Chapter 2 The Mammalian Oocyte 2015 Elsevier Inc
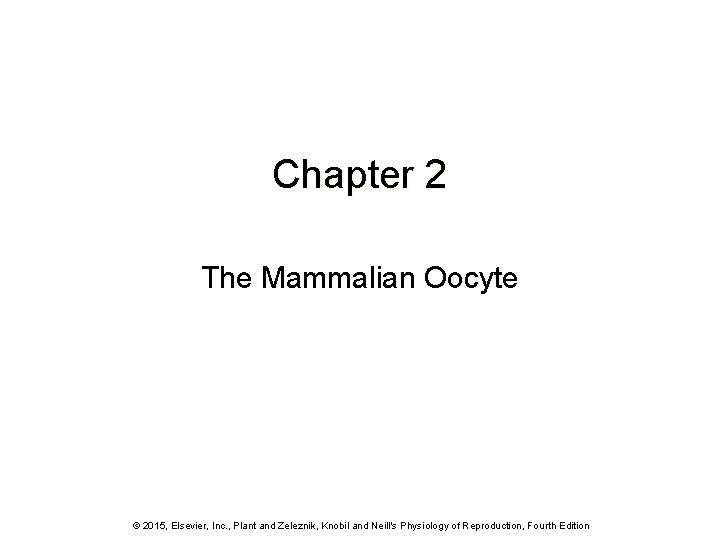
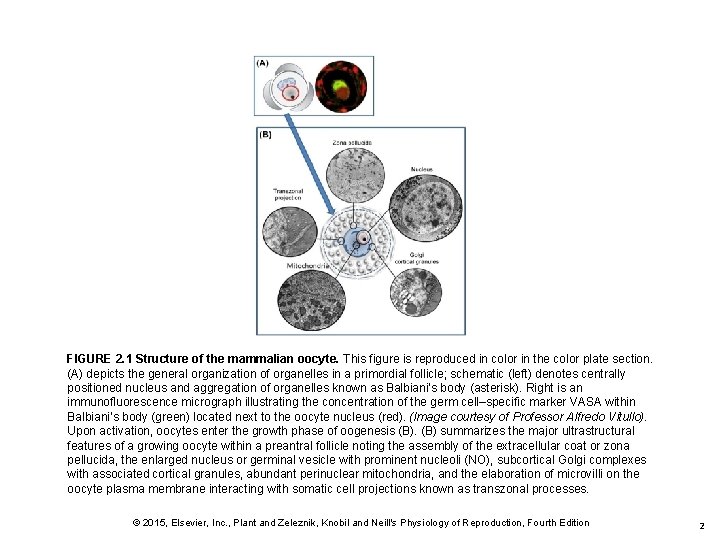
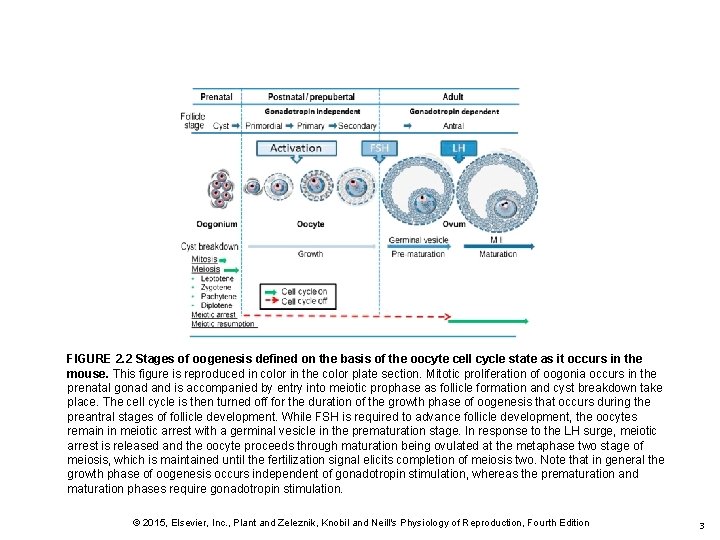
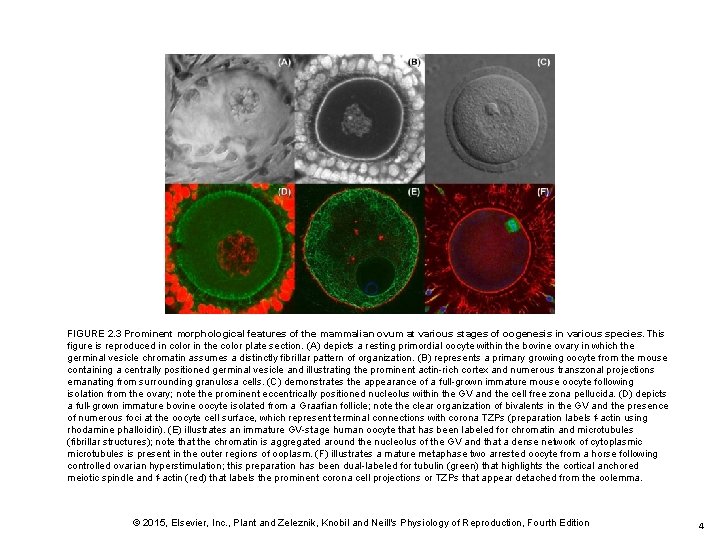
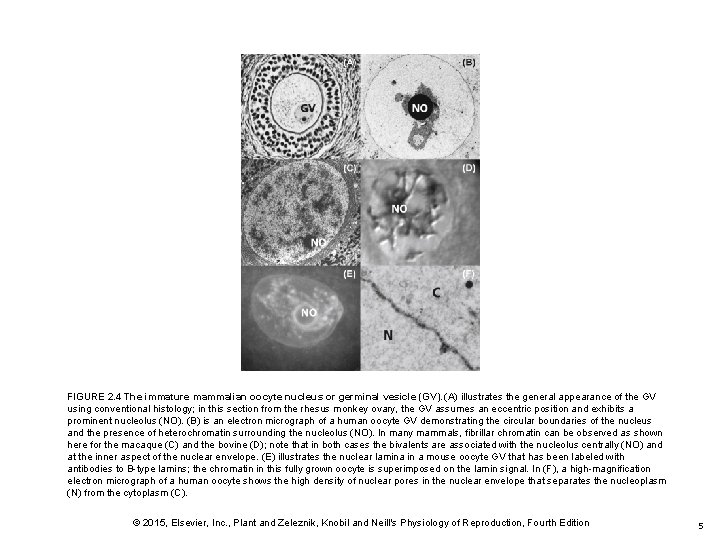
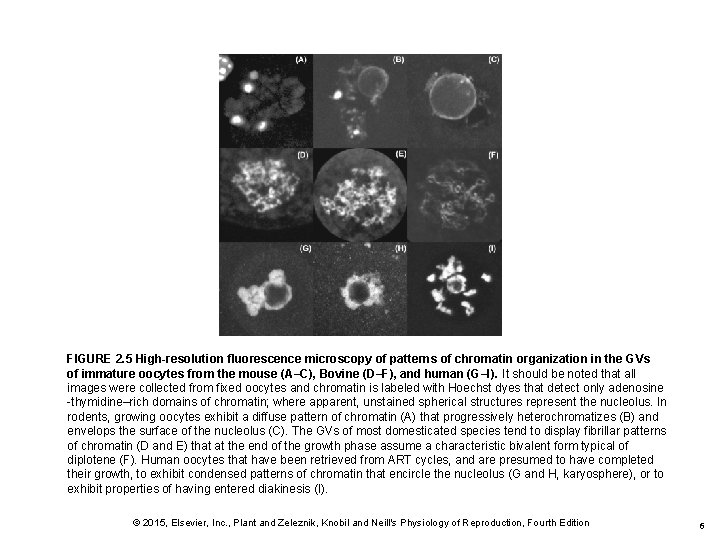
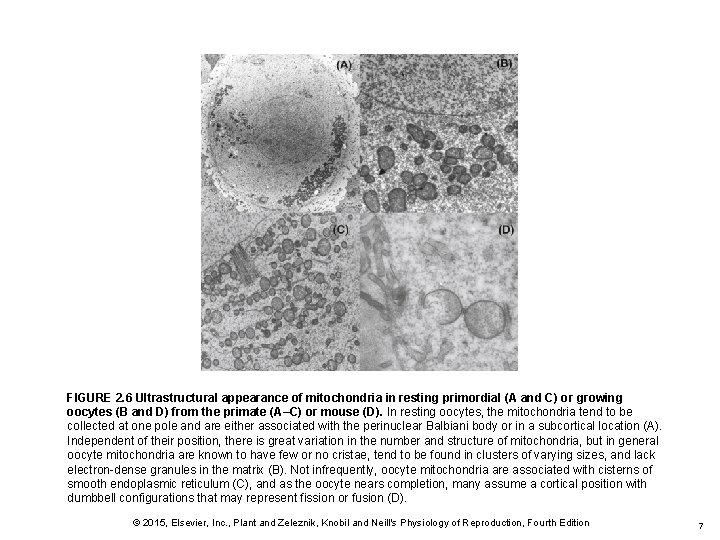
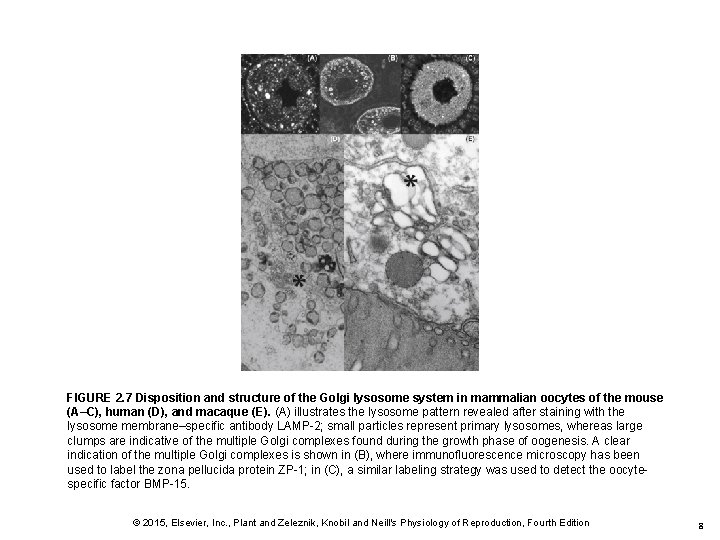
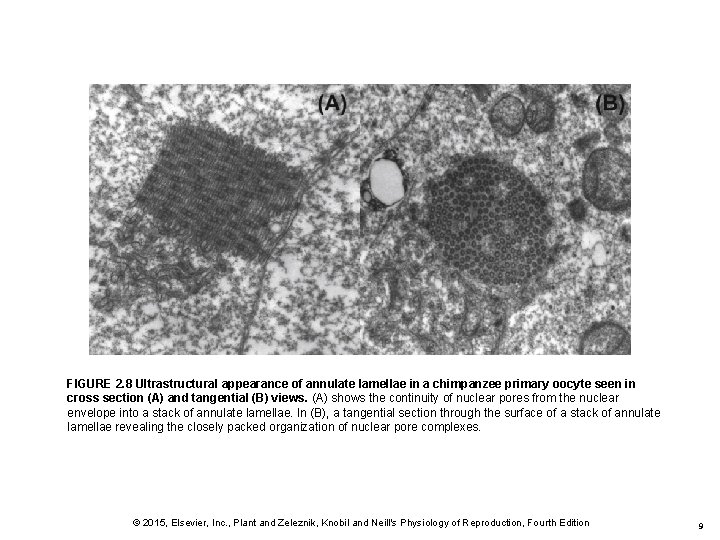
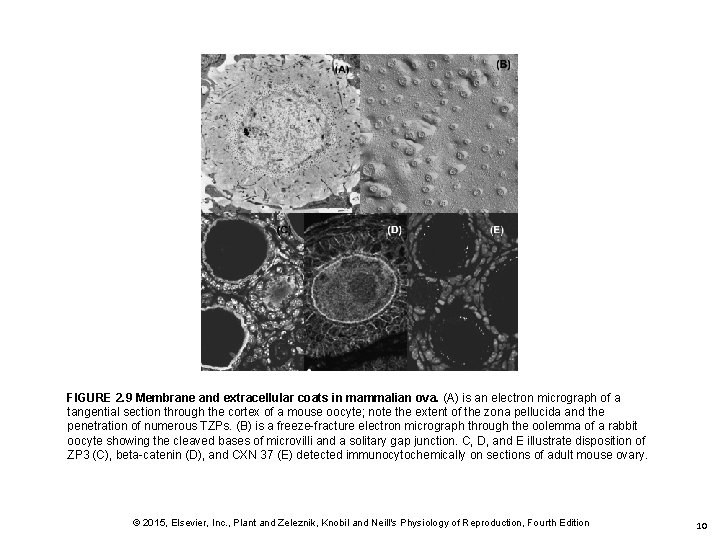
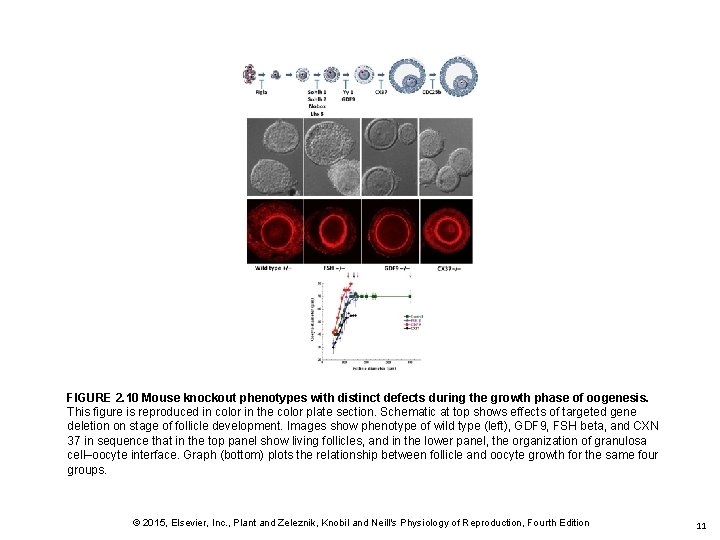
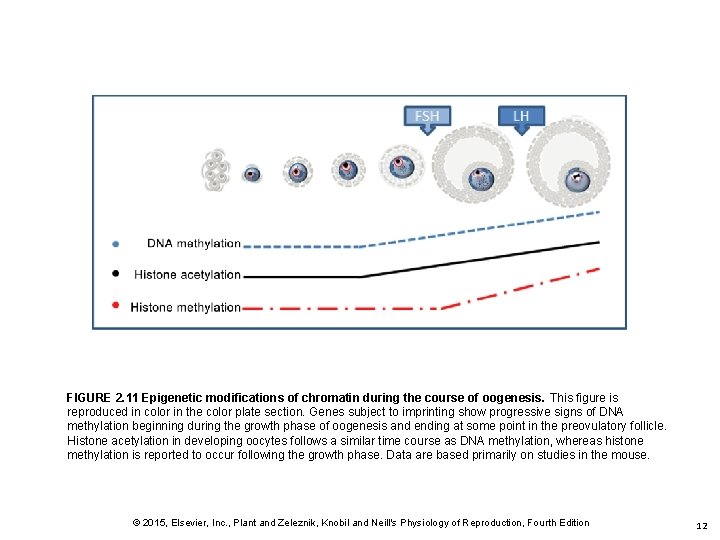
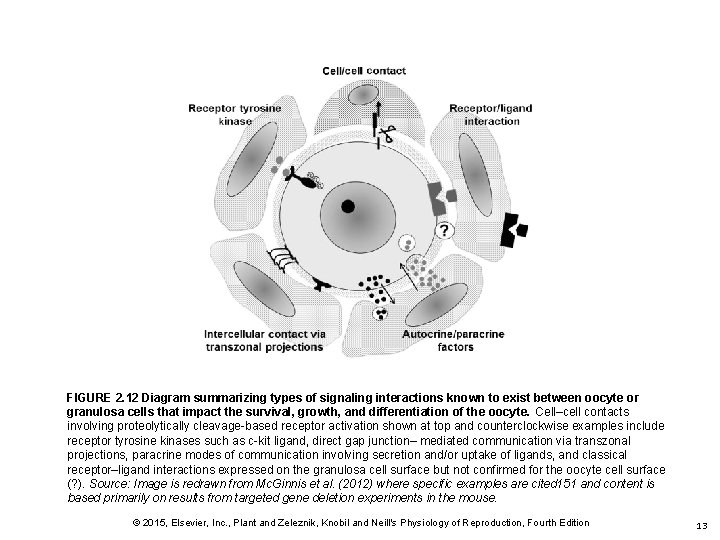
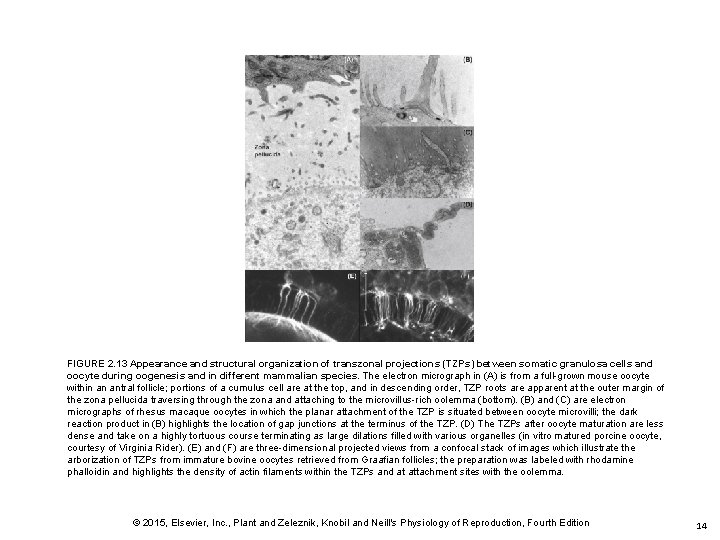
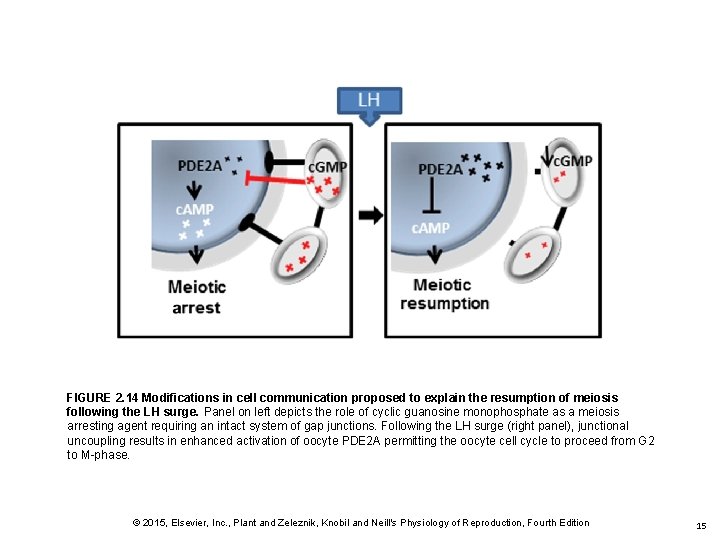
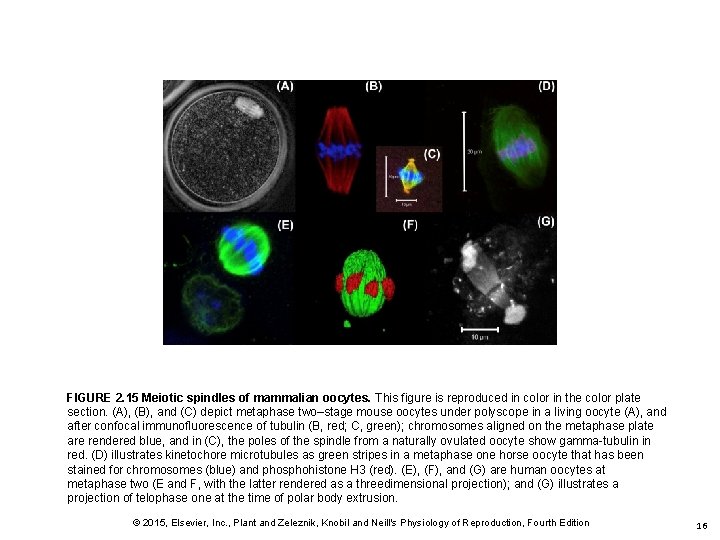
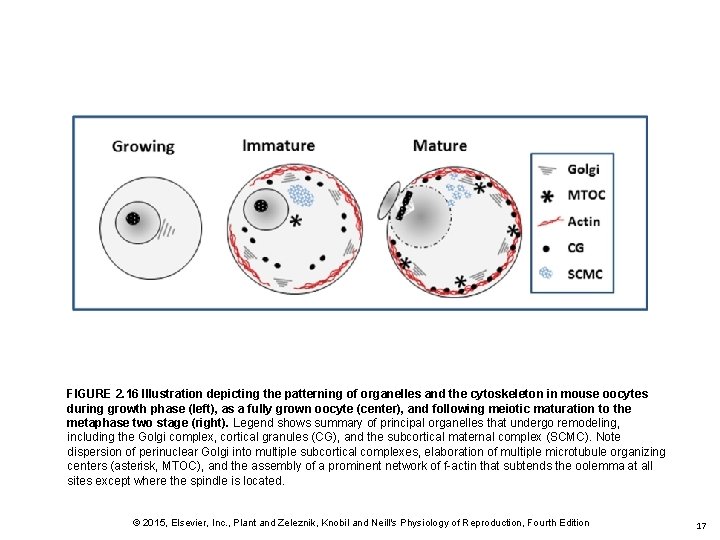
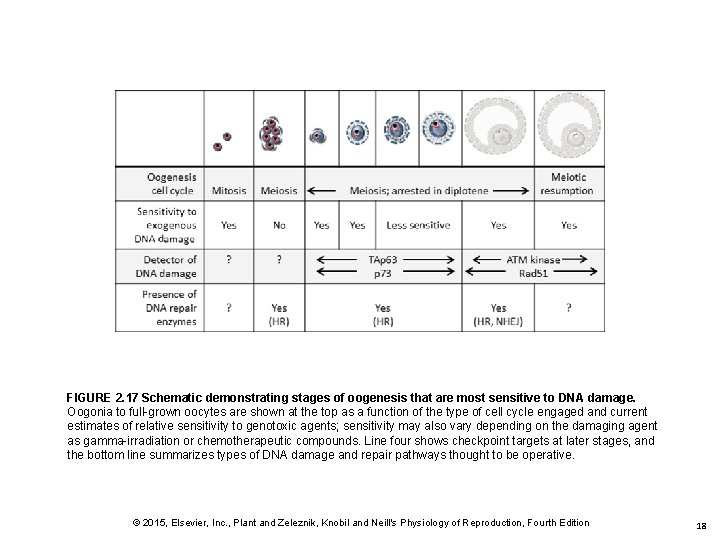
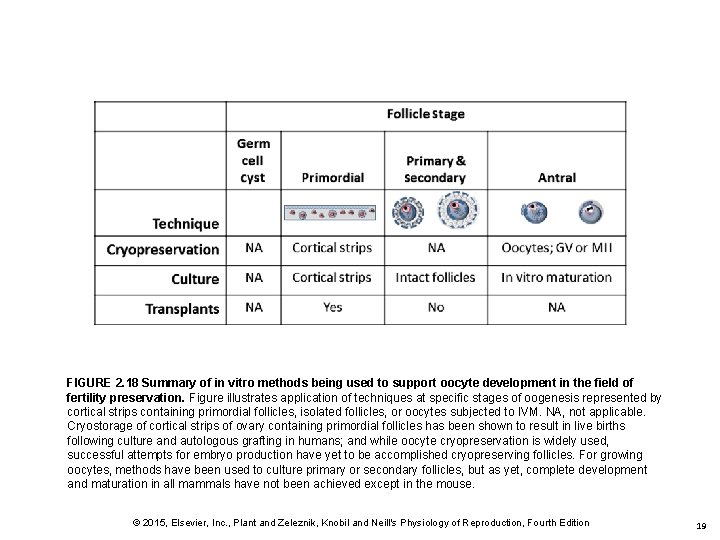
- Slides: 19
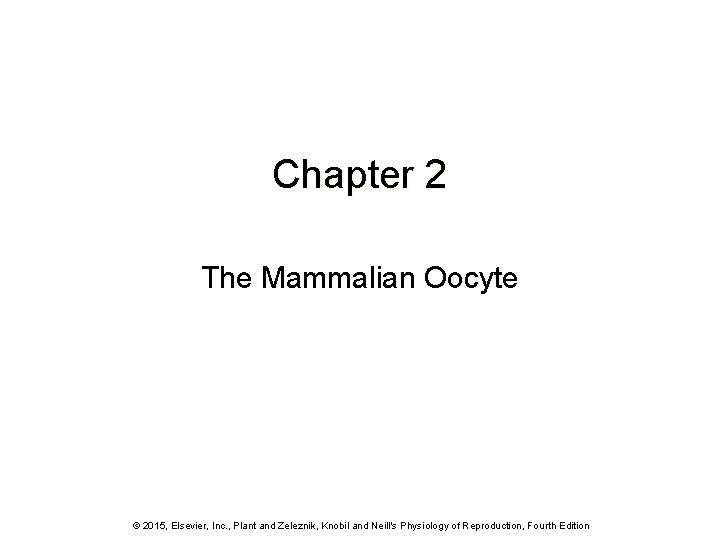
Chapter 2 The Mammalian Oocyte © 2015, Elsevier, Inc. , Plant and Zeleznik, Knobil and Neill's Physiology of Reproduction, Fourth Edition
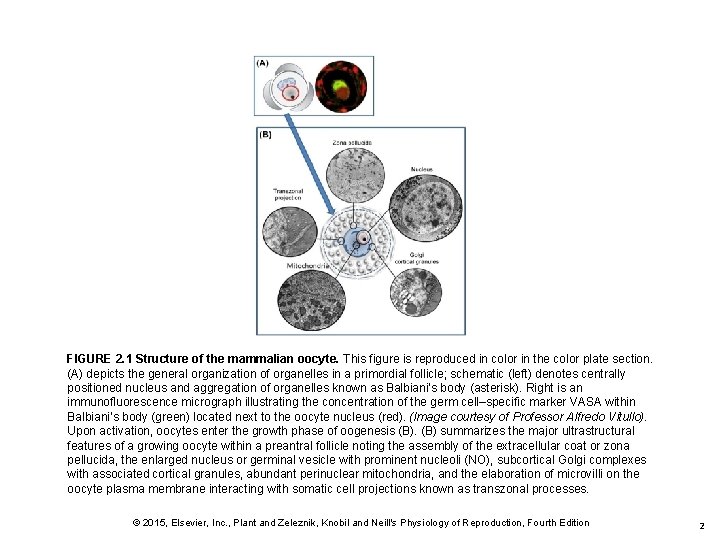
FIGURE 2. 1 Structure of the mammalian oocyte. This figure is reproduced in color in the color plate section. (A) depicts the general organization of organelles in a primordial follicle; schematic (left) denotes centrally positioned nucleus and aggregation of organelles known as Balbiani’s body (asterisk). Right is an immunofluorescence micrograph illustrating the concentration of the germ cell–specific marker VASA within Balbiani’s body (green) located next to the oocyte nucleus (red). (Image courtesy of Professor Alfredo Vitullo). Upon activation, oocytes enter the growth phase of oogenesis (B) summarizes the major ultrastructural features of a growing oocyte within a preantral follicle noting the assembly of the extracellular coat or zona pellucida, the enlarged nucleus or germinal vesicle with prominent nucleoli (NO), subcortical Golgi complexes with associated cortical granules, abundant perinuclear mitochondria, and the elaboration of microvilli on the oocyte plasma membrane interacting with somatic cell projections known as transzonal processes. © 2015, Elsevier, Inc. , Plant and Zeleznik, Knobil and Neill's Physiology of Reproduction, Fourth Edition 2
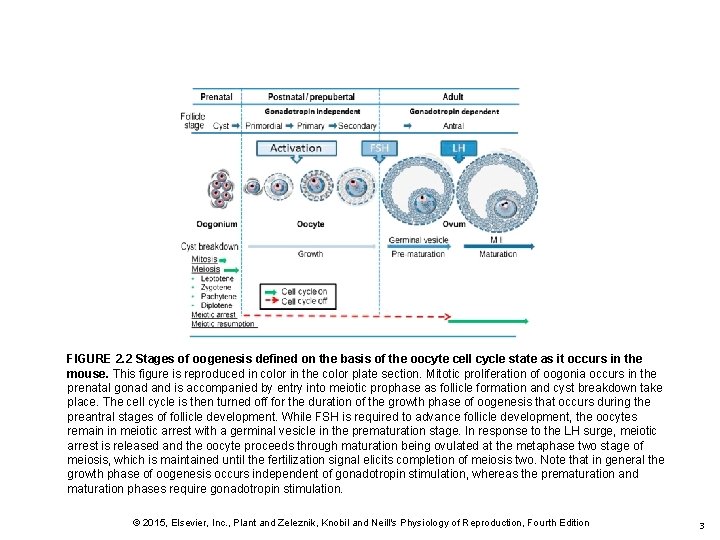
FIGURE 2. 2 Stages of oogenesis defined on the basis of the oocyte cell cycle state as it occurs in the mouse. This figure is reproduced in color in the color plate section. Mitotic proliferation of oogonia occurs in the prenatal gonad and is accompanied by entry into meiotic prophase as follicle formation and cyst breakdown take place. The cell cycle is then turned off for the duration of the growth phase of oogenesis that occurs during the preantral stages of follicle development. While FSH is required to advance follicle development, the oocytes remain in meiotic arrest with a germinal vesicle in the prematuration stage. In response to the LH surge, meiotic arrest is released and the oocyte proceeds through maturation being ovulated at the metaphase two stage of meiosis, which is maintained until the fertilization signal elicits completion of meiosis two. Note that in general the growth phase of oogenesis occurs independent of gonadotropin stimulation, whereas the prematuration and maturation phases require gonadotropin stimulation. © 2015, Elsevier, Inc. , Plant and Zeleznik, Knobil and Neill's Physiology of Reproduction, Fourth Edition 3
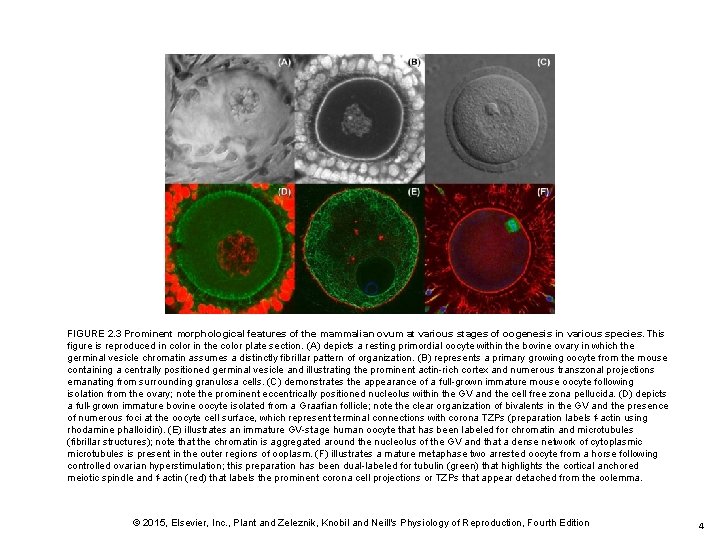
FIGURE 2. 3 Prominent morphological features of the mammalian ovum at various stages of oogenesis in various species. This figure is reproduced in color in the color plate section. (A) depicts a resting primordial oocyte within the bovine ovary in which the germinal vesicle chromatin assumes a distinctly fibrillar pattern of organization. (B) represents a primary growing oocyte from the mouse containing a centrally positioned germinal vesicle and illustrating the prominent actin-rich cortex and numerous transzonal projections emanating from surrounding granulosa cells. (C) demonstrates the appearance of a full-grown immature mouse oocyte following isolation from the ovary; note the prominent eccentrically positioned nucleolus within the GV and the cell free zona pellucida. (D) depicts a full-grown immature bovine oocyte isolated from a Graafian follicle; note the clear organization of bivalents in the GV and the presence of numerous foci at the oocyte cell surface, which represent terminal connections with corona TZPs (preparation labels f-actin using rhodamine phalloidin). (E) illustrates an immature GV-stage human oocyte that has been labeled for chromatin and microtubules (fibrillar structures); note that the chromatin is aggregated around the nucleolus of the GV and that a dense network of cytoplasmic microtubules is present in the outer regions of ooplasm. (F) illustrates a mature metaphase two arrested oocyte from a horse following controlled ovarian hyperstimulation; this preparation has been dual-labeled for tubulin (green) that highlights the cortical anchored meiotic spindle and f-actin (red) that labels the prominent corona cell projections or TZPs that appear detached from the oolemma. © 2015, Elsevier, Inc. , Plant and Zeleznik, Knobil and Neill's Physiology of Reproduction, Fourth Edition 4
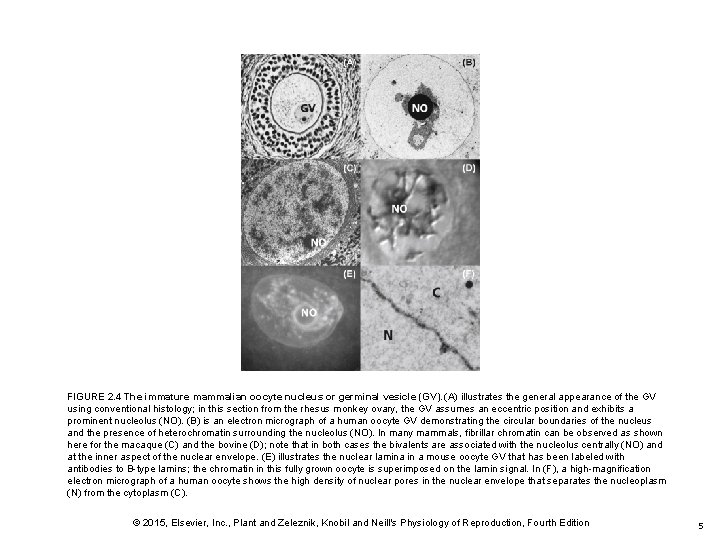
FIGURE 2. 4 The immature mammalian oocyte nucleus or germinal vesicle (GV). (A) illustrates the general appearance of the GV using conventional histology; in this section from the rhesus monkey ovary, the GV assumes an eccentric position and exhibits a prominent nucleolus (NO). (B) is an electron micrograph of a human oocyte GV demonstrating the circular boundaries of the nucleus and the presence of heterochromatin surrounding the nucleolus (NO). In many mammals, fibrillar chromatin can be observed as shown here for the macaque (C) and the bovine (D); note that in both cases the bivalents are associated with the nucleolus centrally (NO) and at the inner aspect of the nuclear envelope. (E) illustrates the nuclear lamina in a mouse oocyte GV that has been labeled with antibodies to B-type lamins; the chromatin in this fully grown oocyte is superimposed on the lamin signal. In (F), a high-magnification electron micrograph of a human oocyte shows the high density of nuclear pores in the nuclear envelope that separates the nucleoplasm (N) from the cytoplasm (C). © 2015, Elsevier, Inc. , Plant and Zeleznik, Knobil and Neill's Physiology of Reproduction, Fourth Edition 5
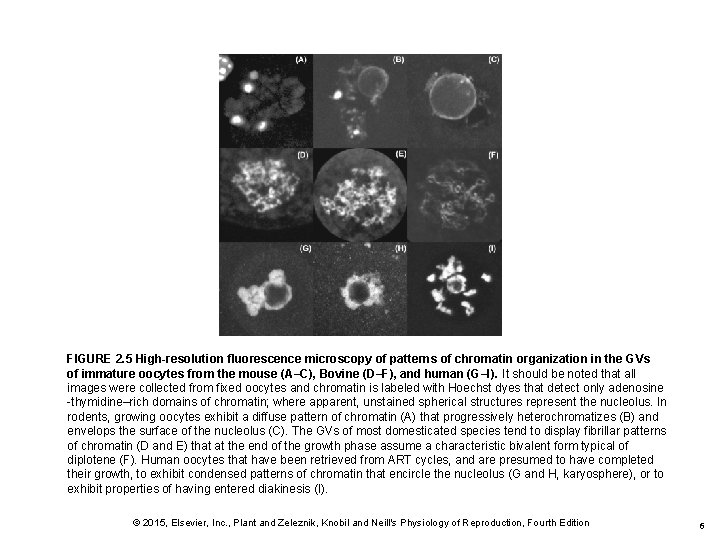
FIGURE 2. 5 High-resolution fluorescence microscopy of patterns of chromatin organization in the GVs of immature oocytes from the mouse (A–C), Bovine (D–F), and human (G–I). It should be noted that all images were collected from fixed oocytes and chromatin is labeled with Hoechst dyes that detect only adenosine -thymidine–rich domains of chromatin; where apparent, unstained spherical structures represent the nucleolus. In rodents, growing oocytes exhibit a diffuse pattern of chromatin (A) that progressively heterochromatizes (B) and envelops the surface of the nucleolus (C). The GVs of most domesticated species tend to display fibrillar patterns of chromatin (D and E) that at the end of the growth phase assume a characteristic bivalent form typical of diplotene (F). Human oocytes that have been retrieved from ART cycles, and are presumed to have completed their growth, to exhibit condensed patterns of chromatin that encircle the nucleolus (G and H, karyosphere), or to exhibit properties of having entered diakinesis (I). © 2015, Elsevier, Inc. , Plant and Zeleznik, Knobil and Neill's Physiology of Reproduction, Fourth Edition 6
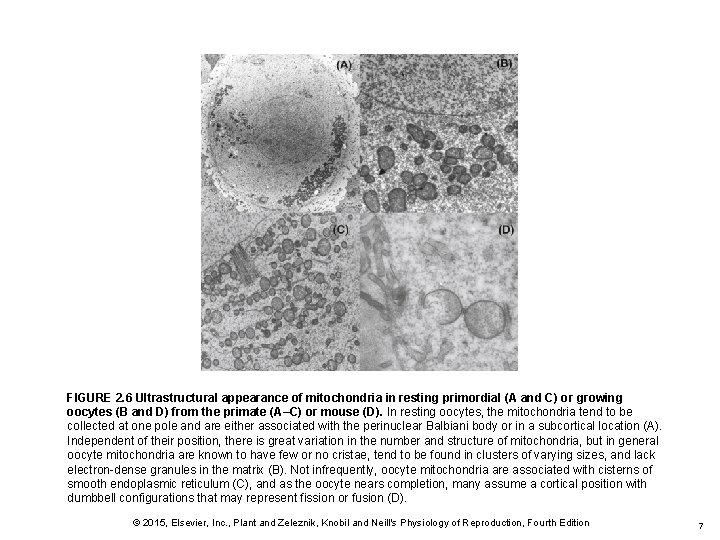
FIGURE 2. 6 Ultrastructural appearance of mitochondria in resting primordial (A and C) or growing oocytes (B and D) from the primate (A–C) or mouse (D). In resting oocytes, the mitochondria tend to be collected at one pole and are either associated with the perinuclear Balbiani body or in a subcortical location (A). Independent of their position, there is great variation in the number and structure of mitochondria, but in general oocyte mitochondria are known to have few or no cristae, tend to be found in clusters of varying sizes, and lack electron-dense granules in the matrix (B). Not infrequently, oocyte mitochondria are associated with cisterns of smooth endoplasmic reticulum (C), and as the oocyte nears completion, many assume a cortical position with dumbbell configurations that may represent fission or fusion (D). © 2015, Elsevier, Inc. , Plant and Zeleznik, Knobil and Neill's Physiology of Reproduction, Fourth Edition 7
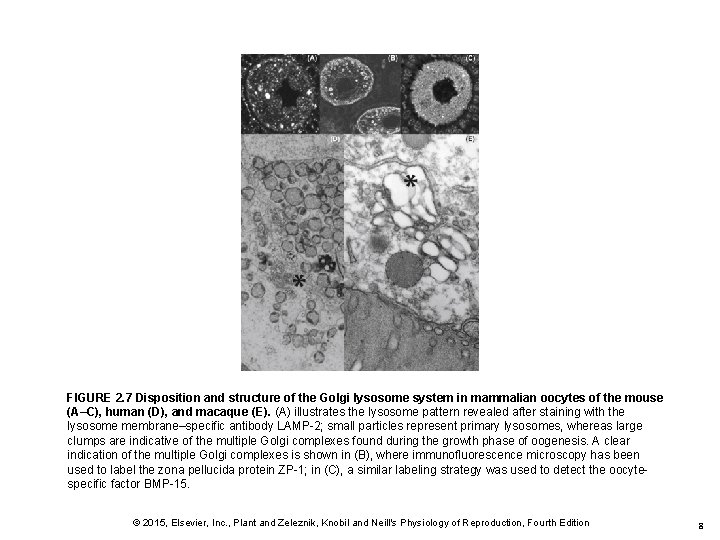
FIGURE 2. 7 Disposition and structure of the Golgi lysosome system in mammalian oocytes of the mouse (A–C), human (D), and macaque (E). (A) illustrates the lysosome pattern revealed after staining with the lysosome membrane–specific antibody LAMP-2; small particles represent primary lysosomes, whereas large clumps are indicative of the multiple Golgi complexes found during the growth phase of oogenesis. A clear indication of the multiple Golgi complexes is shown in (B), where immunofluorescence microscopy has been used to label the zona pellucida protein ZP-1; in (C), a similar labeling strategy was used to detect the oocytespecific factor BMP-15. © 2015, Elsevier, Inc. , Plant and Zeleznik, Knobil and Neill's Physiology of Reproduction, Fourth Edition 8
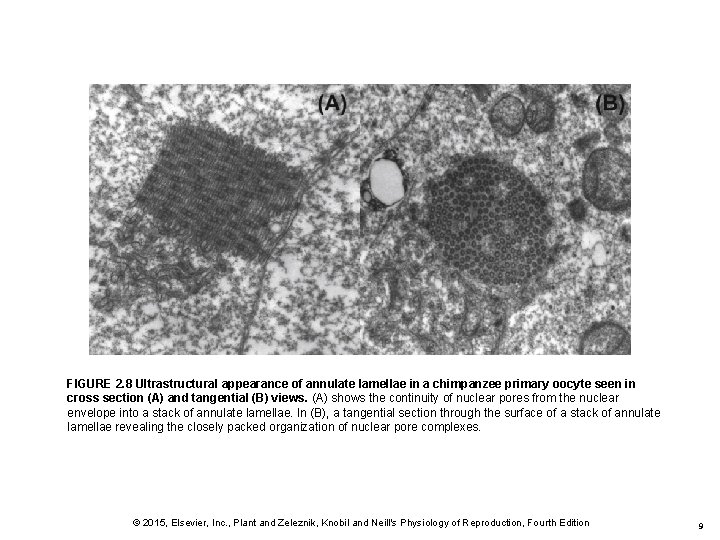
FIGURE 2. 8 Ultrastructural appearance of annulate lamellae in a chimpanzee primary oocyte seen in cross section (A) and tangential (B) views. (A) shows the continuity of nuclear pores from the nuclear envelope into a stack of annulate lamellae. In (B), a tangential section through the surface of a stack of annulate lamellae revealing the closely packed organization of nuclear pore complexes. © 2015, Elsevier, Inc. , Plant and Zeleznik, Knobil and Neill's Physiology of Reproduction, Fourth Edition 9
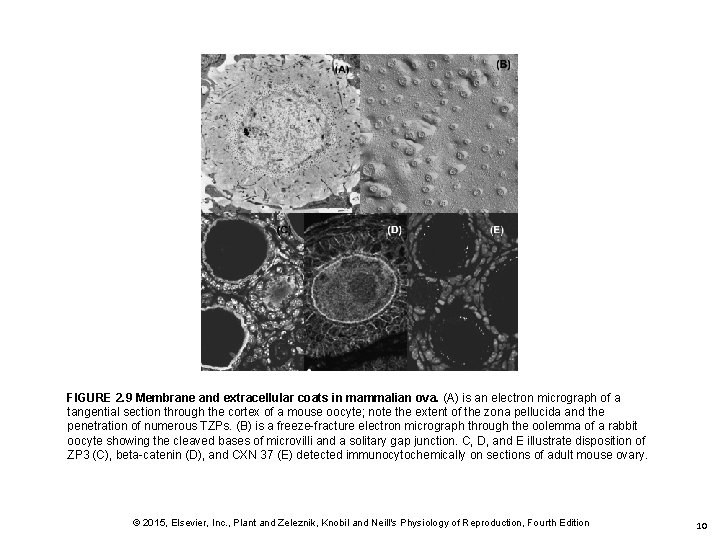
FIGURE 2. 9 Membrane and extracellular coats in mammalian ova. (A) is an electron micrograph of a tangential section through the cortex of a mouse oocyte; note the extent of the zona pellucida and the penetration of numerous TZPs. (B) is a freeze-fracture electron micrograph through the oolemma of a rabbit oocyte showing the cleaved bases of microvilli and a solitary gap junction. C, D, and E illustrate disposition of ZP 3 (C), beta-catenin (D), and CXN 37 (E) detected immunocytochemically on sections of adult mouse ovary. © 2015, Elsevier, Inc. , Plant and Zeleznik, Knobil and Neill's Physiology of Reproduction, Fourth Edition 10
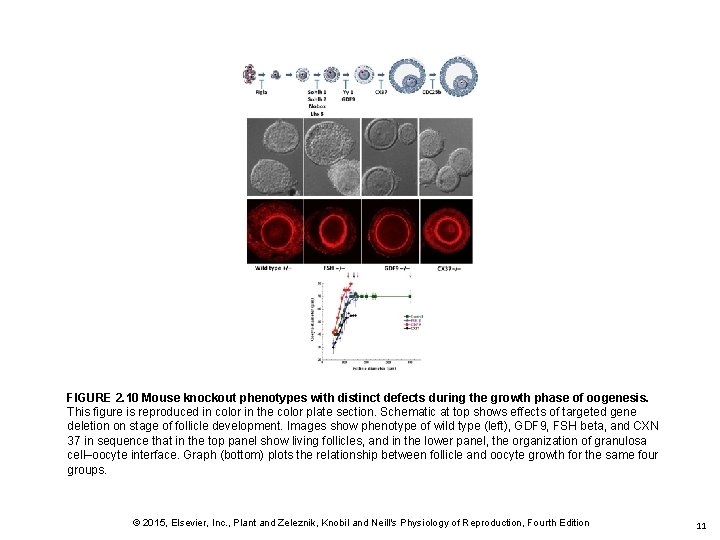
FIGURE 2. 10 Mouse knockout phenotypes with distinct defects during the growth phase of oogenesis. This figure is reproduced in color in the color plate section. Schematic at top shows effects of targeted gene deletion on stage of follicle development. Images show phenotype of wild type (left), GDF 9, FSH beta, and CXN 37 in sequence that in the top panel show living follicles, and in the lower panel, the organization of granulosa cell–oocyte interface. Graph (bottom) plots the relationship between follicle and oocyte growth for the same four groups. © 2015, Elsevier, Inc. , Plant and Zeleznik, Knobil and Neill's Physiology of Reproduction, Fourth Edition 11
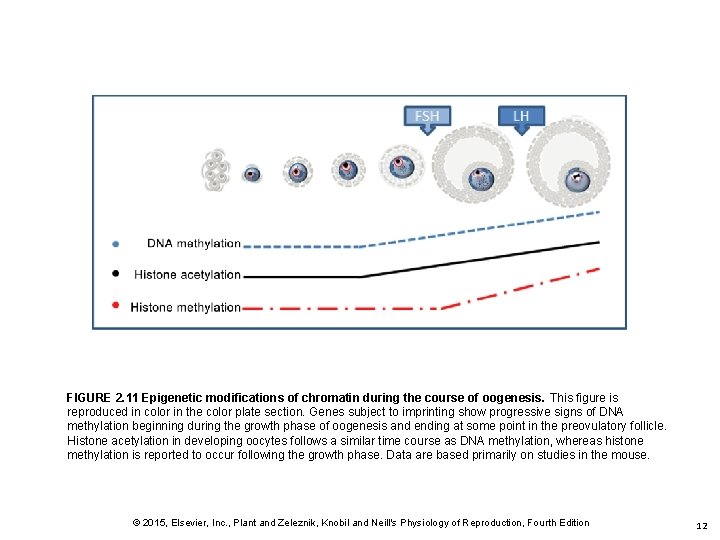
FIGURE 2. 11 Epigenetic modifications of chromatin during the course of oogenesis. This figure is reproduced in color in the color plate section. Genes subject to imprinting show progressive signs of DNA methylation beginning during the growth phase of oogenesis and ending at some point in the preovulatory follicle. Histone acetylation in developing oocytes follows a similar time course as DNA methylation, whereas histone methylation is reported to occur following the growth phase. Data are based primarily on studies in the mouse. © 2015, Elsevier, Inc. , Plant and Zeleznik, Knobil and Neill's Physiology of Reproduction, Fourth Edition 12
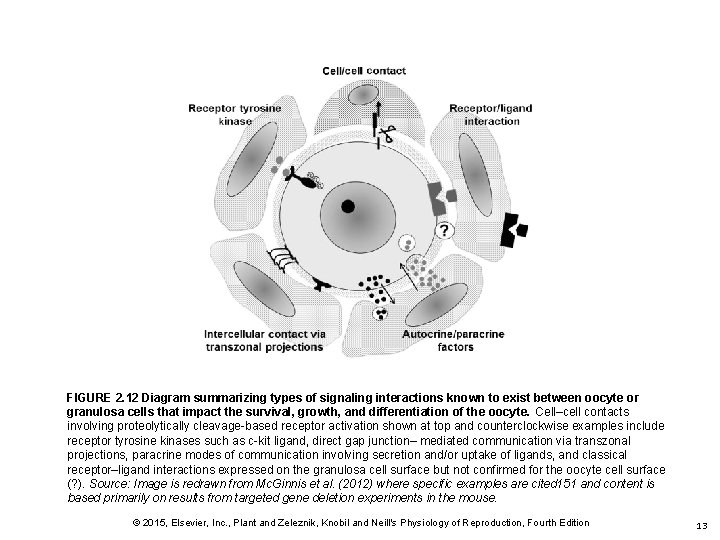
FIGURE 2. 12 Diagram summarizing types of signaling interactions known to exist between oocyte or granulosa cells that impact the survival, growth, and differentiation of the oocyte. Cell–cell contacts involving proteolytically cleavage-based receptor activation shown at top and counterclockwise examples include receptor tyrosine kinases such as c-kit ligand, direct gap junction– mediated communication via transzonal projections, paracrine modes of communication involving secretion and/or uptake of ligands, and classical receptor–ligand interactions expressed on the granulosa cell surface but not confirmed for the oocyte cell surface (? ). Source: Image is redrawn from Mc. Ginnis et al. (2012) where specific examples are cited 151 and content is based primarily on results from targeted gene deletion experiments in the mouse. © 2015, Elsevier, Inc. , Plant and Zeleznik, Knobil and Neill's Physiology of Reproduction, Fourth Edition 13
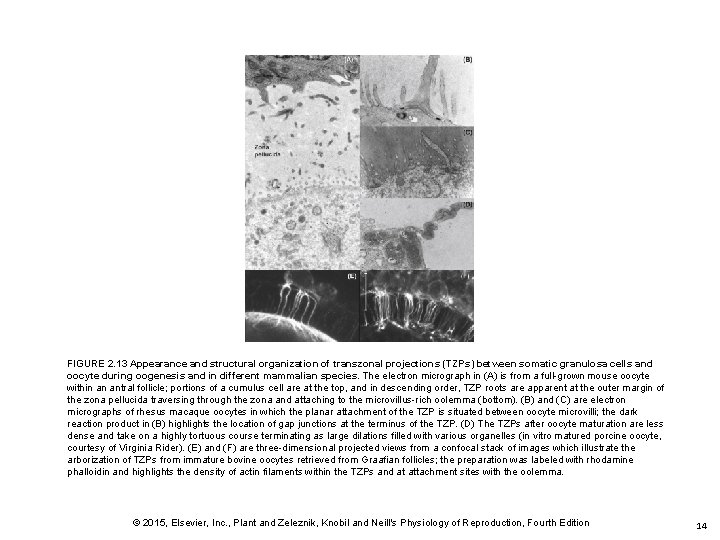
FIGURE 2. 13 Appearance and structural organization of transzonal projections (TZPs) between somatic granulosa cells and oocyte during oogenesis and in different mammalian species. The electron micrograph in (A) is from a full-grown mouse oocyte within an antral follicle; portions of a cumulus cell are at the top, and in descending order, TZP roots are apparent at the outer margin of the zona pellucida traversing through the zona and attaching to the microvillus-rich oolemma (bottom). (B) and (C) are electron micrographs of rhesus macaque oocytes in which the planar attachment of the TZP is situated between oocyte microvilli; the dark reaction product in (B) highlights the location of gap junctions at the terminus of the TZP. (D) The TZPs after oocyte maturation are less dense and take on a highly tortuous course terminating as large dilations filled with various organelles (in vitro matured porcine oocyte, courtesy of Virginia Rider). (E) and (F) are three-dimensional projected views from a confocal stack of images which illustrate the arborization of TZPs from immature bovine oocytes retrieved from Graafian follicles; the preparation was labeled with rhodamine phalloidin and highlights the density of actin filaments within the TZPs and at attachment sites with the oolemma. © 2015, Elsevier, Inc. , Plant and Zeleznik, Knobil and Neill's Physiology of Reproduction, Fourth Edition 14
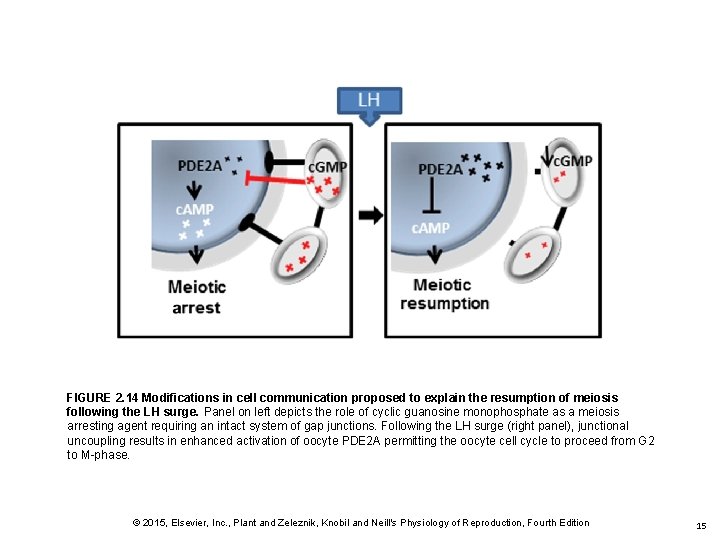
FIGURE 2. 14 Modifications in cell communication proposed to explain the resumption of meiosis following the LH surge. Panel on left depicts the role of cyclic guanosine monophosphate as a meiosis arresting agent requiring an intact system of gap junctions. Following the LH surge (right panel), junctional uncoupling results in enhanced activation of oocyte PDE 2 A permitting the oocyte cell cycle to proceed from G 2 to M-phase. © 2015, Elsevier, Inc. , Plant and Zeleznik, Knobil and Neill's Physiology of Reproduction, Fourth Edition 15
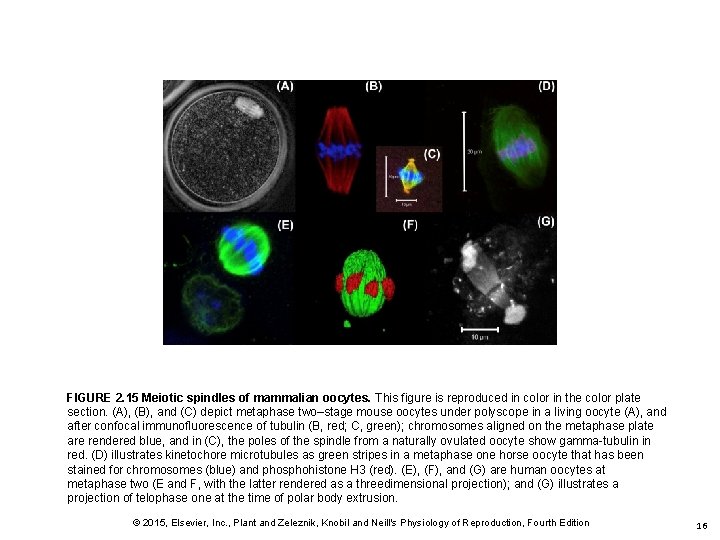
FIGURE 2. 15 Meiotic spindles of mammalian oocytes. This figure is reproduced in color in the color plate section. (A), (B), and (C) depict metaphase two–stage mouse oocytes under polyscope in a living oocyte (A), and after confocal immunofluorescence of tubulin (B, red; C, green); chromosomes aligned on the metaphase plate are rendered blue, and in (C), the poles of the spindle from a naturally ovulated oocyte show gamma-tubulin in red. (D) illustrates kinetochore microtubules as green stripes in a metaphase one horse oocyte that has been stained for chromosomes (blue) and phosphohistone H 3 (red). (E), (F), and (G) are human oocytes at metaphase two (E and F, with the latter rendered as a threedimensional projection); and (G) illustrates a projection of telophase one at the time of polar body extrusion. © 2015, Elsevier, Inc. , Plant and Zeleznik, Knobil and Neill's Physiology of Reproduction, Fourth Edition 16
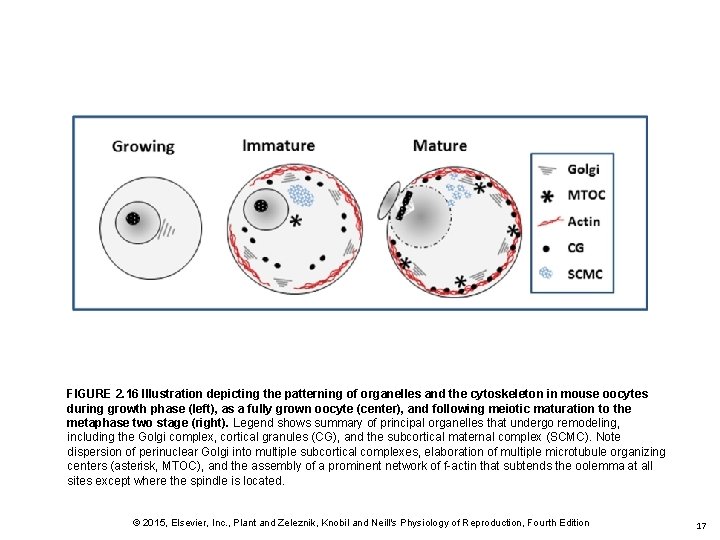
FIGURE 2. 16 Illustration depicting the patterning of organelles and the cytoskeleton in mouse oocytes during growth phase (left), as a fully grown oocyte (center), and following meiotic maturation to the metaphase two stage (right). Legend shows summary of principal organelles that undergo remodeling, including the Golgi complex, cortical granules (CG), and the subcortical maternal complex (SCMC). Note dispersion of perinuclear Golgi into multiple subcortical complexes, elaboration of multiple microtubule organizing centers (asterisk, MTOC), and the assembly of a prominent network of f-actin that subtends the oolemma at all sites except where the spindle is located. © 2015, Elsevier, Inc. , Plant and Zeleznik, Knobil and Neill's Physiology of Reproduction, Fourth Edition 17
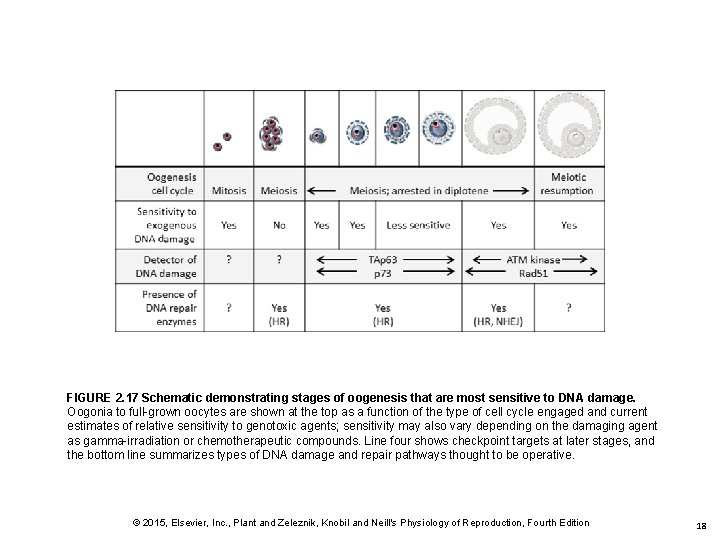
FIGURE 2. 17 Schematic demonstrating stages of oogenesis that are most sensitive to DNA damage. Oogonia to full-grown oocytes are shown at the top as a function of the type of cell cycle engaged and current estimates of relative sensitivity to genotoxic agents; sensitivity may also vary depending on the damaging agent as gamma-irradiation or chemotherapeutic compounds. Line four shows checkpoint targets at later stages, and the bottom line summarizes types of DNA damage and repair pathways thought to be operative. © 2015, Elsevier, Inc. , Plant and Zeleznik, Knobil and Neill's Physiology of Reproduction, Fourth Edition 18
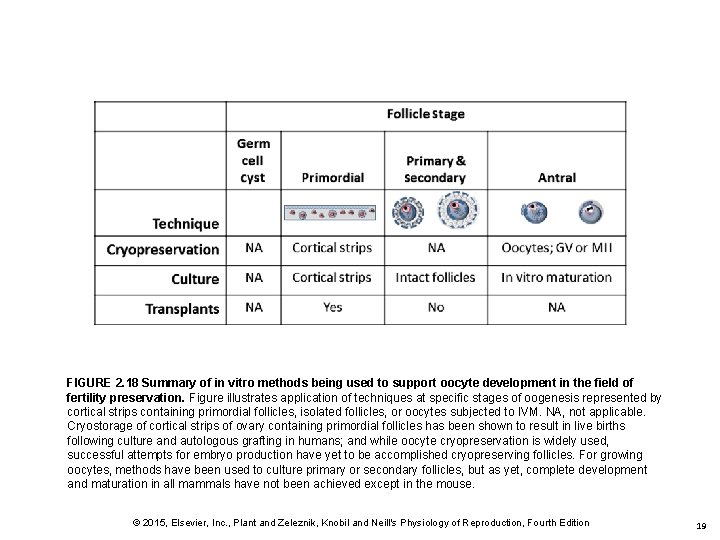
FIGURE 2. 18 Summary of in vitro methods being used to support oocyte development in the field of fertility preservation. Figure illustrates application of techniques at specific stages of oogenesis represented by cortical strips containing primordial follicles, isolated follicles, or oocytes subjected to IVM. NA, not applicable. Cryostorage of cortical strips of ovary containing primordial follicles has been shown to result in live births following culture and autologous grafting in humans; and while oocyte cryopreservation is widely used, successful attempts for embryo production have yet to be accomplished cryopreserving follicles. For growing oocytes, methods have been used to culture primary or secondary follicles, but as yet, complete development and maturation in all mammals have not been achieved except in the mouse. © 2015, Elsevier, Inc. , Plant and Zeleznik, Knobil and Neill's Physiology of Reproduction, Fourth Edition 19
Galactokinesis
Class mammalia characteristics
Prophase 2
Primary spermatocyte
Facts about miosis
Primary oocyte haploid or diploid
Meiosis ii stages
Primary oocyte definition
Germinal epithelium
Secondary follicles
Primary oocyte
An oocyte is
An oocyte is
Oocyte
Allantois
D
Secondary oocyte
Oogenesis secondary oocyte
Types of placenta ppt
Polar body