A 540 Stellar Atmospheres Organizational Details Meeting times
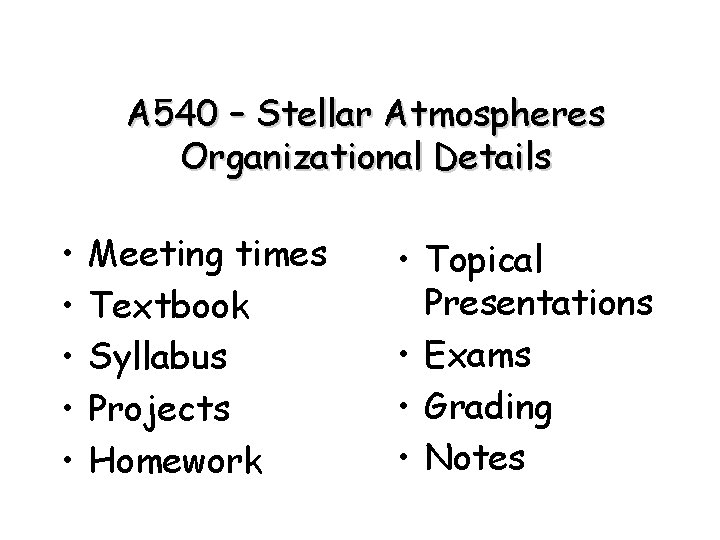
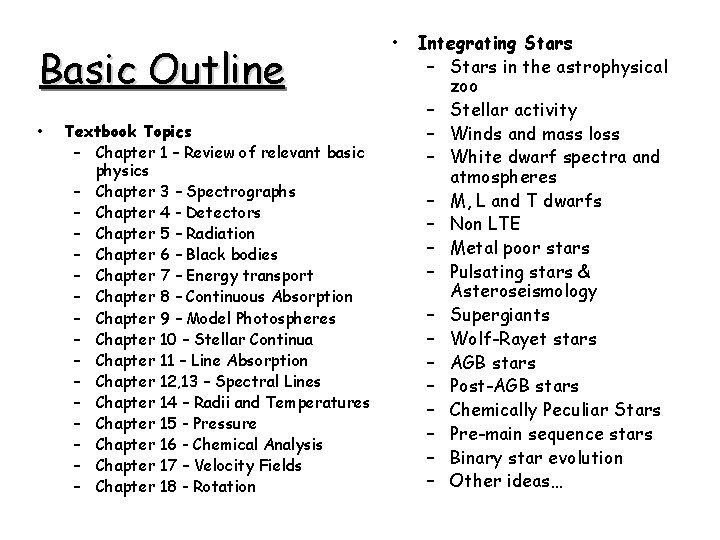
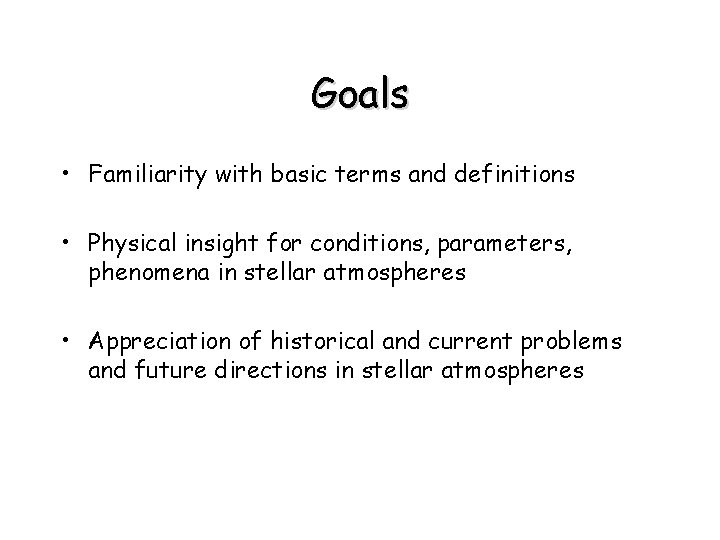
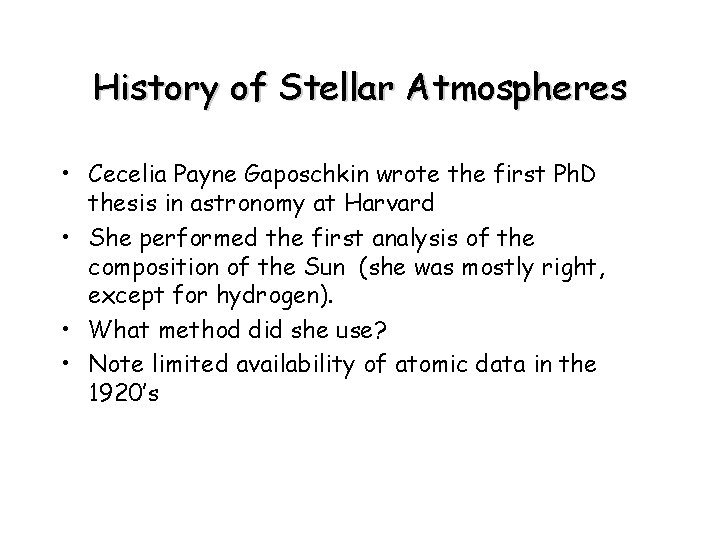
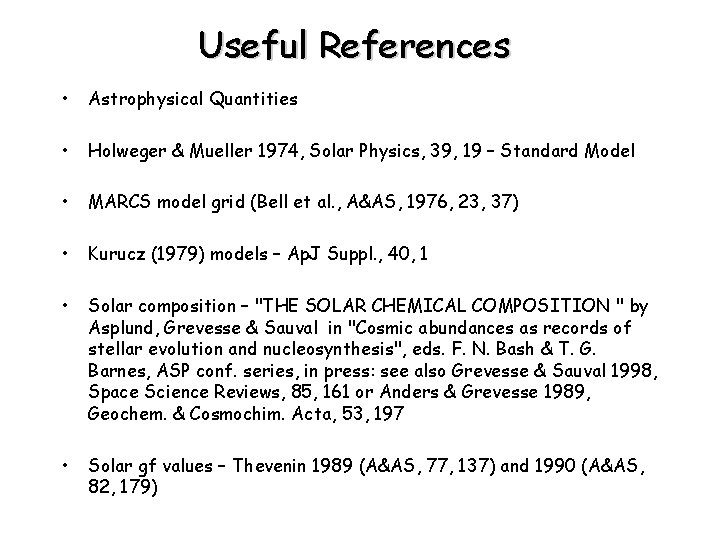
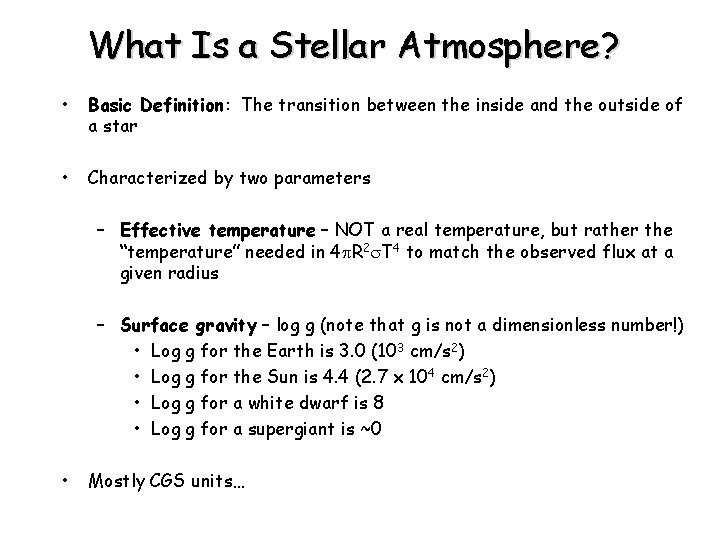
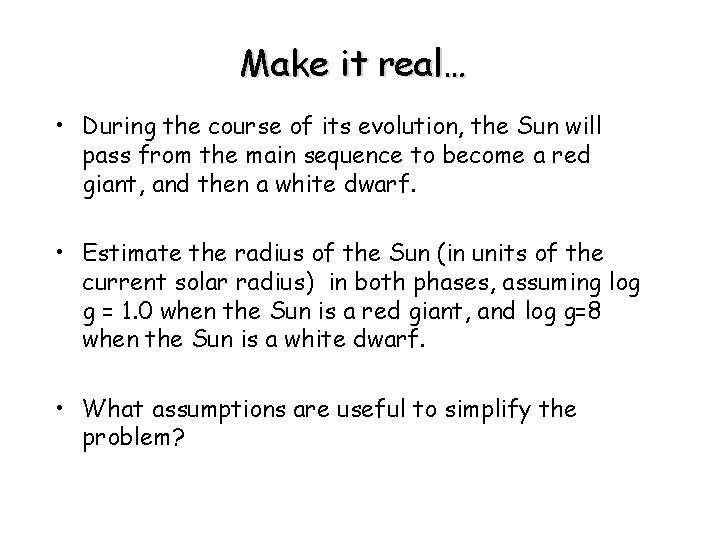
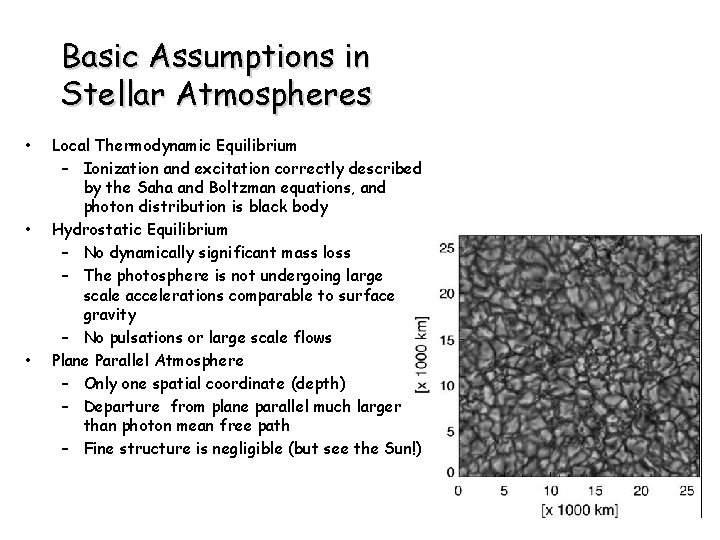
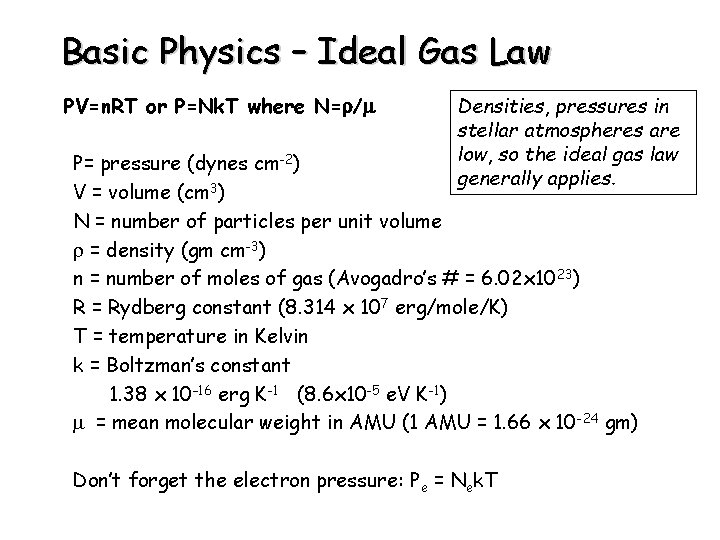
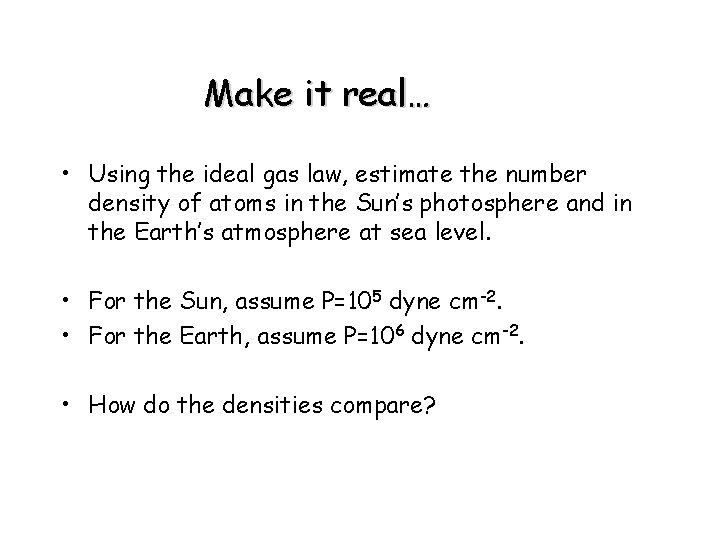
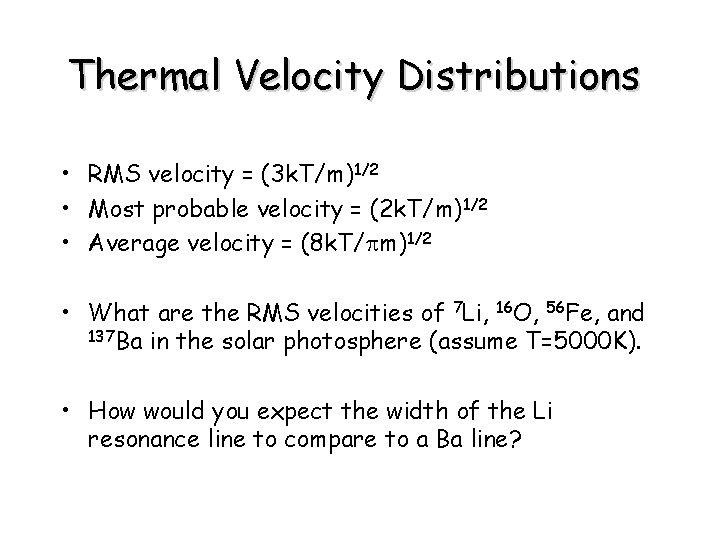
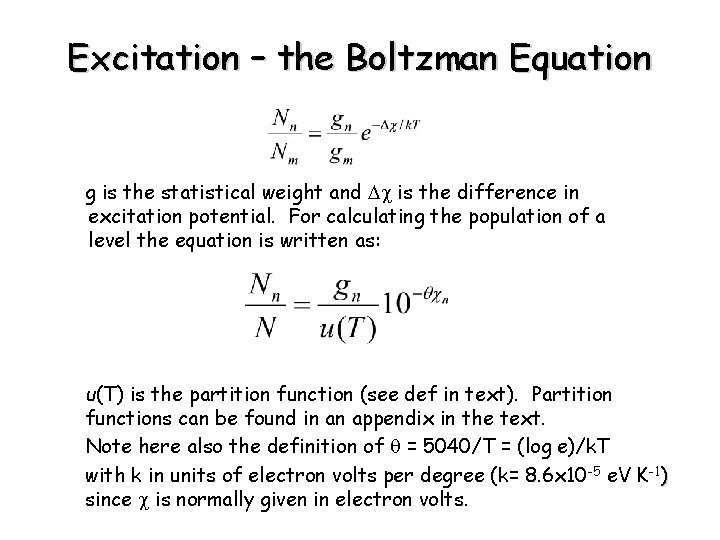
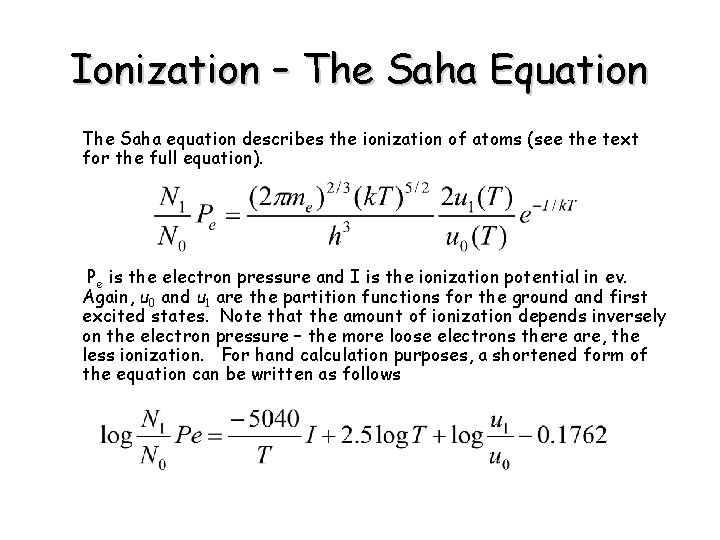
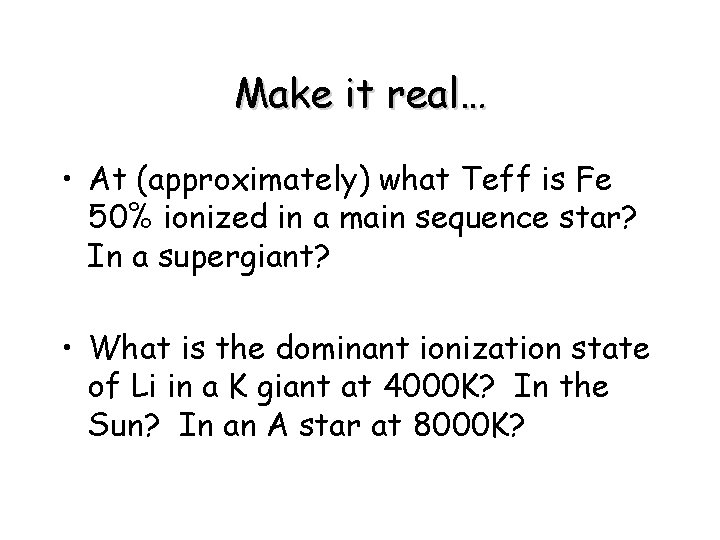
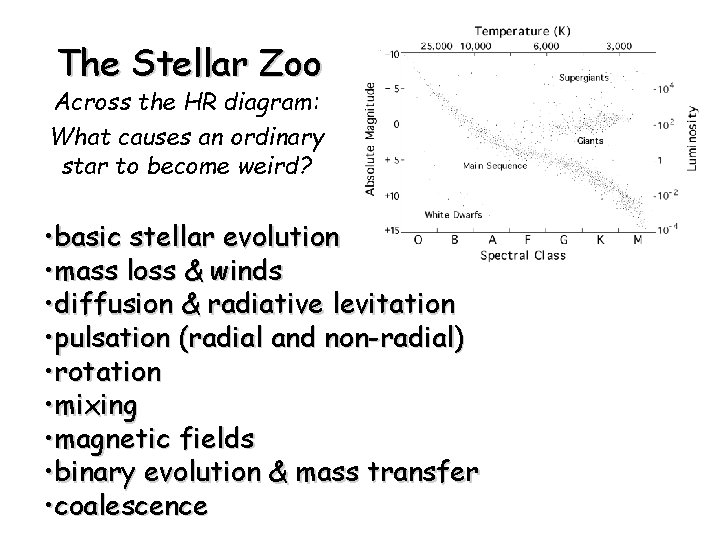
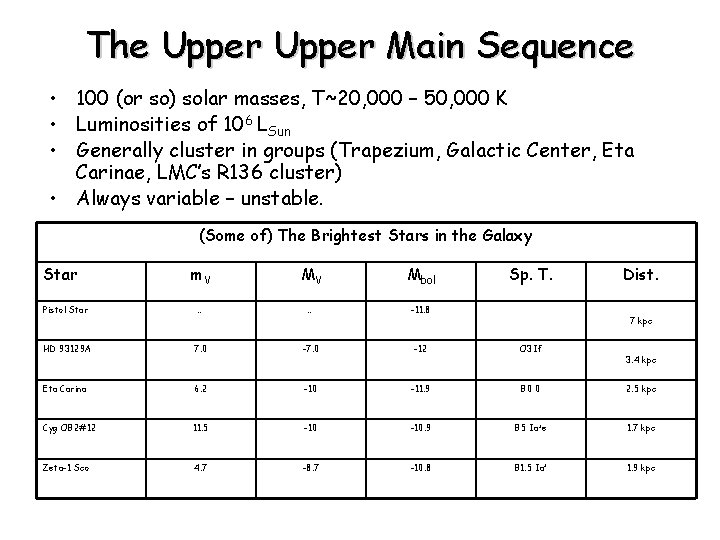
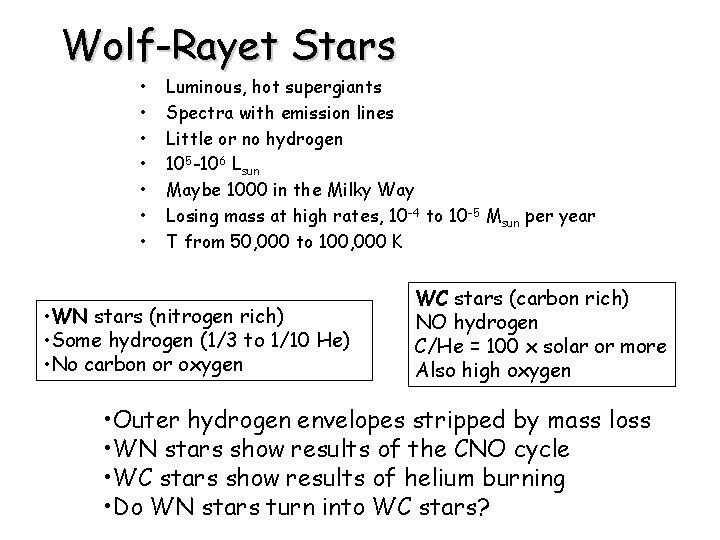
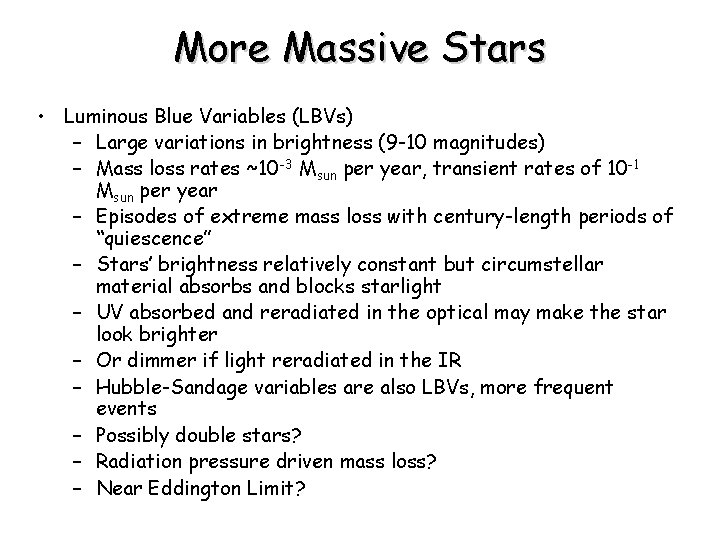
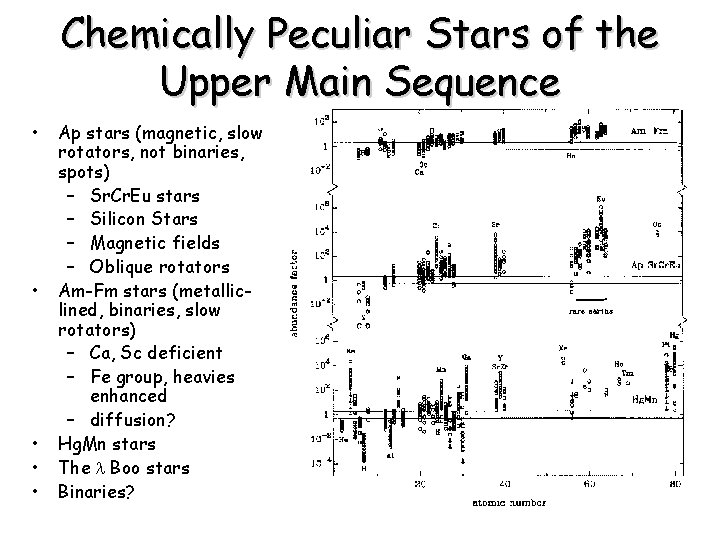
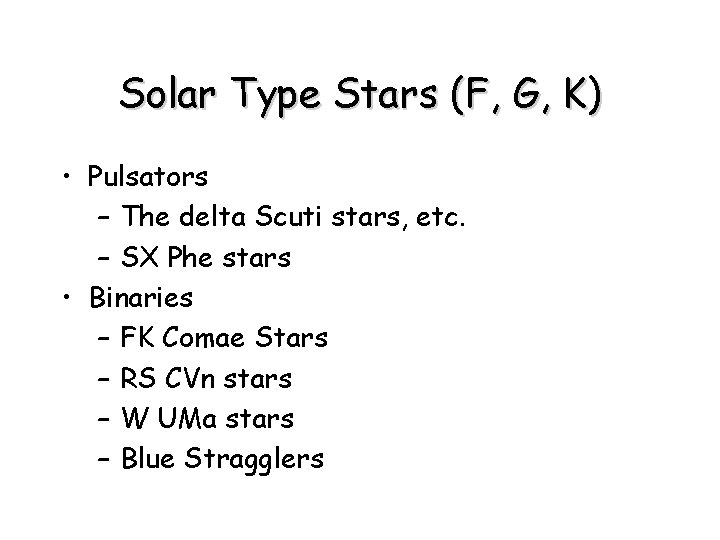
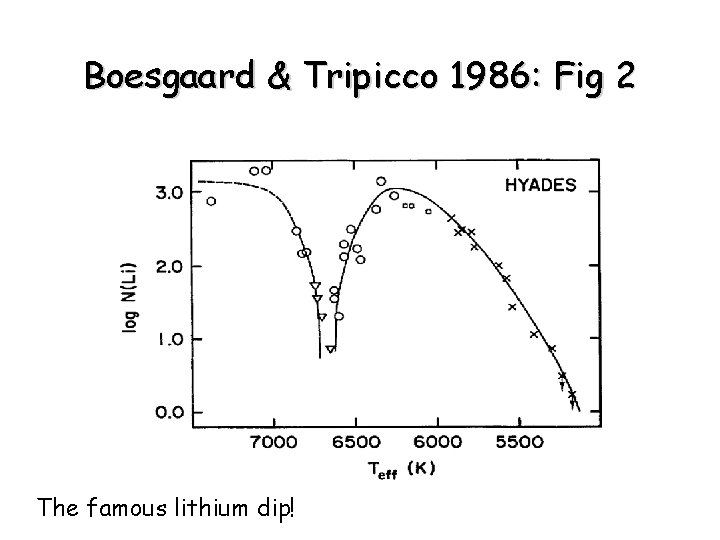
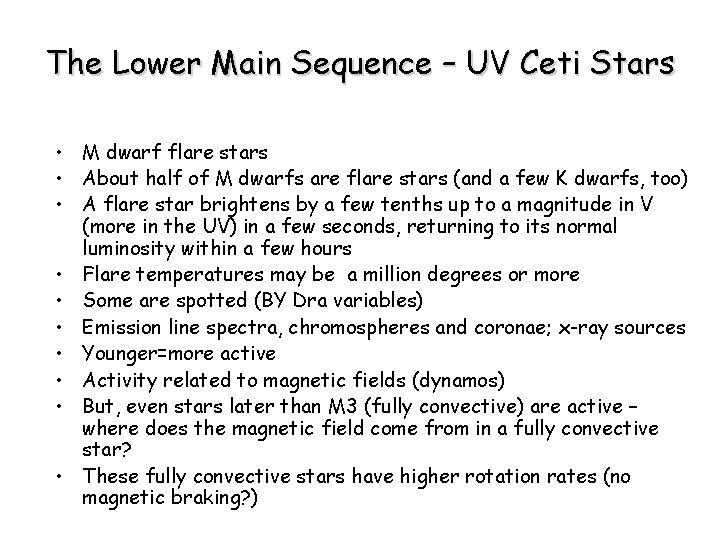
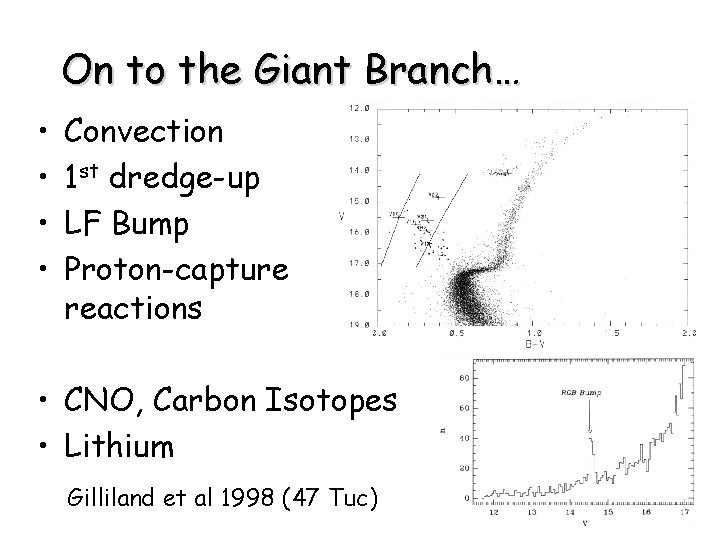
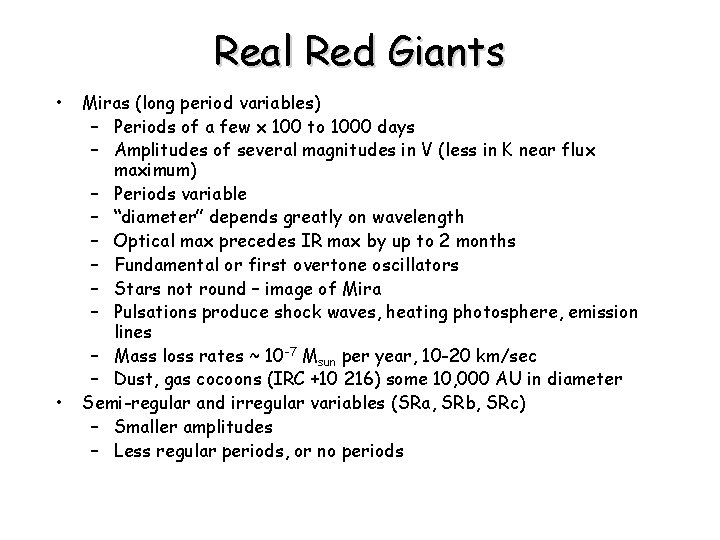
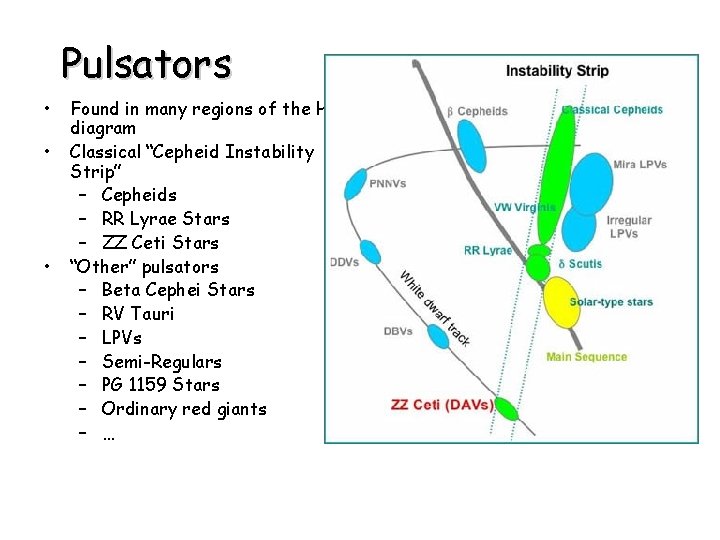
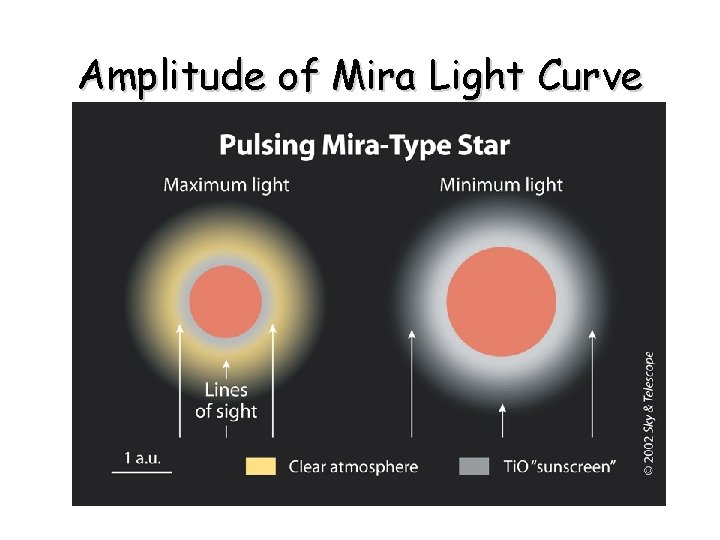
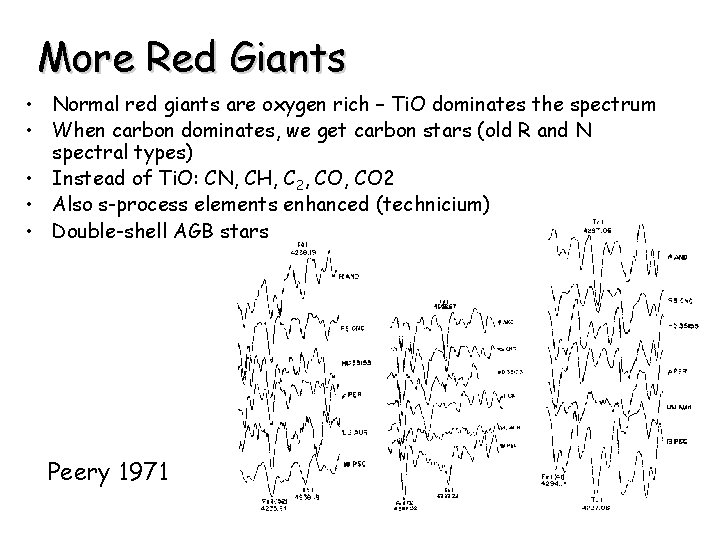
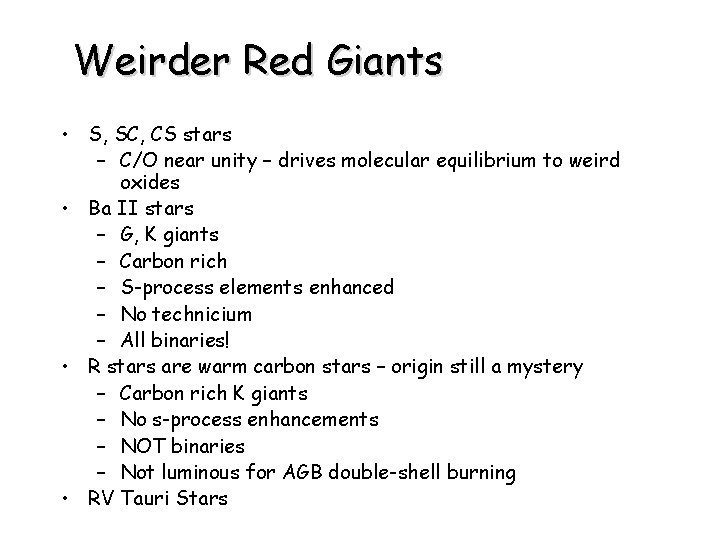
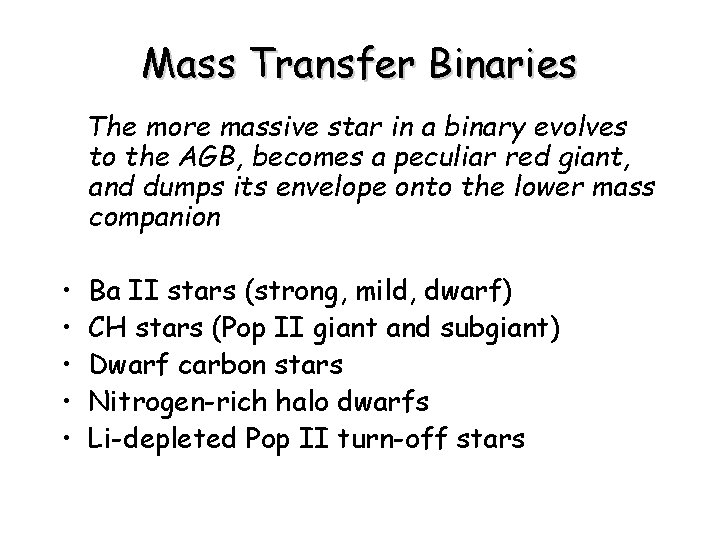
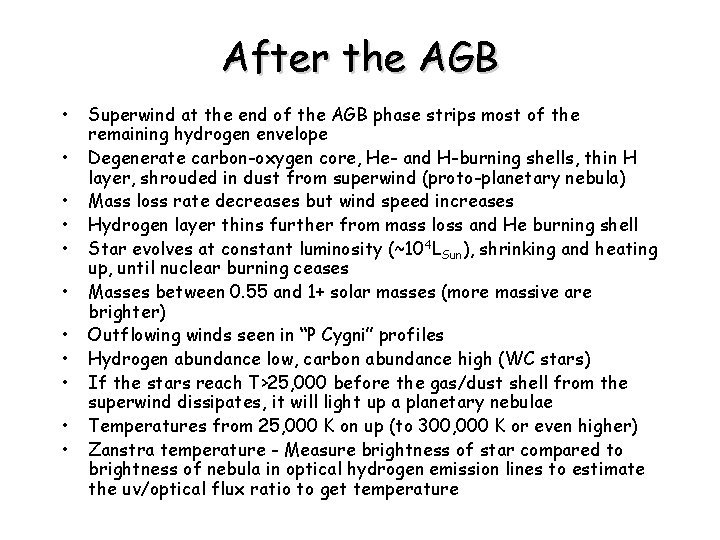
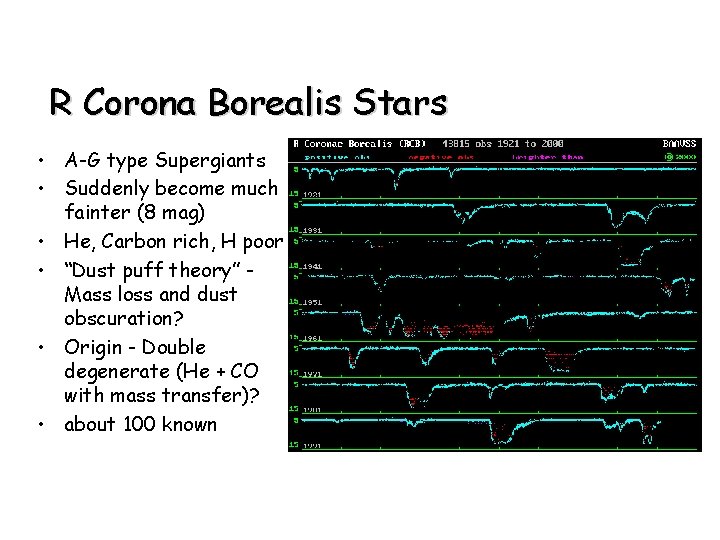
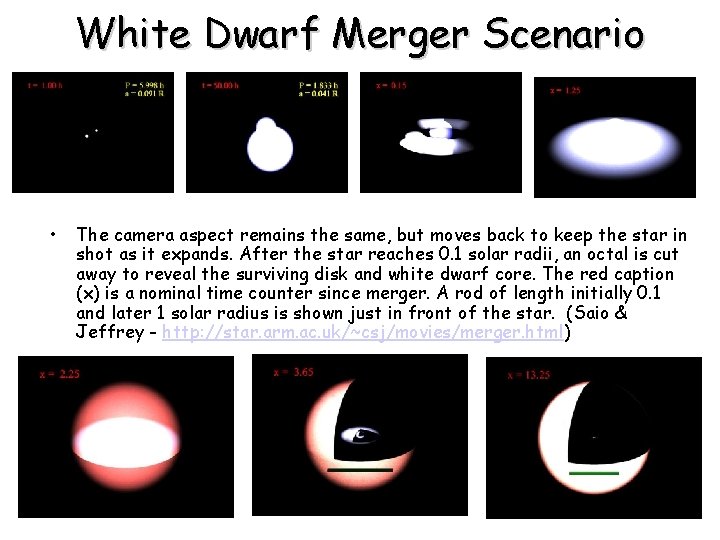
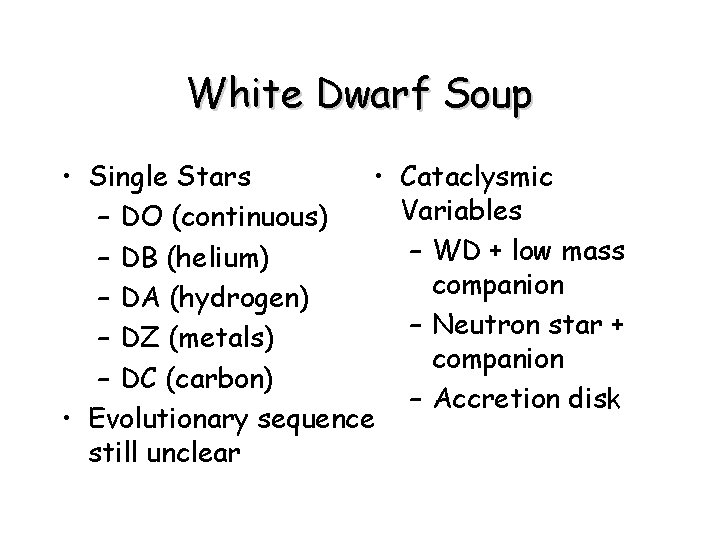
- Slides: 33
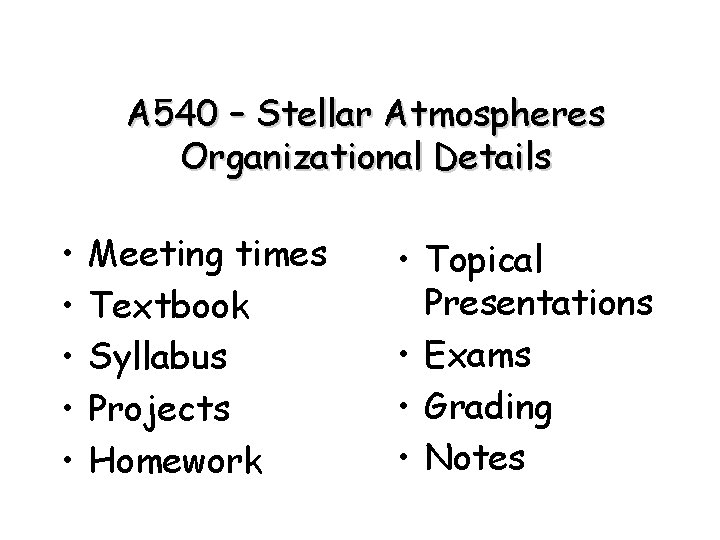
A 540 – Stellar Atmospheres Organizational Details • • • Meeting times Textbook Syllabus Projects Homework • Topical Presentations • Exams • Grading • Notes
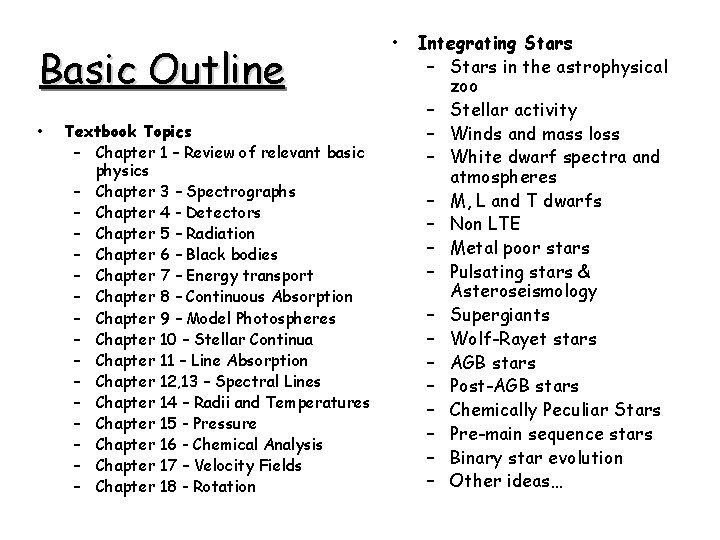
Basic Outline • Textbook Topics – Chapter 1 – Review of relevant basic physics – Chapter 3 – Spectrographs – Chapter 4 - Detectors – Chapter 5 – Radiation – Chapter 6 – Black bodies – Chapter 7 – Energy transport – Chapter 8 – Continuous Absorption – Chapter 9 – Model Photospheres – Chapter 10 – Stellar Continua – Chapter 11 – Line Absorption – Chapter 12, 13 – Spectral Lines – Chapter 14 – Radii and Temperatures – Chapter 15 - Pressure – Chapter 16 - Chemical Analysis – Chapter 17 – Velocity Fields – Chapter 18 - Rotation • Integrating Stars – Stars in the astrophysical zoo – Stellar activity – Winds and mass loss – White dwarf spectra and atmospheres – M, L and T dwarfs – Non LTE – Metal poor stars – Pulsating stars & Asteroseismology – Supergiants – Wolf-Rayet stars – AGB stars – Post-AGB stars – Chemically Peculiar Stars – Pre-main sequence stars – Binary star evolution – Other ideas…
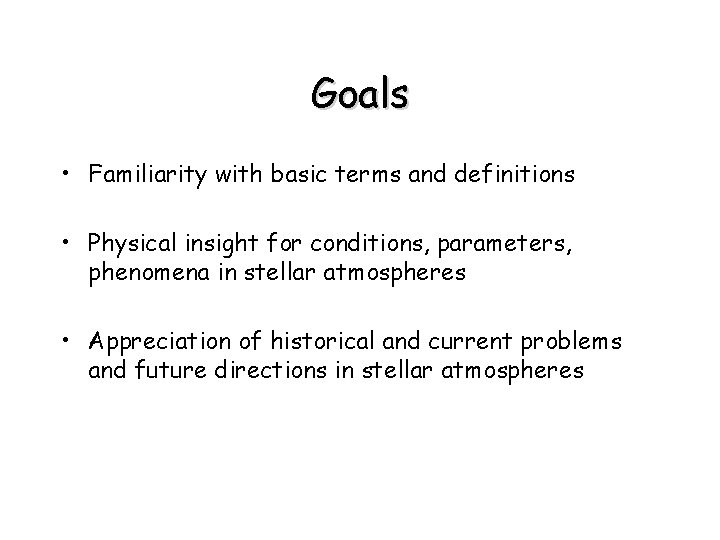
Goals • Familiarity with basic terms and definitions • Physical insight for conditions, parameters, phenomena in stellar atmospheres • Appreciation of historical and current problems and future directions in stellar atmospheres
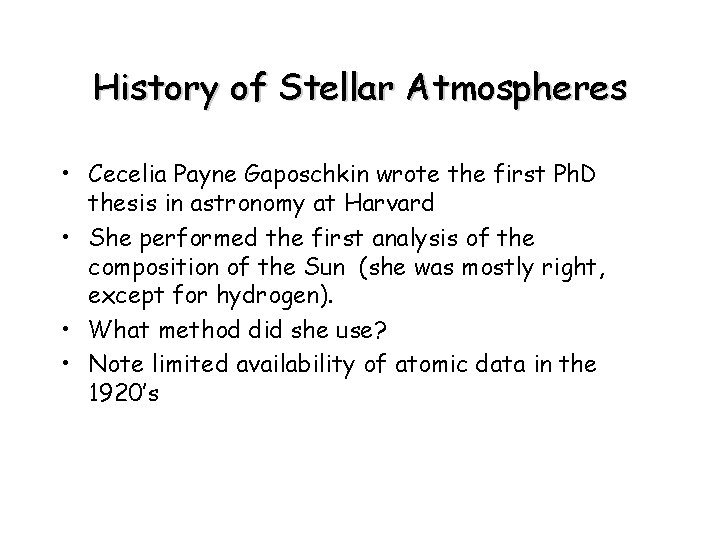
History of Stellar Atmospheres • Cecelia Payne Gaposchkin wrote the first Ph. D thesis in astronomy at Harvard • She performed the first analysis of the composition of the Sun (she was mostly right, except for hydrogen). • What method did she use? • Note limited availability of atomic data in the 1920’s
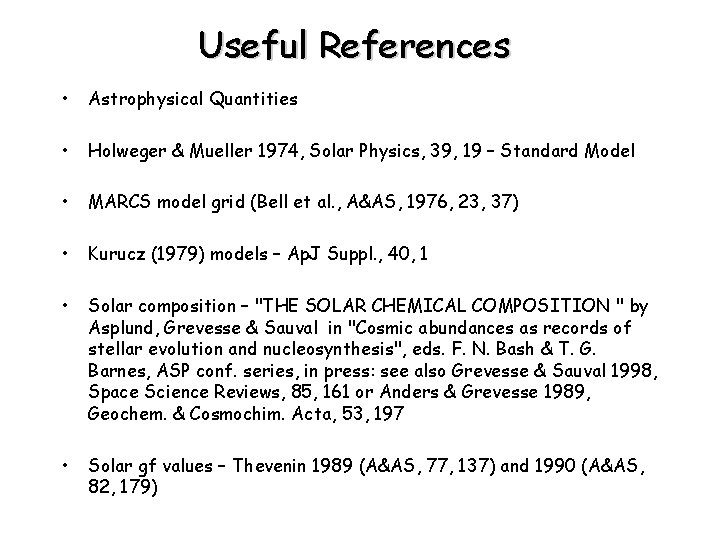
Useful References • Astrophysical Quantities • Holweger & Mueller 1974, Solar Physics, 39, 19 – Standard Model • MARCS model grid (Bell et al. , A&AS, 1976, 23, 37) • Kurucz (1979) models – Ap. J Suppl. , 40, 1 • Solar composition – "THE SOLAR CHEMICAL COMPOSITION " by Asplund, Grevesse & Sauval in "Cosmic abundances as records of stellar evolution and nucleosynthesis", eds. F. N. Bash & T. G. Barnes, ASP conf. series, in press: see also Grevesse & Sauval 1998, Space Science Reviews, 85, 161 or Anders & Grevesse 1989, Geochem. & Cosmochim. Acta, 53, 197 • Solar gf values – Thevenin 1989 (A&AS, 77, 137) and 1990 (A&AS, 82, 179)
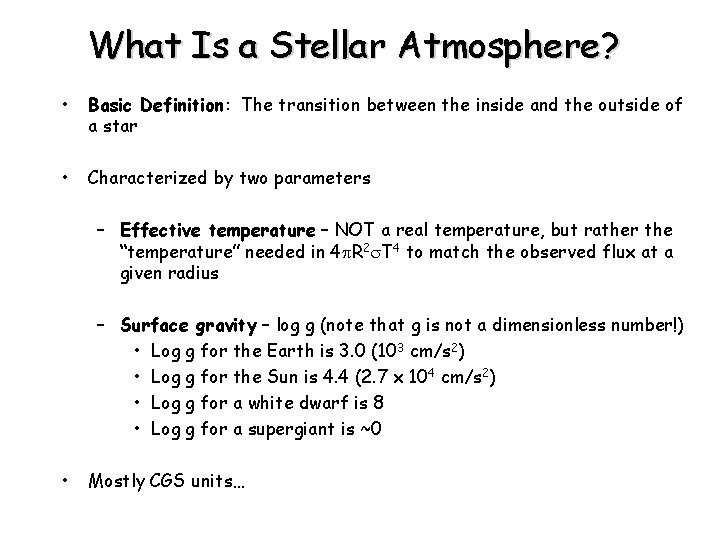
What Is a Stellar Atmosphere? • Basic Definition: The transition between the inside and the outside of a star • Characterized by two parameters – Effective temperature – NOT a real temperature, but rather the “temperature” needed in 4 p. R 2 s. T 4 to match the observed flux at a given radius – Surface gravity – log g (note that g is not a dimensionless number!) • Log g for the Earth is 3. 0 (103 cm/s 2) • Log g for the Sun is 4. 4 (2. 7 x 104 cm/s 2) • Log g for a white dwarf is 8 • Log g for a supergiant is ~0 • Mostly CGS units…
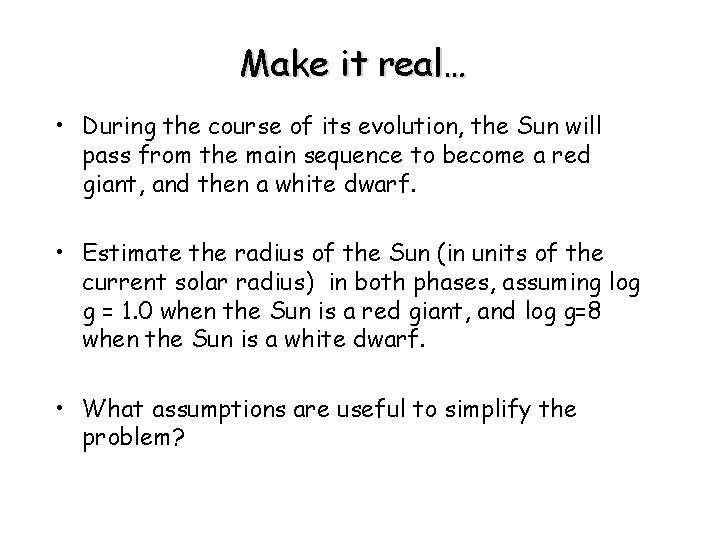
Make it real… • During the course of its evolution, the Sun will pass from the main sequence to become a red giant, and then a white dwarf. • Estimate the radius of the Sun (in units of the current solar radius) in both phases, assuming log g = 1. 0 when the Sun is a red giant, and log g=8 when the Sun is a white dwarf. • What assumptions are useful to simplify the problem?
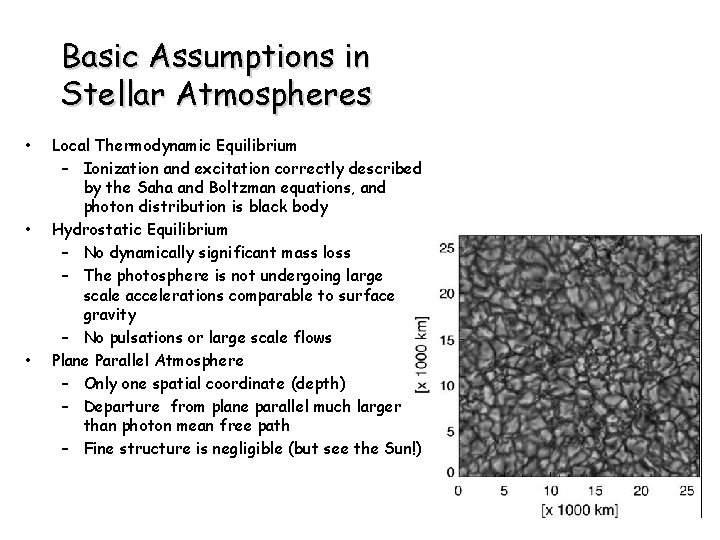
Basic Assumptions in Stellar Atmospheres • • • Local Thermodynamic Equilibrium – Ionization and excitation correctly described by the Saha and Boltzman equations, and photon distribution is black body Hydrostatic Equilibrium – No dynamically significant mass loss – The photosphere is not undergoing large scale accelerations comparable to surface gravity – No pulsations or large scale flows Plane Parallel Atmosphere – Only one spatial coordinate (depth) – Departure from plane parallel much larger than photon mean free path – Fine structure is negligible (but see the Sun!)
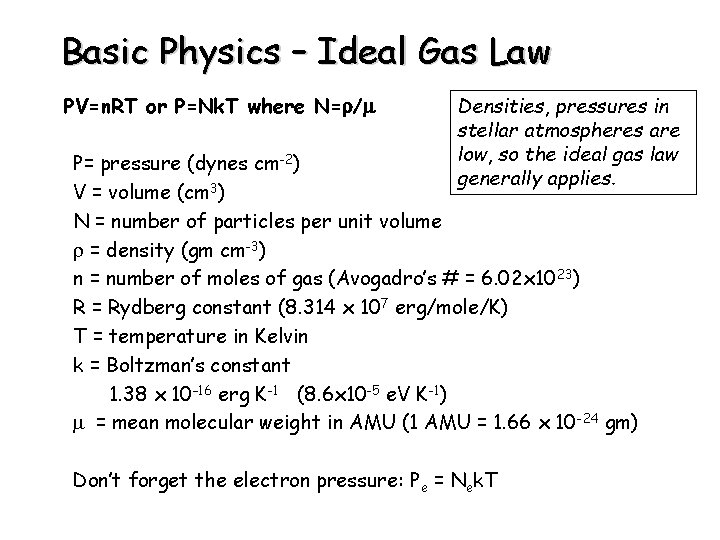
Basic Physics – Ideal Gas Law PV=n. RT or P=Nk. T where N=r/m Densities, pressures in stellar atmospheres are low, so the ideal gas law generally applies. P= pressure (dynes cm-2) V = volume (cm 3) N = number of particles per unit volume r = density (gm cm-3) n = number of moles of gas (Avogadro’s # = 6. 02 x 1023) R = Rydberg constant (8. 314 x 107 erg/mole/K) T = temperature in Kelvin k = Boltzman’s constant 1. 38 x 10– 16 erg K-1 (8. 6 x 10 -5 e. V K-1) m = mean molecular weight in AMU (1 AMU = 1. 66 x 10 -24 gm) Don’t forget the electron pressure: Pe = Nek. T
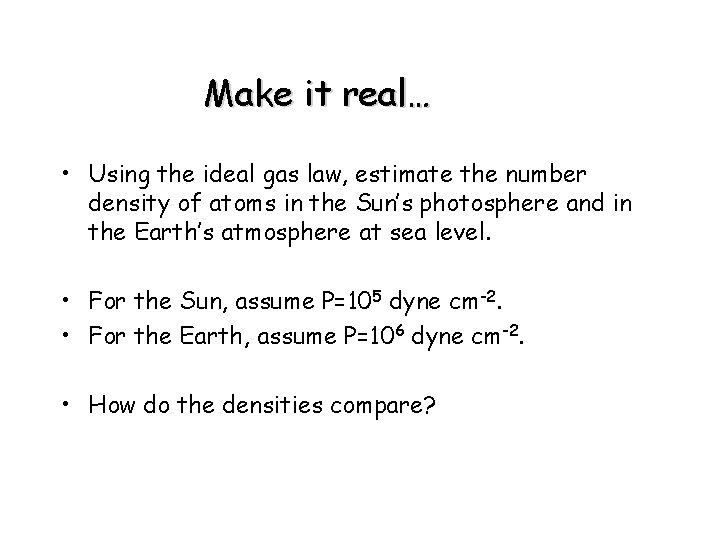
Make it real… • Using the ideal gas law, estimate the number density of atoms in the Sun’s photosphere and in the Earth’s atmosphere at sea level. • For the Sun, assume P=105 dyne cm-2. • For the Earth, assume P=106 dyne cm-2. • How do the densities compare?
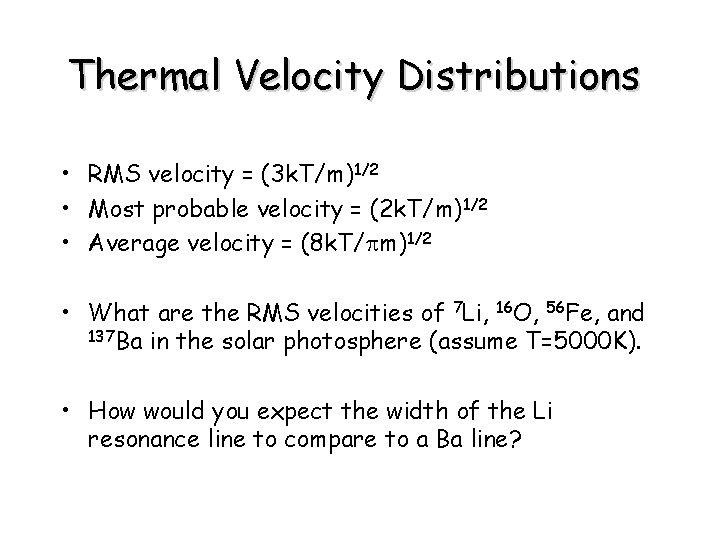
Thermal Velocity Distributions • RMS velocity = (3 k. T/m)1/2 • Most probable velocity = (2 k. T/m)1/2 • Average velocity = (8 k. T/pm)1/2 • What are the RMS velocities of 7 Li, 16 O, 56 Fe, and 137 Ba in the solar photosphere (assume T=5000 K). • How would you expect the width of the Li resonance line to compare to a Ba line?
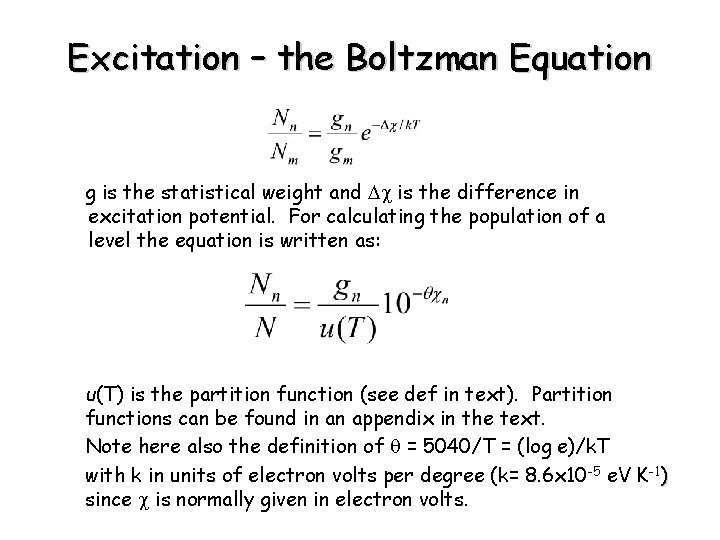
Excitation – the Boltzman Equation g is the statistical weight and Dc is the difference in excitation potential. For calculating the population of a level the equation is written as: u(T) is the partition function (see def in text). Partition functions can be found in an appendix in the text. Note here also the definition of q = 5040/T = (log e)/k. T with k in units of electron volts per degree (k= 8. 6 x 10 -5 e. V K-1) since c is normally given in electron volts.
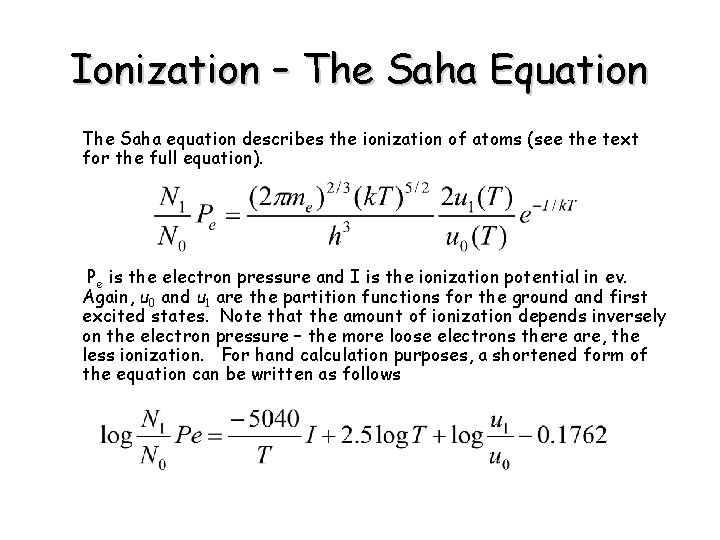
Ionization – The Saha Equation The Saha equation describes the ionization of atoms (see the text for the full equation). Pe is the electron pressure and I is the ionization potential in ev. Again, u 0 and u 1 are the partition functions for the ground and first excited states. Note that the amount of ionization depends inversely on the electron pressure – the more loose electrons there are, the less ionization. For hand calculation purposes, a shortened form of the equation can be written as follows
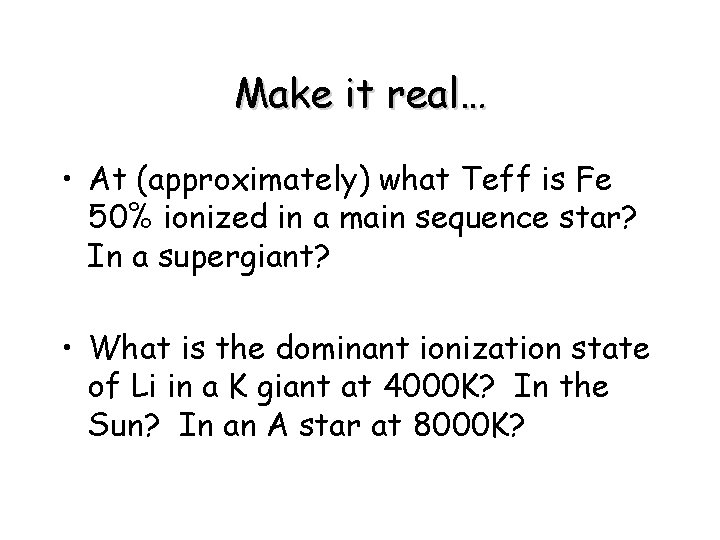
Make it real… • At (approximately) what Teff is Fe 50% ionized in a main sequence star? In a supergiant? • What is the dominant ionization state of Li in a K giant at 4000 K? In the Sun? In an A star at 8000 K?
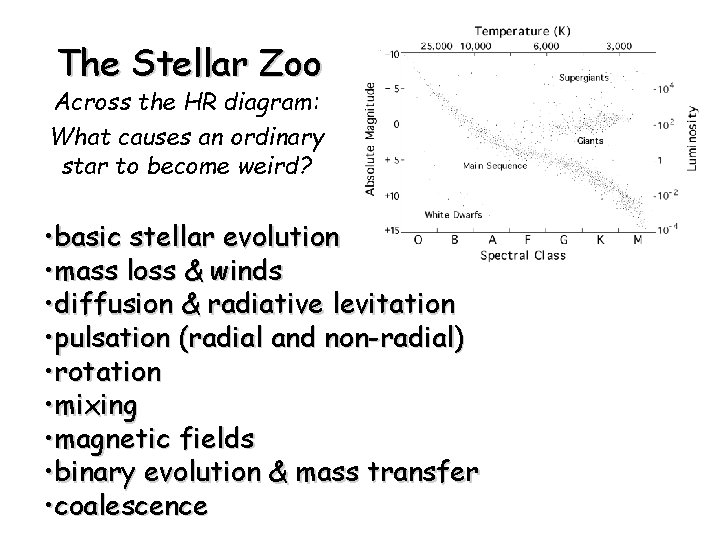
The Stellar Zoo Across the HR diagram: What causes an ordinary star to become weird? • basic stellar evolution • mass loss & winds • diffusion & radiative levitation • pulsation (radial and non-radial) • rotation • mixing • magnetic fields • binary evolution & mass transfer • coalescence
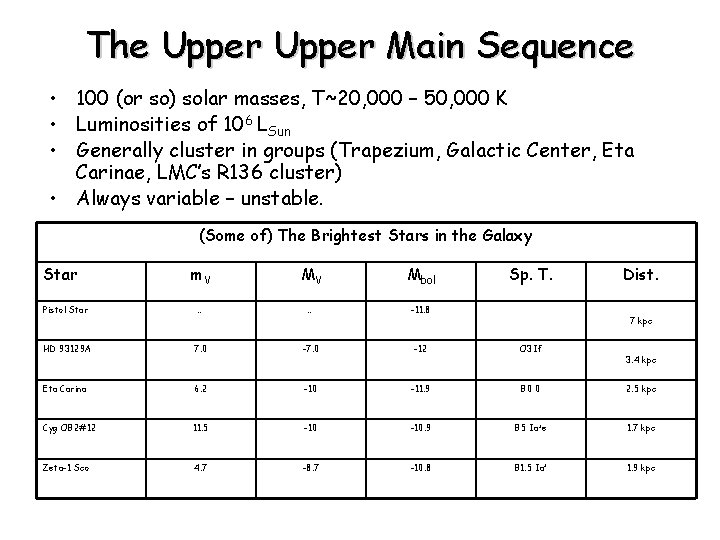
The Upper Main Sequence • 100 (or so) solar masses, T~20, 000 – 50, 000 K • Luminosities of 106 LSun • Generally cluster in groups (Trapezium, Galactic Center, Eta Carinae, LMC’s R 136 cluster) • Always variable – unstable. (Some of) The Brightest Stars in the Galaxy Star m. V MV Mbol Sp. T. Dist. Pistol Star … … -11. 8 HD 93129 A 7. 0 -12 O 3 If Eta Carina 6. 2 -10 -11. 9 B 0 0 2. 5 kpc Cyg OB 2#12 11. 5 -10. 9 B 5 Ia+e 1. 7 kpc Zeta-1 Sco 4. 7 -8. 7 -10. 8 B 1. 5 Ia+ 1. 9 kpc 7 kpc 3. 4 kpc
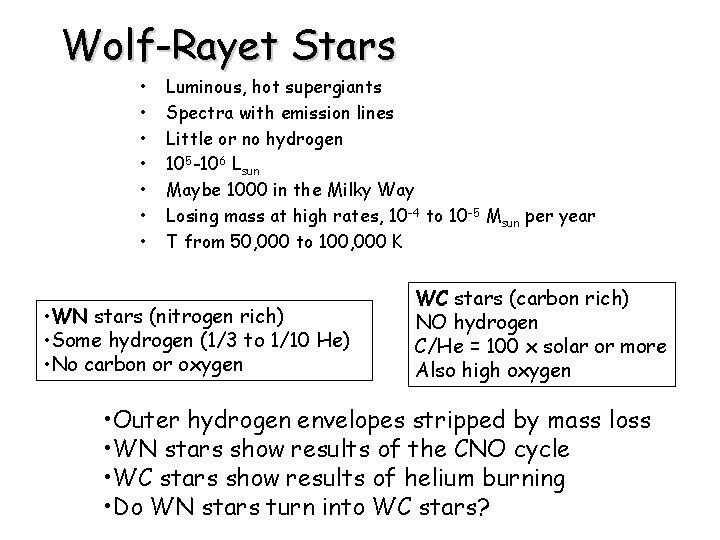
Wolf-Rayet Stars • • Luminous, hot supergiants Spectra with emission lines Little or no hydrogen 105 -106 Lsun Maybe 1000 in the Milky Way Losing mass at high rates, 10 -4 to 10 -5 Msun per year T from 50, 000 to 100, 000 K • WN stars (nitrogen rich) • Some hydrogen (1/3 to 1/10 He) • No carbon or oxygen WC stars (carbon rich) NO hydrogen C/He = 100 x solar or more Also high oxygen • Outer hydrogen envelopes stripped by mass loss • WN stars show results of the CNO cycle • WC stars show results of helium burning • Do WN stars turn into WC stars?
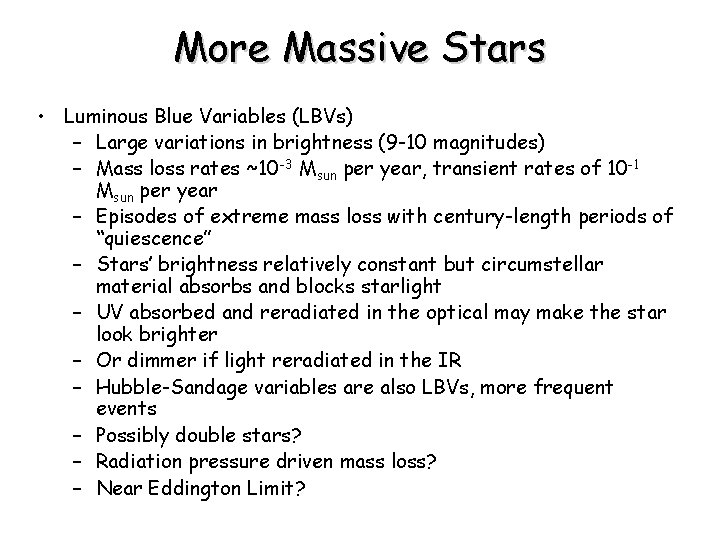
More Massive Stars • Luminous Blue Variables (LBVs) – Large variations in brightness (9 -10 magnitudes) – Mass loss rates ~10 -3 Msun per year, transient rates of 10 -1 Msun per year – Episodes of extreme mass loss with century-length periods of “quiescence” – Stars’ brightness relatively constant but circumstellar material absorbs and blocks starlight – UV absorbed and reradiated in the optical may make the star look brighter – Or dimmer if light reradiated in the IR – Hubble-Sandage variables are also LBVs, more frequent events – Possibly double stars? – Radiation pressure driven mass loss? – Near Eddington Limit?
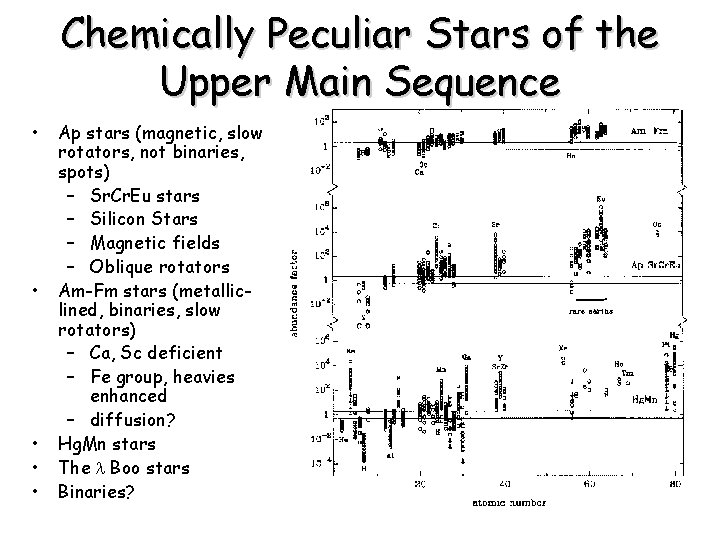
Chemically Peculiar Stars of the Upper Main Sequence • • • Ap stars (magnetic, slow rotators, not binaries, spots) – Sr. Cr. Eu stars – Silicon Stars – Magnetic fields – Oblique rotators Am-Fm stars (metalliclined, binaries, slow rotators) – Ca, Sc deficient – Fe group, heavies enhanced – diffusion? Hg. Mn stars The l Boo stars Binaries?
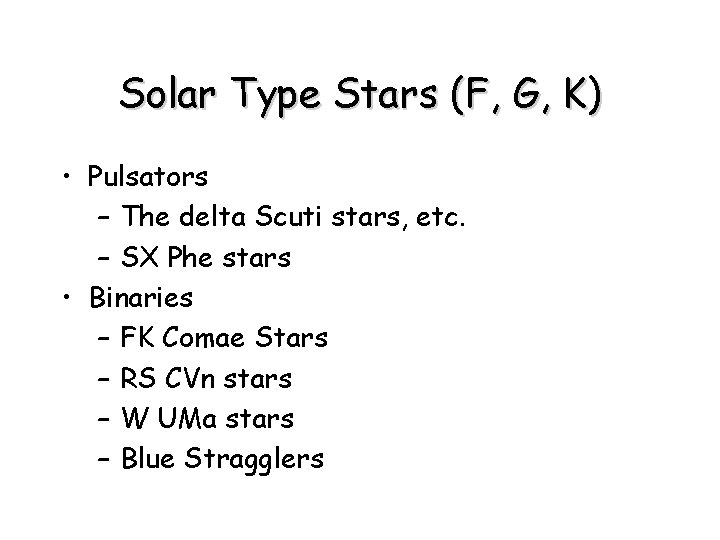
Solar Type Stars (F, G, K) • Pulsators – The delta Scuti stars, etc. – SX Phe stars • Binaries – FK Comae Stars – RS CVn stars – W UMa stars – Blue Stragglers
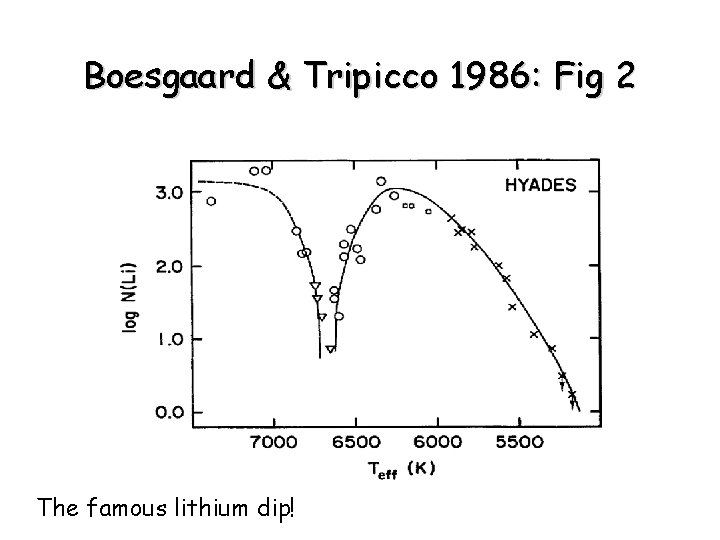
Boesgaard & Tripicco 1986: Fig 2 The famous lithium dip!
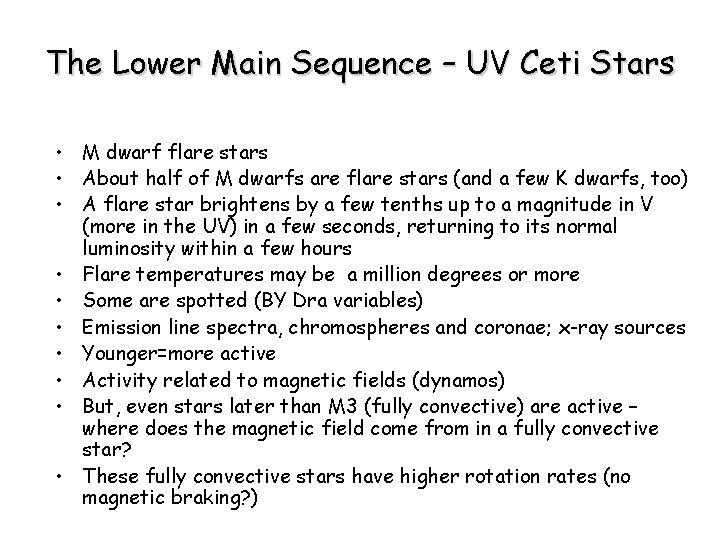
The Lower Main Sequence – UV Ceti Stars • M dwarf flare stars • About half of M dwarfs are flare stars (and a few K dwarfs, too) • A flare star brightens by a few tenths up to a magnitude in V (more in the UV) in a few seconds, returning to its normal luminosity within a few hours • Flare temperatures may be a million degrees or more • Some are spotted (BY Dra variables) • Emission line spectra, chromospheres and coronae; x-ray sources • Younger=more active • Activity related to magnetic fields (dynamos) • But, even stars later than M 3 (fully convective) are active – where does the magnetic field come from in a fully convective star? • These fully convective stars have higher rotation rates (no magnetic braking? )
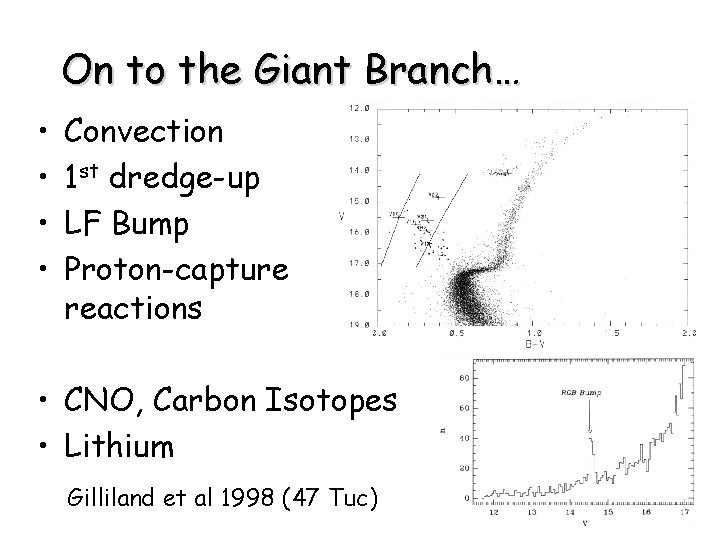
On to the Giant Branch… • • Convection 1 st dredge-up LF Bump Proton-capture reactions • CNO, Carbon Isotopes • Lithium Gilliland et al 1998 (47 Tuc)
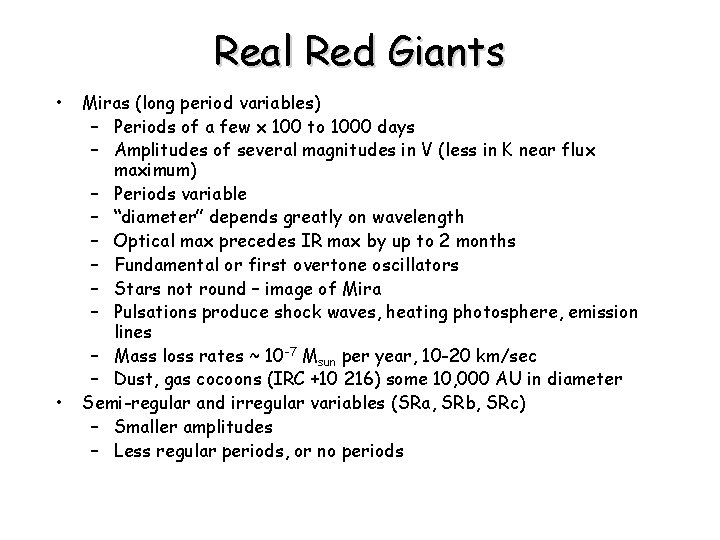
Real Red Giants • • Miras (long period variables) – Periods of a few x 100 to 1000 days – Amplitudes of several magnitudes in V (less in K near flux maximum) – Periods variable – “diameter” depends greatly on wavelength – Optical max precedes IR max by up to 2 months – Fundamental or first overtone oscillators – Stars not round – image of Mira – Pulsations produce shock waves, heating photosphere, emission lines – Mass loss rates ~ 10 -7 Msun per year, 10 -20 km/sec – Dust, gas cocoons (IRC +10 216) some 10, 000 AU in diameter Semi-regular and irregular variables (SRa, SRb, SRc) – Smaller amplitudes – Less regular periods, or no periods
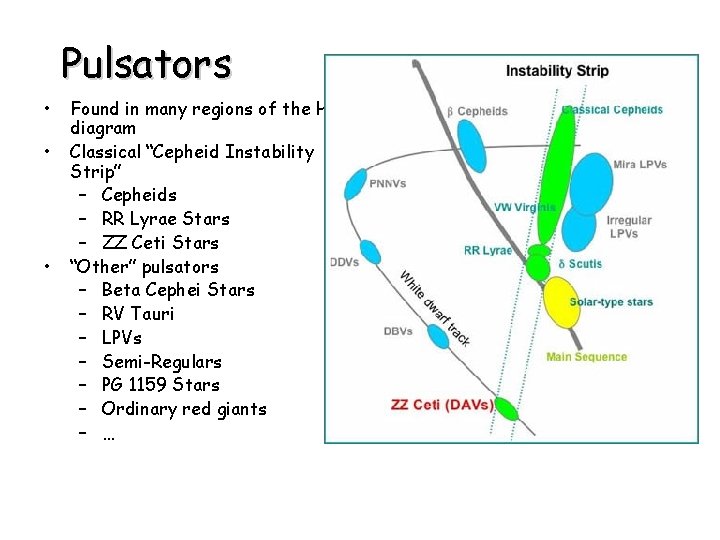
Pulsators • • • Found in many regions of the HR diagram Classical “Cepheid Instability Strip” – Cepheids – RR Lyrae Stars – ZZ Ceti Stars “Other” pulsators – Beta Cephei Stars – RV Tauri – LPVs – Semi-Regulars – PG 1159 Stars – Ordinary red giants – …
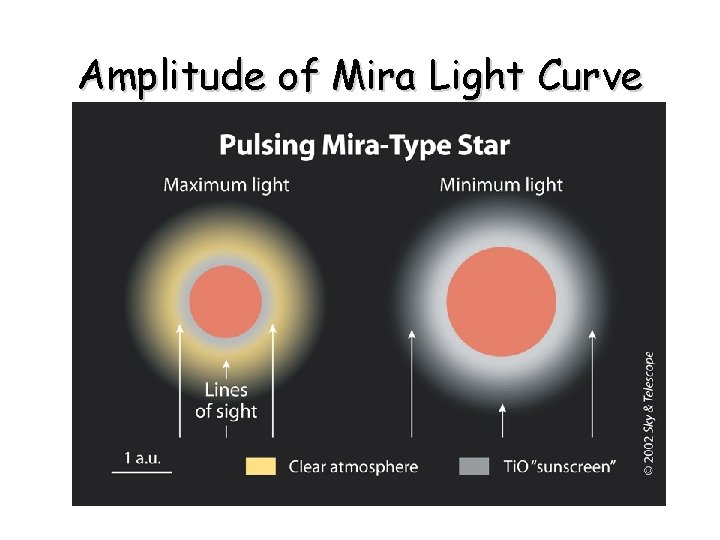
Amplitude of Mira Light Curve
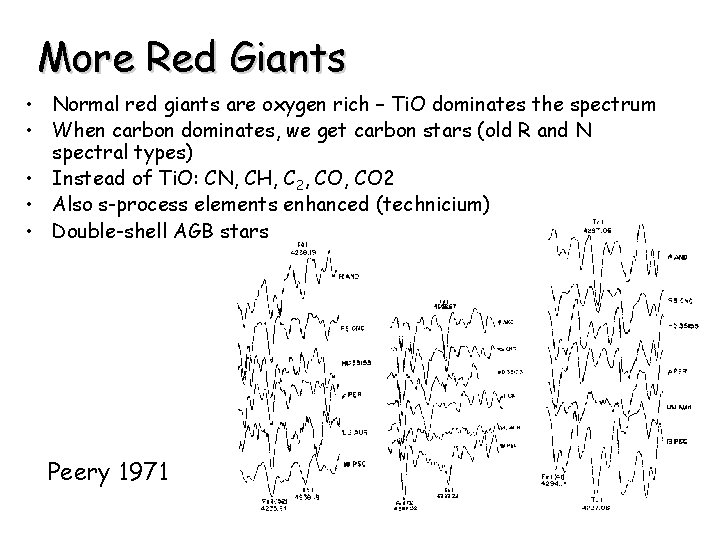
More Red Giants • Normal red giants are oxygen rich – Ti. O dominates the spectrum • When carbon dominates, we get carbon stars (old R and N spectral types) • Instead of Ti. O: CN, CH, C 2, CO 2 • Also s-process elements enhanced (technicium) • Double-shell AGB stars Peery 1971
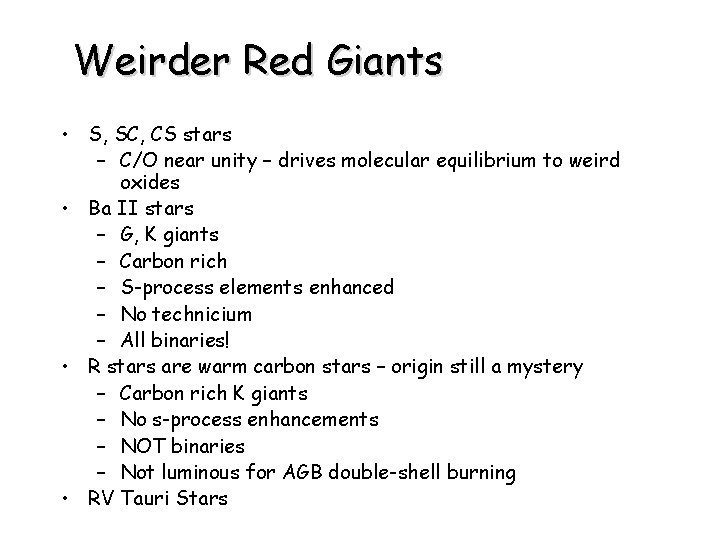
Weirder Red Giants • S, SC, CS stars – C/O near unity – drives molecular equilibrium to weird oxides • Ba II stars – G, K giants – Carbon rich – S-process elements enhanced – No technicium – All binaries! • R stars are warm carbon stars – origin still a mystery – Carbon rich K giants – No s-process enhancements – NOT binaries – Not luminous for AGB double-shell burning • RV Tauri Stars
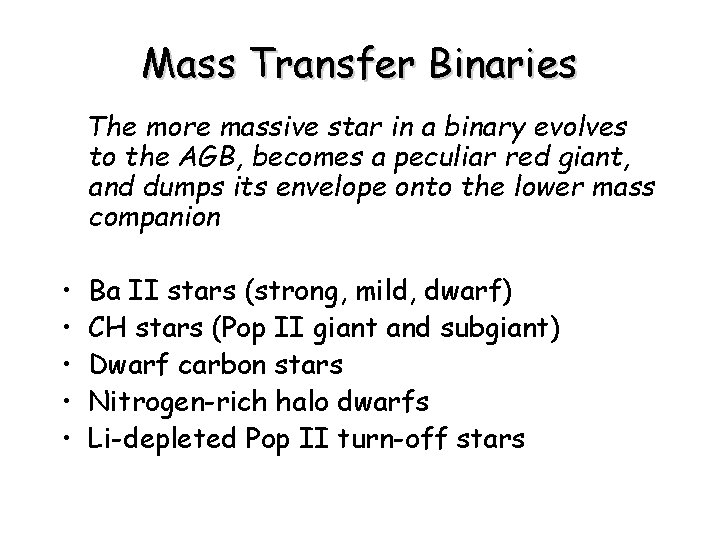
Mass Transfer Binaries The more massive star in a binary evolves to the AGB, becomes a peculiar red giant, and dumps its envelope onto the lower mass companion • • • Ba II stars (strong, mild, dwarf) CH stars (Pop II giant and subgiant) Dwarf carbon stars Nitrogen-rich halo dwarfs Li-depleted Pop II turn-off stars
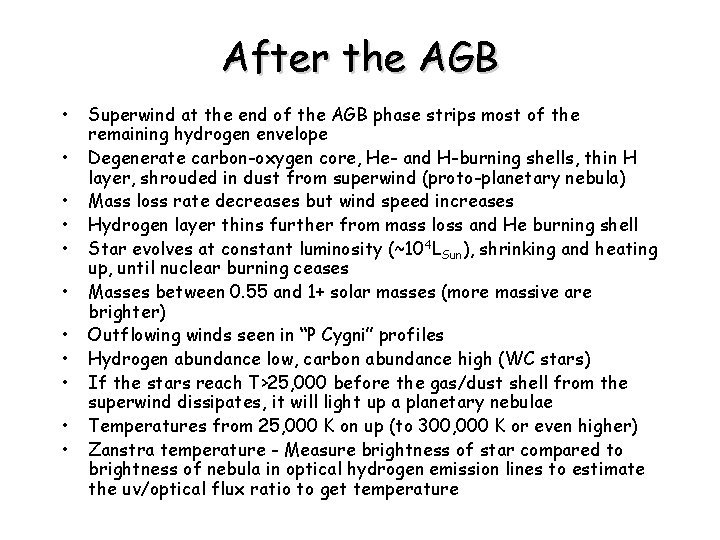
After the AGB • • • Superwind at the end of the AGB phase strips most of the remaining hydrogen envelope Degenerate carbon-oxygen core, He- and H-burning shells, thin H layer, shrouded in dust from superwind (proto-planetary nebula) Mass loss rate decreases but wind speed increases Hydrogen layer thins further from mass loss and He burning shell Star evolves at constant luminosity (~104 LSun), shrinking and heating up, until nuclear burning ceases Masses between 0. 55 and 1+ solar masses (more massive are brighter) Outflowing winds seen in “P Cygni” profiles Hydrogen abundance low, carbon abundance high (WC stars) If the stars reach T>25, 000 before the gas/dust shell from the superwind dissipates, it will light up a planetary nebulae Temperatures from 25, 000 K on up (to 300, 000 K or even higher) Zanstra temperature - Measure brightness of star compared to brightness of nebula in optical hydrogen emission lines to estimate the uv/optical flux ratio to get temperature
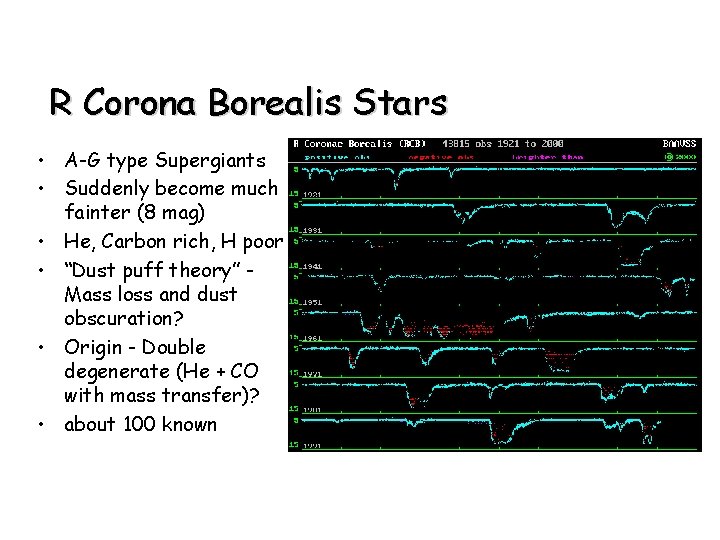
R Corona Borealis Stars • A-G type Supergiants • Suddenly become much fainter (8 mag) • He, Carbon rich, H poor • “Dust puff theory” Mass loss and dust obscuration? • Origin - Double degenerate (He + CO with mass transfer)? • about 100 known
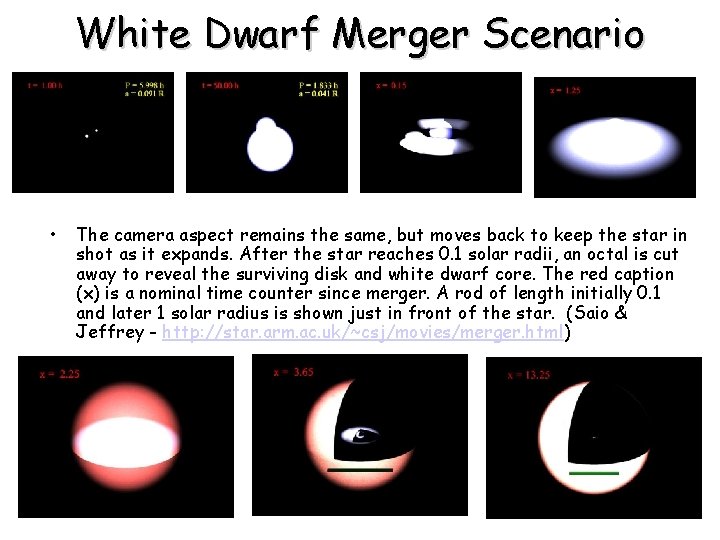
White Dwarf Merger Scenario • The camera aspect remains the same, but moves back to keep the star in shot as it expands. After the star reaches 0. 1 solar radii, an octal is cut away to reveal the surviving disk and white dwarf core. The red caption (x) is a nominal time counter since merger. A rod of length initially 0. 1 and later 1 solar radius is shown just in front of the star. (Saio & Jeffrey - http: //star. arm. ac. uk/~csj/movies/merger. html)
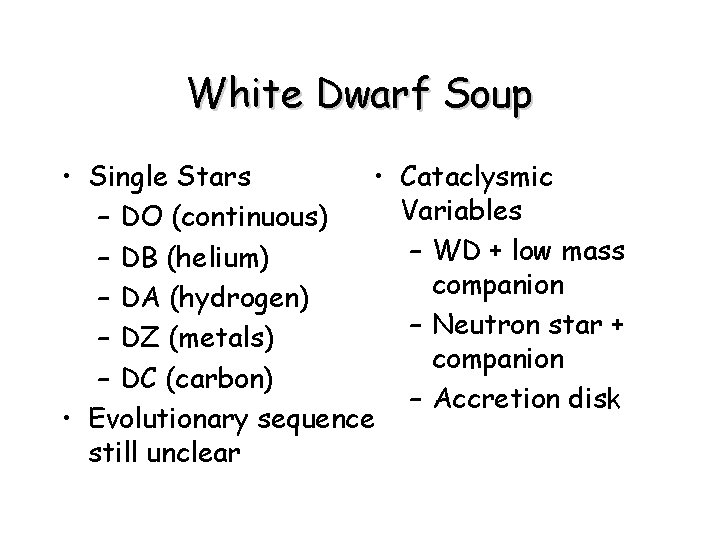
White Dwarf Soup • Single Stars • – DO (continuous) – DB (helium) – DA (hydrogen) – DZ (metals) – DC (carbon) • Evolutionary sequence still unclear Cataclysmic Variables – WD + low mass companion – Neutron star + companion – Accretion disk
Mat0022
Signal word
Minor details examples
Emploiqubec
Chemistry density problems
Cpcu 540 formulas
Au-c 540
Cis 540
12 fam 540
Kate kate 540
Cas200
Isa 540
Sebuah tape recorder dibeli dengan harga rp300.000
Segi n beraturan
Dmca square root
Norma 540
Ano ang masasabi mo sa daigdig bilang planeta
Seorang pedagang membeli 3 kodi pakaian dengan harga
Colorea los múltiplos del número del círculo
Unclear standards in performance appraisal
Jika sq = 48 derajat maka besar prq adalah
Cis 540
Types of meeting
Today meeting or today's meeting
Proposal kickoff meeting agenda
What is meeting and types of meeting
Stellar evolution diagram
Stellar evolution
Stellar assessment
Stellar
Stellar graveyard
Stellar evolution diagram
Stellar wifi
Stellar nucleosynthesis