7 Fluorescence microscopy 7 3 FRET microscopy The
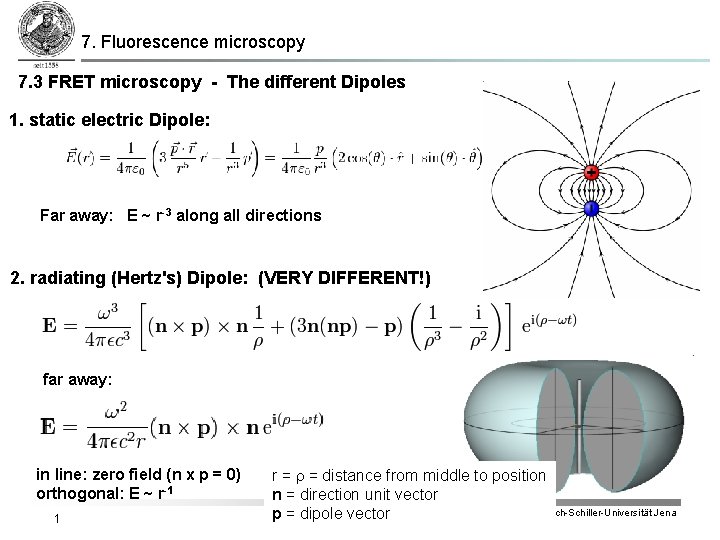
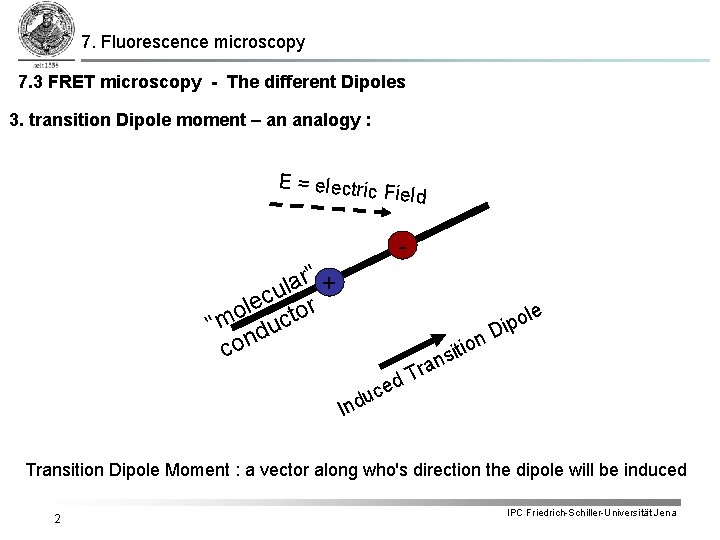
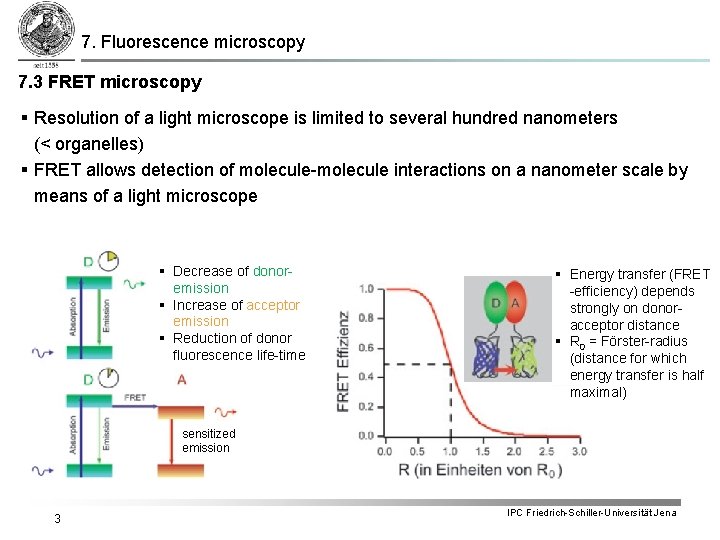
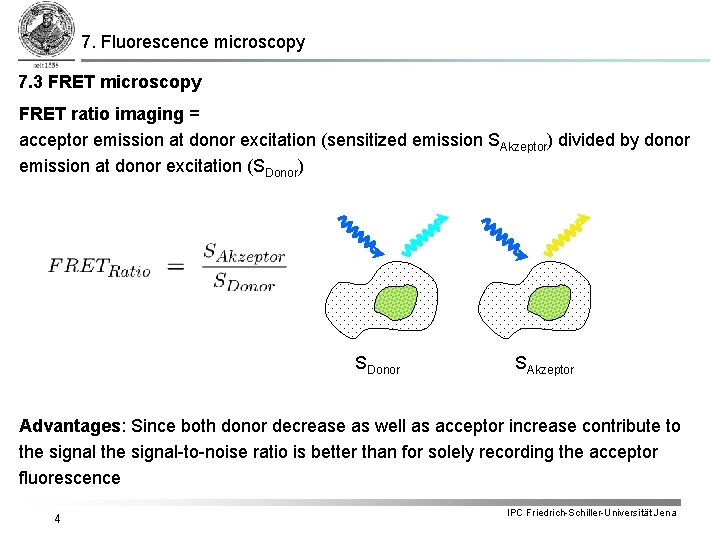
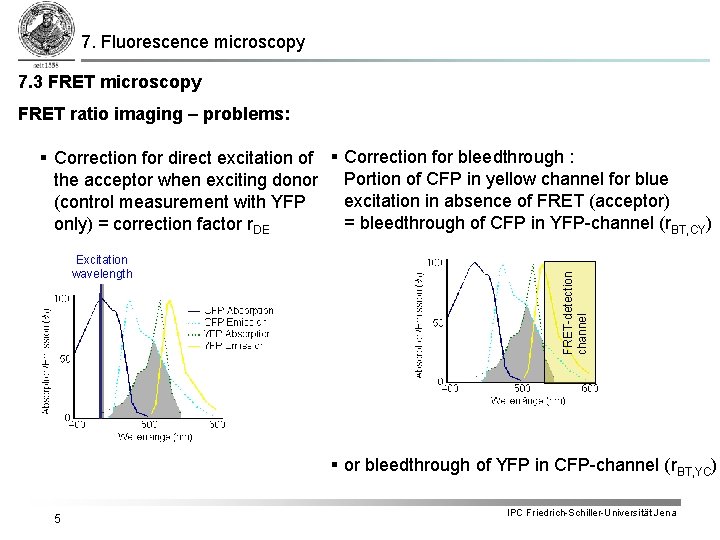
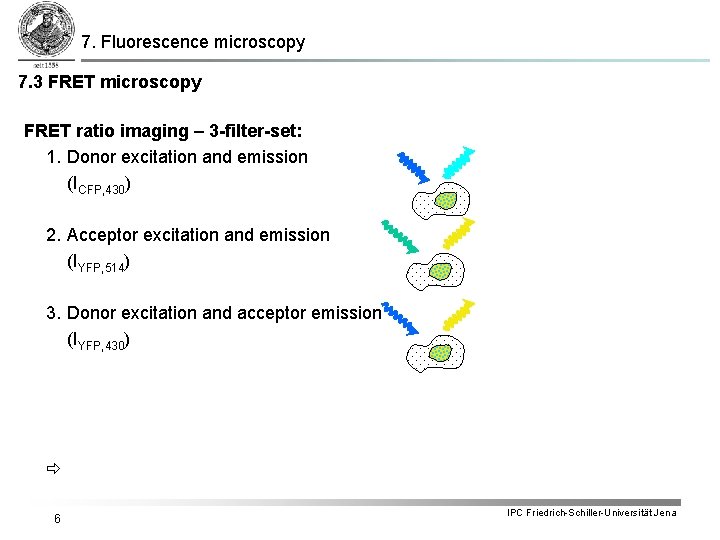
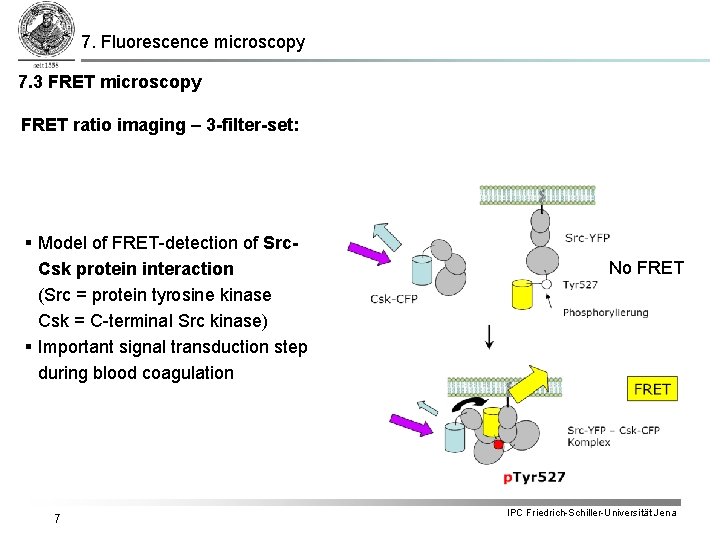
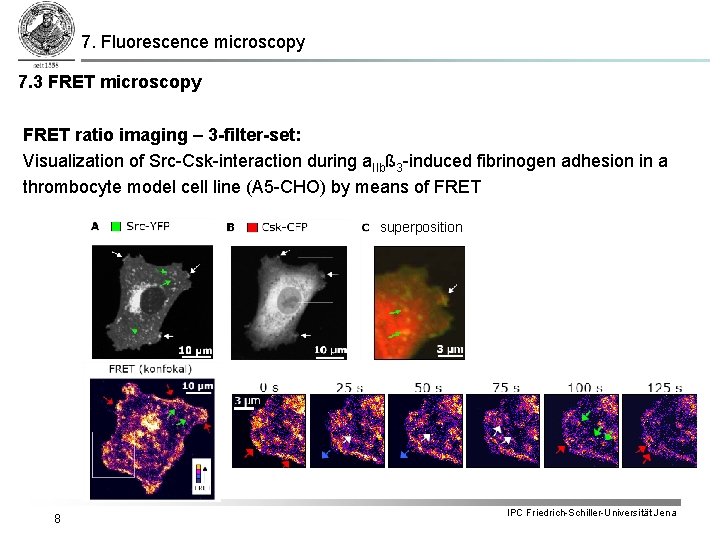
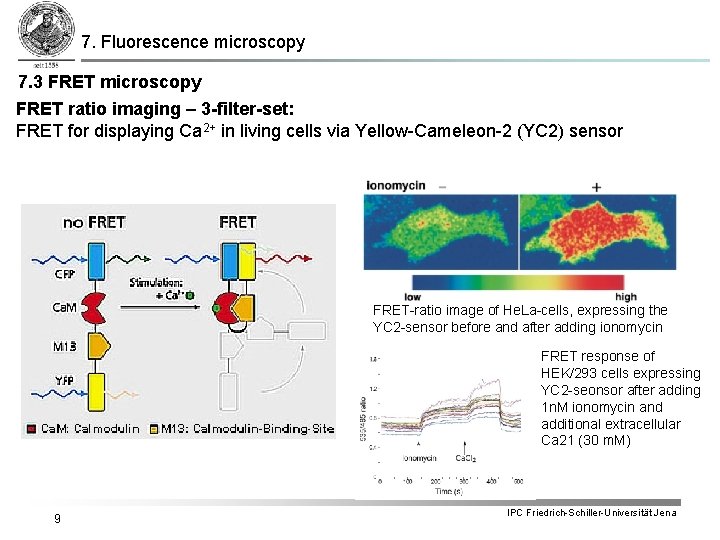
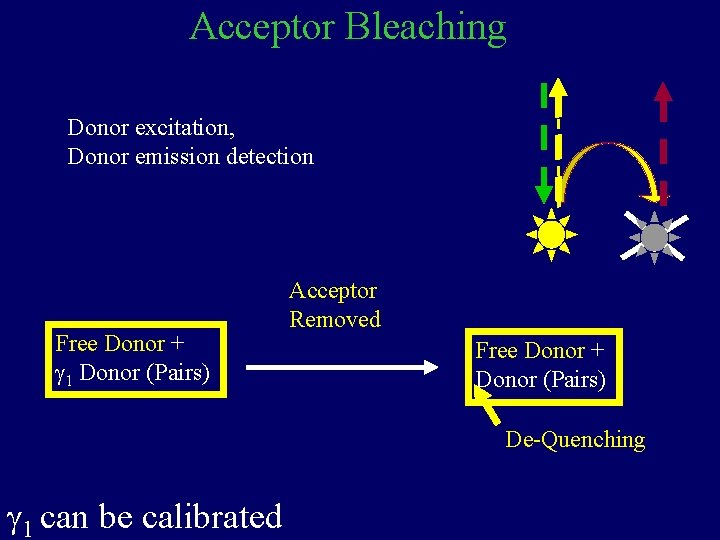
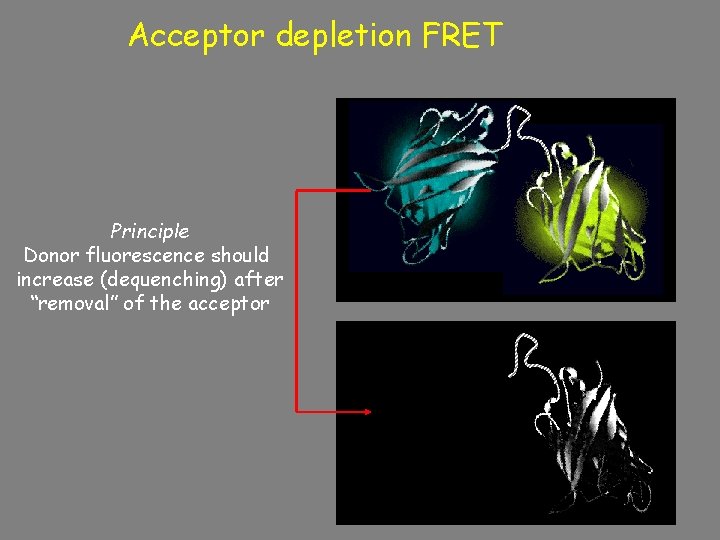
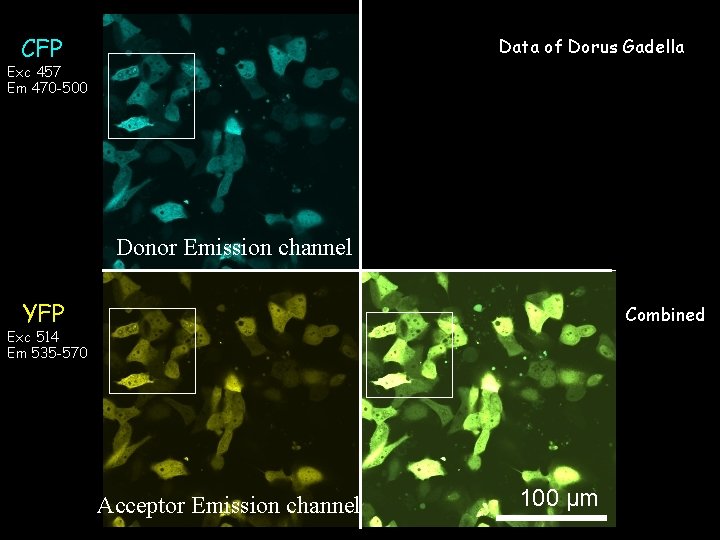
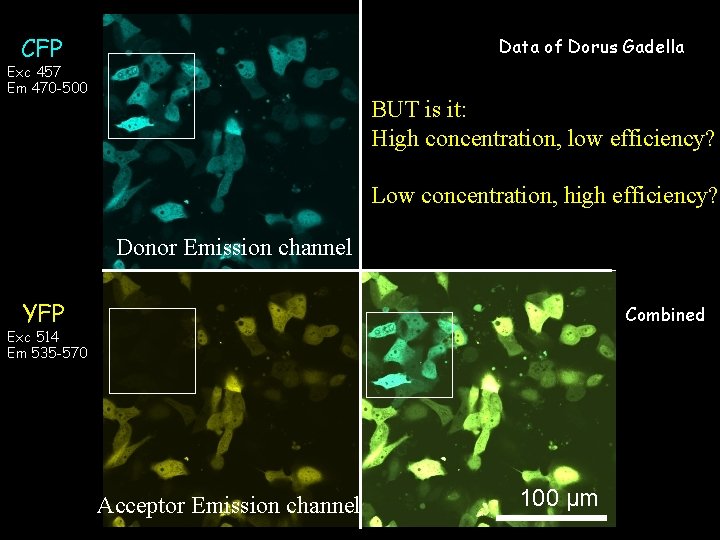
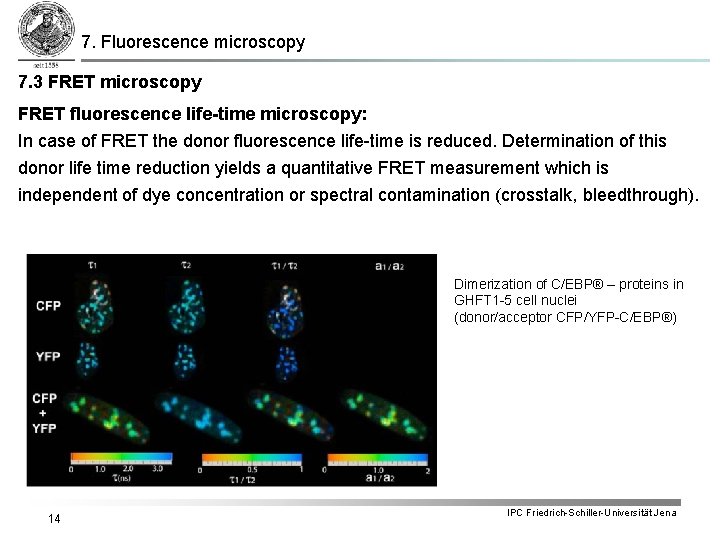
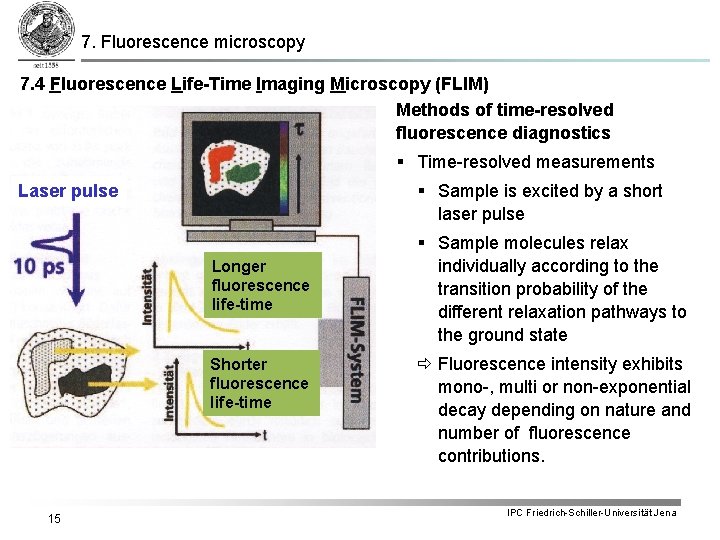
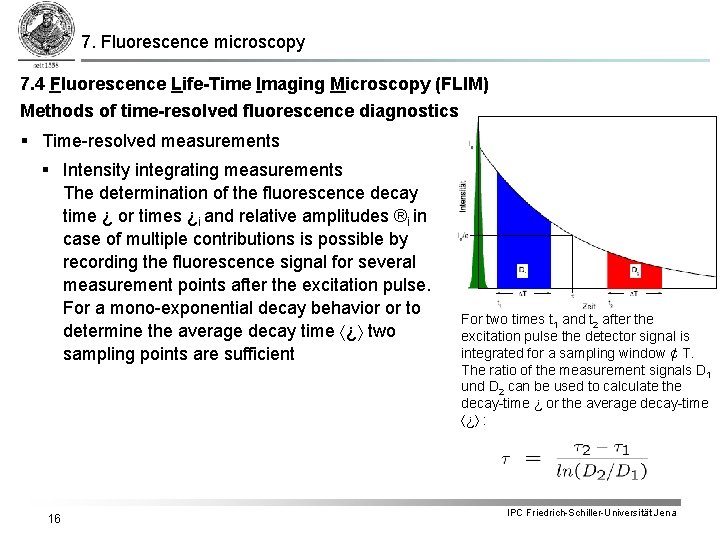
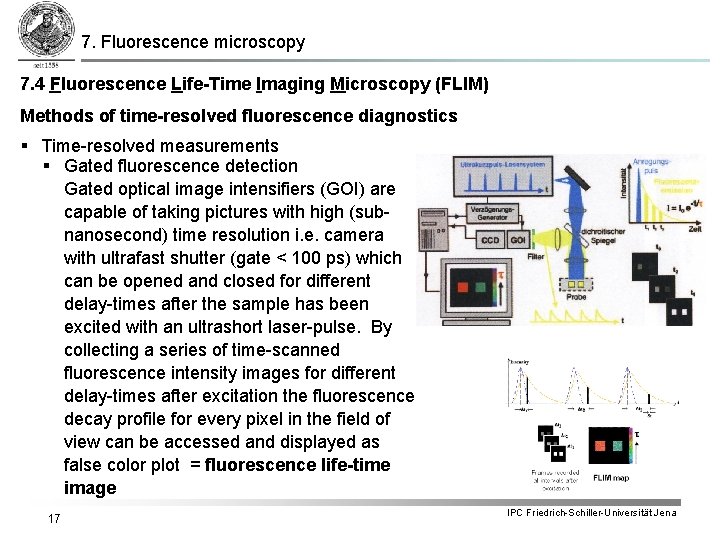
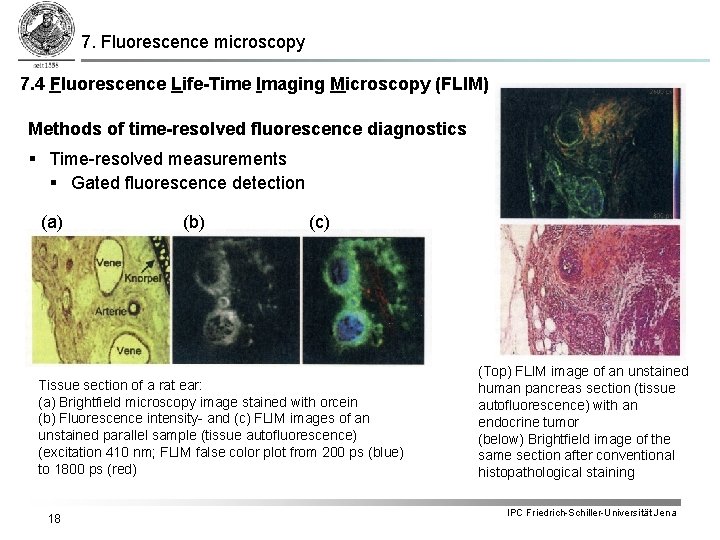
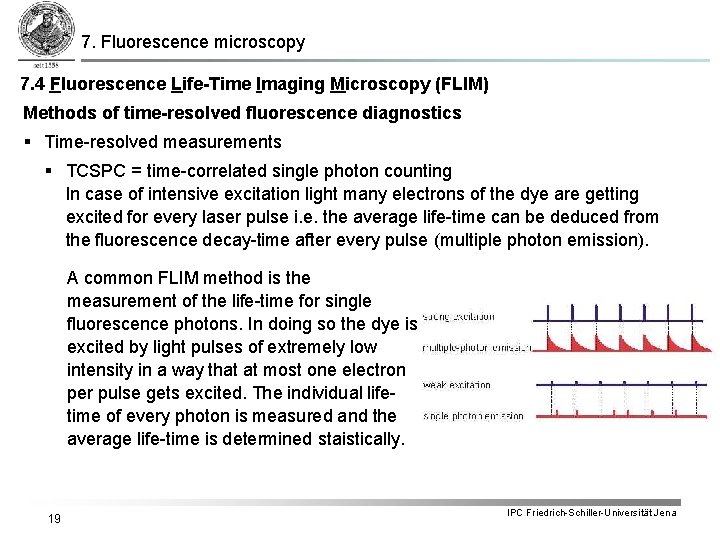
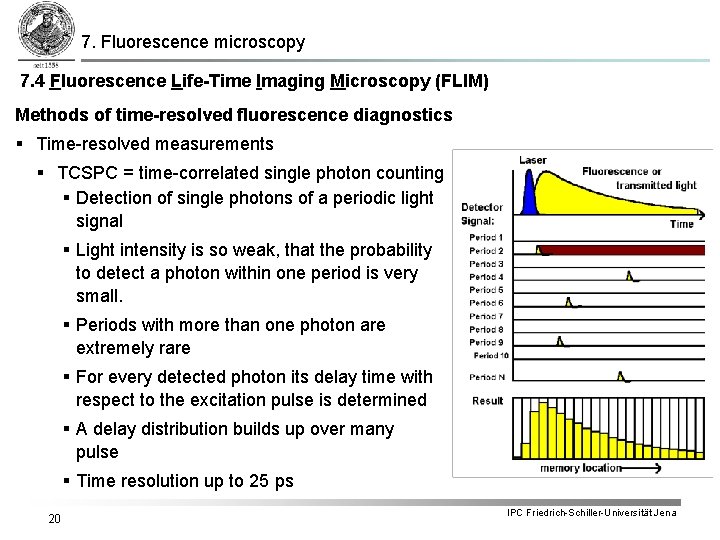
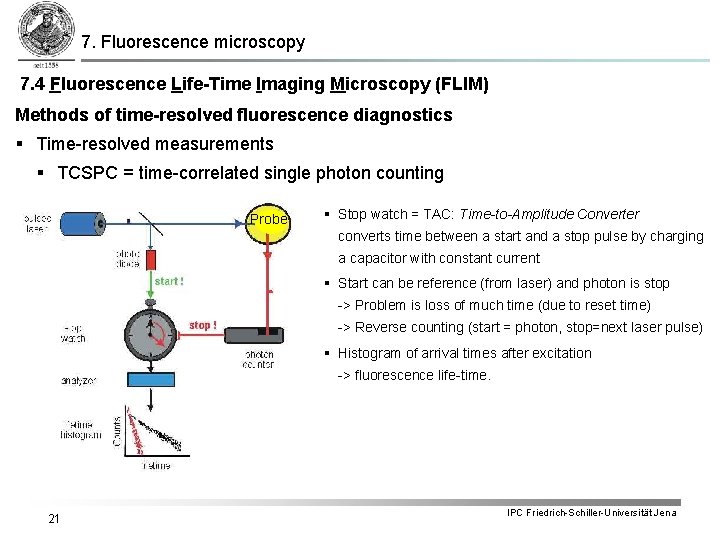
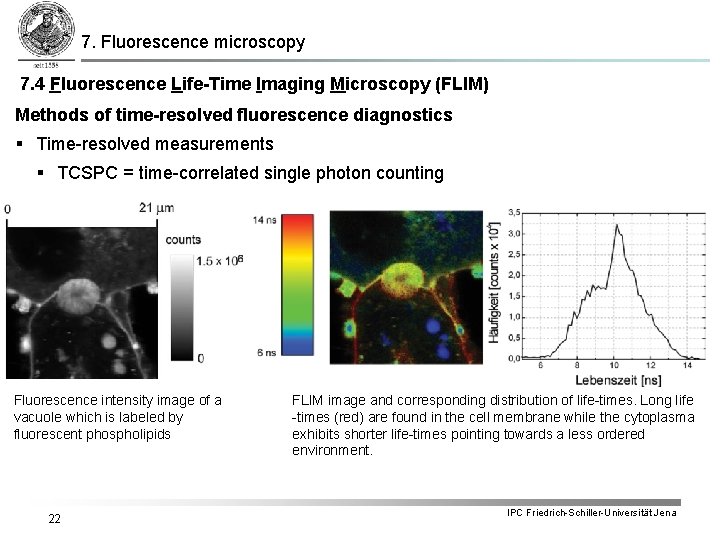
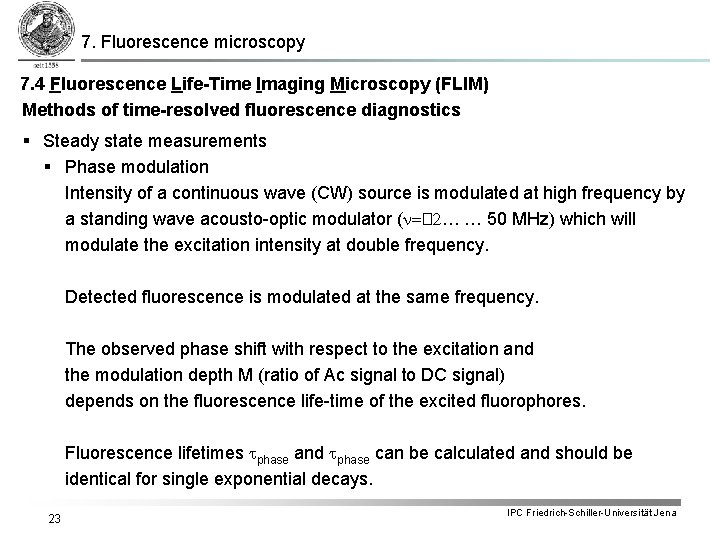
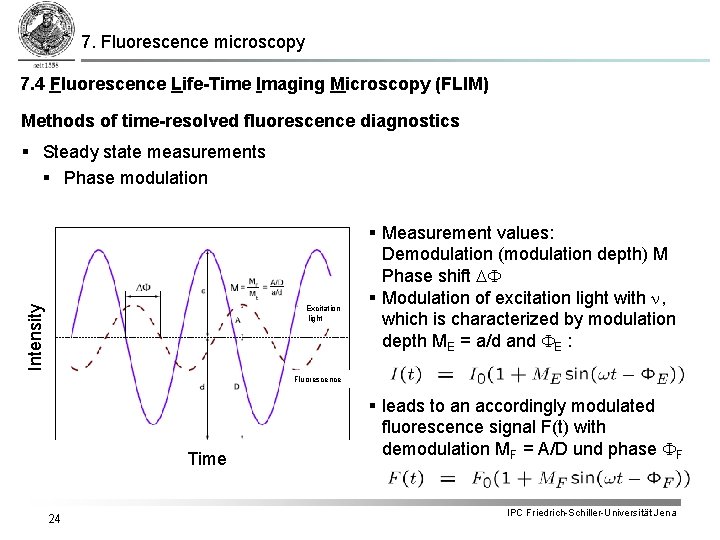
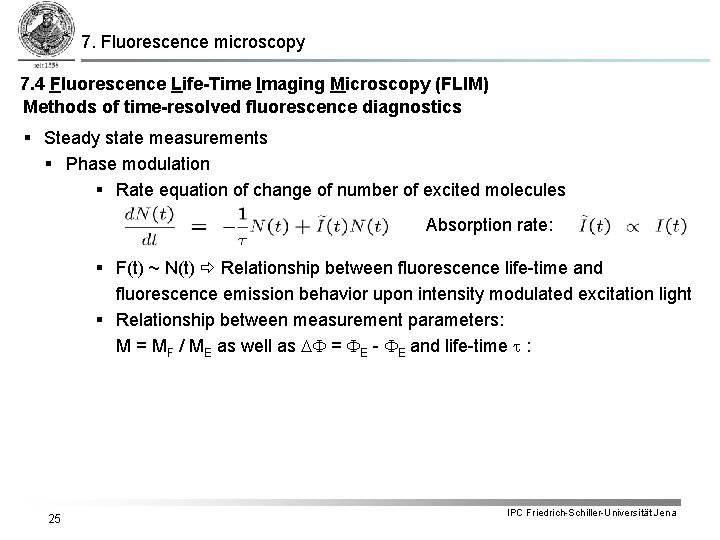
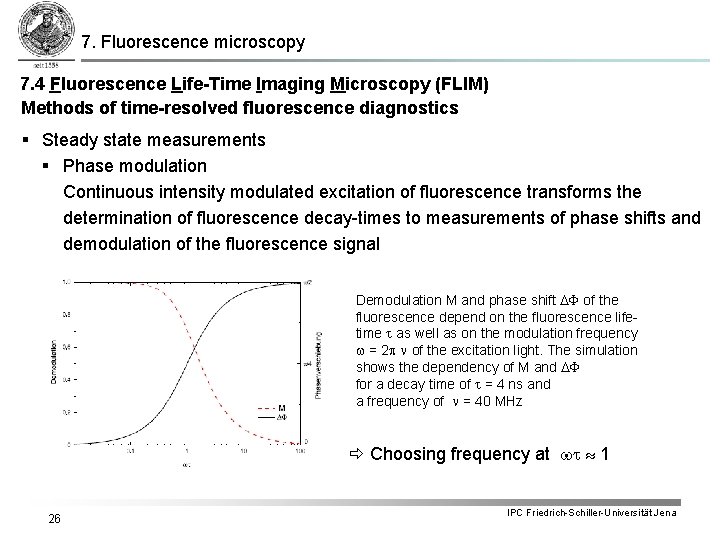
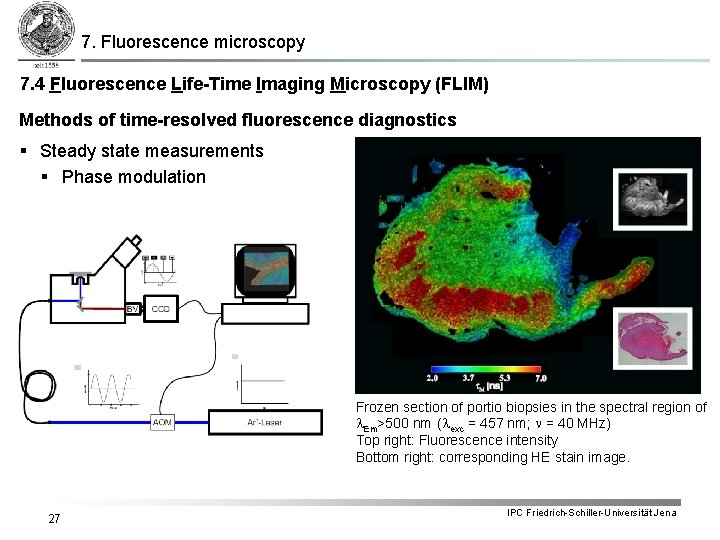
- Slides: 27
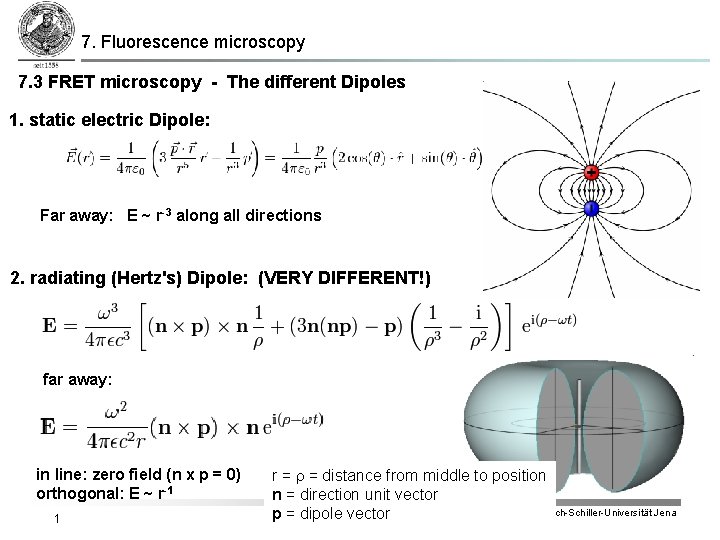
7. Fluorescence microscopy 7. 3 FRET microscopy - The different Dipoles 1. static electric Dipole: Far away: E ~ r-3 along all directions 2. radiating (Hertz's) Dipole: (VERY DIFFERENT!) far away: in line: zero field (n x p = 0) orthogonal: E ~ r-1 1 r = distance from middle to position n = direction unit vector IPC Friedrich-Schiller-Universität Jena p = dipole vector
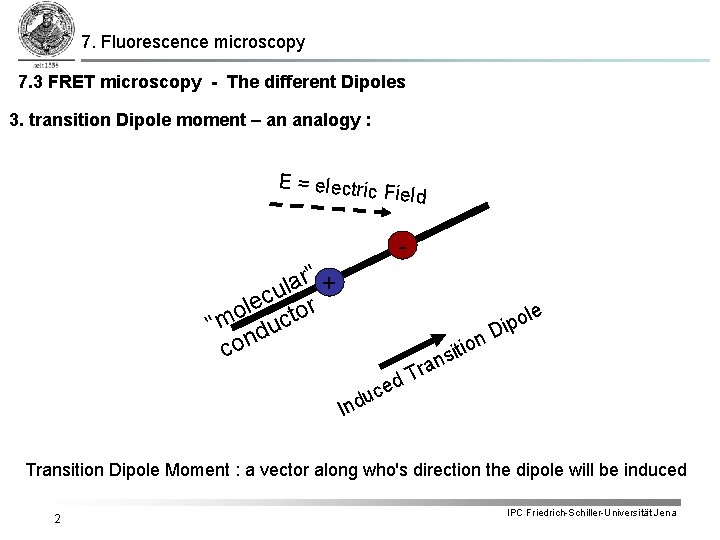
7. Fluorescence microscopy 7. 3 FRET microscopy - The different Dipoles 3. transition Dipole moment – an analogy : E = electric Field - r" + a l u c e l r o o t "m duc con o Dip n o i sit le an r T d ce u Ind Transition Dipole Moment : a vector along who's direction the dipole will be induced 2 IPC Friedrich-Schiller-Universität Jena
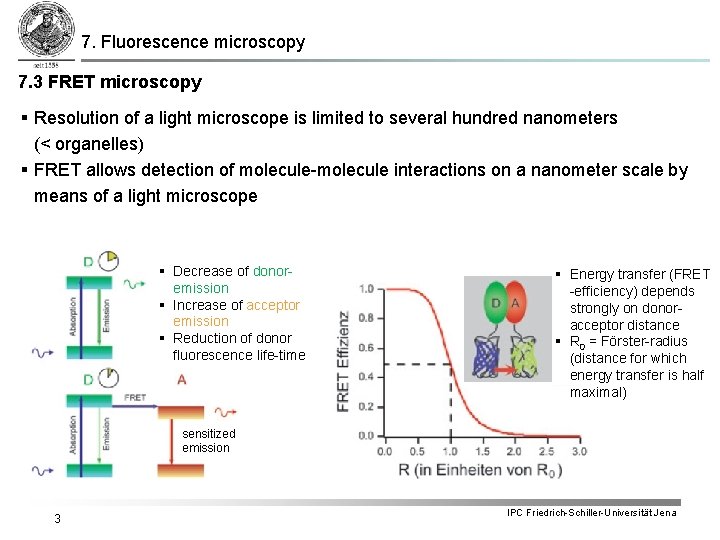
7. Fluorescence microscopy 7. 3 FRET microscopy § Resolution of a light microscope is limited to several hundred nanometers (< organelles) § FRET allows detection of molecule-molecule interactions on a nanometer scale by means of a light microscope § Decrease of donoremission § Increase of acceptor emission § Reduction of donor fluorescence life-time § Energy transfer (FRET -efficiency) depends strongly on donoracceptor distance § R 0 = Förster-radius (distance for which energy transfer is half maximal) sensitized emission 3 IPC Friedrich-Schiller-Universität Jena
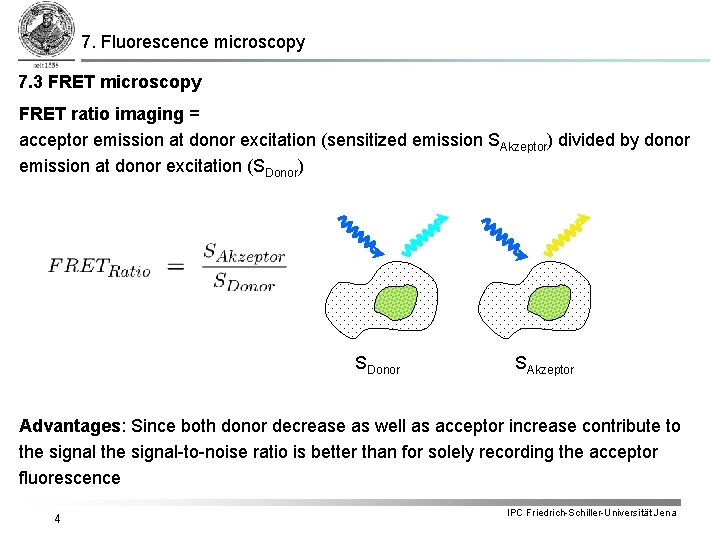
7. Fluorescence microscopy 7. 3 FRET microscopy FRET ratio imaging = acceptor emission at donor excitation (sensitized emission SAkzeptor) divided by donor emission at donor excitation (SDonor) SDonor SAkzeptor Advantages: Since both donor decrease as well as acceptor increase contribute to the signal-to-noise ratio is better than for solely recording the acceptor fluorescence 4 IPC Friedrich-Schiller-Universität Jena
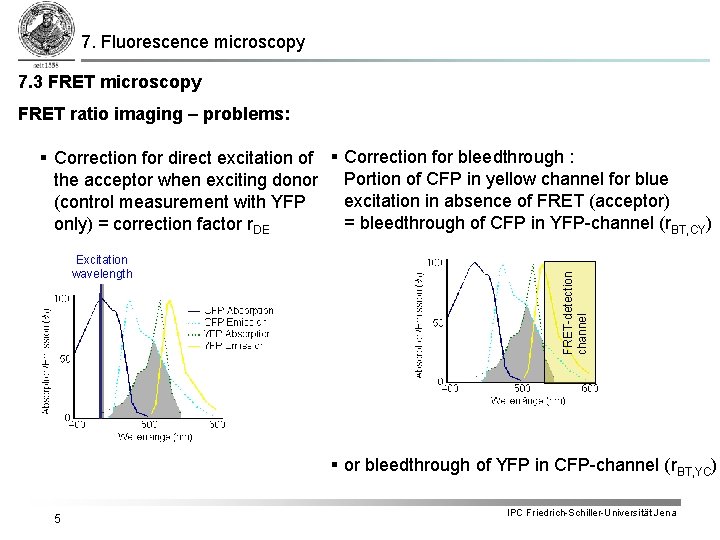
7. Fluorescence microscopy 7. 3 FRET microscopy FRET ratio imaging – problems: Excitation wavelength FRET-detection channel § Correction for direct excitation of § Correction for bleedthrough : the acceptor when exciting donor Portion of CFP in yellow channel for blue excitation in absence of FRET (acceptor) (control measurement with YFP = bleedthrough of CFP in YFP-channel (r. BT, CY) only) = correction factor r. DE § or bleedthrough of YFP in CFP-channel (r. BT, YC) 5 IPC Friedrich-Schiller-Universität Jena
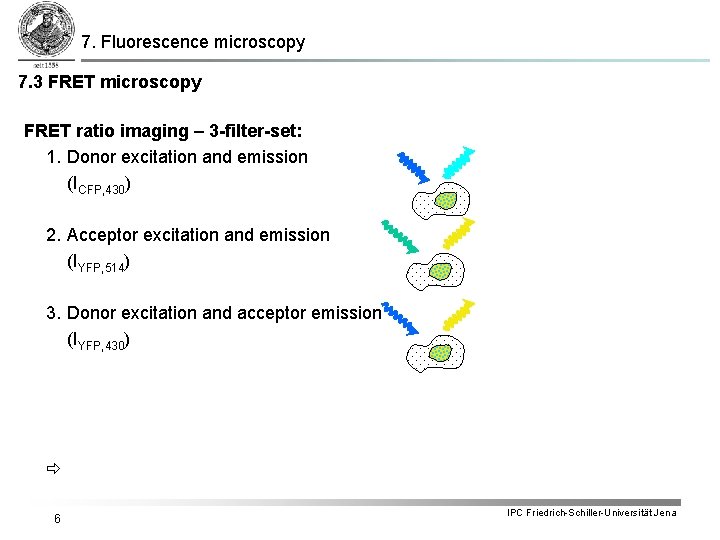
7. Fluorescence microscopy 7. 3 FRET microscopy FRET ratio imaging – 3 -filter-set: 1. Donor excitation and emission (ICFP, 430) 2. Acceptor excitation and emission (IYFP, 514) 3. Donor excitation and acceptor emission (IYFP, 430) 6 IPC Friedrich-Schiller-Universität Jena
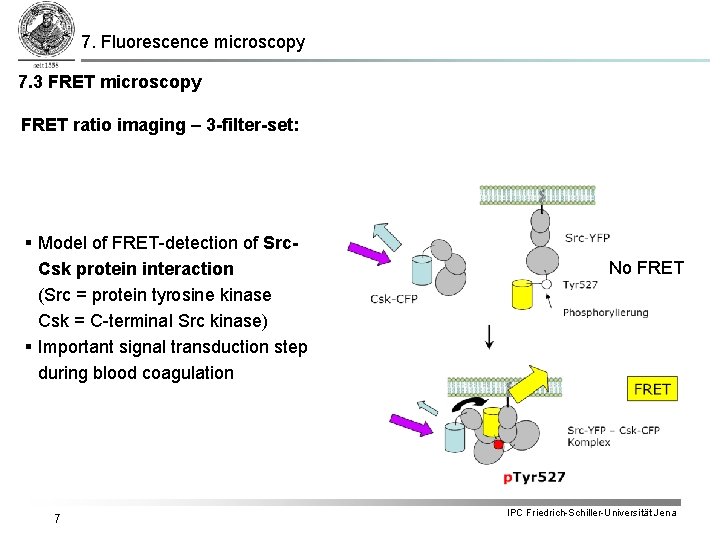
7. Fluorescence microscopy 7. 3 FRET microscopy FRET ratio imaging – 3 -filter-set: § Model of FRET-detection of Src. Csk protein interaction (Src = protein tyrosine kinase Csk = C-terminal Src kinase) § Important signal transduction step during blood coagulation 7 No FRET IPC Friedrich-Schiller-Universität Jena
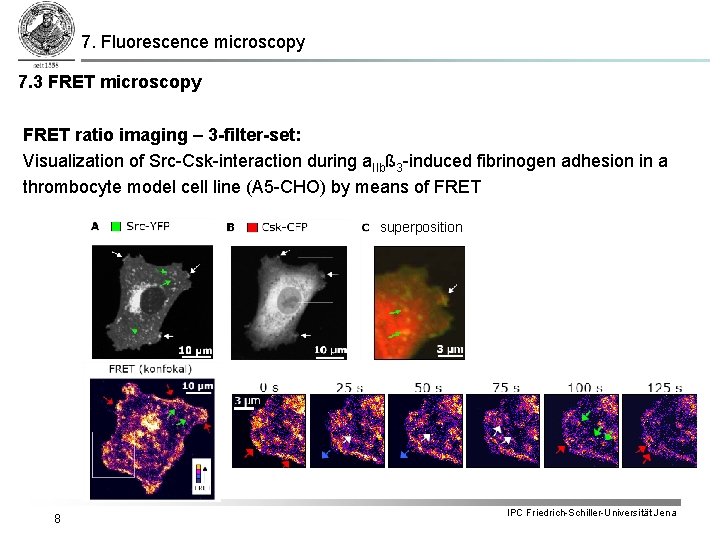
7. Fluorescence microscopy 7. 3 FRET microscopy FRET ratio imaging – 3 -filter-set: Visualization of Src-Csk-interaction during a. IIbß 3 -induced fibrinogen adhesion in a thrombocyte model cell line (A 5 -CHO) by means of FRET superposition 8 IPC Friedrich-Schiller-Universität Jena
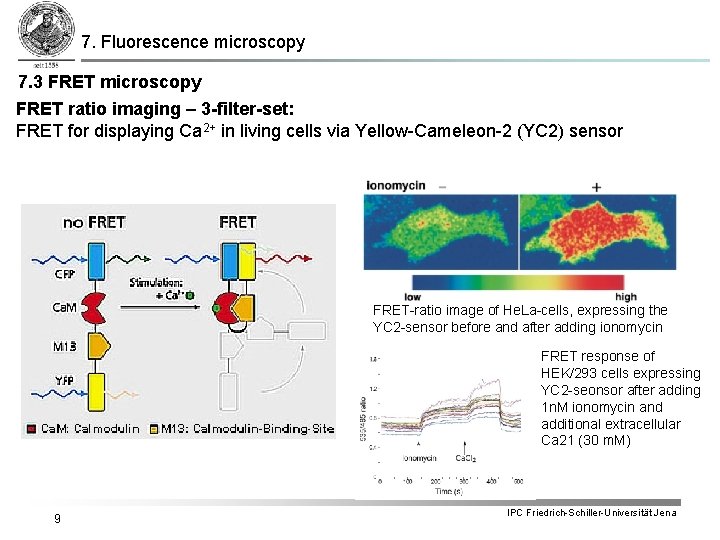
7. Fluorescence microscopy 7. 3 FRET microscopy FRET ratio imaging – 3 -filter-set: FRET for displaying Ca 2+ in living cells via Yellow-Cameleon-2 (YC 2) sensor FRET-ratio image of He. La-cells, expressing the YC 2 -sensor before and after adding ionomycin FRET response of HEK/293 cells expressing YC 2 -seonsor after adding 1 n. M ionomycin and additional extracellular Ca 21 (30 m. M) 9 IPC Friedrich-Schiller-Universität Jena
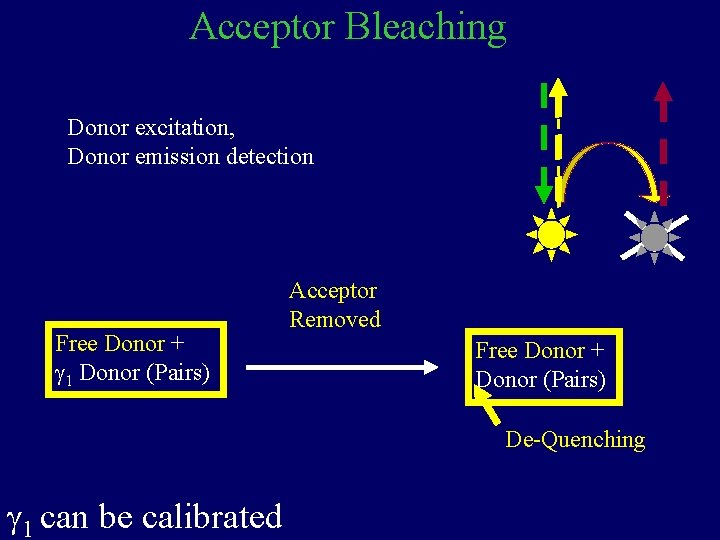
Acceptor Bleaching Donor excitation, Donor emission detection Free Donor + g 1 Donor (Pairs) Acceptor Removed Free Donor + Donor (Pairs) De-Quenching g 1 can be calibrated
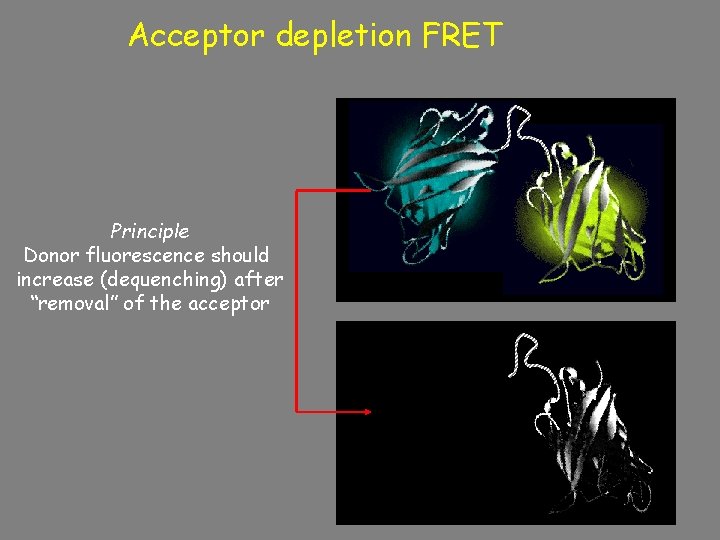
Acceptor depletion FRET Principle of Acceptor-bleaching. FRET microscopy Principle Donor fluorescence should increase (dequenching) after “removal” of the acceptor Donor fluorescence should increase after acceptor bleaching
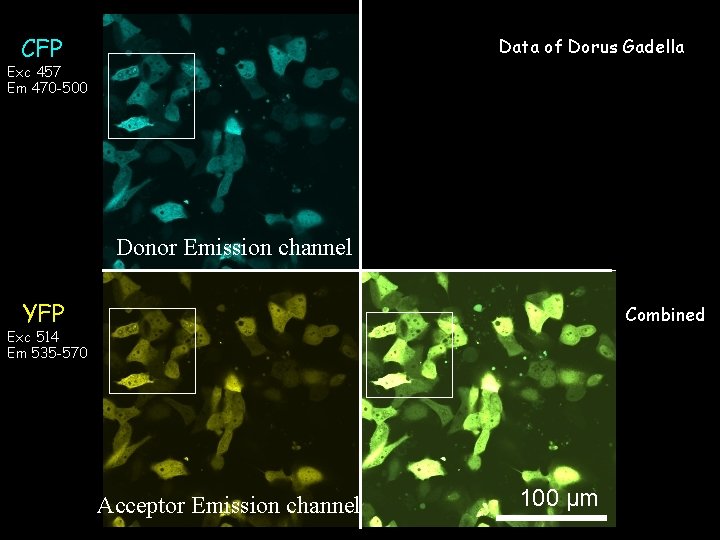
Data of Dorus ‘Sens-YFP’ Gadella CFP Exc 457 Em 470 -500 Exc 457 Em 535 -570 Donor Emission channel YFP Combined Exc 514 Em 535 -570 Acceptor Emission channel 100 µm
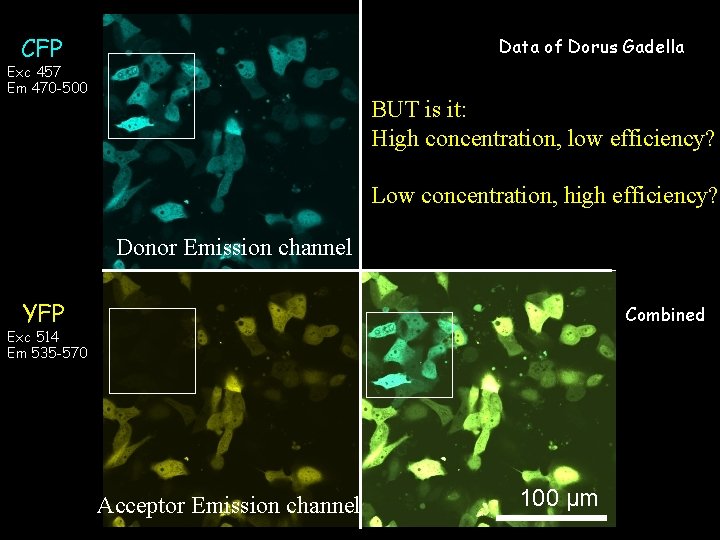
Data of Dorus ‘Sens-YFP’ Gadella CFP Exc 457 Em 470 -500 Exc 457 Em 535 -570 BUT is it: High concentration, low efficiency? Low concentration, high efficiency? Donor Emission channel YFP Combined Exc 514 Em 535 -570 Acceptor Emission channel 100 µm
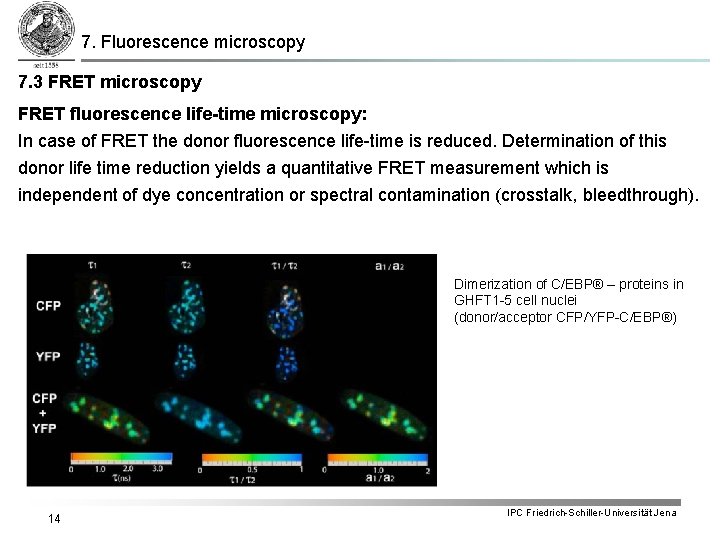
7. Fluorescence microscopy 7. 3 FRET microscopy FRET fluorescence life-time microscopy: In case of FRET the donor fluorescence life-time is reduced. Determination of this donor life time reduction yields a quantitative FRET measurement which is independent of dye concentration or spectral contamination (crosstalk, bleedthrough). Dimerization of C/EBP® – proteins in GHFT 1 -5 cell nuclei (donor/acceptor CFP/YFP-C/EBP®) 14 IPC Friedrich-Schiller-Universität Jena
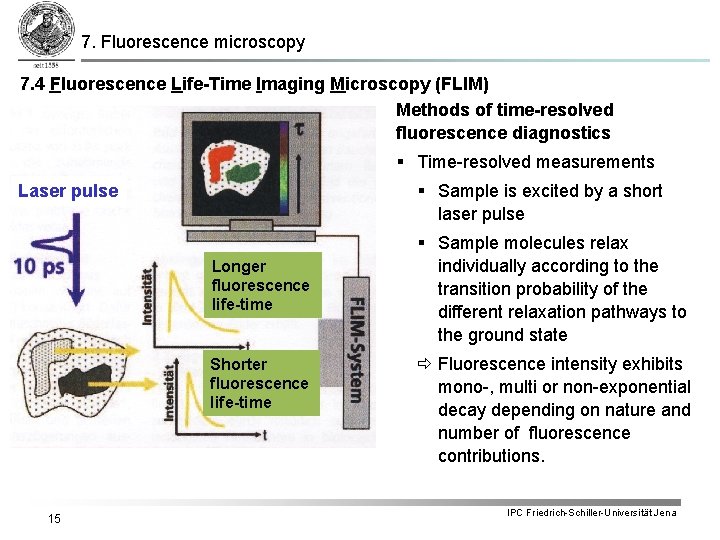
7. Fluorescence microscopy 7. 4 Fluorescence Life-Time Imaging Microscopy (FLIM) Methods of time-resolved fluorescence diagnostics § Time-resolved measurements § Sample is excited by a short laser pulse Longer fluorescence life-time Shorter fluorescence life-time 15 § Sample molecules relax individually according to the transition probability of the different relaxation pathways to the ground state Fluorescence intensity exhibits mono-, multi or non-exponential decay depending on nature and number of fluorescence contributions. IPC Friedrich-Schiller-Universität Jena
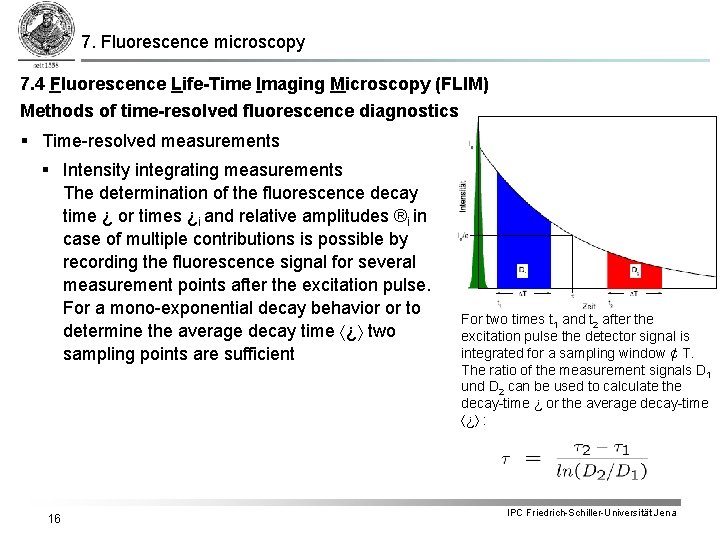
7. Fluorescence microscopy 7. 4 Fluorescence Life-Time Imaging Microscopy (FLIM) Methods of time-resolved fluorescence diagnostics § Time-resolved measurements § Intensity integrating measurements The determination of the fluorescence decay time ¿ or times ¿i and relative amplitudes ®i in case of multiple contributions is possible by recording the fluorescence signal for several measurement points after the excitation pulse. For a mono-exponential decay behavior or to determine the average decay time ¿ two sampling points are sufficient 16 For two times t 1 and t 2 after the excitation pulse the detector signal is integrated for a sampling window ¢ T. The ratio of the measurement signals D 1 und D 2 can be used to calculate the decay-time ¿ or the average decay-time ¿ : IPC Friedrich-Schiller-Universität Jena
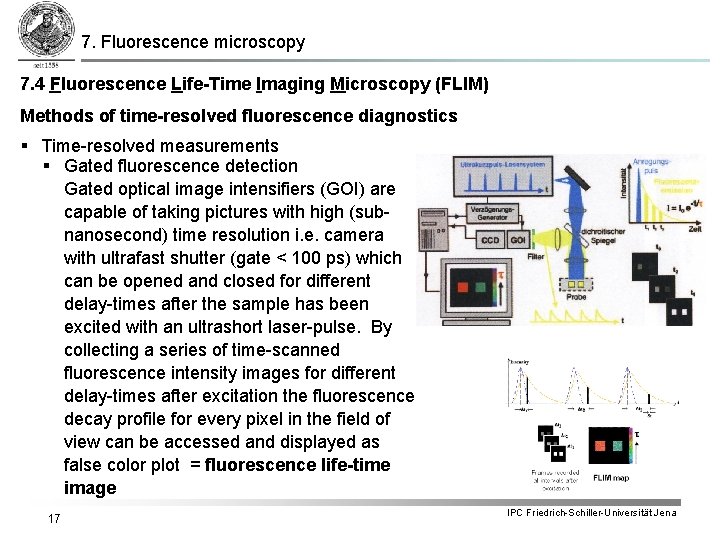
7. Fluorescence microscopy 7. 4 Fluorescence Life-Time Imaging Microscopy (FLIM) Methods of time-resolved fluorescence diagnostics § Time-resolved measurements § Gated fluorescence detection Gated optical image intensifiers (GOI) are capable of taking pictures with high (subnanosecond) time resolution i. e. camera with ultrafast shutter (gate < 100 ps) which can be opened and closed for different delay-times after the sample has been excited with an ultrashort laser-pulse. By collecting a series of time-scanned fluorescence intensity images for different delay-times after excitation the fluorescence decay profile for every pixel in the field of view can be accessed and displayed as false color plot = fluorescence life-time image 17 IPC Friedrich-Schiller-Universität Jena
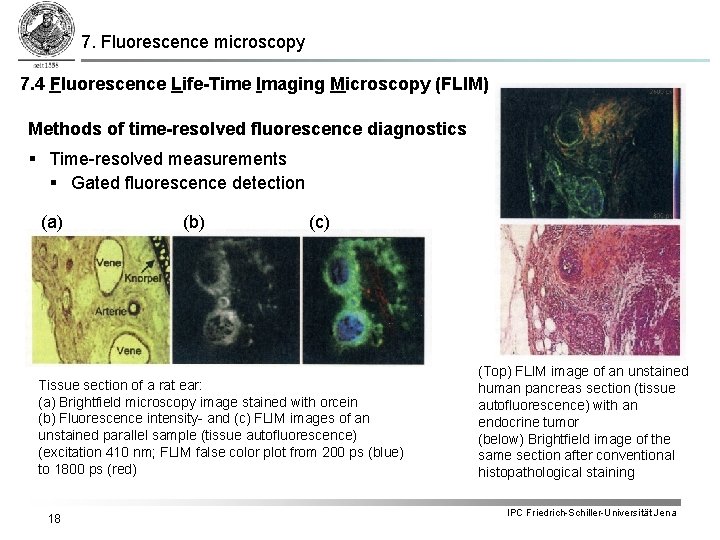
7. Fluorescence microscopy 7. 4 Fluorescence Life-Time Imaging Microscopy (FLIM) Methods of time-resolved fluorescence diagnostics § Time-resolved measurements § Gated fluorescence detection (a) (b) (c) Tissue section of a rat ear: (a) Brightfield microscopy image stained with orcein (b) Fluorescence intensity- and (c) FLIM images of an unstained parallel sample (tissue autofluorescence) (excitation 410 nm; FLIM false color plot from 200 ps (blue) to 1800 ps (red) 18 (Top) FLIM image of an unstained human pancreas section (tissue autofluorescence) with an endocrine tumor (below) Brightfield image of the same section after conventional histopathological staining IPC Friedrich-Schiller-Universität Jena
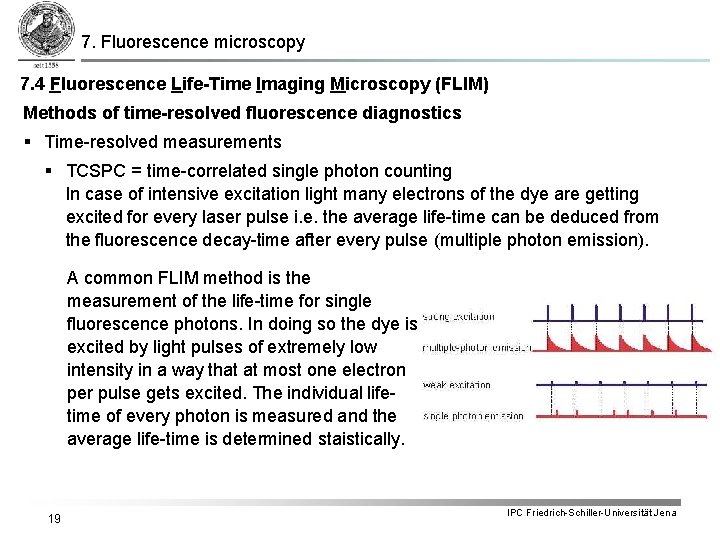
7. Fluorescence microscopy 7. 4 Fluorescence Life-Time Imaging Microscopy (FLIM) Methods of time-resolved fluorescence diagnostics § Time-resolved measurements § TCSPC = time-correlated single photon counting In case of intensive excitation light many electrons of the dye are getting excited for every laser pulse i. e. the average life-time can be deduced from the fluorescence decay-time after every pulse (multiple photon emission). A common FLIM method is the measurement of the life-time for single fluorescence photons. In doing so the dye is excited by light pulses of extremely low intensity in a way that at most one electron per pulse gets excited. The individual lifetime of every photon is measured and the average life-time is determined staistically. 19 IPC Friedrich-Schiller-Universität Jena
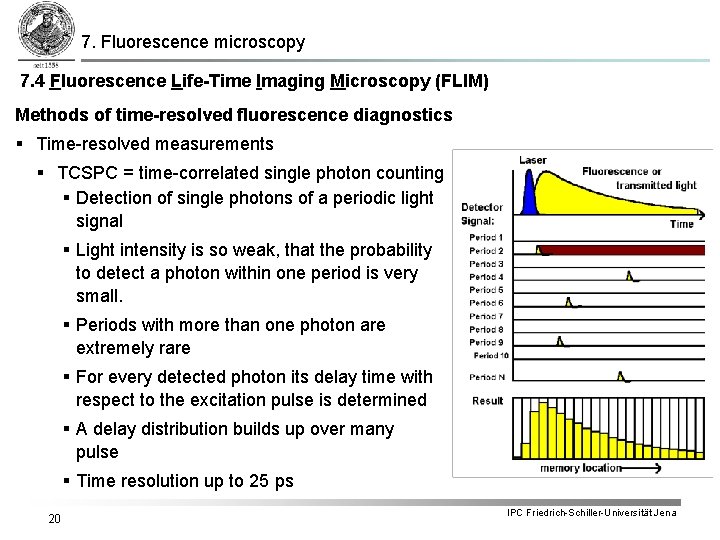
7. Fluorescence microscopy 7. 4 Fluorescence Life-Time Imaging Microscopy (FLIM) Methods of time-resolved fluorescence diagnostics § Time-resolved measurements § TCSPC = time-correlated single photon counting § Detection of single photons of a periodic light signal § Light intensity is so weak, that the probability to detect a photon within one period is very small. § Periods with more than one photon are extremely rare § For every detected photon its delay time with respect to the excitation pulse is determined § A delay distribution builds up over many pulse § Time resolution up to 25 ps 20 IPC Friedrich-Schiller-Universität Jena
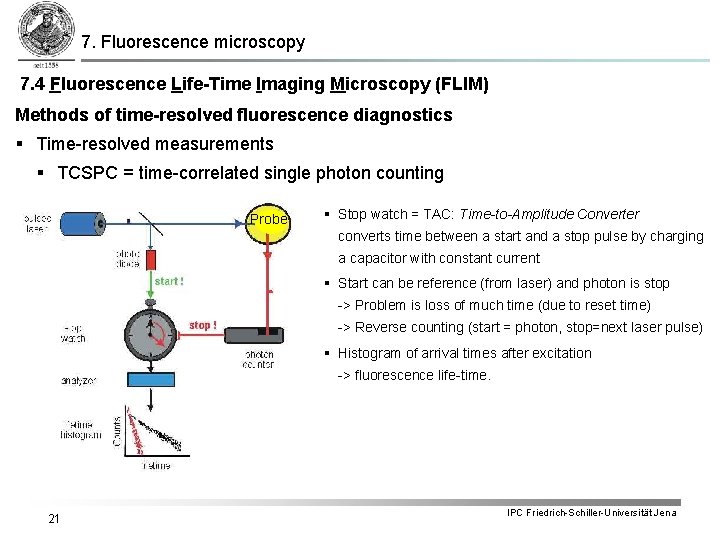
7. Fluorescence microscopy 7. 4 Fluorescence Life-Time Imaging Microscopy (FLIM) Methods of time-resolved fluorescence diagnostics § Time-resolved measurements § TCSPC = time-correlated single photon counting Probe § Stop watch = TAC: Time-to-Amplitude Converter converts time between a start and a stop pulse by charging a capacitor with constant current § Start can be reference (from laser) and photon is stop -> Problem is loss of much time (due to reset time) -> Reverse counting (start = photon, stop=next laser pulse) § Histogram of arrival times after excitation -> fluorescence life-time. 21 IPC Friedrich-Schiller-Universität Jena
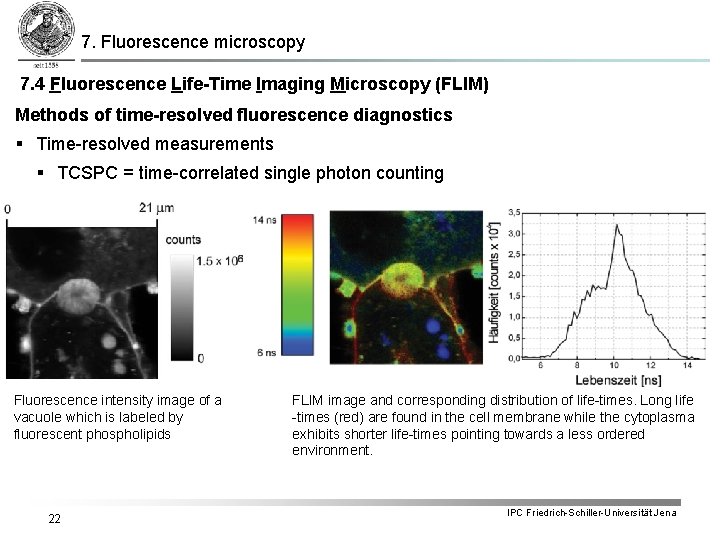
7. Fluorescence microscopy 7. 4 Fluorescence Life-Time Imaging Microscopy (FLIM) Methods of time-resolved fluorescence diagnostics § Time-resolved measurements § TCSPC = time-correlated single photon counting Fluorescence intensity image of a vacuole which is labeled by fluorescent phospholipids 22 FLIM image and corresponding distribution of life-times. Long life -times (red) are found in the cell membrane while the cytoplasma exhibits shorter life-times pointing towards a less ordered environment. IPC Friedrich-Schiller-Universität Jena
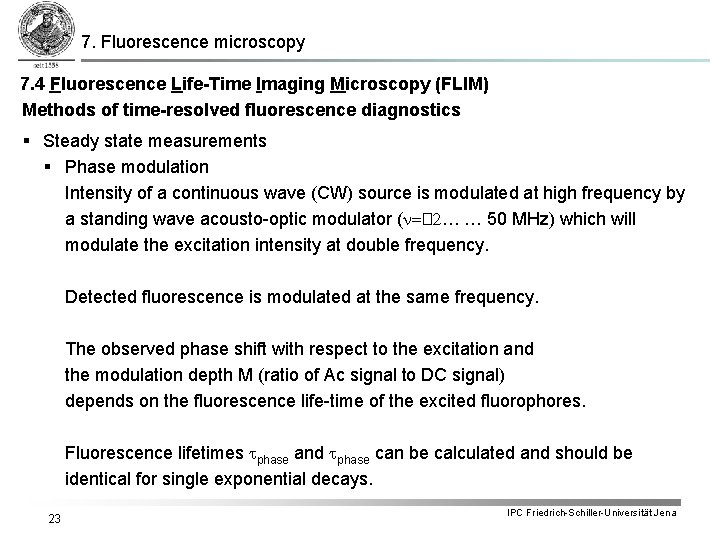
7. Fluorescence microscopy 7. 4 Fluorescence Life-Time Imaging Microscopy (FLIM) Methods of time-resolved fluorescence diagnostics § Steady state measurements § Phase modulation Intensity of a continuous wave (CW) source is modulated at high frequency by a standing wave acousto-optic modulator (n=� 2¼ ¼ 50 MHz) which will modulate the excitation intensity at double frequency. Detected fluorescence is modulated at the same frequency. The observed phase shift with respect to the excitation and the modulation depth M (ratio of Ac signal to DC signal) depends on the fluorescence life-time of the excited fluorophores. Fluorescence lifetimes tphase and tphase can be calculated and should be identical for single exponential decays. 23 IPC Friedrich-Schiller-Universität Jena
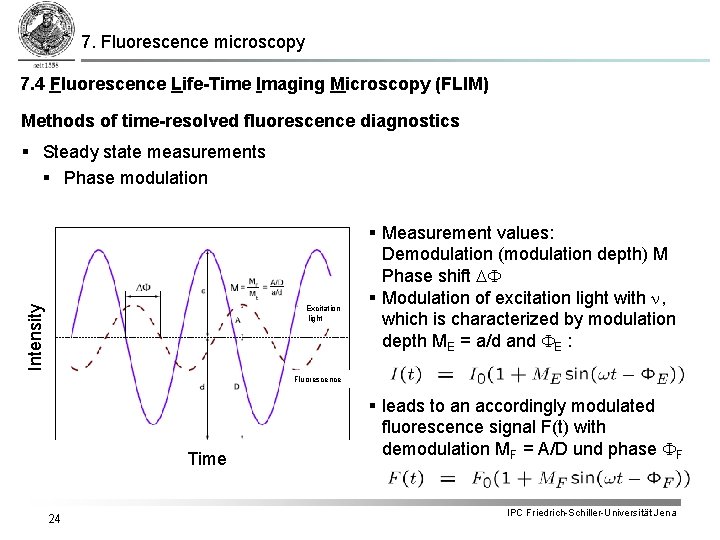
7. Fluorescence microscopy 7. 4 Fluorescence Life-Time Imaging Microscopy (FLIM) Methods of time-resolved fluorescence diagnostics Intensity § Steady state measurements § Phase modulation Excitation light § Measurement values: Demodulation (modulation depth) M Phase shift DF § Modulation of excitation light with n, which is characterized by modulation depth ME = a/d and FE : Fluorescence Time 24 § leads to an accordingly modulated fluorescence signal F(t) with demodulation MF = A/D und phase FF IPC Friedrich-Schiller-Universität Jena
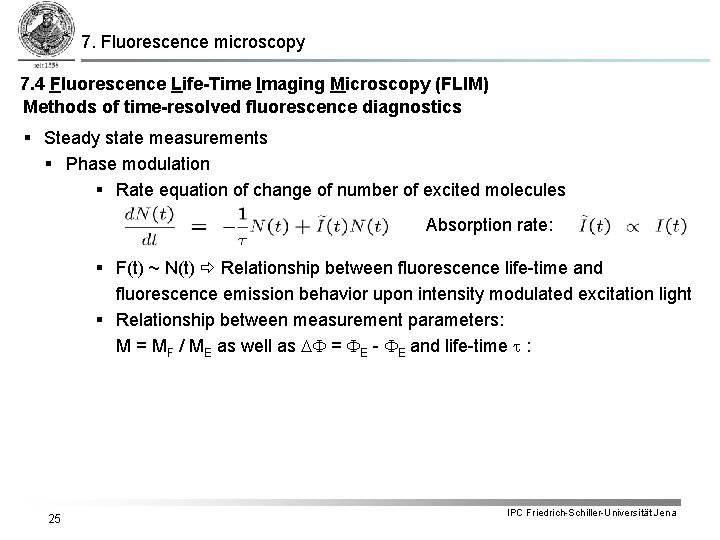
7. Fluorescence microscopy 7. 4 Fluorescence Life-Time Imaging Microscopy (FLIM) Methods of time-resolved fluorescence diagnostics § Steady state measurements § Phase modulation § Rate equation of change of number of excited molecules Absorption rate: § F(t) ~ N(t) Relationship between fluorescence life-time and fluorescence emission behavior upon intensity modulated excitation light § Relationship between measurement parameters: M = MF / ME as well as DF = FE - FE and life-time t : 25 IPC Friedrich-Schiller-Universität Jena
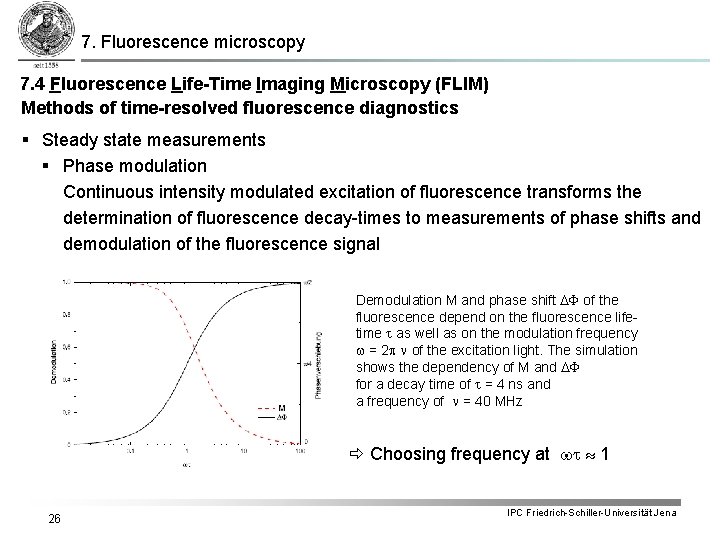
7. Fluorescence microscopy 7. 4 Fluorescence Life-Time Imaging Microscopy (FLIM) Methods of time-resolved fluorescence diagnostics § Steady state measurements § Phase modulation Continuous intensity modulated excitation of fluorescence transforms the determination of fluorescence decay-times to measurements of phase shifts and demodulation of the fluorescence signal Demodulation M and phase shift DF of the fluorescence depend on the fluorescence lifetime t as well as on the modulation frequency w = 2 p n of the excitation light. The simulation shows the dependency of M and DF for a decay time of t = 4 ns and a frequency of n = 40 MHz Choosing frequency at wt 1 26 IPC Friedrich-Schiller-Universität Jena
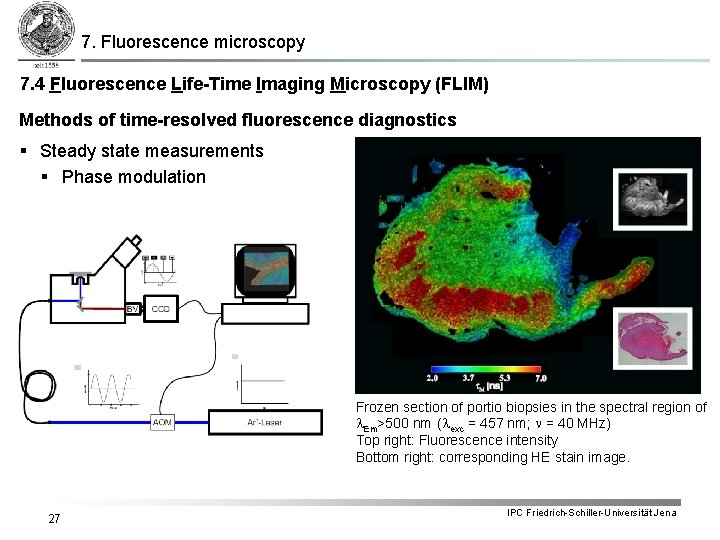
7. Fluorescence microscopy 7. 4 Fluorescence Life-Time Imaging Microscopy (FLIM) Methods of time-resolved fluorescence diagnostics § Steady state measurements § Phase modulation Frozen section of portio biopsies in the spectral region of l. Em>500 nm (lexc = 457 nm; n = 40 MHz) Top right: Fluorescence intensity Bottom right: corresponding HE stain image. 27 IPC Friedrich-Schiller-Universität Jena
Confocal fluorescence microscopy
Laser confocal microscopy
Bright field microscopy
Fluorescence microscopy uses
Light sources for fluorescence microscopy
Rt pcr primer efficiency
Stifttand konijn
Fret formula
Saggio fret
Cold vapor atomic fluorescence spectrometry
Jablonksi diagram
Cold vapor atomic fluorescence spectrometry
Explain quantum yield
Jablonksi diagram
Fluorescence bandpass filter
Fluorescence recovery after photobleaching
Atomic emission spectroscopy ppt
Principles of fluorescence spectroscopy
Chemiluminescence vs fluorescence
Atomic fluorescence spectroscopy principle
Limitations of the beer lambert law
Fluorescence recovery after photobleaching
Fluorescence activated cell sorting
Fluorescence-activated cell sorting (facs)
Principle of fluorescence spectroscopy
Biotek flx800 fluorescence microplate reader
Rfu fluorescence
Jablonksi diagram