CGS Ground School Principles Of Flight Manoeuvres Crown
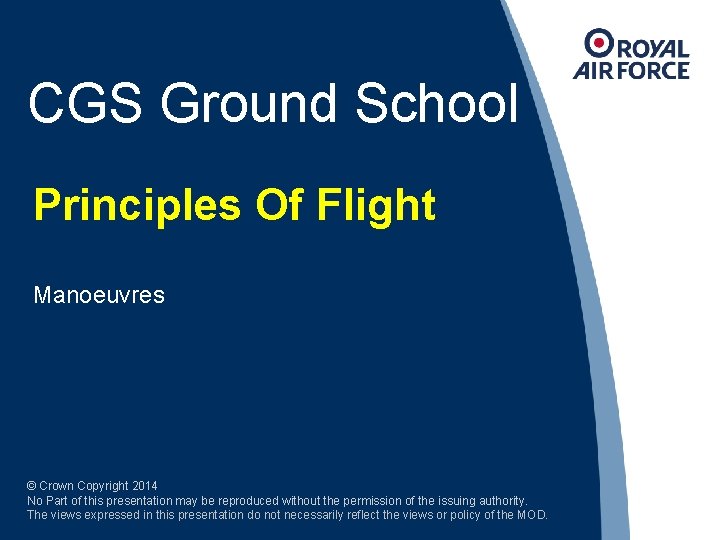
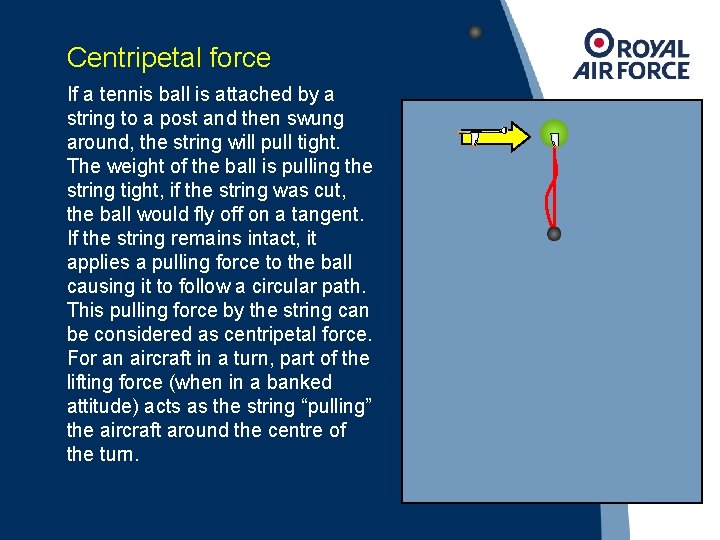
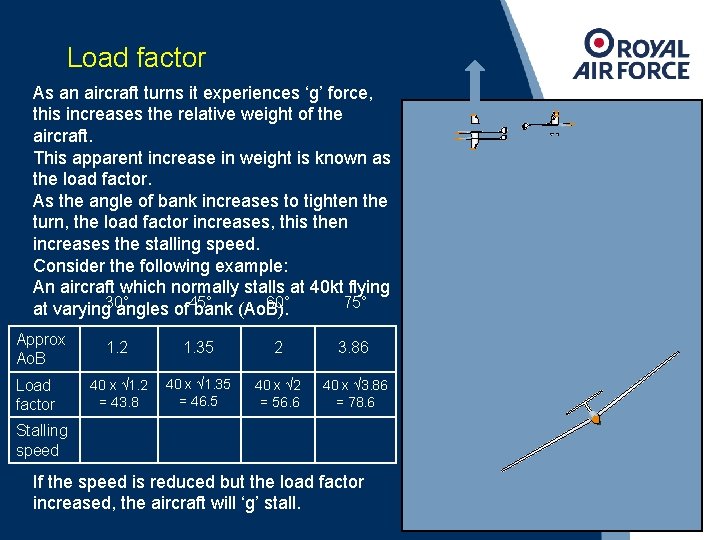
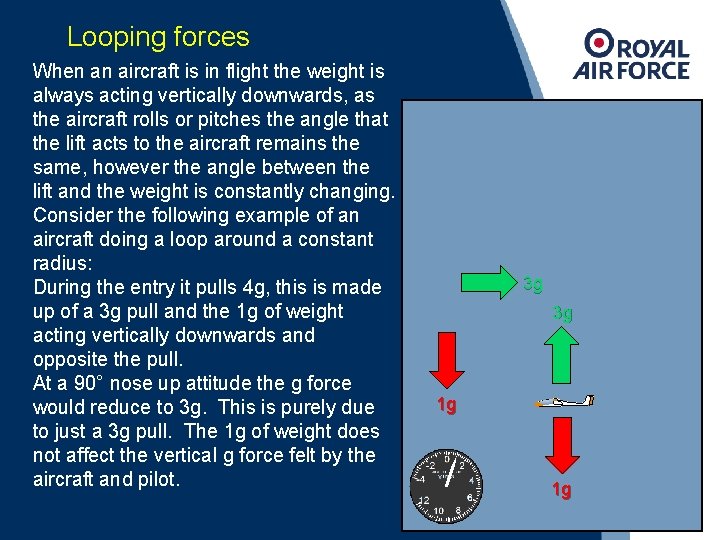
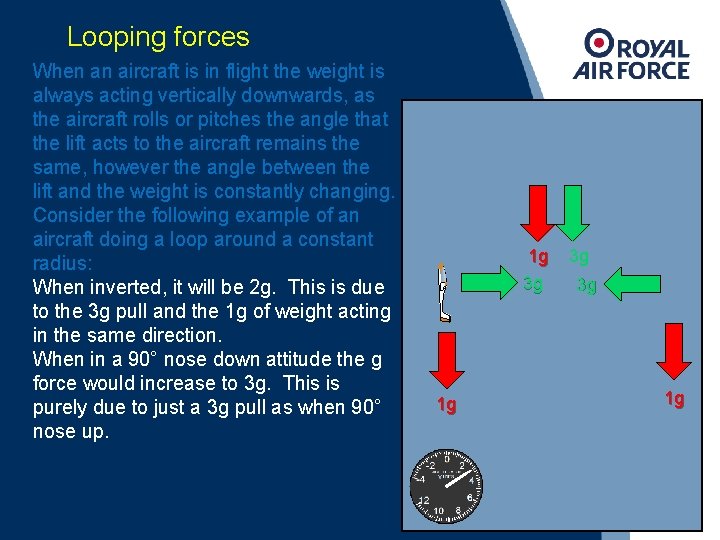
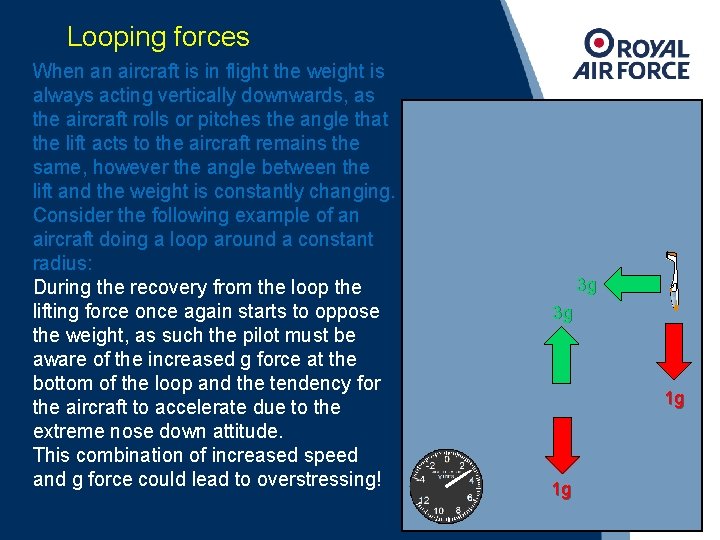
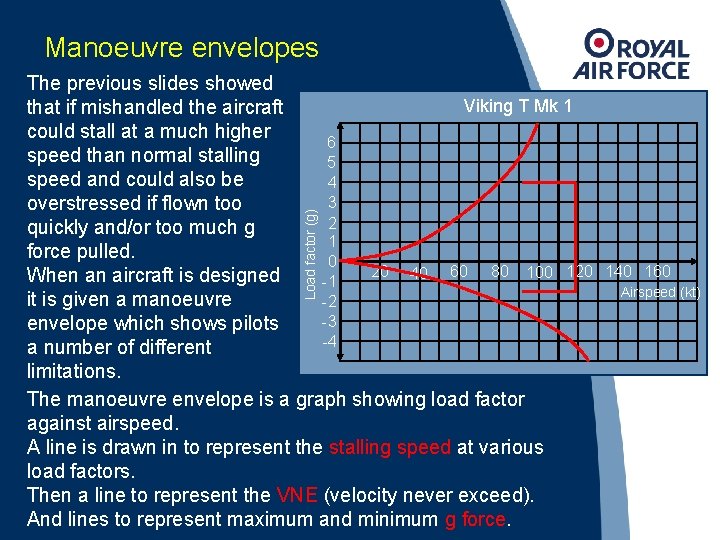
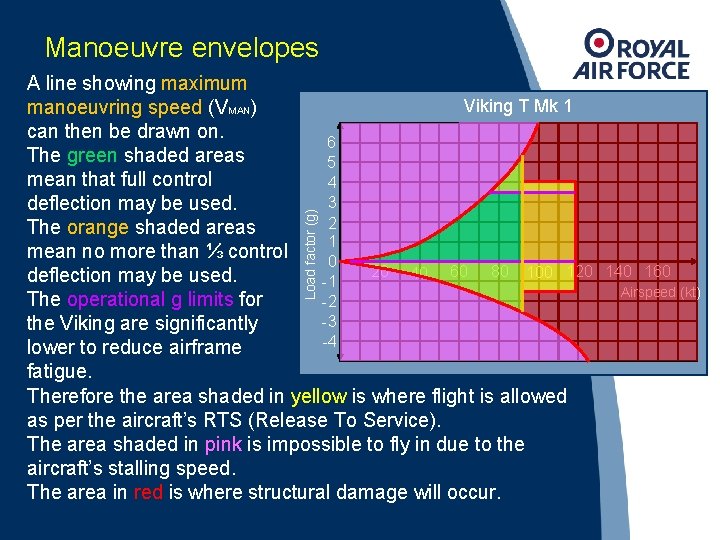
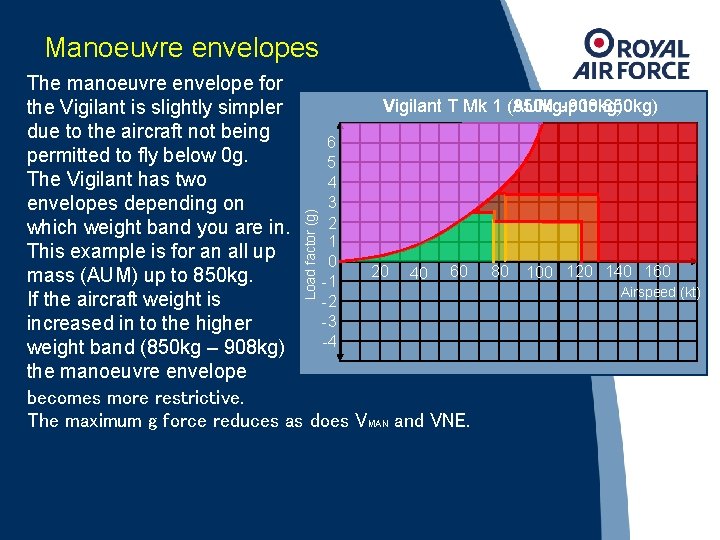
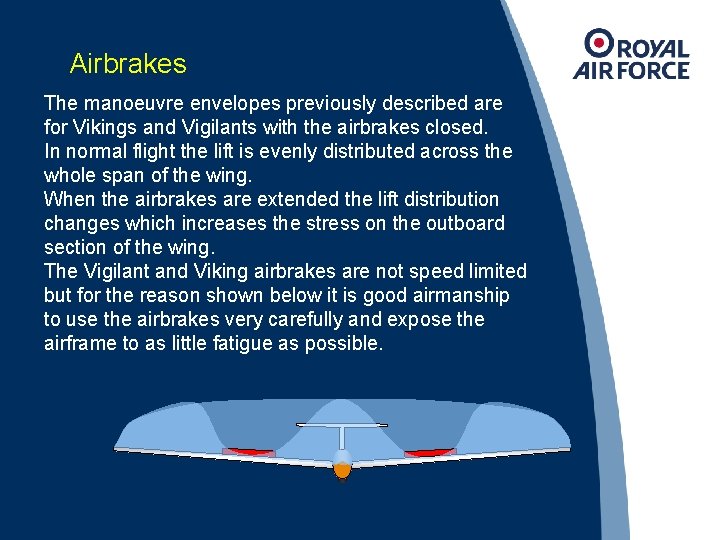
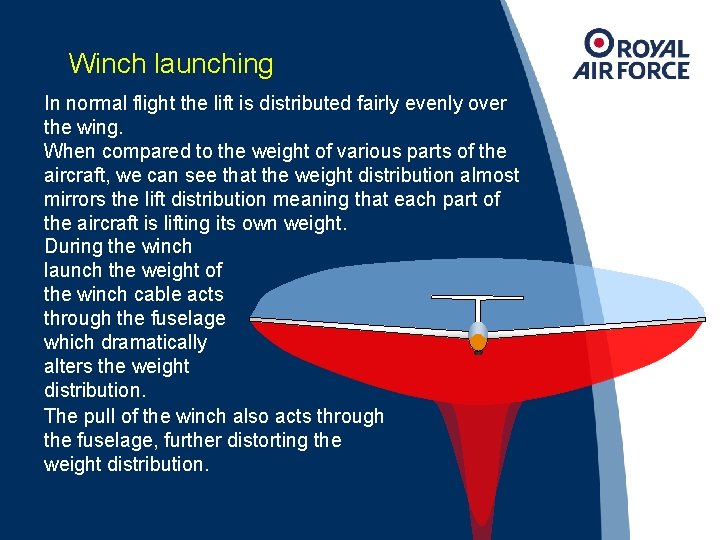
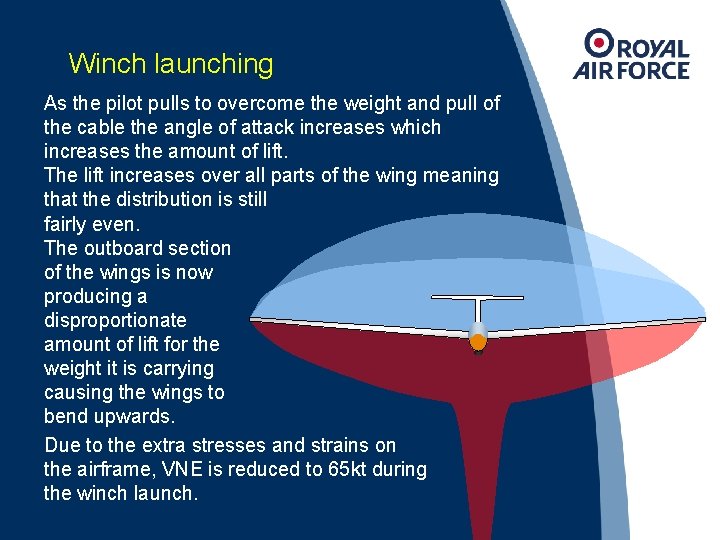
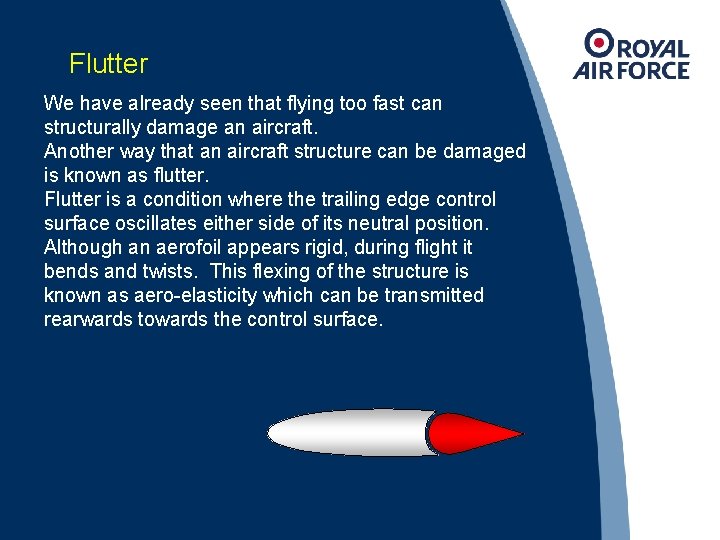
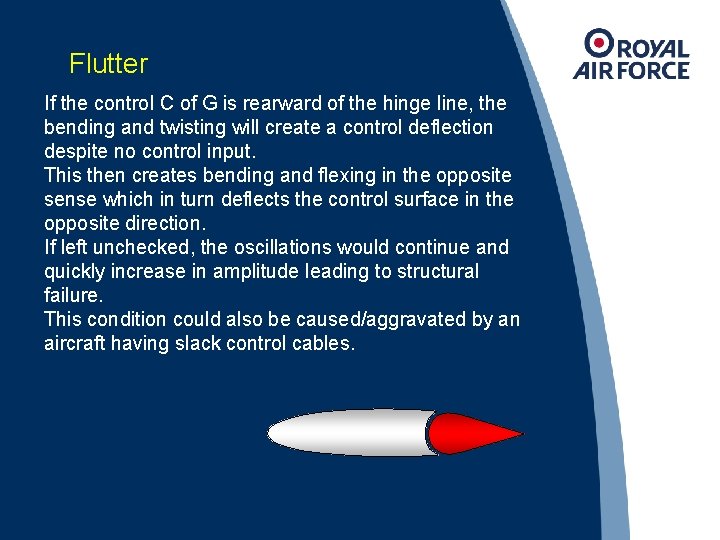
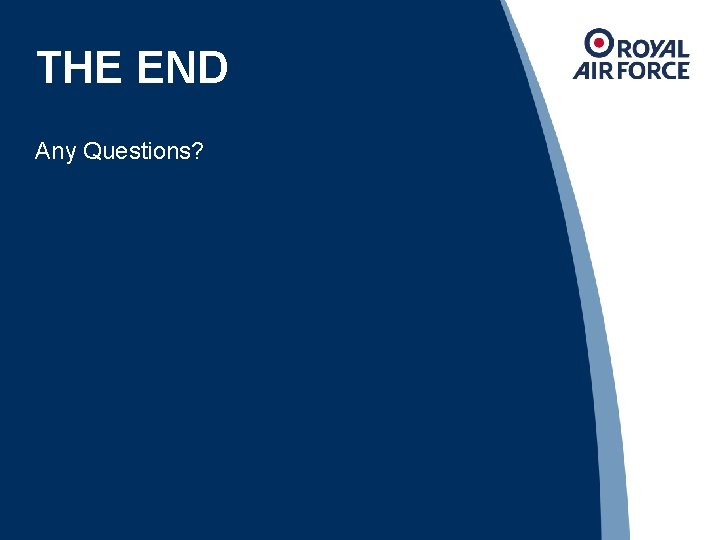
- Slides: 15
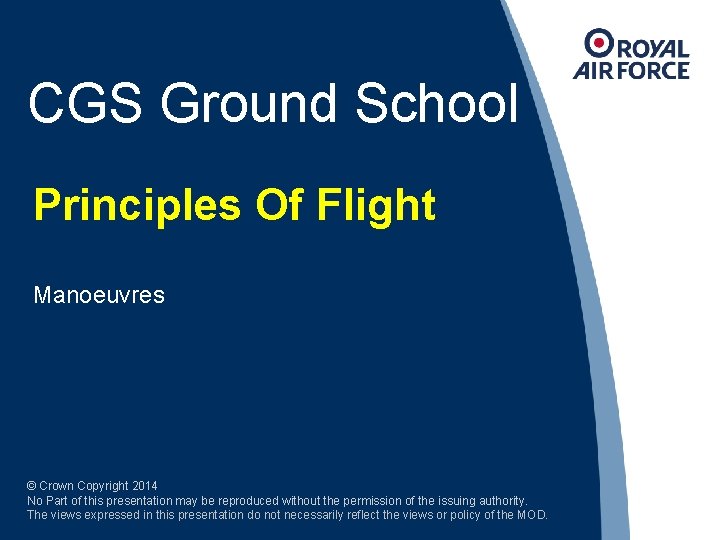
CGS Ground School Principles Of Flight Manoeuvres © Crown Copyright 2014 No Part of this presentation may be reproduced without the permission of the issuing authority. The views expressed in this presentation do not necessarily reflect the views or policy of the MOD.
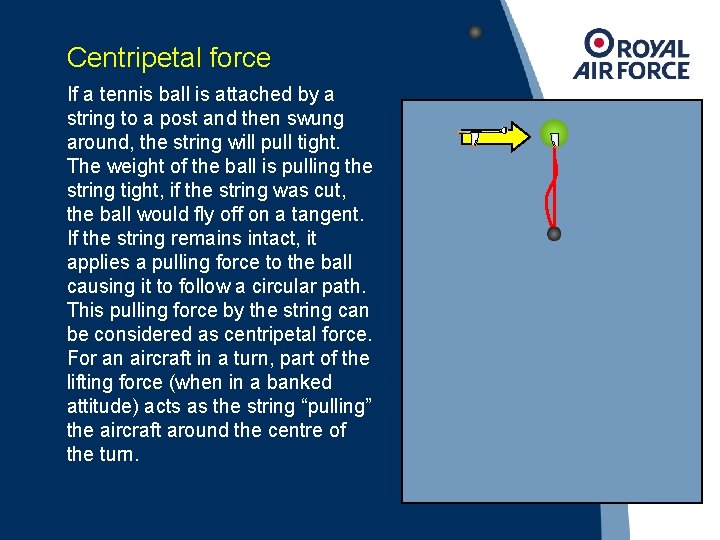
Centripetal force If a tennis ball is attached by a string to a post and then swung around, the string will pull tight. The weight of the ball is pulling the string tight, if the string was cut, the ball would fly off on a tangent. If the string remains intact, it applies a pulling force to the ball causing it to follow a circular path. This pulling force by the string can be considered as centripetal force. For an aircraft in a turn, part of the lifting force (when in a banked attitude) acts as the string “pulling” the aircraft around the centre of the turn.
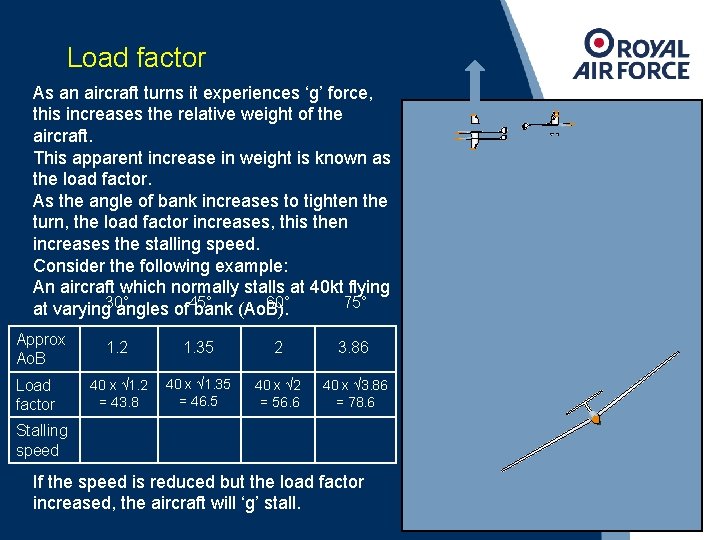
Load factor As an aircraft turns it experiences ‘g’ force, this increases the relative weight of the aircraft. This apparent increase in weight is known as the load factor. As the angle of bank increases to tighten the turn, the load factor increases, this then increases the stalling speed. Consider the following example: An aircraft which normally stalls at 40 kt flying 60° 75° at varying 30° angles of 45° bank (Ao. B). Approx Ao. B Load factor 1. 2 1. 35 2 3. 86 40 x √ 1. 2 = 43. 8 40 x √ 1. 35 = 46. 5 40 x √ 2 = 56. 6 40 x √ 3. 86 = 78. 6 Stalling speed If the speed is reduced but the load factor increased, the aircraft will ‘g’ stall.
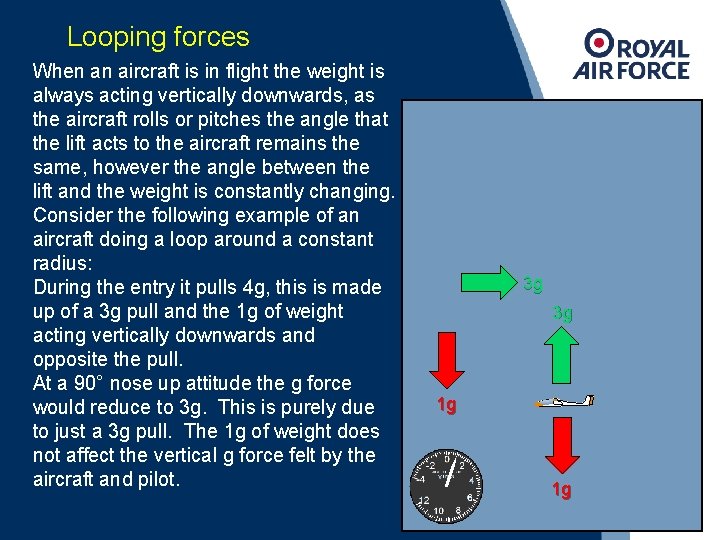
Looping forces When an aircraft is in flight the weight is always acting vertically downwards, as the aircraft rolls or pitches the angle that the lift acts to the aircraft remains the same, however the angle between the lift and the weight is constantly changing. Consider the following example of an aircraft doing a loop around a constant radius: During the entry it pulls 4 g, this is made up of a 3 g pull and the 1 g of weight acting vertically downwards and opposite the pull. At a 90° nose up attitude the g force would reduce to 3 g. This is purely due to just a 3 g pull. The 1 g of weight does not affect the vertical g force felt by the aircraft and pilot. 3 g 3 g 1 g 1 g
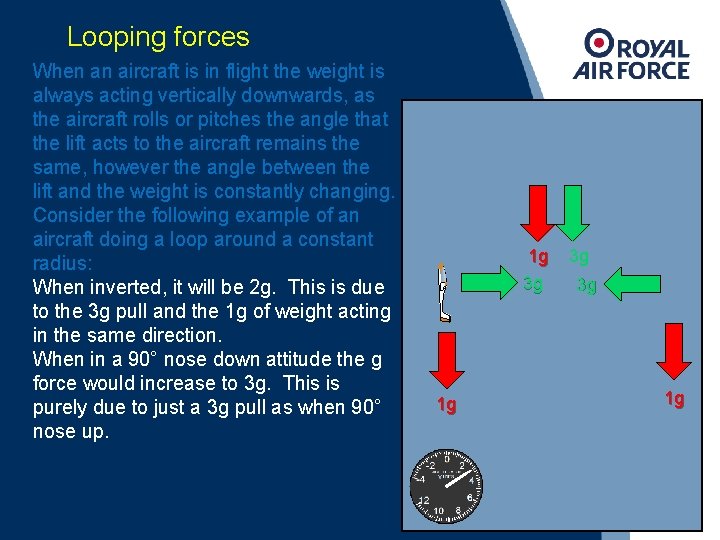
Looping forces When an aircraft is in flight the weight is always acting vertically downwards, as the aircraft rolls or pitches the angle that the lift acts to the aircraft remains the same, however the angle between the lift and the weight is constantly changing. Consider the following example of an aircraft doing a loop around a constant radius: When inverted, it will be 2 g. This is due to the 3 g pull and the 1 g of weight acting in the same direction. When in a 90° nose down attitude the g force would increase to 3 g. This is purely due to just a 3 g pull as when 90° nose up. 1 g 3 g 3 g 1 g
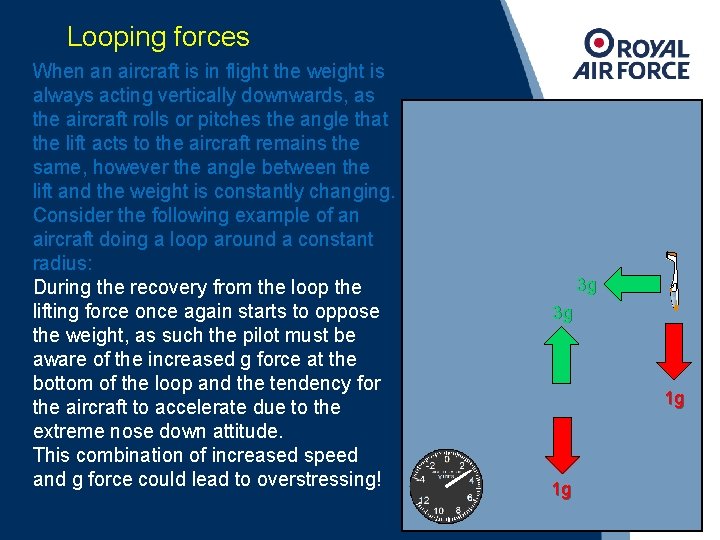
Looping forces When an aircraft is in flight the weight is always acting vertically downwards, as the aircraft rolls or pitches the angle that the lift acts to the aircraft remains the same, however the angle between the lift and the weight is constantly changing. Consider the following example of an aircraft doing a loop around a constant radius: During the recovery from the loop the lifting force once again starts to oppose the weight, as such the pilot must be aware of the increased g force at the bottom of the loop and the tendency for the aircraft to accelerate due to the extreme nose down attitude. This combination of increased speed and g force could lead to overstressing! 3 g 3 g 1 g 1 g
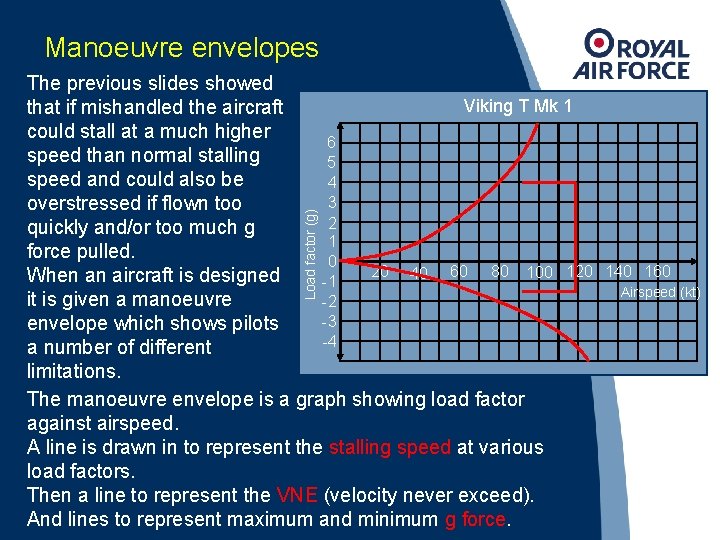
Manoeuvre envelopes Load factor (g) The previous slides showed Viking T Mk 1 that if mishandled the aircraft could stall at a much higher 6 speed than normal stalling 5 speed and could also be 4 3 overstressed if flown too 2 quickly and/or too much g 1 force pulled. 0 20 40 60 80 100 120 When an aircraft is designed -1 it is given a manoeuvre -2 -3 envelope which shows pilots -4 a number of different limitations. The manoeuvre envelope is a graph showing load factor against airspeed. A line is drawn in to represent the stalling speed at various load factors. Then a line to represent the VNE (velocity never exceed). And lines to represent maximum and minimum g force. 140 160 Airspeed (kt)
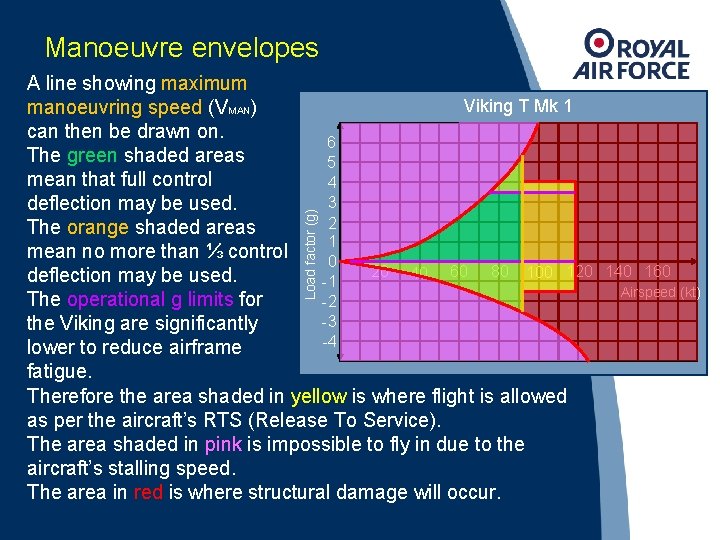
Manoeuvre envelopes Load factor (g) A line showing maximum Viking T Mk 1 manoeuvring speed (VMAN) can then be drawn on. 6 The green shaded areas 5 mean that full control 4 3 deflection may be used. 2 The orange shaded areas 1 mean no more than ⅓ control 0 20 40 60 80 100 120 deflection may be used. -1 The operational g limits for -2 -3 the Viking are significantly -4 lower to reduce airframe fatigue. Therefore the area shaded in yellow is where flight is allowed as per the aircraft’s RTS (Release To Service). The area shaded in pink is impossible to fly in due to the aircraft’s stalling speed. The area in red is where structural damage will occur. 140 160 Airspeed (kt)
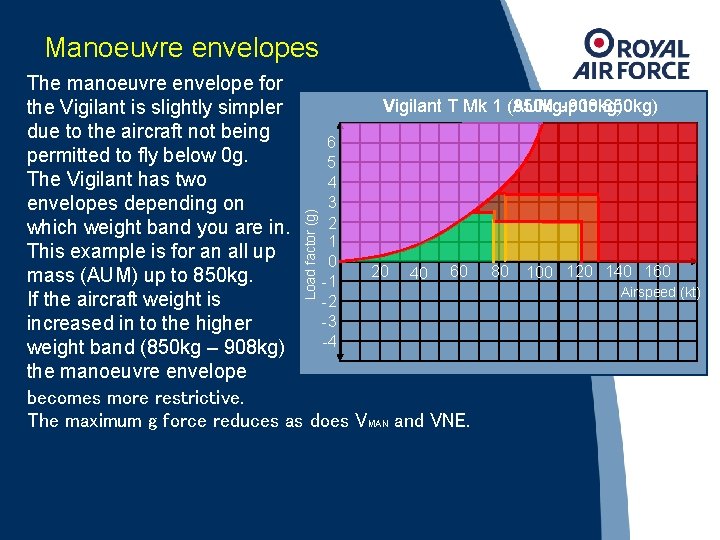
Manoeuvre envelopes Load factor (g) The manoeuvre envelope for Vigilant T Mk 1 (AUM (850 kg-908 kg) up to 850 kg) the Vigilant is slightly simpler due to the aircraft not being 6 permitted to fly below 0 g. 5 The Vigilant has two 4 3 envelopes depending on 2 which weight band you are in. 1 This example is for an all up 0 20 40 60 80 100 120 140 160 mass (AUM) up to 850 kg. -1 Airspeed (kt) If the aircraft weight is -2 -3 increased in to the higher -4 weight band (850 kg – 908 kg) the manoeuvre envelope becomes more restrictive. The maximum g force reduces as does VMAN and VNE.
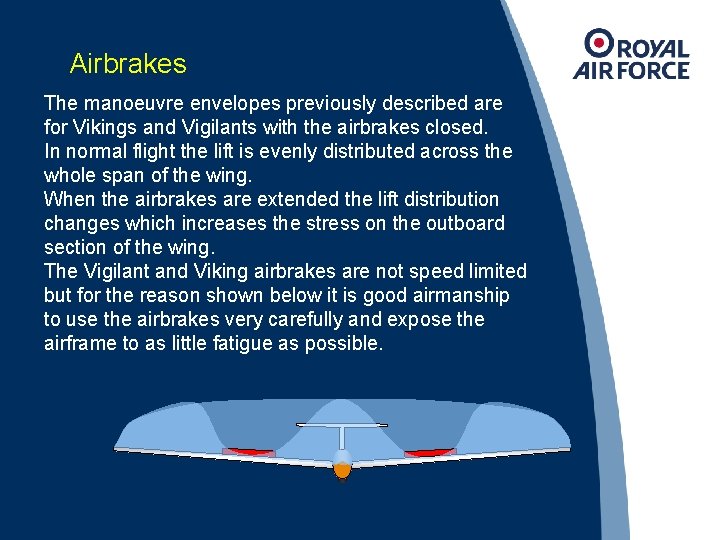
Airbrakes The manoeuvre envelopes previously described are for Vikings and Vigilants with the airbrakes closed. In normal flight the lift is evenly distributed across the whole span of the wing. When the airbrakes are extended the lift distribution changes which increases the stress on the outboard section of the wing. The Vigilant and Viking airbrakes are not speed limited but for the reason shown below it is good airmanship to use the airbrakes very carefully and expose the airframe to as little fatigue as possible.
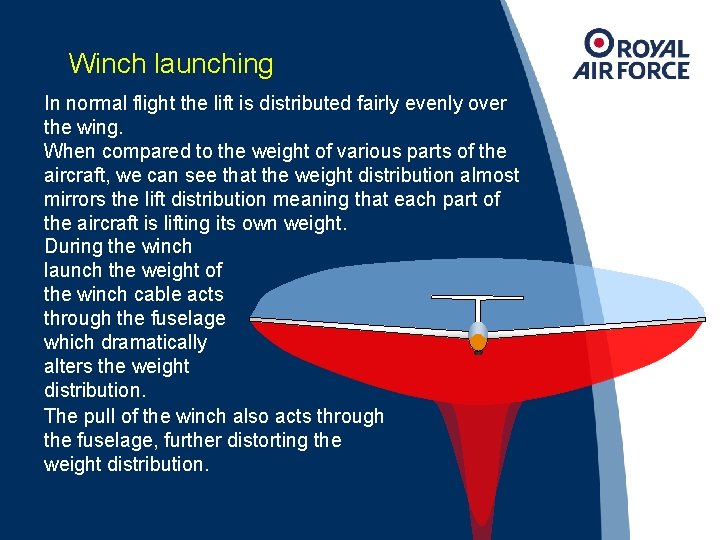
Winch launching In normal flight the lift is distributed fairly evenly over the wing. When compared to the weight of various parts of the aircraft, we can see that the weight distribution almost mirrors the lift distribution meaning that each part of the aircraft is lifting its own weight. During the winch launch the weight of the winch cable acts through the fuselage which dramatically alters the weight distribution. The pull of the winch also acts through the fuselage, further distorting the weight distribution.
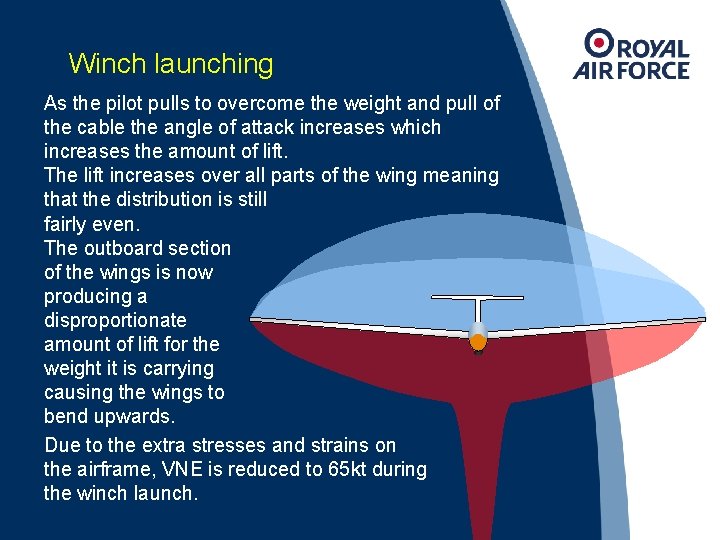
Winch launching As the pilot pulls to overcome the weight and pull of the cable the angle of attack increases which increases the amount of lift. The lift increases over all parts of the wing meaning that the distribution is still fairly even. The outboard section of the wings is now producing a disproportionate amount of lift for the weight it is carrying causing the wings to bend upwards. Due to the extra stresses and strains on the airframe, VNE is reduced to 65 kt during the winch launch.
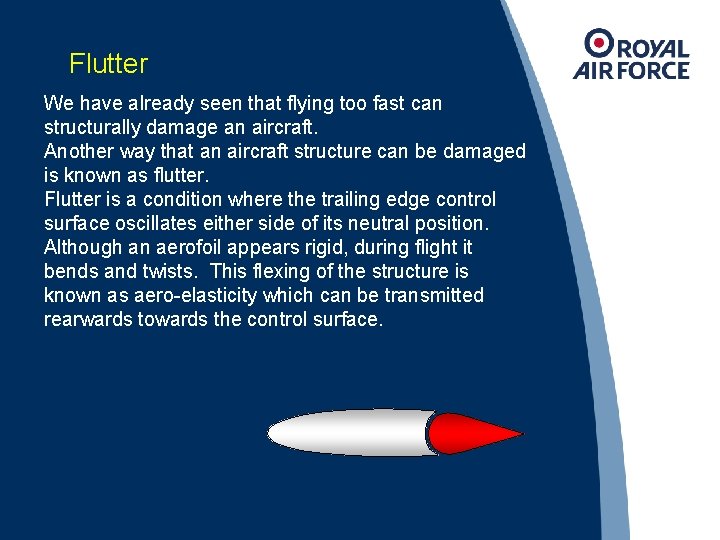
Flutter We have already seen that flying too fast can structurally damage an aircraft. Another way that an aircraft structure can be damaged is known as flutter. Flutter is a condition where the trailing edge control surface oscillates either side of its neutral position. Although an aerofoil appears rigid, during flight it bends and twists. This flexing of the structure is known as aero-elasticity which can be transmitted rearwards towards the control surface.
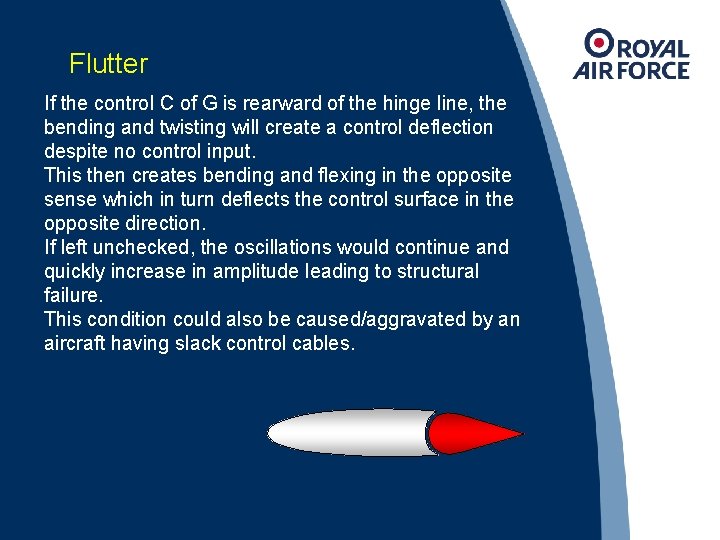
Flutter If the control C of G is rearward of the hinge line, the bending and twisting will create a control deflection despite no control input. This then creates bending and flexing in the opposite sense which in turn deflects the control surface in the opposite direction. If left unchecked, the oscillations would continue and quickly increase in amplitude leading to structural failure. This condition could also be caused/aggravated by an aircraft having slack control cables.
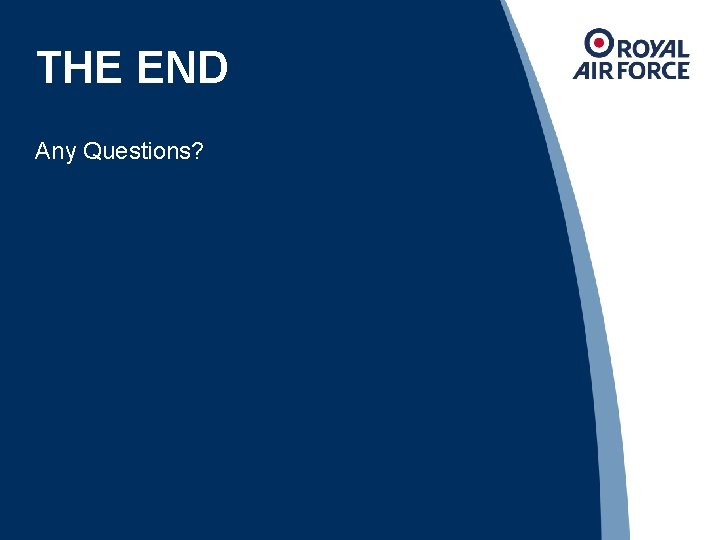
THE END Any Questions?