A twostate homology model of the h ERG
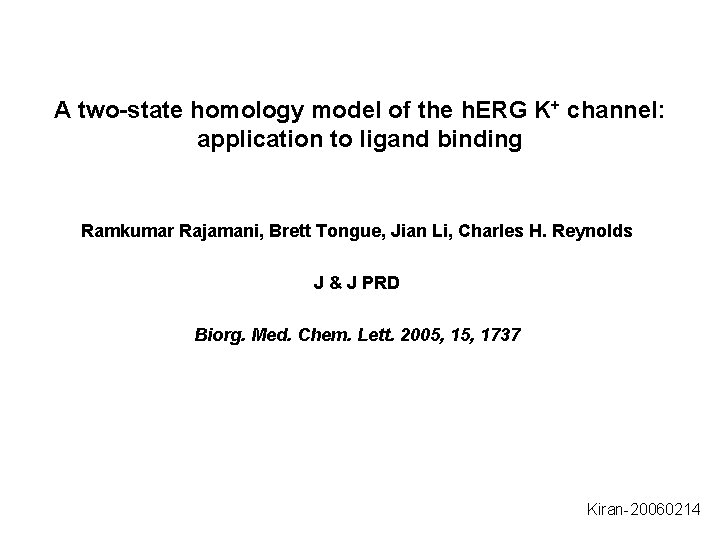
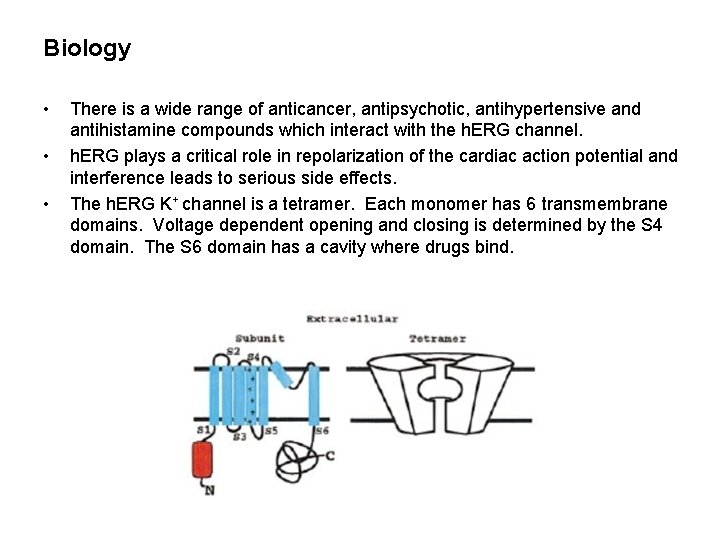
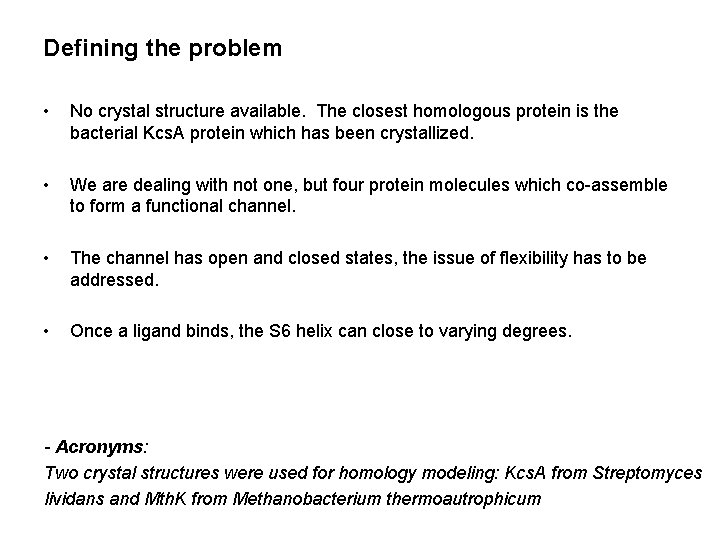
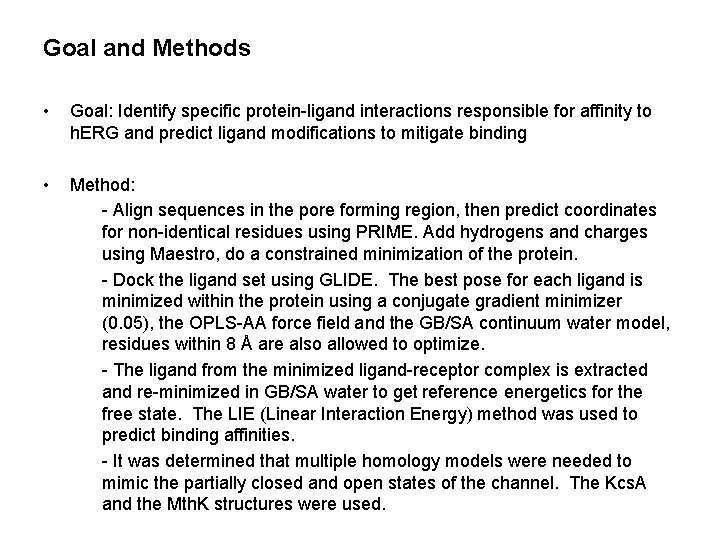
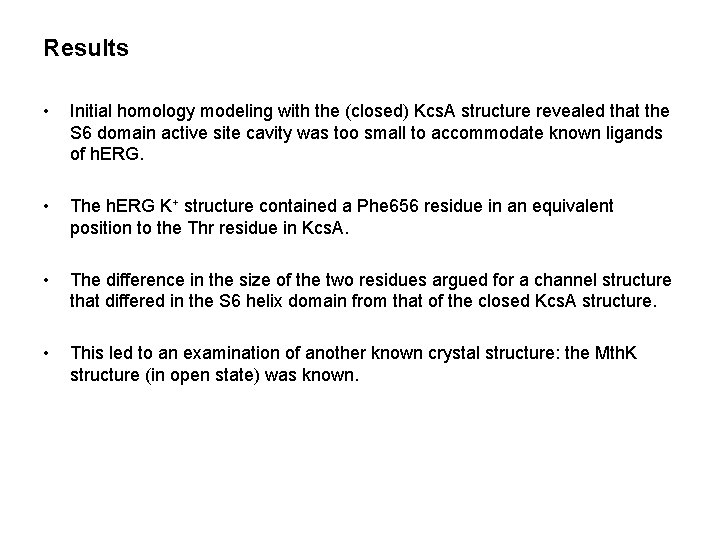
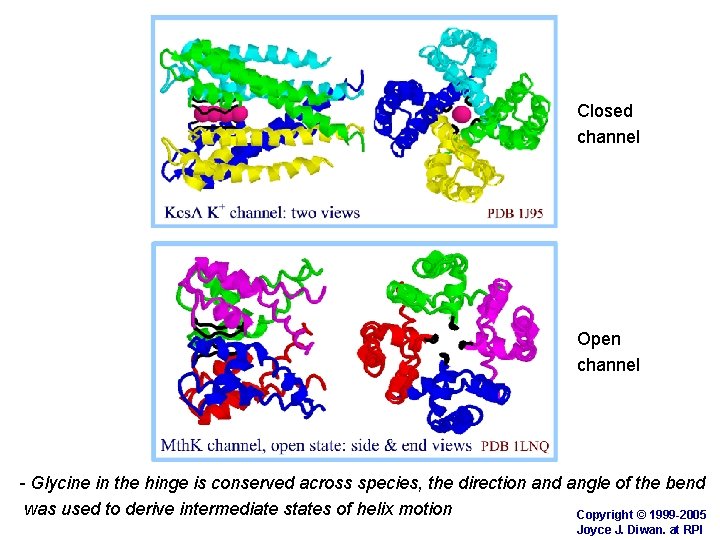
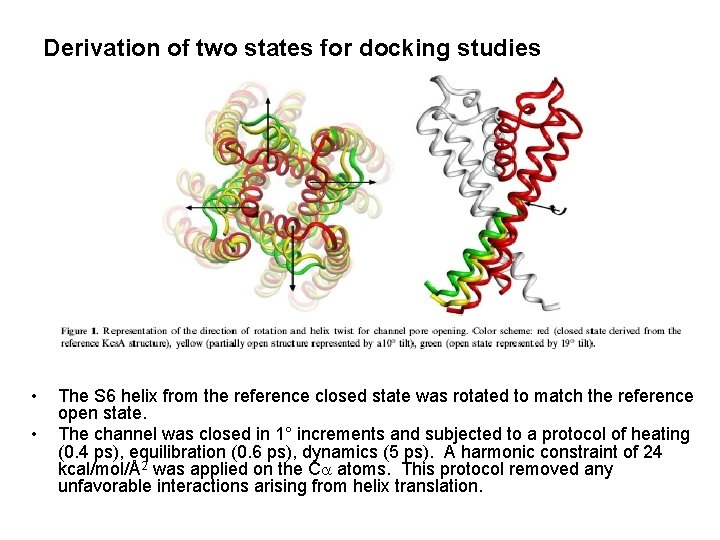
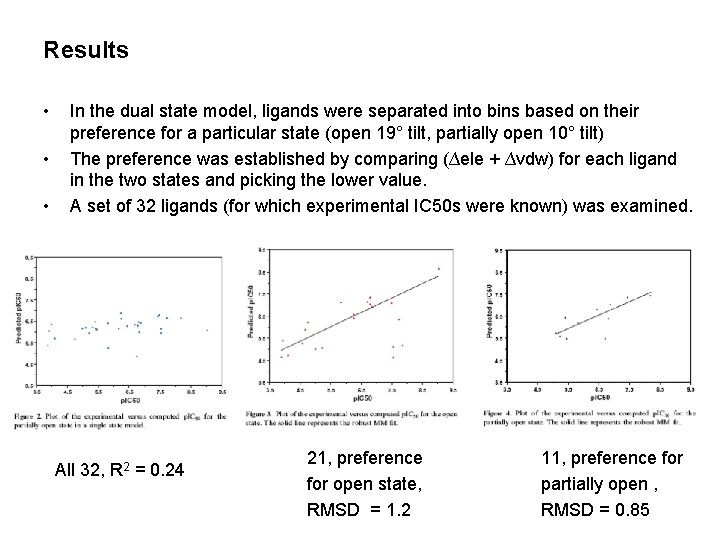
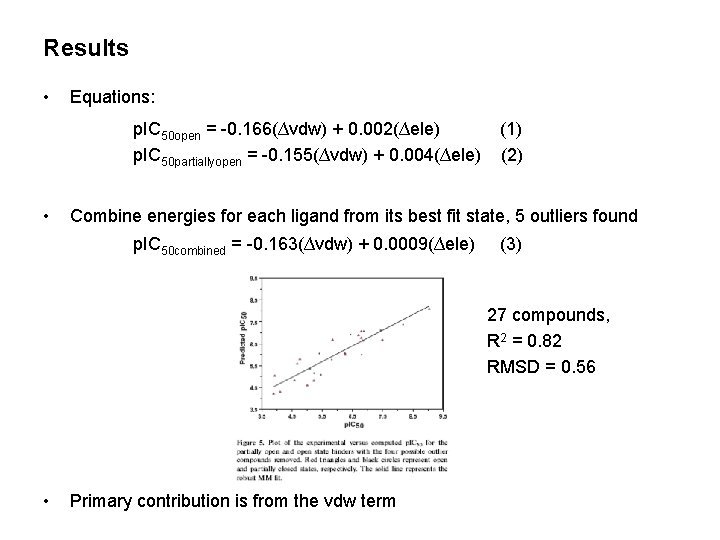
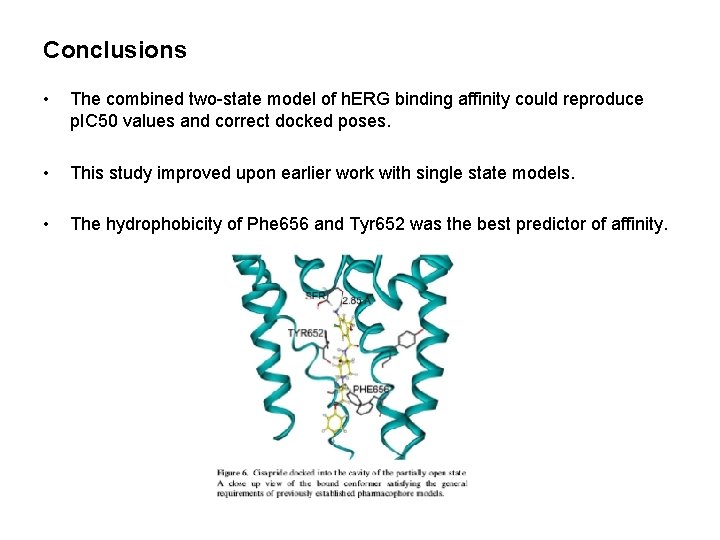
- Slides: 10
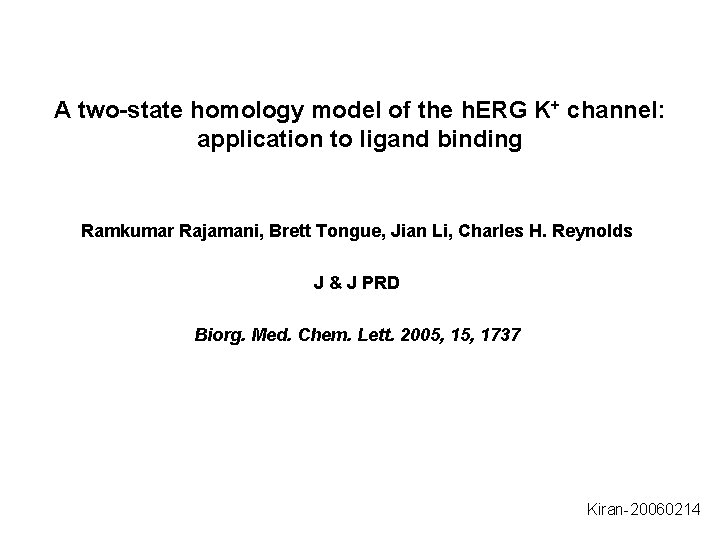
A two-state homology model of the h. ERG K+ channel: application to ligand binding Ramkumar Rajamani, Brett Tongue, Jian Li, Charles H. Reynolds J & J PRD Biorg. Med. Chem. Lett. 2005, 1737 Kiran-20060214
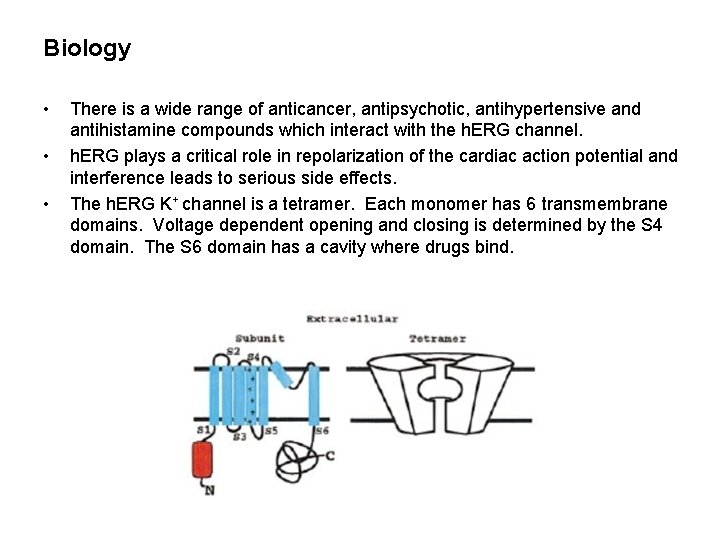
Biology • • • There is a wide range of anticancer, antipsychotic, antihypertensive and antihistamine compounds which interact with the h. ERG channel. h. ERG plays a critical role in repolarization of the cardiac action potential and interference leads to serious side effects. The h. ERG K+ channel is a tetramer. Each monomer has 6 transmembrane domains. Voltage dependent opening and closing is determined by the S 4 domain. The S 6 domain has a cavity where drugs bind.
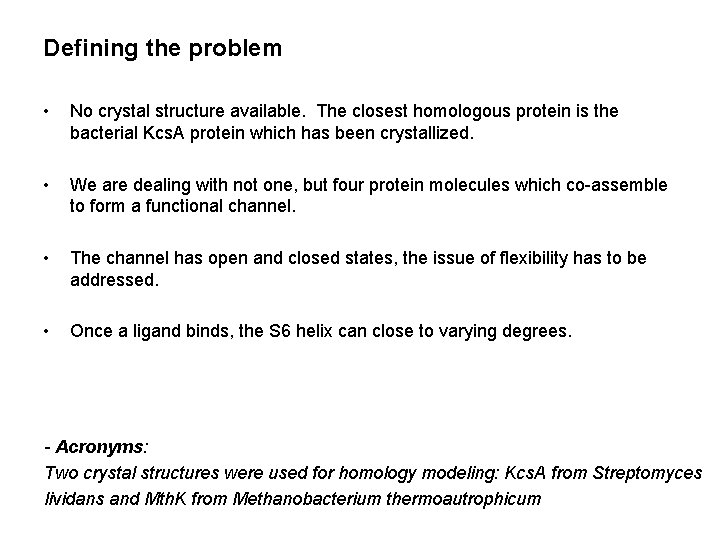
Defining the problem • No crystal structure available. The closest homologous protein is the bacterial Kcs. A protein which has been crystallized. • We are dealing with not one, but four protein molecules which co-assemble to form a functional channel. • The channel has open and closed states, the issue of flexibility has to be addressed. • Once a ligand binds, the S 6 helix can close to varying degrees. - Acronyms: Two crystal structures were used for homology modeling: Kcs. A from Streptomyces lividans and Mth. K from Methanobacterium thermoautrophicum
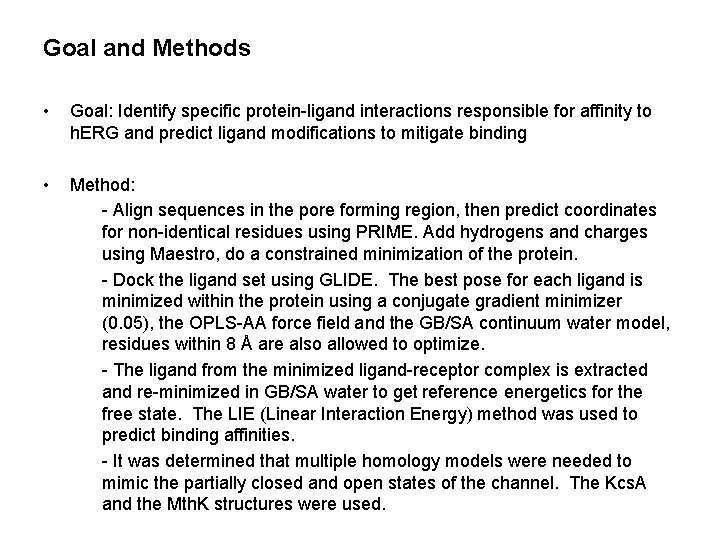
Goal and Methods • Goal: Identify specific protein-ligand interactions responsible for affinity to h. ERG and predict ligand modifications to mitigate binding • Method: - Align sequences in the pore forming region, then predict coordinates for non-identical residues using PRIME. Add hydrogens and charges using Maestro, do a constrained minimization of the protein. - Dock the ligand set using GLIDE. The best pose for each ligand is minimized within the protein using a conjugate gradient minimizer (0. 05), the OPLS-AA force field and the GB/SA continuum water model, residues within 8 Å are also allowed to optimize. - The ligand from the minimized ligand-receptor complex is extracted and re-minimized in GB/SA water to get reference energetics for the free state. The LIE (Linear Interaction Energy) method was used to predict binding affinities. - It was determined that multiple homology models were needed to mimic the partially closed and open states of the channel. The Kcs. A and the Mth. K structures were used.
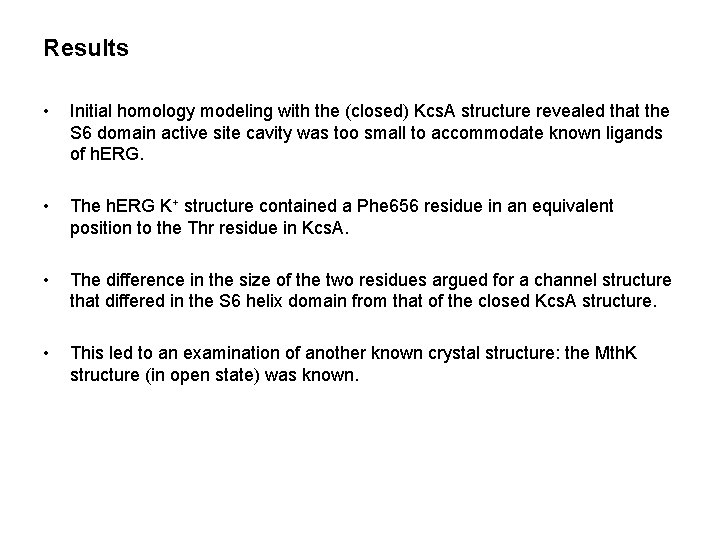
Results • Initial homology modeling with the (closed) Kcs. A structure revealed that the S 6 domain active site cavity was too small to accommodate known ligands of h. ERG. • The h. ERG K+ structure contained a Phe 656 residue in an equivalent position to the Thr residue in Kcs. A. • The difference in the size of the two residues argued for a channel structure that differed in the S 6 helix domain from that of the closed Kcs. A structure. • This led to an examination of another known crystal structure: the Mth. K structure (in open state) was known.
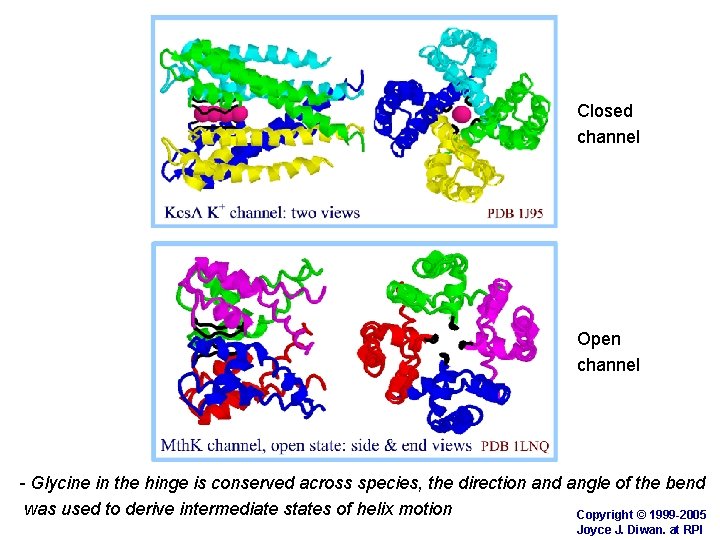
Closed channel Open channel - Glycine in the hinge is conserved across species, the direction and angle of the bend was used to derive intermediate states of helix motion Copyright © 1999 -2005 Joyce J. Diwan. at RPI
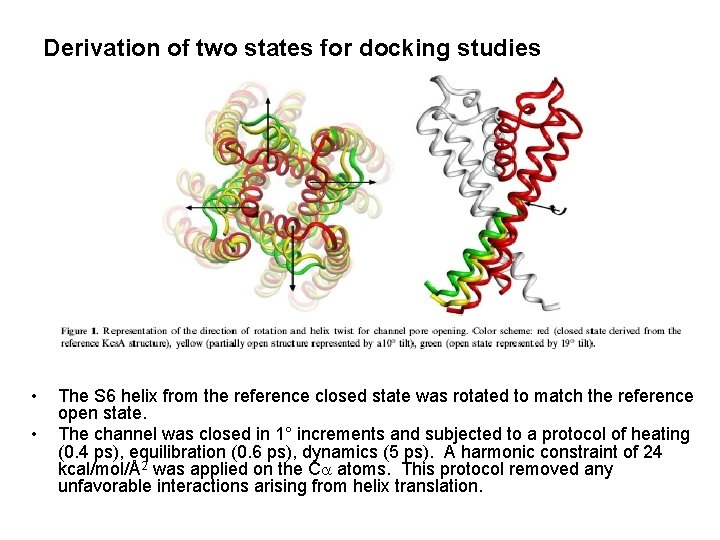
Derivation of two states for docking studies • • The S 6 helix from the reference closed state was rotated to match the reference open state. The channel was closed in 1° increments and subjected to a protocol of heating (0. 4 ps), equilibration (0. 6 ps), dynamics (5 ps). A harmonic constraint of 24 kcal/mol/Å2 was applied on the Ca atoms. This protocol removed any unfavorable interactions arising from helix translation.
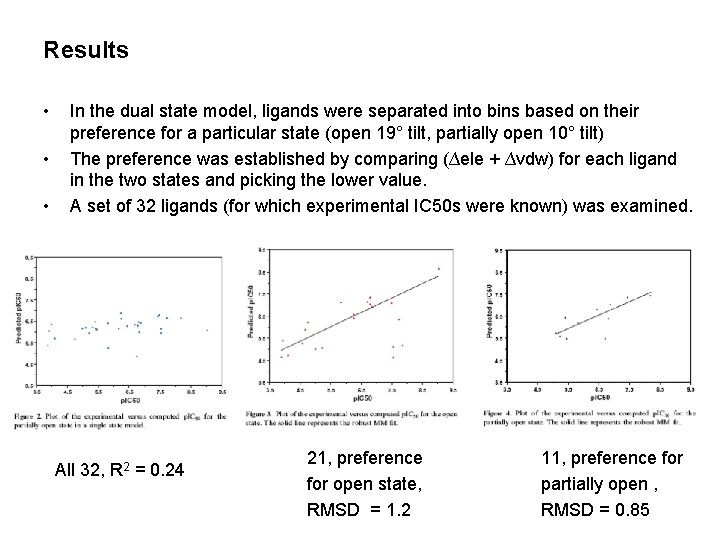
Results • • • In the dual state model, ligands were separated into bins based on their preference for a particular state (open 19° tilt, partially open 10° tilt) The preference was established by comparing (∆ele + ∆vdw) for each ligand in the two states and picking the lower value. A set of 32 ligands (for which experimental IC 50 s were known) was examined. All 32, R 2 = 0. 24 21, preference for open state, RMSD = 1. 2 11, preference for partially open , RMSD = 0. 85
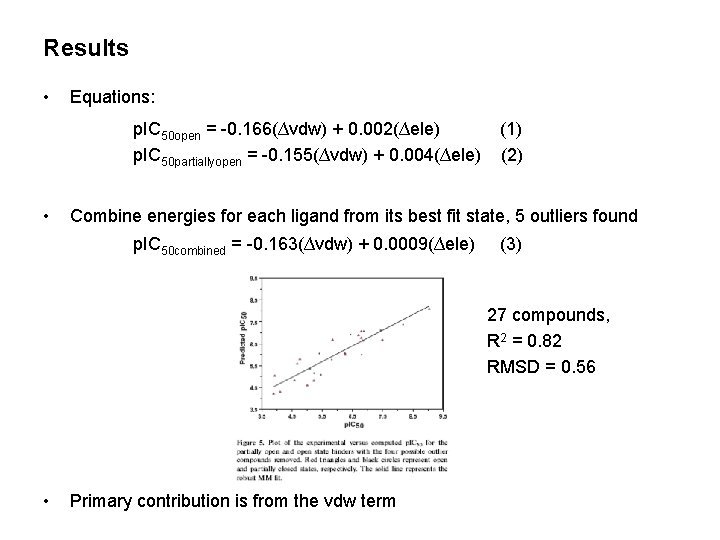
Results • Equations: p. IC 50 open = -0. 166(∆vdw) + 0. 002(∆ele) p. IC 50 partiallyopen = -0. 155(∆vdw) + 0. 004(∆ele) • (1) (2) Combine energies for each ligand from its best fit state, 5 outliers found p. IC 50 combined = -0. 163(∆vdw) + 0. 0009(∆ele) (3) 27 compounds, R 2 = 0. 82 RMSD = 0. 56 • Primary contribution is from the vdw term
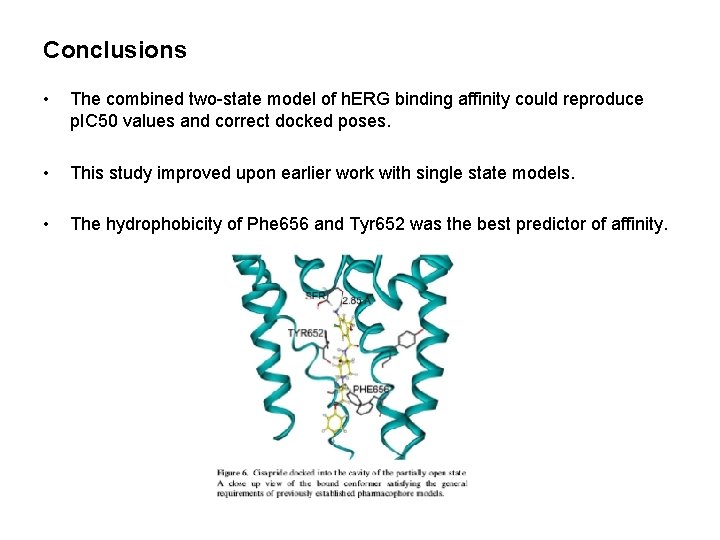
Conclusions • The combined two-state model of h. ERG binding affinity could reproduce p. IC 50 values and correct docked poses. • This study improved upon earlier work with single state models. • The hydrophobicity of Phe 656 and Tyr 652 was the best predictor of affinity.