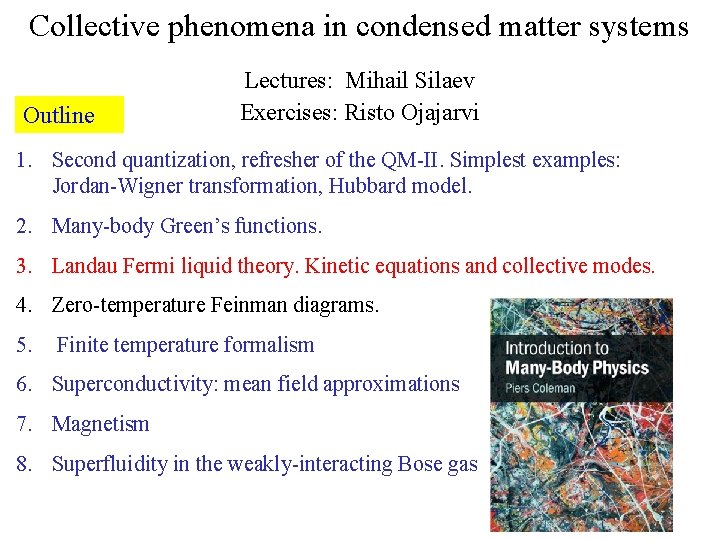
Collective phenomena in condensed matter systems Outline Lectures: Mihail Silaev Exercises: Risto Ojajarvi 1. Second quantization, refresher of the QM-II. Simplest examples: Jordan-Wigner transformation, Hubbard model. 2. Many-body Green’s functions. 3. Landau Fermi liquid theory. Kinetic equations and collective modes. 4. Zero-temperature Feinman diagrams. 5. Finite temperature formalism 6. Superconductivity: mean field approximations 7. Magnetism 8. Superfluidity in the weakly-interacting Bose gas
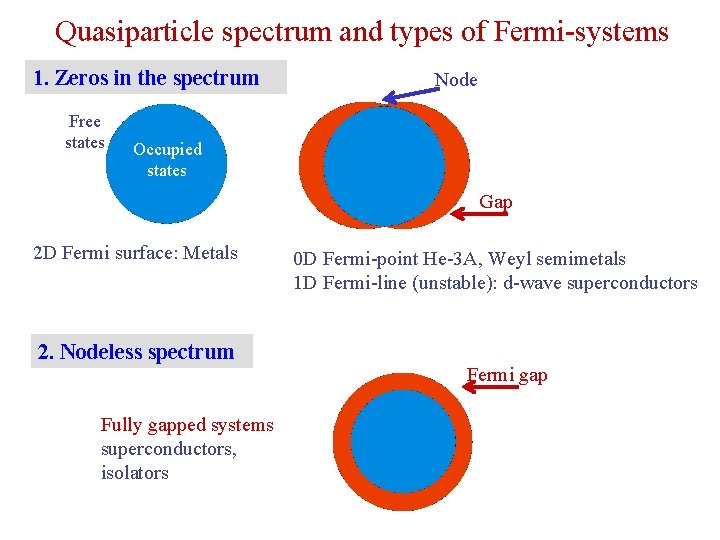
Quasiparticle spectrum and types of Fermi-systems 1. Zeros in the spectrum Free states Node Occupied states Gap 2 D Fermi surface: Metals 2. Nodeless spectrum Fully gapped systems superconductors, isolators 0 D Fermi-point He-3 А, Weyl semimetals 1 D Fermi-line (unstable): d-wave superconductors Fermi gap
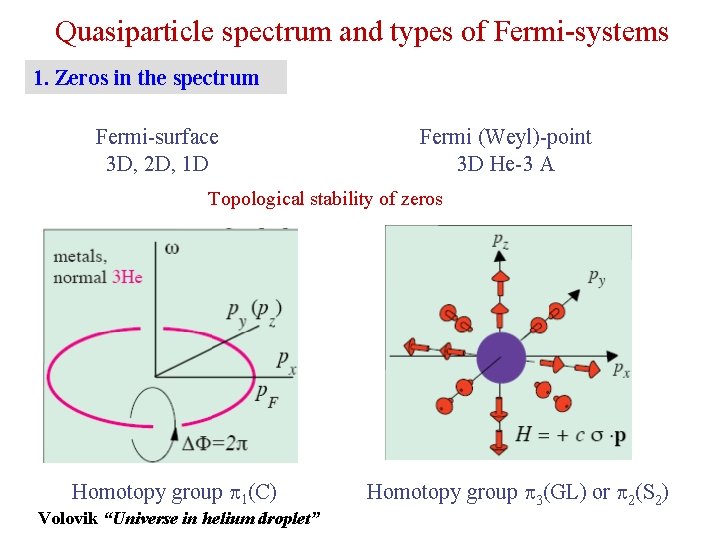
Quasiparticle spectrum and types of Fermi-systems 1. Zeros in the spectrum Fermi-surface 3 D, 2 D, 1 D Fermi (Weyl)-point 3 D He-3 А Topological stability of zeros Homotopy group 1(C) Volovik “Universe in helium droplet” Homotopy group 3(GL) or 2(S 2)
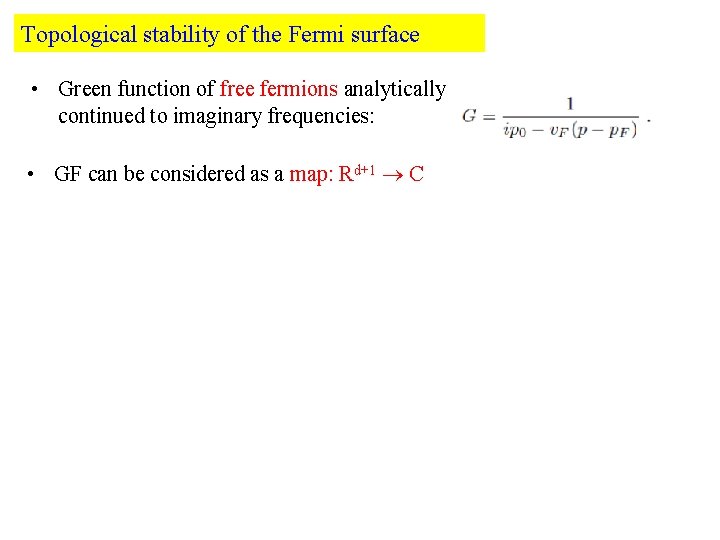
Topological stability of the Fermi surface • Green function of free fermions analytically continued to imaginary frequencies: • GF can be considered as a map: Rd+1 C
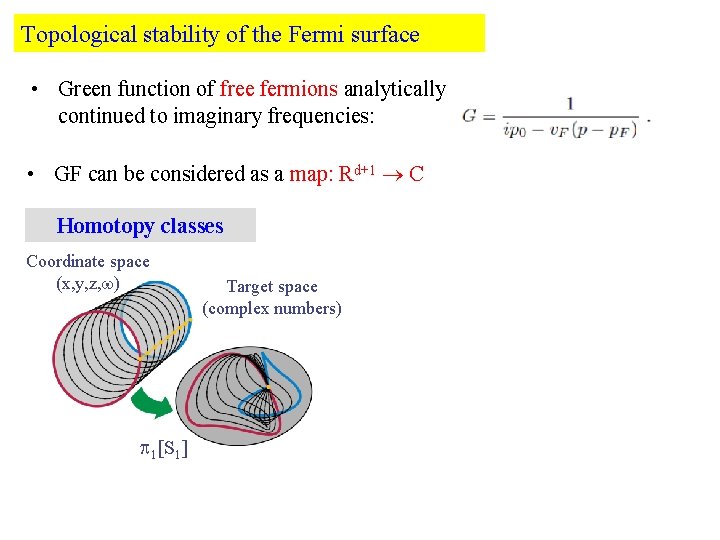
Topological stability of the Fermi surface • Green function of free fermions analytically continued to imaginary frequencies: • GF can be considered as a map: Rd+1 C Homotopy classes Coordinate space (x, y, z, ) 1[S 1] Target space (complex numbers)
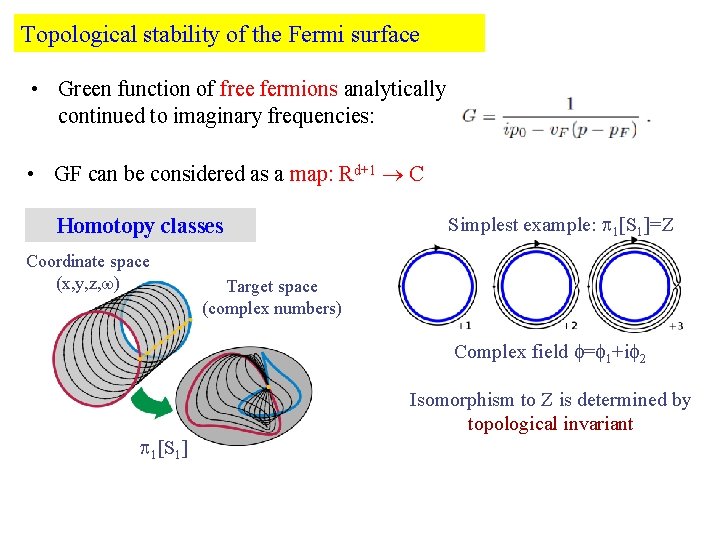
Topological stability of the Fermi surface • Green function of free fermions analytically continued to imaginary frequencies: • GF can be considered as a map: Rd+1 C Homotopy classes Coordinate space (x, y, z, ) Simplest example: 1[S 1]=Z Target space (complex numbers) Complex field = 1+i 2 1[S 1] Isomorphism to Z is determined by topological invariant
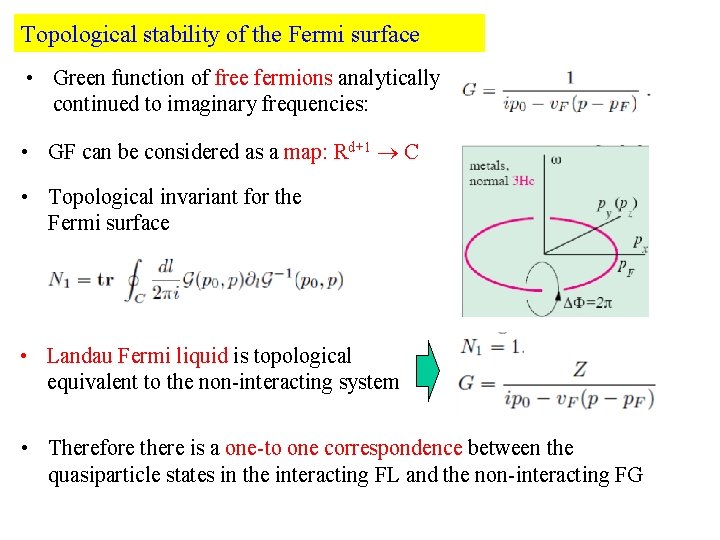
Topological stability of the Fermi surface • Green function of free fermions analytically continued to imaginary frequencies: • GF can be considered as a map: Rd+1 C • Topological invariant for the Fermi surface • Landau Fermi liquid is topological equivalent to the non-interacting system • Therefore there is a one-to one correspondence between the quasiparticle states in the interacting FL and the non-interacting FG
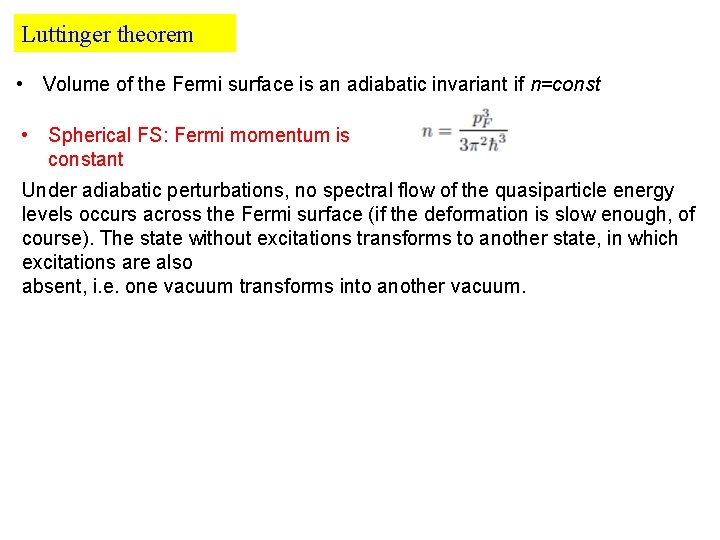
Luttinger theorem • Volume of the Fermi surface is an adiabatic invariant if n=const • Spherical FS: Fermi momentum is constant Under adiabatic perturbations, no spectral flow of the quasiparticle energy levels occurs across the Fermi surface (if the deformation is slow enough, of course). The state without excitations transforms to another state, in which excitations are also absent, i. e. one vacuum transforms into another vacuum.
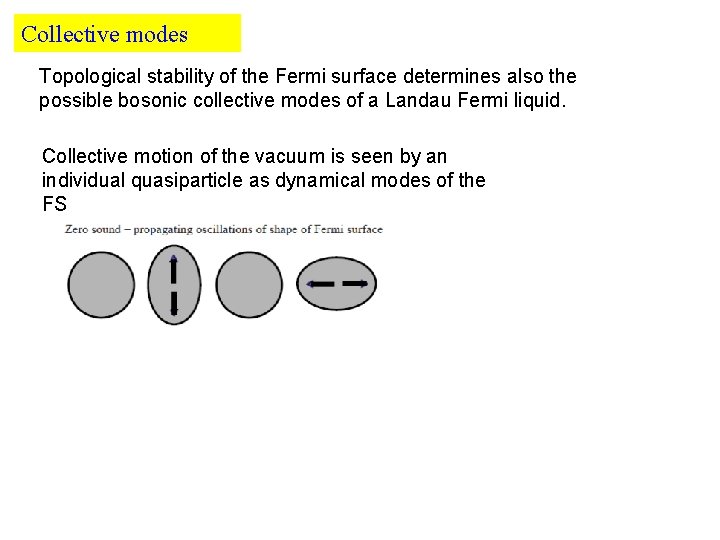
Collective modes Topological stability of the Fermi surface determines also the possible bosonic collective modes of a Landau Fermi liquid. Collective motion of the vacuum is seen by an individual quasiparticle as dynamical modes of the FS
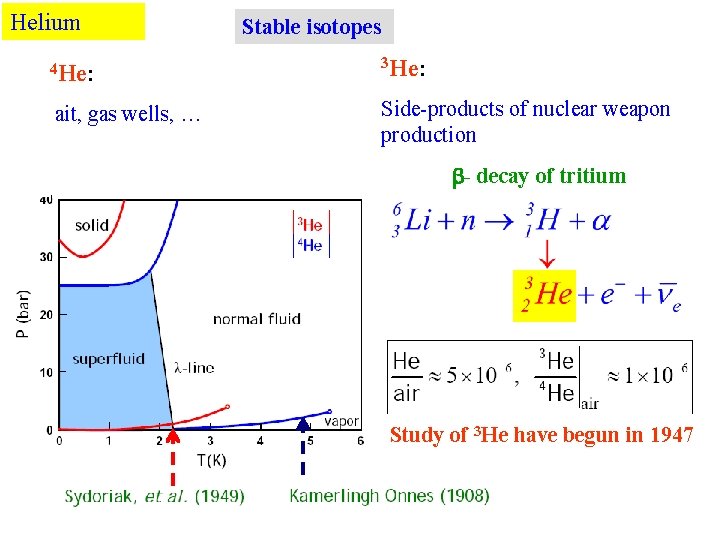
Helium 4 He: ait, gas wells, … Stable isotopes 3 He: Side-products of nuclear weapon production - decay of tritium Study of 3 He have begun in 1947
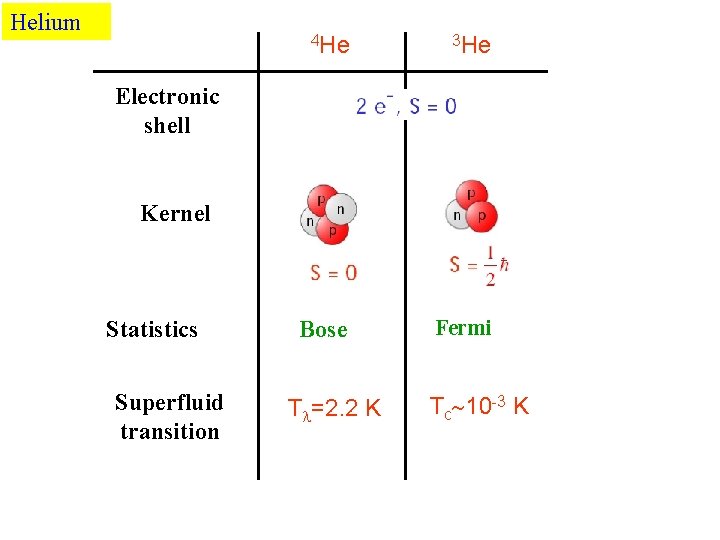
Helium 4 He 3 He Bose Fermi Electronic shell Kernel Statistics Superfluid transition T =2. 2 K Tc 10 -3 K
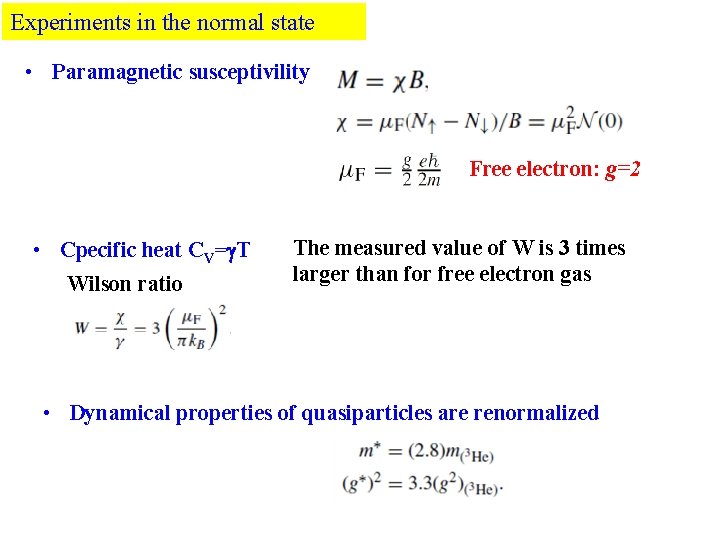
Experiments in the normal state • Paramagnetic susceptivility Free electron: g=2 • Cpecific heat CV= T Wilson ratio The measured value of W is 3 times larger than for free electron gas • Dynamical properties of quasiparticles are renormalized
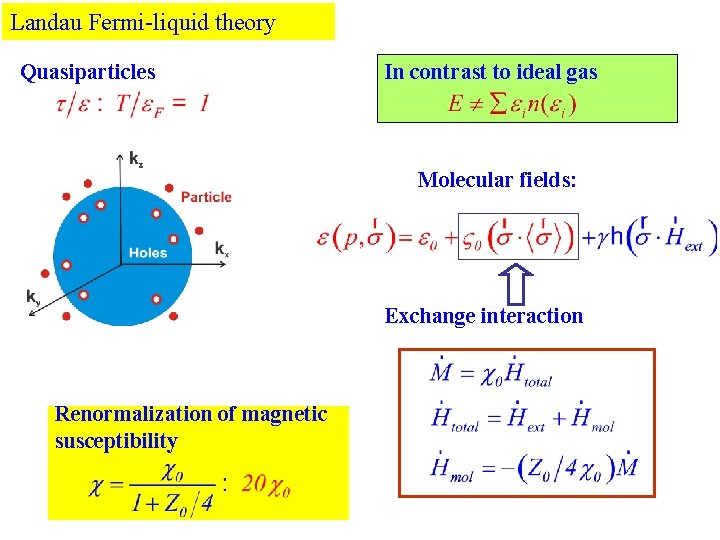
Landau Fermi-liquid theory Quasiparticles In contrast to ideal gas Molecular fields: Exchange interaction Renormalization of magnetic susceptibility
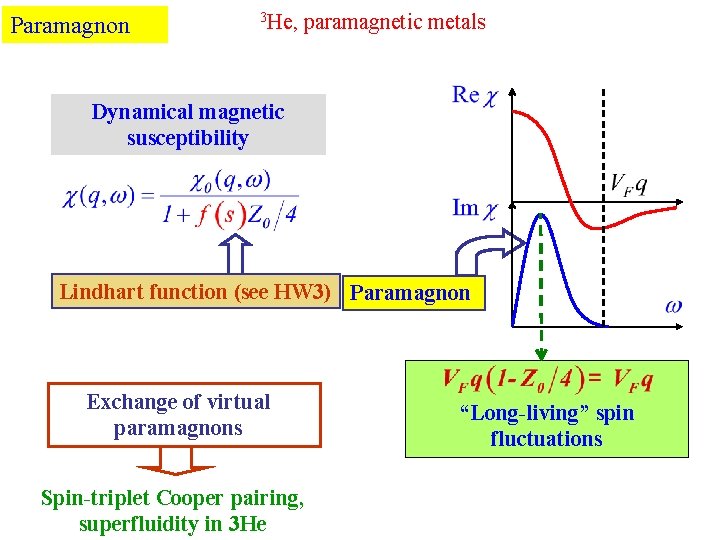
Paramagnon 3 He, paramagnetic metals Dynamical magnetic susceptibility Lindhart function (see HW 3) Paramagnon Exchange of virtual paramagnons Spin-triplet Cooper pairing, superfluidity in 3 He “Long-living” spin fluctuations
Condensed matter theory center
Classification of matter section 1 composition of matter
White matter of brain
Composition of matter section 1
Chapter 2 section 1 classifying matter answer key
Cerebral aqueduct
Section 1 composition of matter
Gray matter and white matter
Grey matter and white matter in brain
Ecological succession
Evidence sandwich example
Phenomenon monikko
Ppt on some natural phenomena
Physical and chemical phenomena
Phenomena related to refraction