Cellular Respiration Step 4 Electron Transport Chain Chemiosmosis
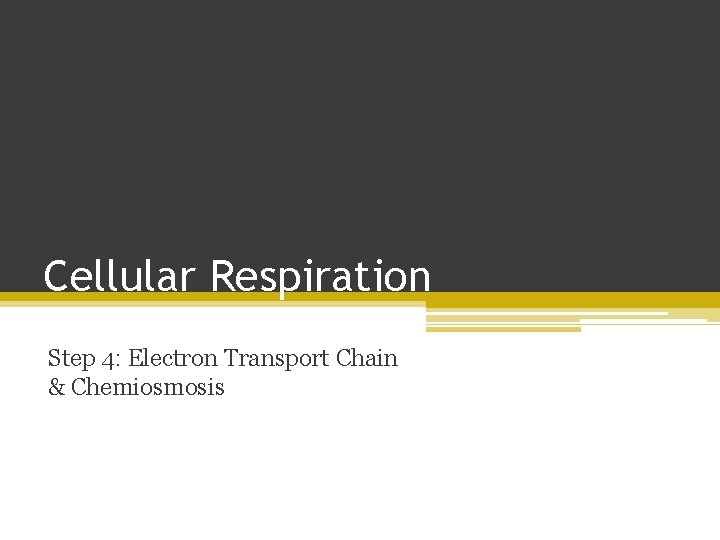
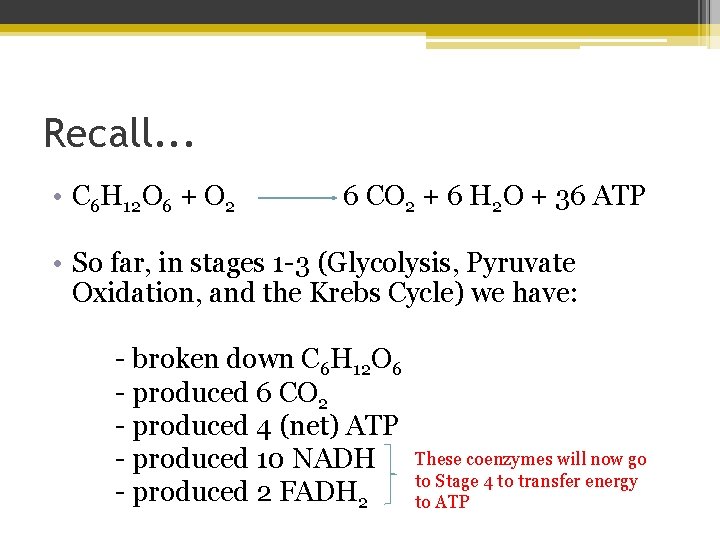
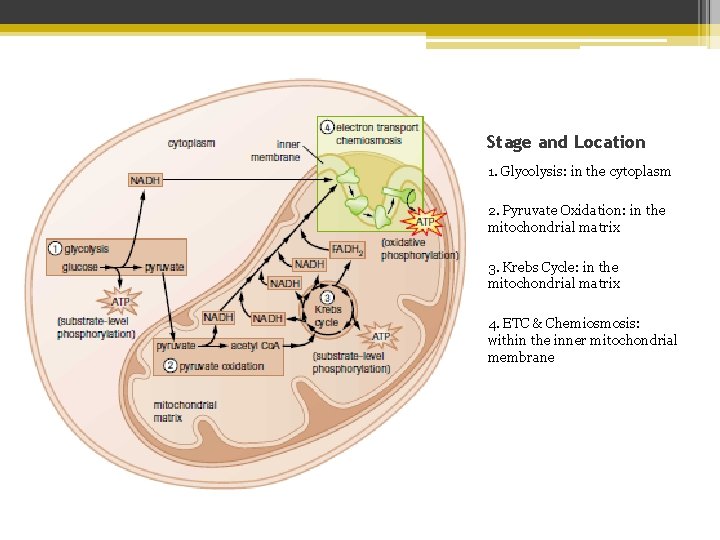
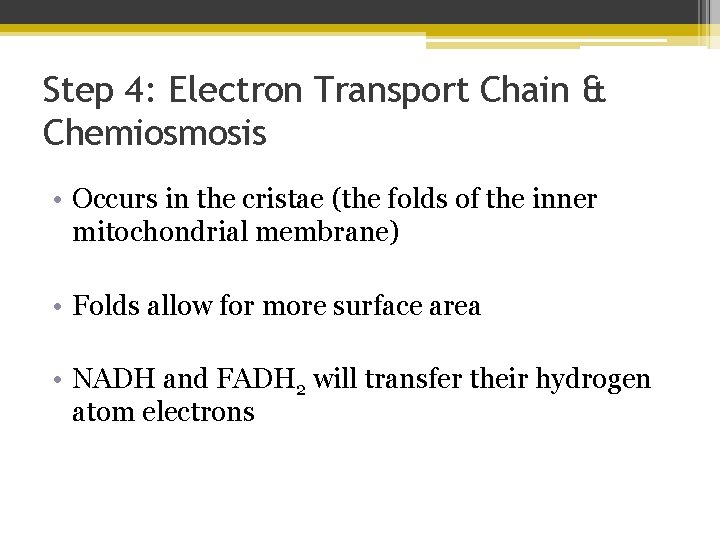
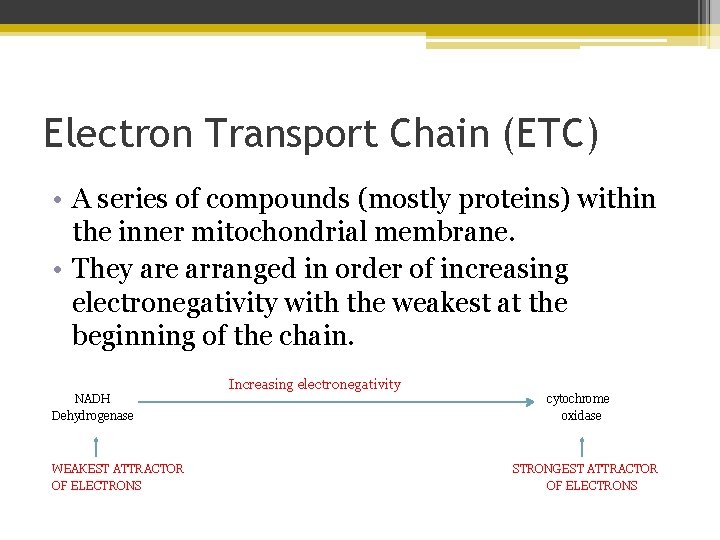
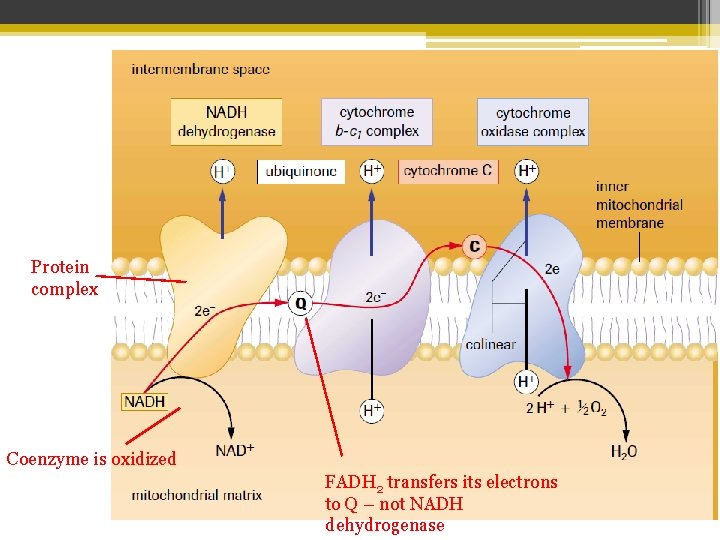
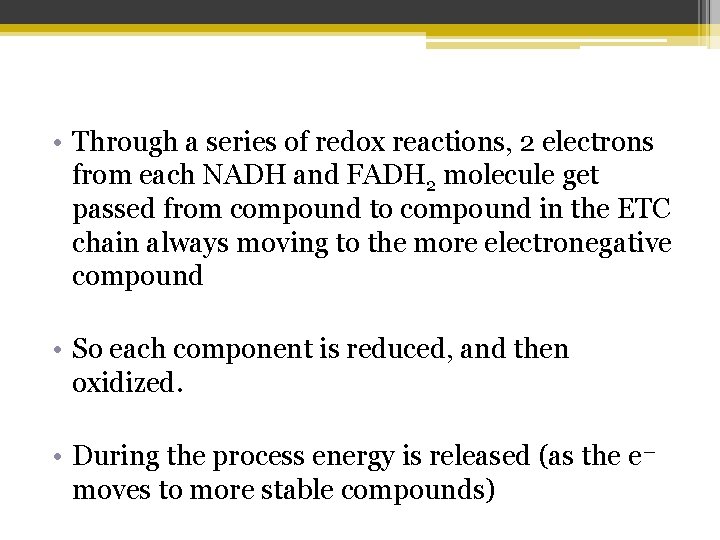
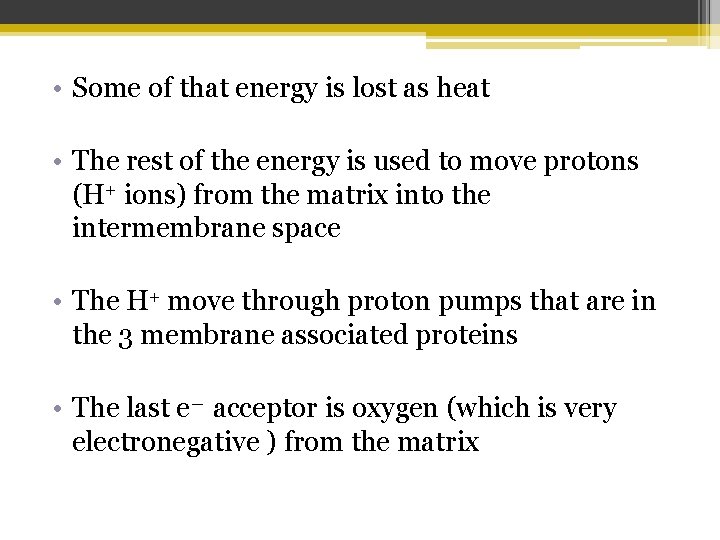
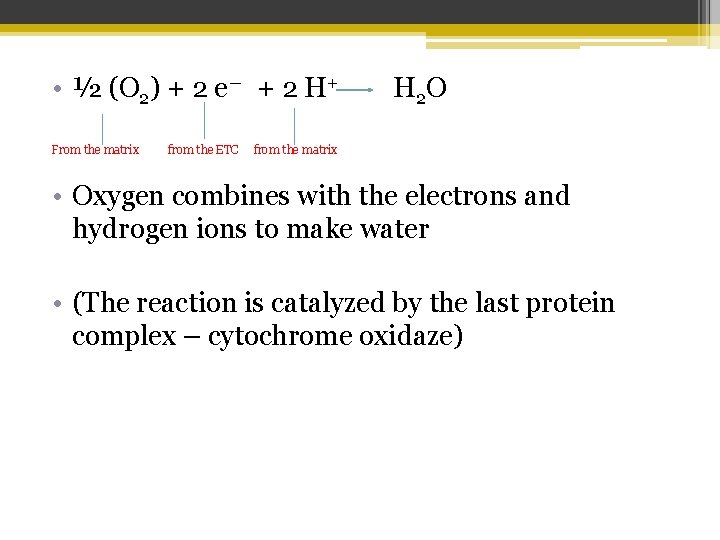
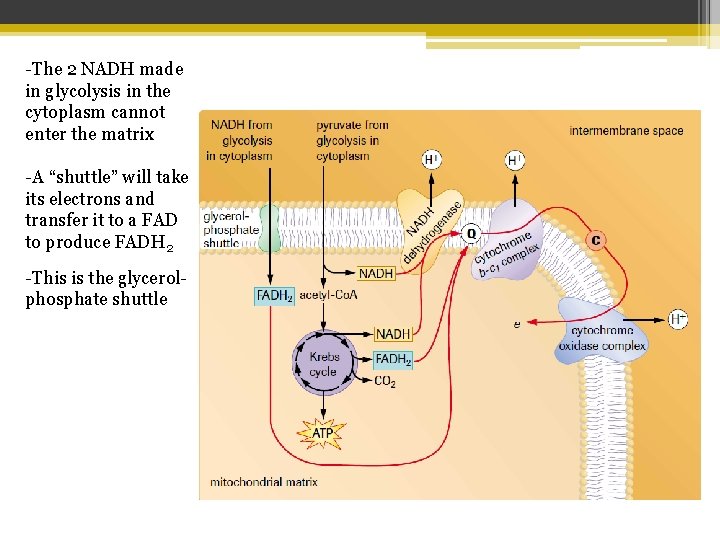
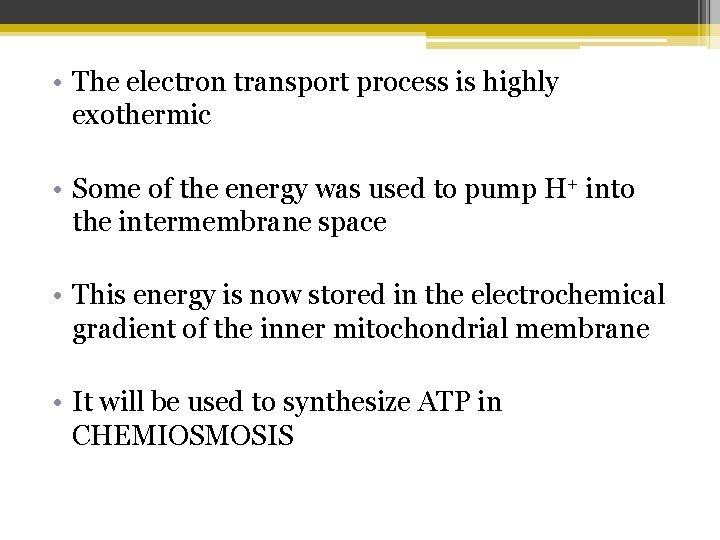
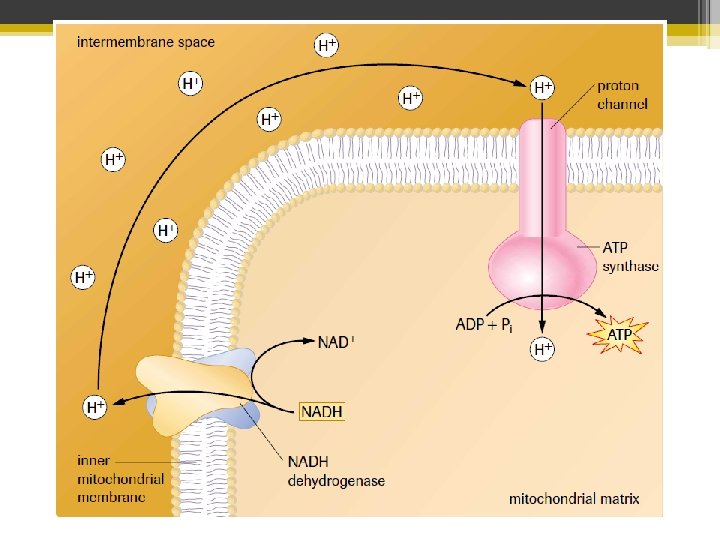
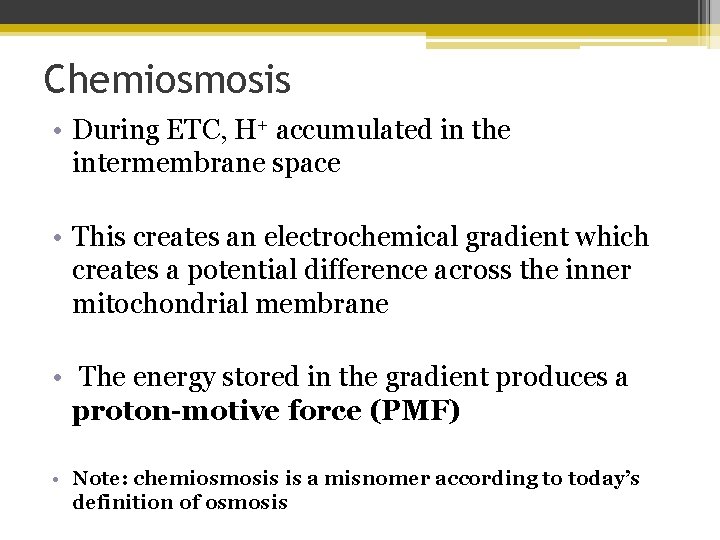
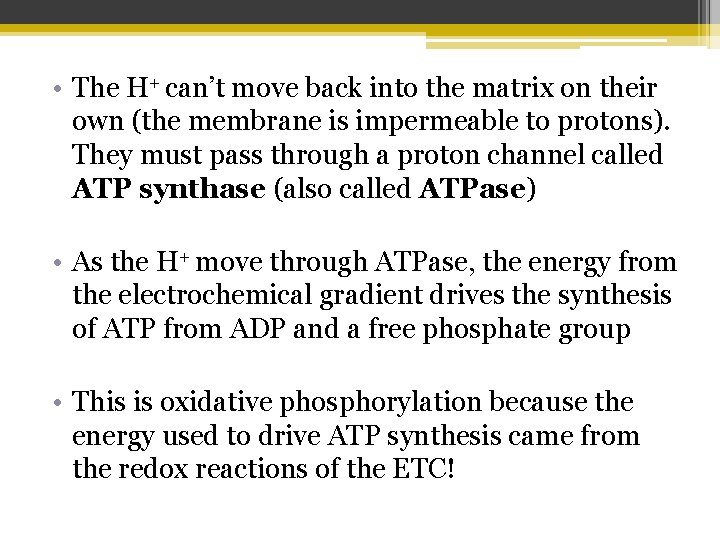
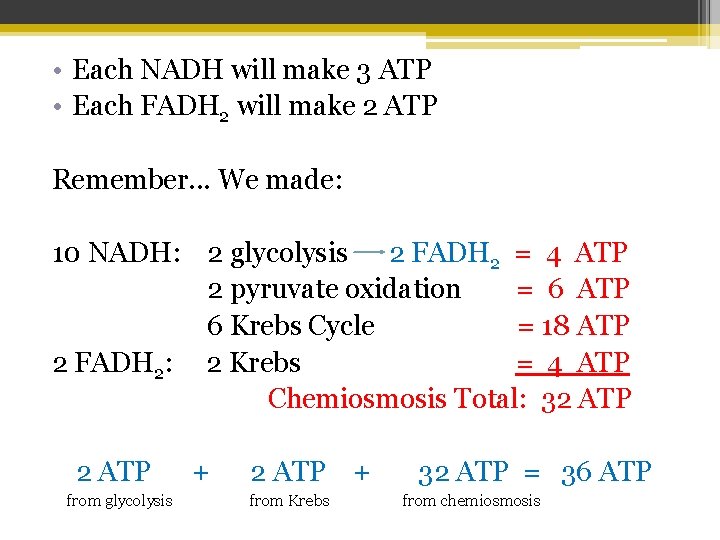
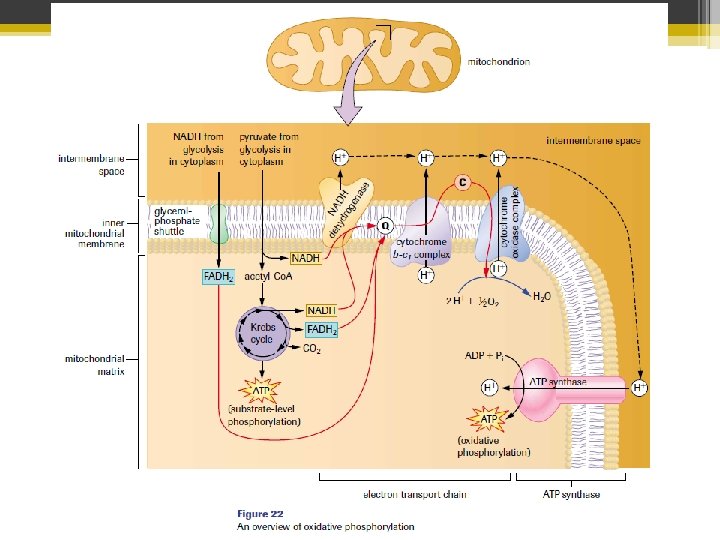
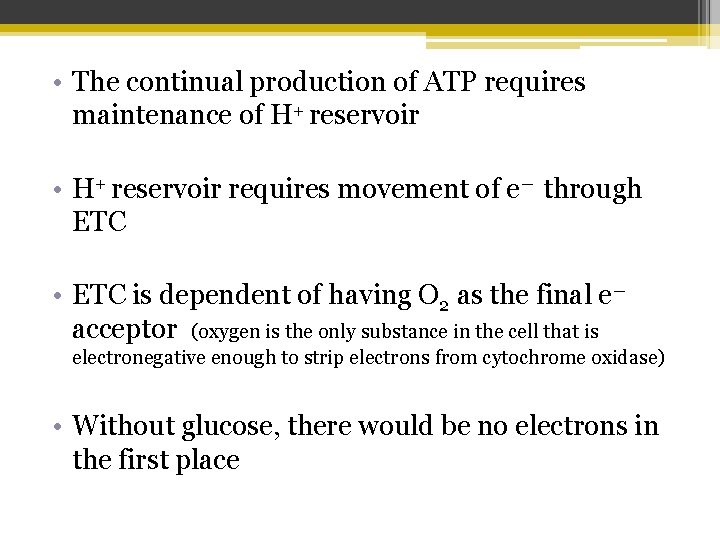
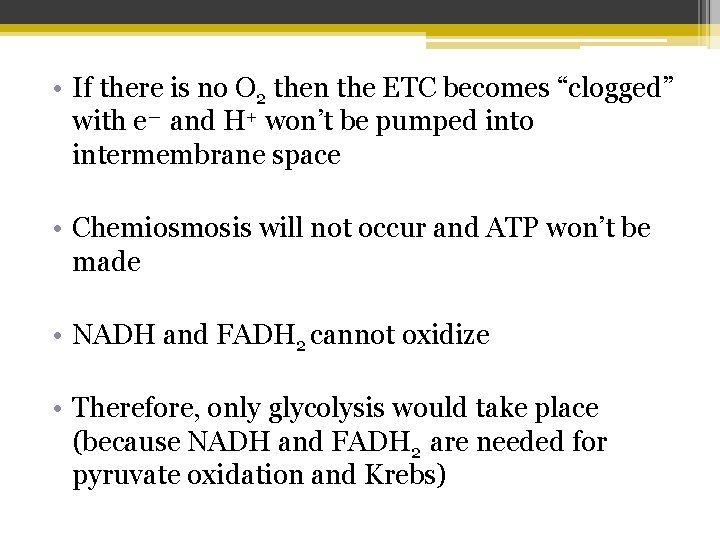
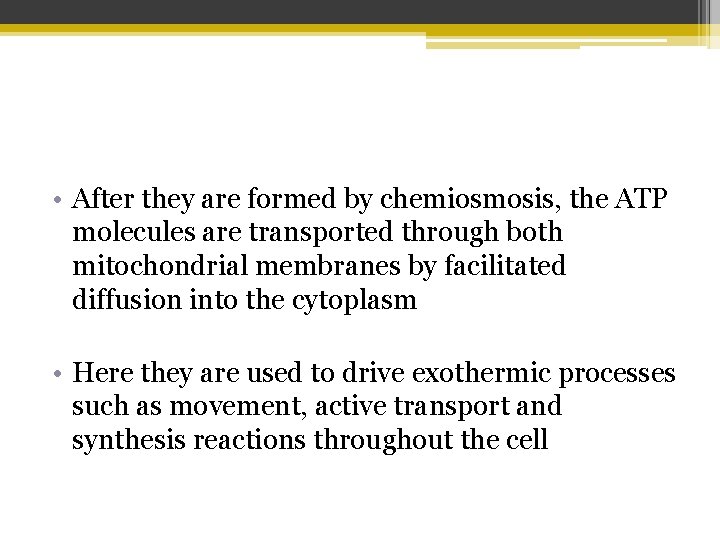
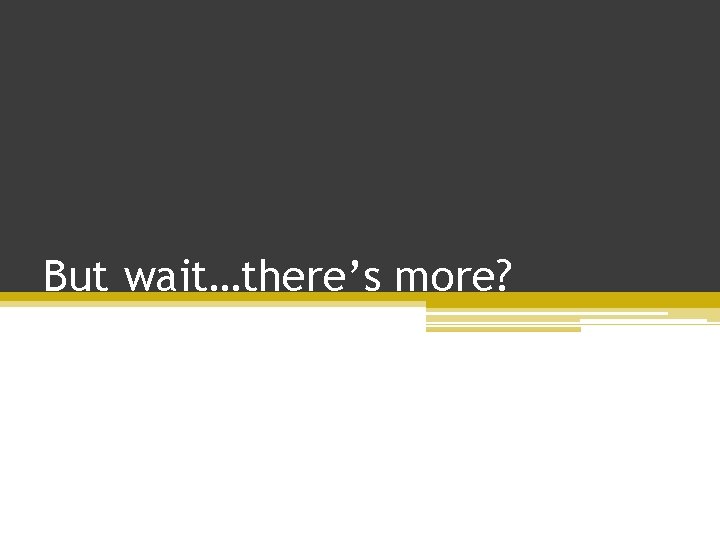
- Slides: 20
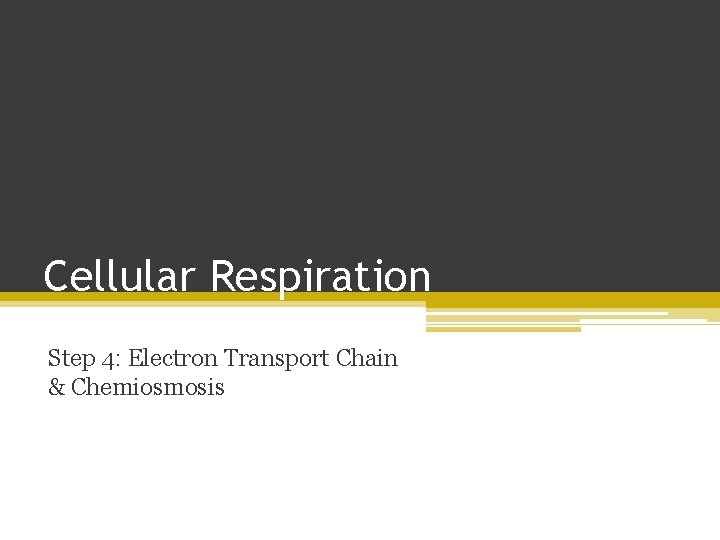
Cellular Respiration Step 4: Electron Transport Chain & Chemiosmosis
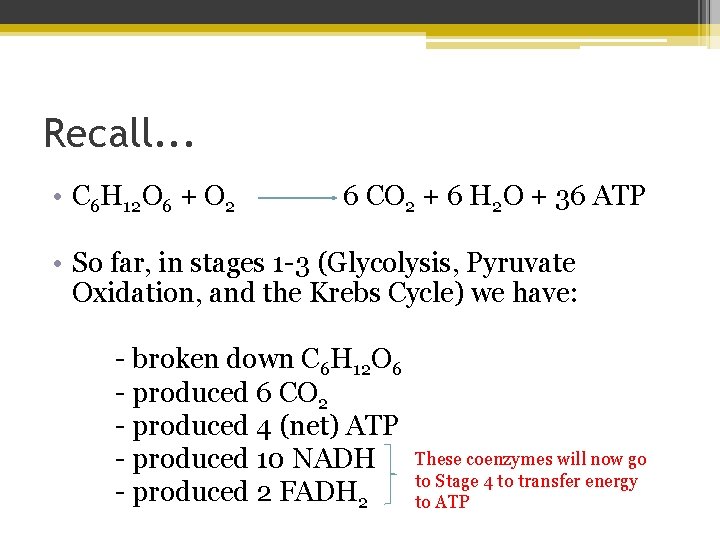
Recall. . . • C 6 H 12 O 6 + O 2 6 CO 2 + 6 H 2 O + 36 ATP • So far, in stages 1 -3 (Glycolysis, Pyruvate Oxidation, and the Krebs Cycle) we have: - broken down C 6 H 12 O 6 - produced 6 CO 2 - produced 4 (net) ATP - produced 10 NADH - produced 2 FADH 2 These coenzymes will now go to Stage 4 to transfer energy to ATP
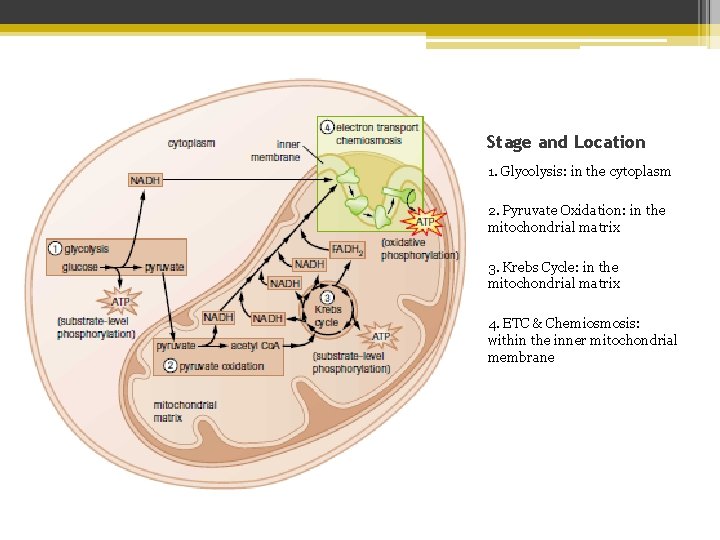
Stage and Location 1. Glycolysis: in the cytoplasm 2. Pyruvate Oxidation: in the mitochondrial matrix 3. Krebs Cycle: in the mitochondrial matrix 4. ETC & Chemiosmosis: within the inner mitochondrial membrane
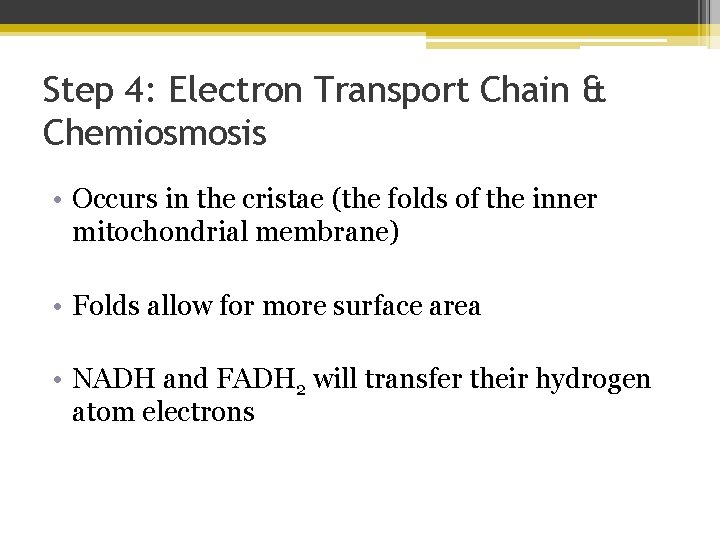
Step 4: Electron Transport Chain & Chemiosmosis • Occurs in the cristae (the folds of the inner mitochondrial membrane) • Folds allow for more surface area • NADH and FADH 2 will transfer their hydrogen atom electrons
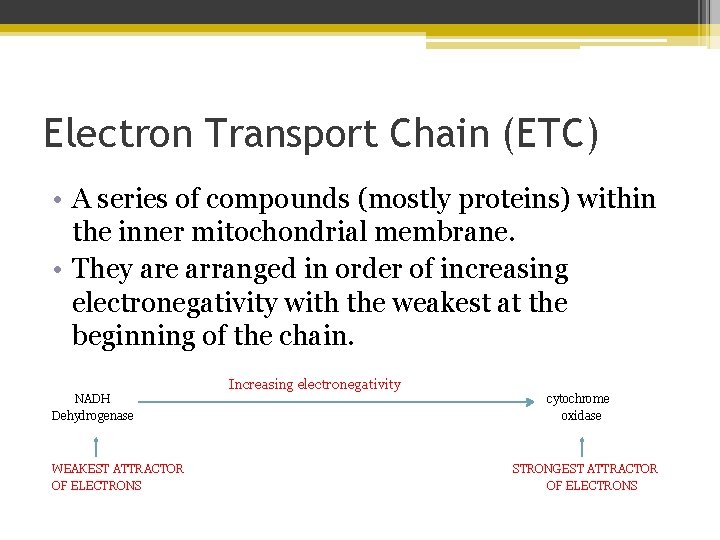
Electron Transport Chain (ETC) • A series of compounds (mostly proteins) within the inner mitochondrial membrane. • They are arranged in order of increasing electronegativity with the weakest at the beginning of the chain. NADH Dehydrogenase WEAKEST ATTRACTOR OF ELECTRONS Increasing electronegativity cytochrome oxidase STRONGEST ATTRACTOR OF ELECTRONS
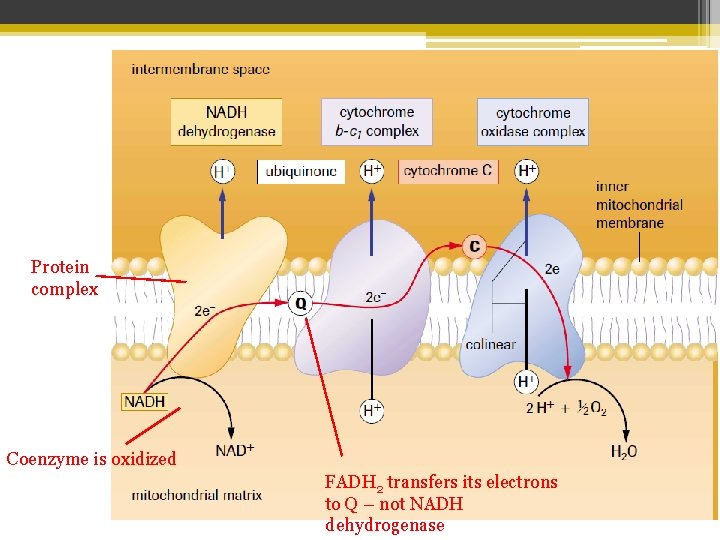
Protein complex Coenzyme is oxidized FADH 2 transfers its electrons to Q – not NADH dehydrogenase
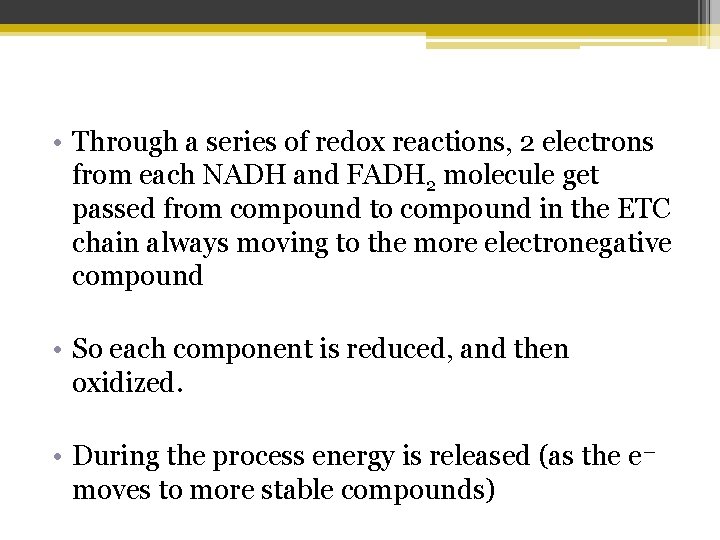
• Through a series of redox reactions, 2 electrons from each NADH and FADH 2 molecule get passed from compound to compound in the ETC chain always moving to the more electronegative compound • So each component is reduced, and then oxidized. • During the process energy is released (as the e― moves to more stable compounds)
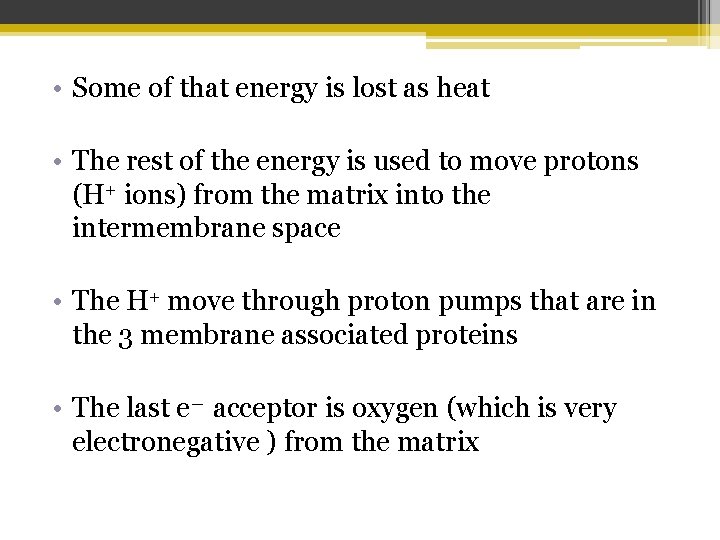
• Some of that energy is lost as heat • The rest of the energy is used to move protons (H+ ions) from the matrix into the intermembrane space • The H+ move through proton pumps that are in the 3 membrane associated proteins • The last e― acceptor is oxygen (which is very electronegative ) from the matrix
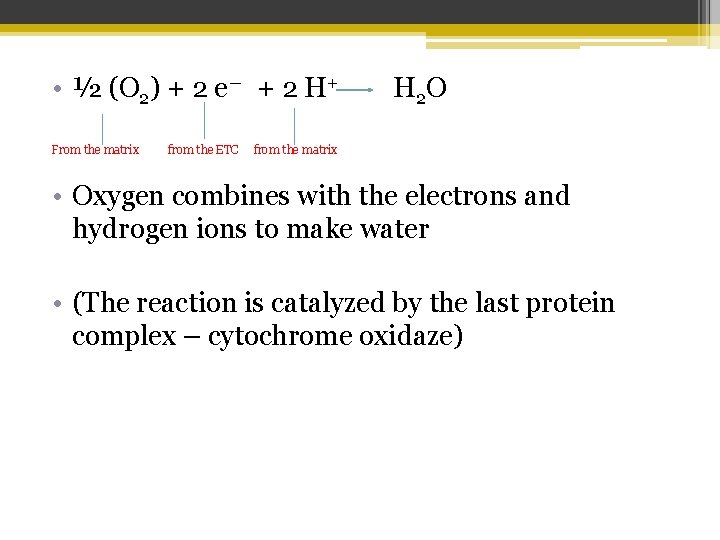
• ½ (O 2) + 2 e― + 2 H+ From the matrix from the ETC H 2 O from the matrix • Oxygen combines with the electrons and hydrogen ions to make water • (The reaction is catalyzed by the last protein complex – cytochrome oxidaze)
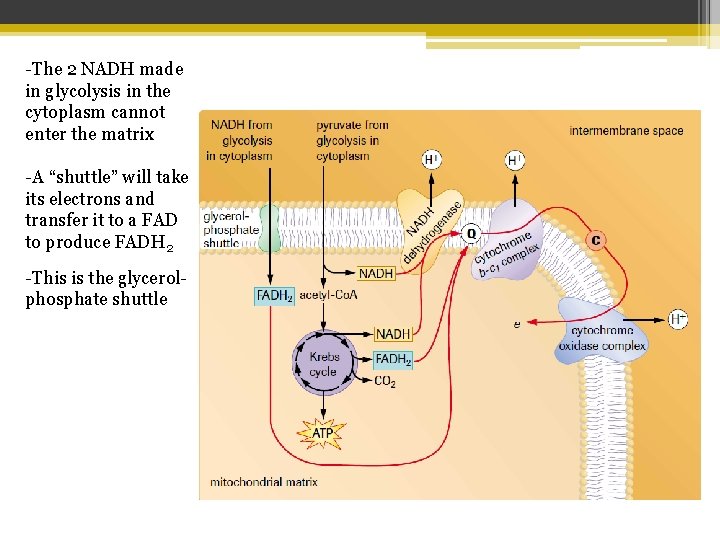
-The 2 NADH made in glycolysis in the cytoplasm cannot enter the matrix -A “shuttle” will take its electrons and transfer it to a FAD to produce FADH 2 -This is the glycerolphosphate shuttle
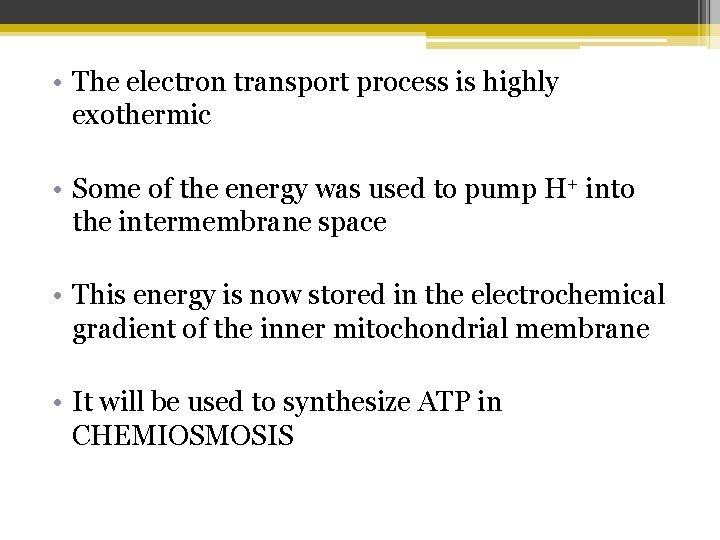
• The electron transport process is highly exothermic • Some of the energy was used to pump H+ into the intermembrane space • This energy is now stored in the electrochemical gradient of the inner mitochondrial membrane • It will be used to synthesize ATP in CHEMIOSMOSIS
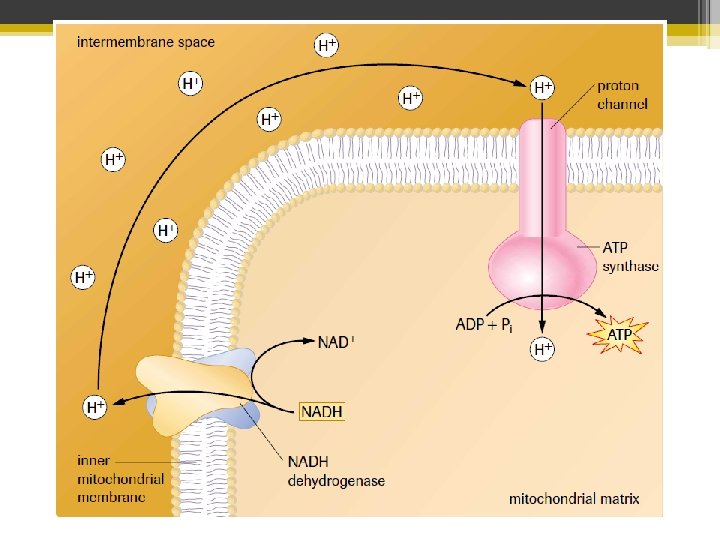
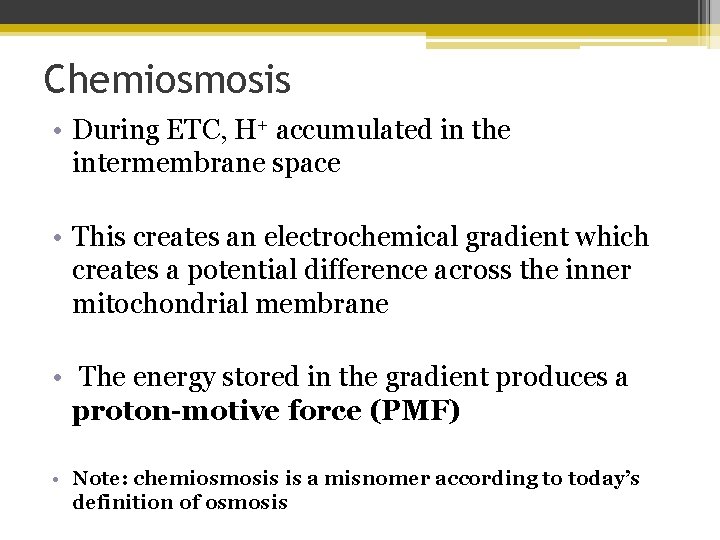
Chemiosmosis • During ETC, H+ accumulated in the intermembrane space • This creates an electrochemical gradient which creates a potential difference across the inner mitochondrial membrane • The energy stored in the gradient produces a proton-motive force (PMF) • Note: chemiosmosis is a misnomer according to today’s definition of osmosis
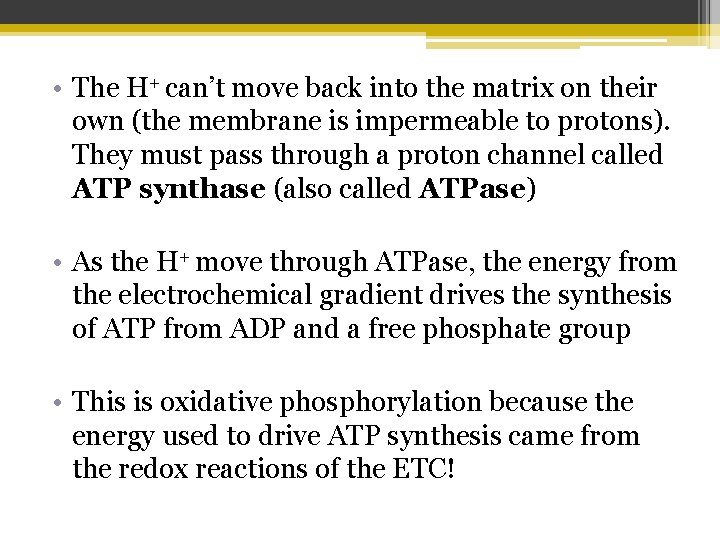
• The H+ can’t move back into the matrix on their own (the membrane is impermeable to protons). They must pass through a proton channel called ATP synthase (also called ATPase) • As the H+ move through ATPase, the energy from the electrochemical gradient drives the synthesis of ATP from ADP and a free phosphate group • This is oxidative phosphorylation because the energy used to drive ATP synthesis came from the redox reactions of the ETC!
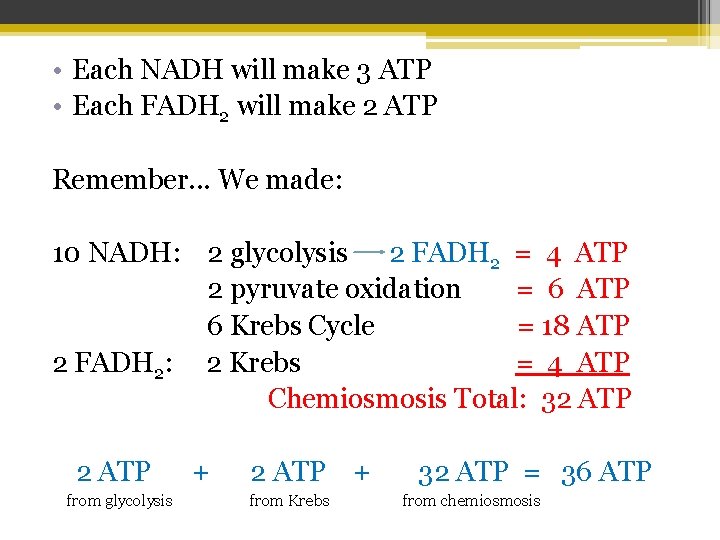
• Each NADH will make 3 ATP • Each FADH 2 will make 2 ATP Remember. . . We made: 10 NADH: 2 glycolysis 2 FADH 2 = 4 ATP 2 pyruvate oxidation = 6 ATP 6 Krebs Cycle = 18 ATP 2 FADH 2: 2 Krebs = 4 ATP Chemiosmosis Total: 32 ATP from glycolysis + 2 ATP + from Krebs 32 ATP = 36 ATP from chemiosmosis
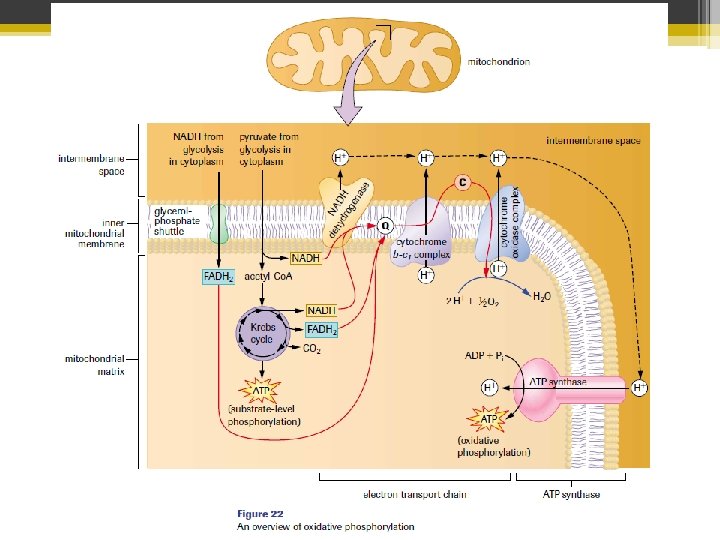
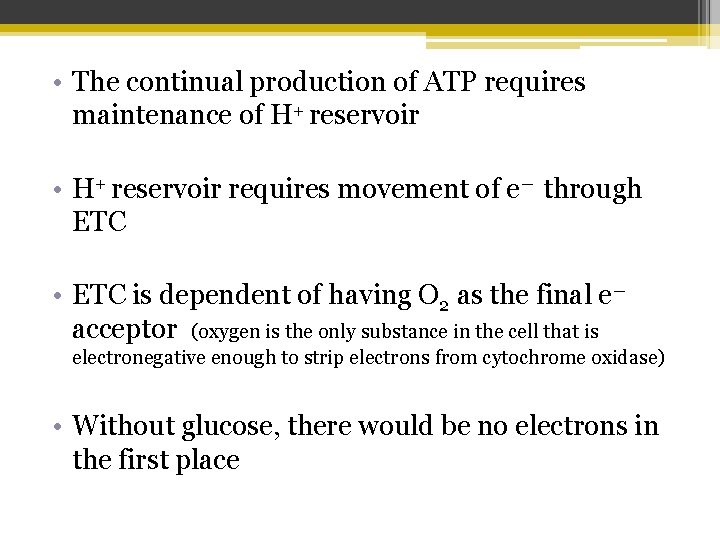
• The continual production of ATP requires maintenance of H+ reservoir • H+ reservoir requires movement of e― through ETC • ETC is dependent of having O 2 as the final e― acceptor (oxygen is the only substance in the cell that is electronegative enough to strip electrons from cytochrome oxidase) • Without glucose, there would be no electrons in the first place
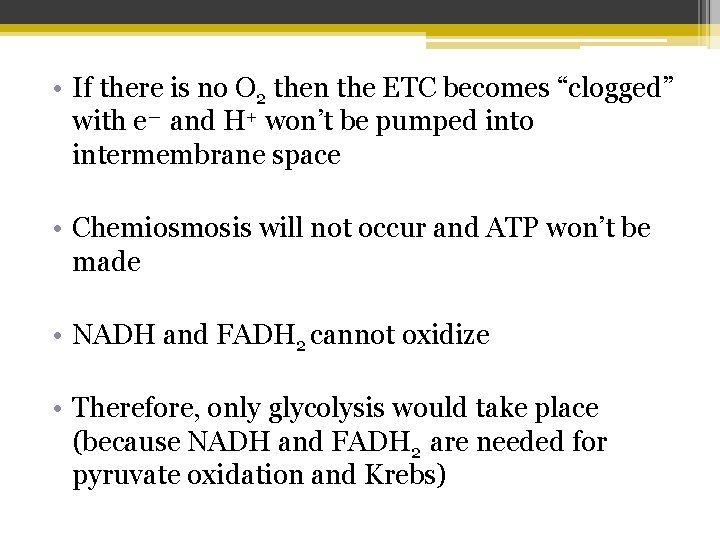
• If there is no O 2 then the ETC becomes “clogged” with e― and H+ won’t be pumped into intermembrane space • Chemiosmosis will not occur and ATP won’t be made • NADH and FADH 2 cannot oxidize • Therefore, only glycolysis would take place (because NADH and FADH 2 are needed for pyruvate oxidation and Krebs)
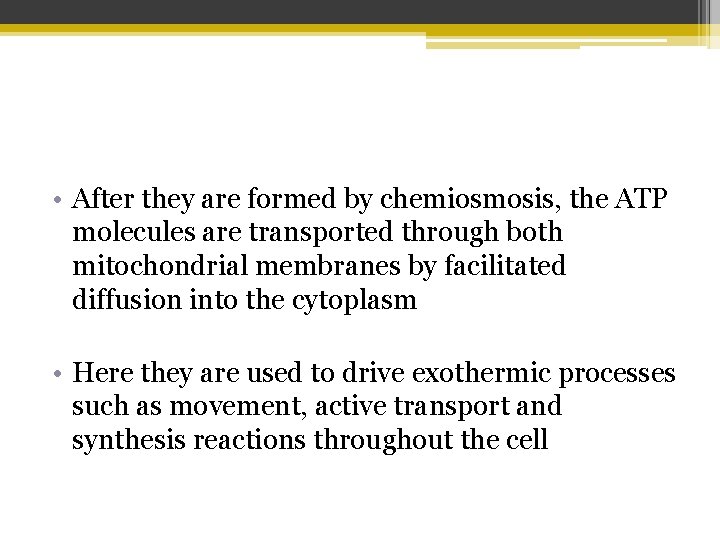
• After they are formed by chemiosmosis, the ATP molecules are transported through both mitochondrial membranes by facilitated diffusion into the cytoplasm • Here they are used to drive exothermic processes such as movement, active transport and synthesis reactions throughout the cell
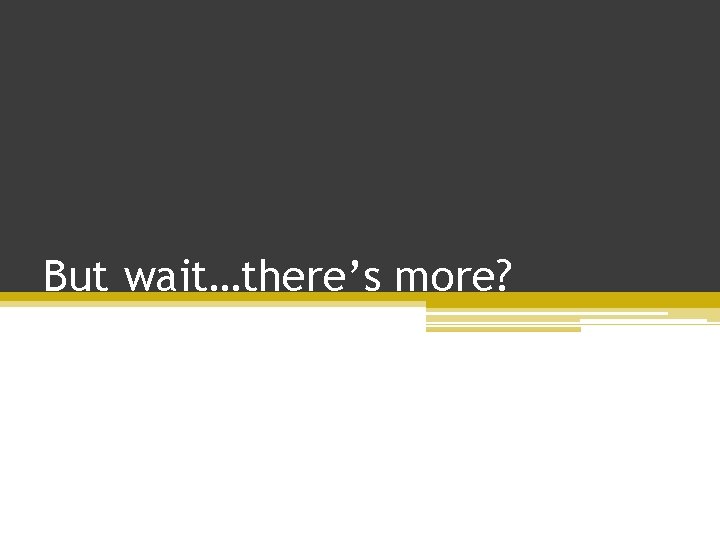
But wait…there’s more?
Electron transport chain cellular respiration
Electron transport chain cellular respiration
Step 1 step 2 step 3 step 4
What happens during glycolysis
Electron carriers in cellular respiration
Fermentation
Sodium azide electron transport chain
Tca回路
Figure retoriche pearson
Electron transport chain complexes
Where does the krebs cycle take place?
Catabolism
What does aerobic cellular respiration mean
Electron transport chain
Cellular respiration harvesting chemical energy
Electron transport chain
Significance of electron transport chain
Electron transport chain
Components of electron transport chain
Tca cycle animation
Citric acid cycle and electron transport chain