5 Electromagnetic Optics Light is an electromagnetic wave
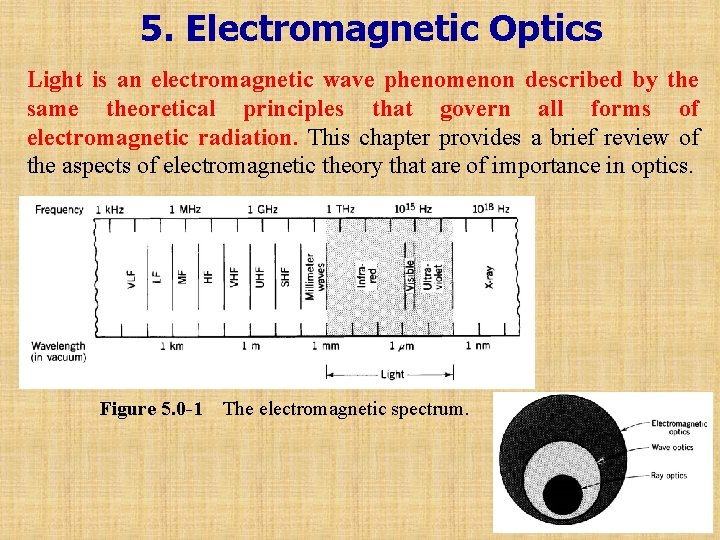
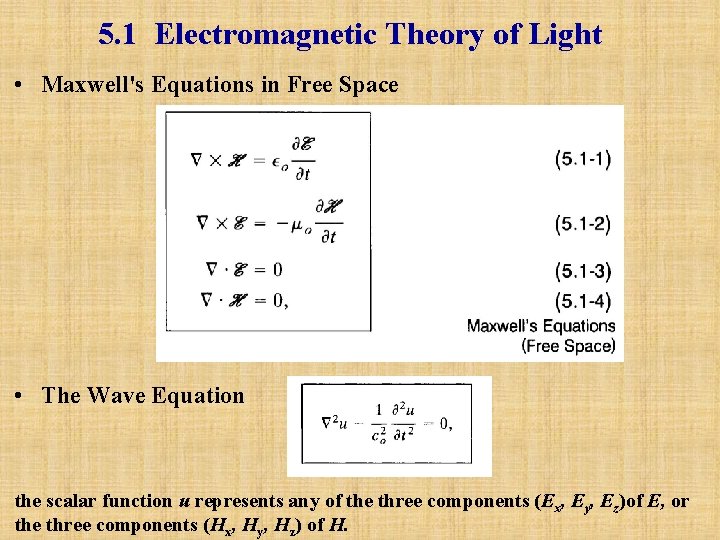
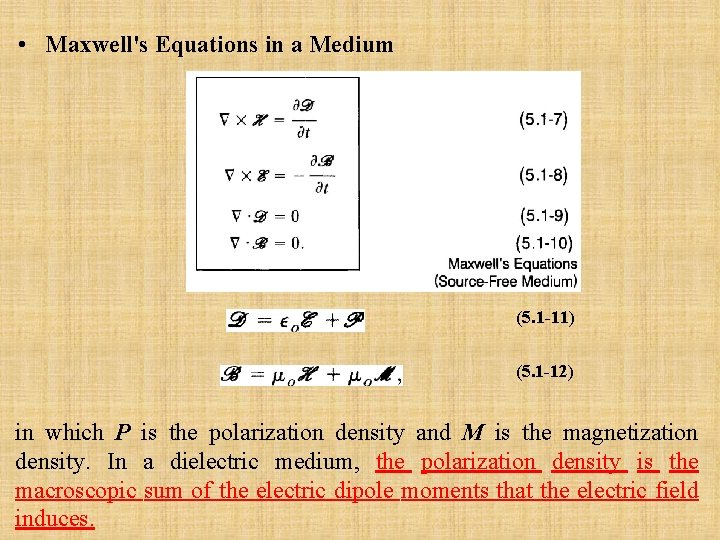
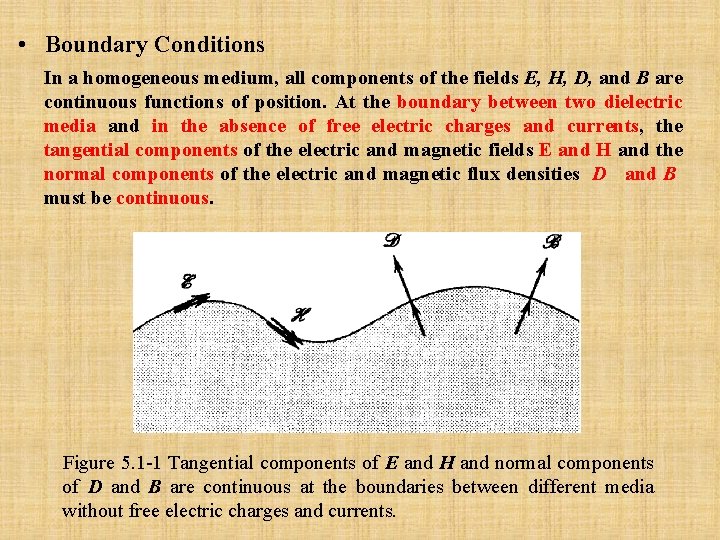
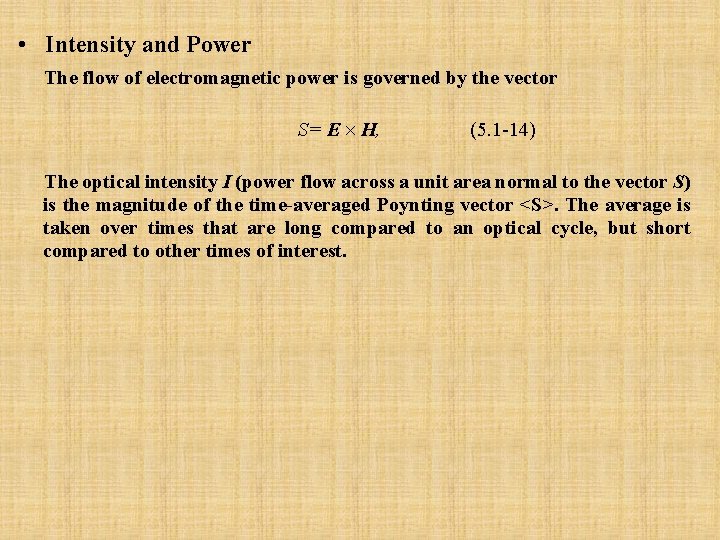
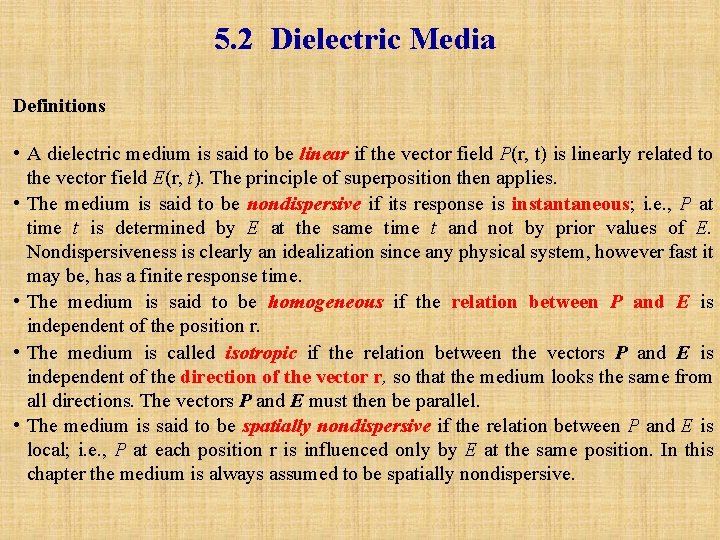
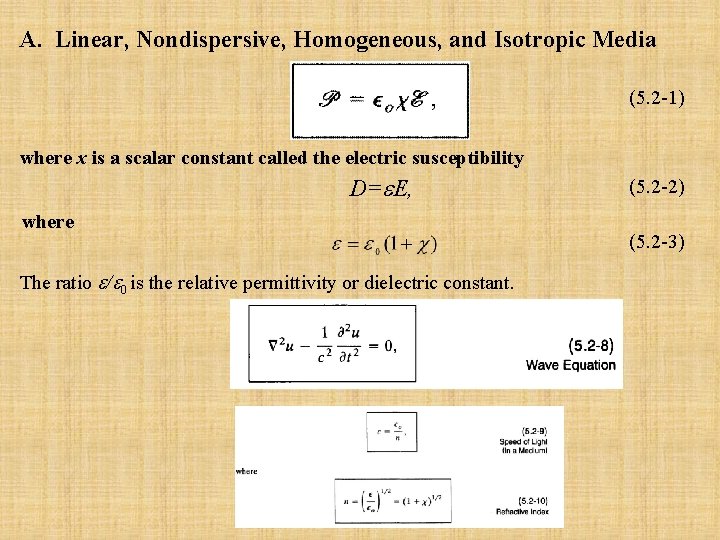
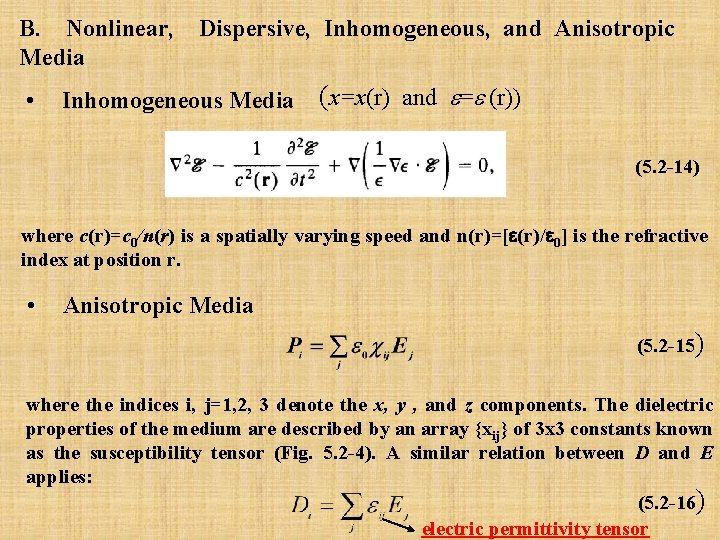
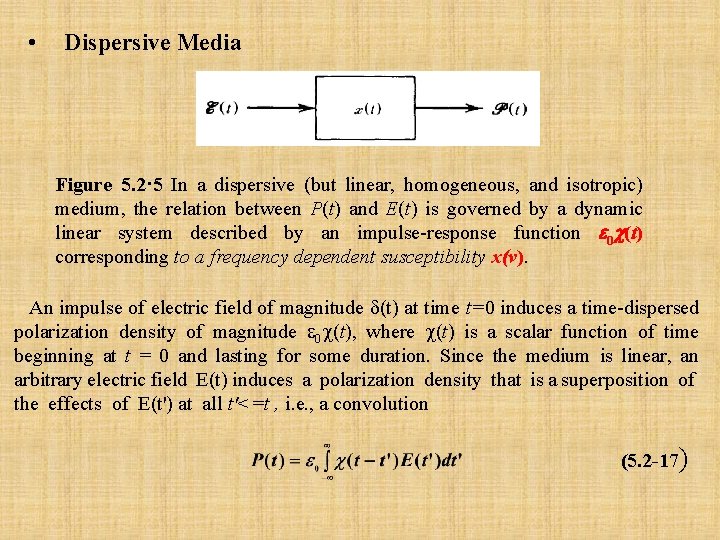
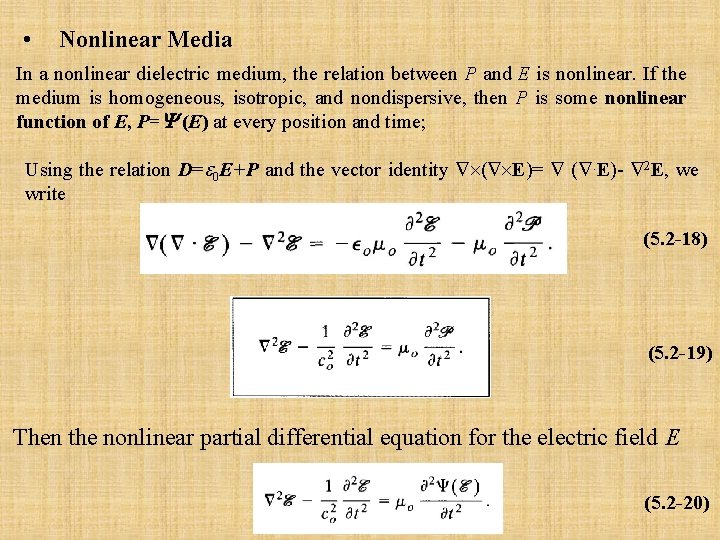
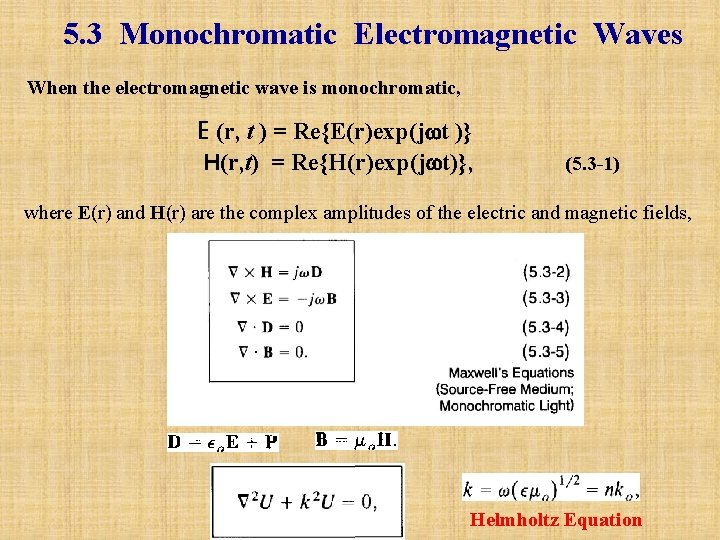
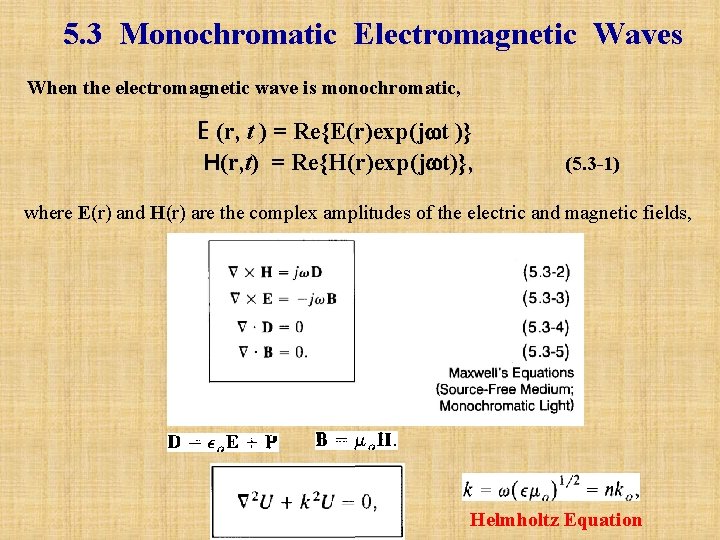
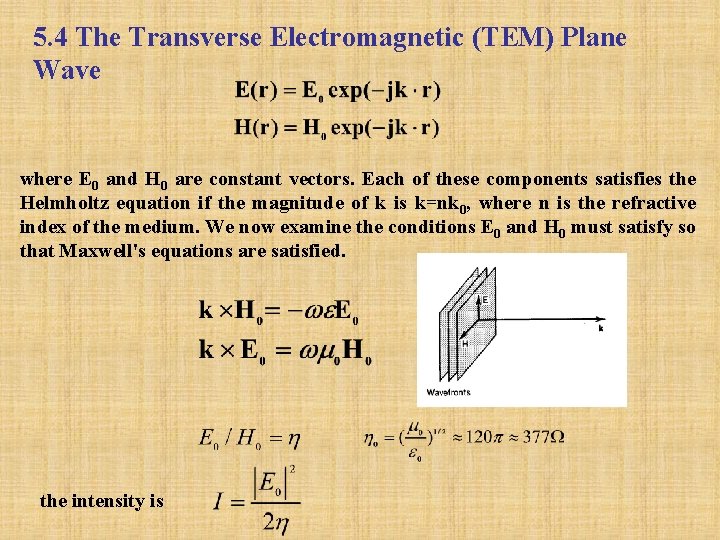
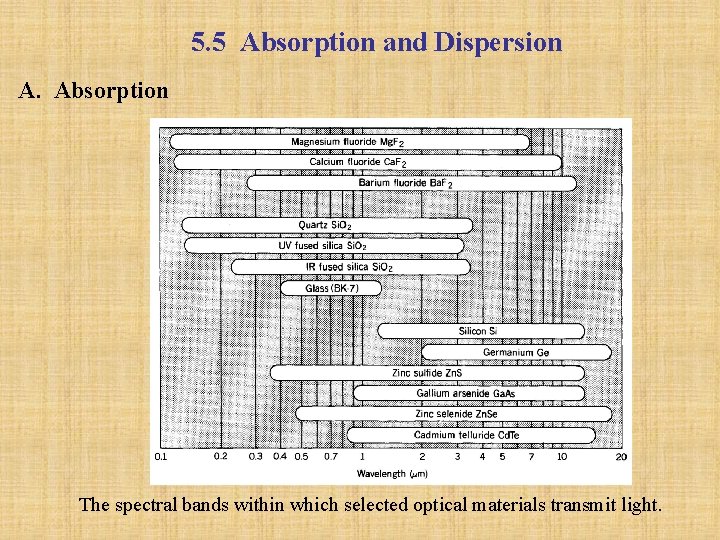
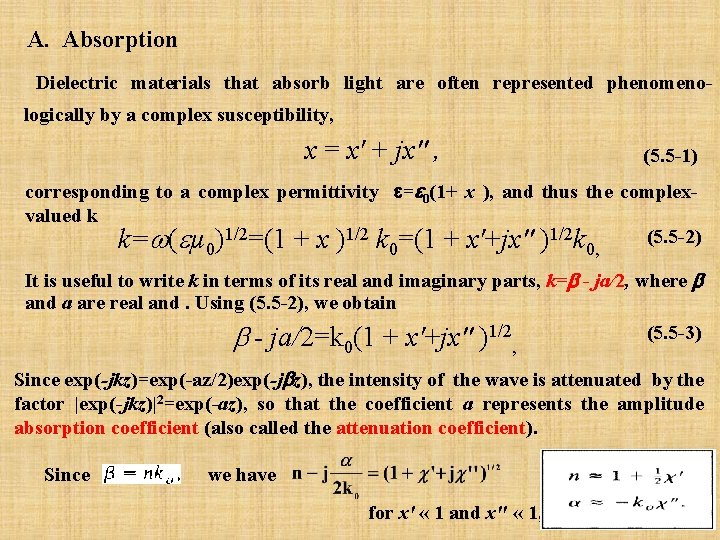
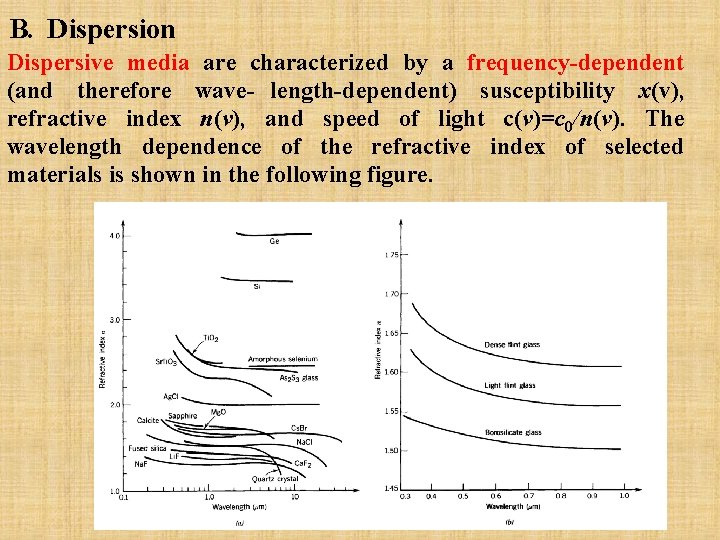
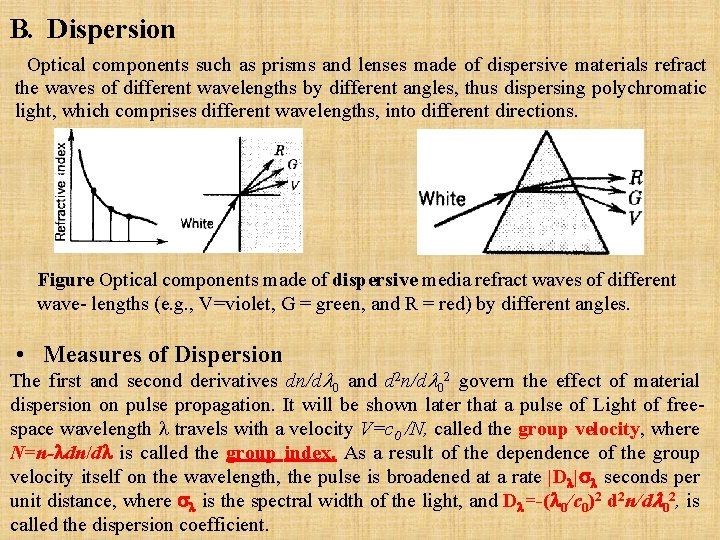
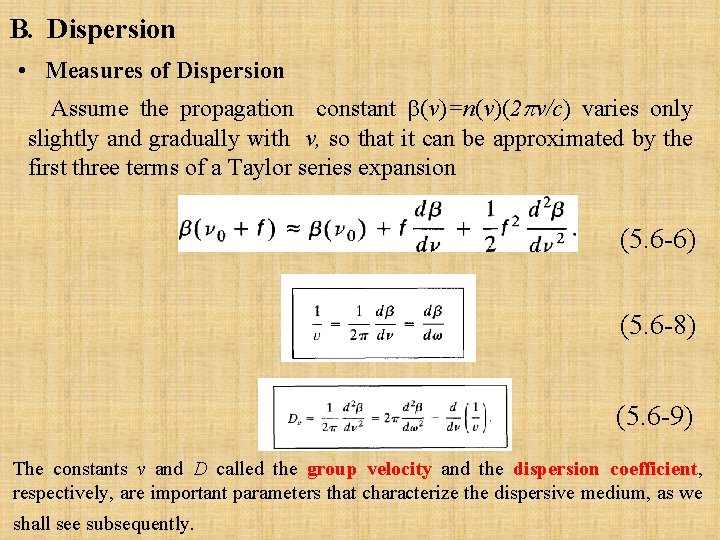
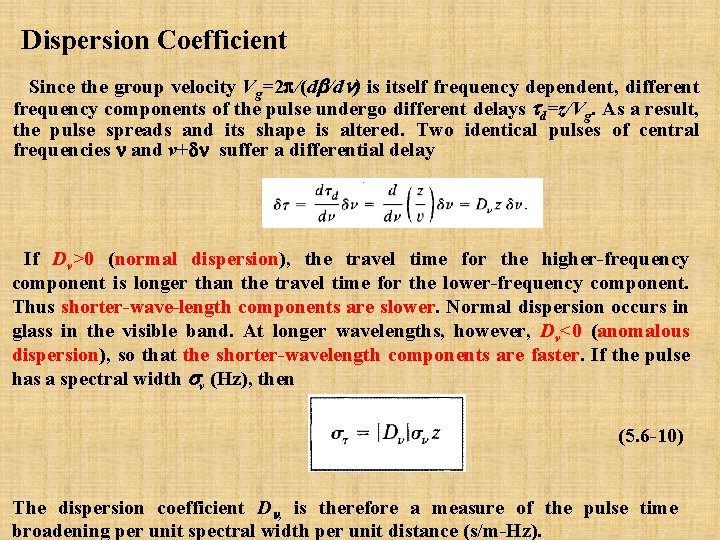
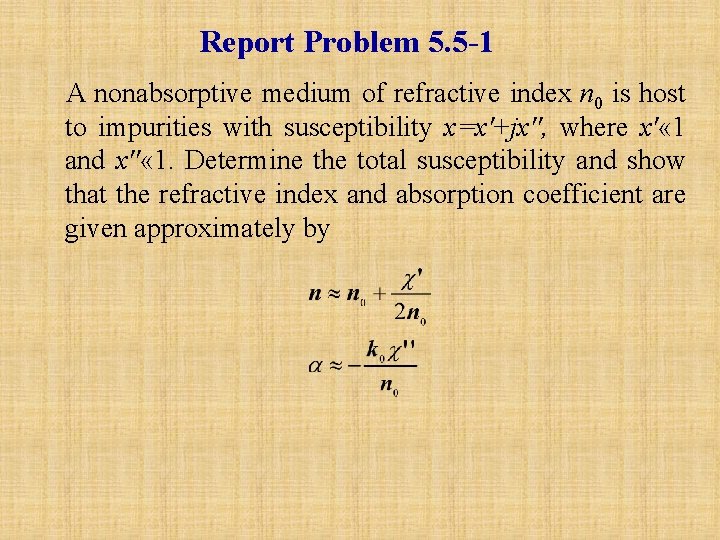
- Slides: 20
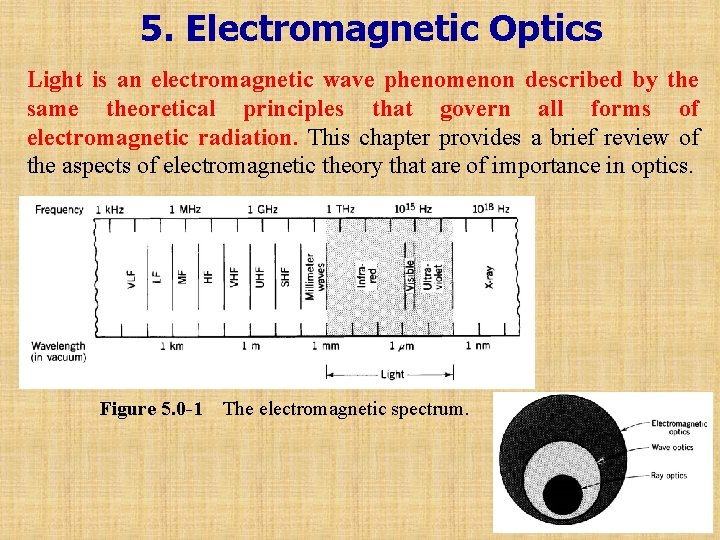
5. Electromagnetic Optics Light is an electromagnetic wave phenomenon described by the same theoretical principles that govern all forms of electromagnetic radiation. This chapter provides a brief review of the aspects of electromagnetic theory that are of importance in optics. Figure 5. 0 1 The electromagnetic spectrum.
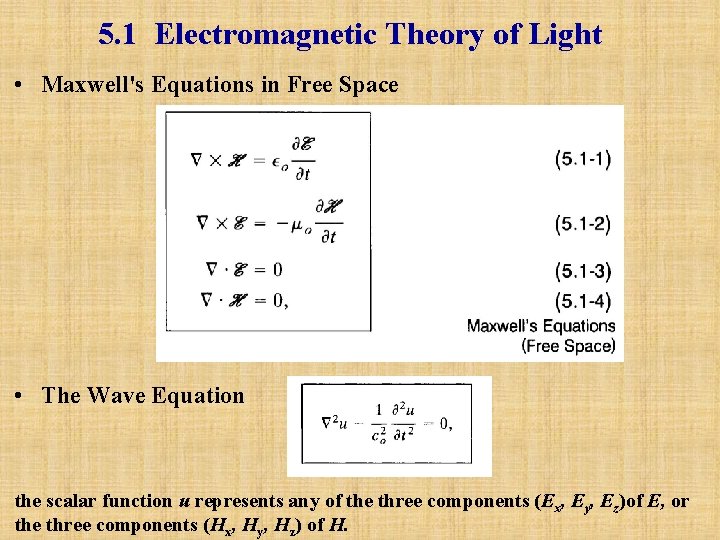
5. 1 Electromagnetic Theory of Light • Maxwell's Equations in Free Space • The Wave Equation the scalar function u represents any of the three components (Ex, Ey, Ez)of E, or the three components (Hx, Hy, Hz) of H.
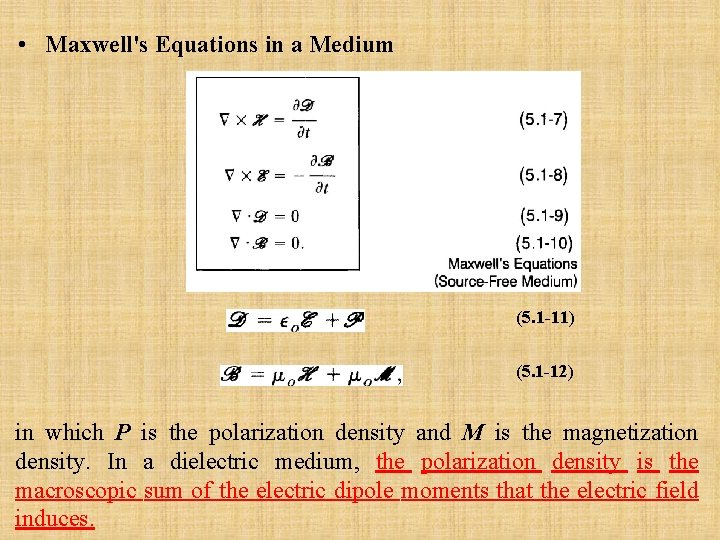
• Maxwell's Equations in a Medium (5. 1 11) (5. 1 12) in which P is the polarization density and M is the magnetization density. In a dielectric medium, the polarization density is the macroscopic sum of the electric dipole moments that the electric field induces.
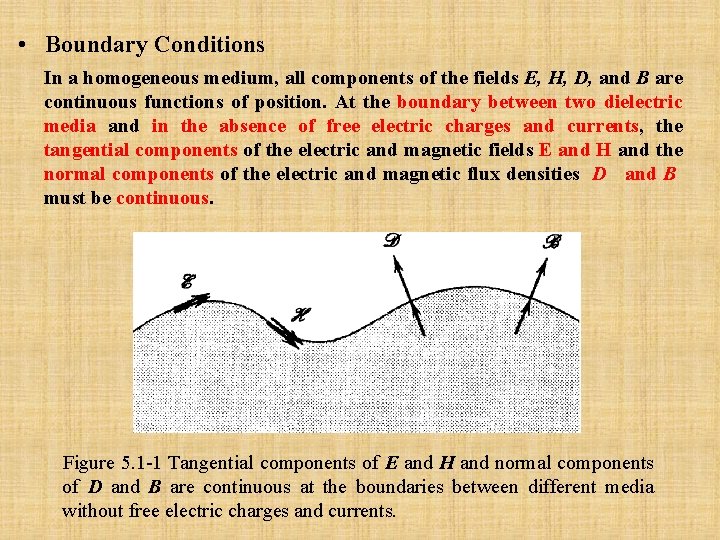
• Boundary Conditions In a homogeneous medium, all components of the fields E, H, D, and B are continuous functions of position. At the boundary between two dielectric media and in the absence of free electric charges and currents, the tangential components of the electric and magnetic fields E and H and the normal components of the electric and magnetic flux densities D and B must be continuous. Figure 5. 1 1 Tangential components of E and H and normal components of D and B are continuous at the boundaries between different media without free electric charges and currents.
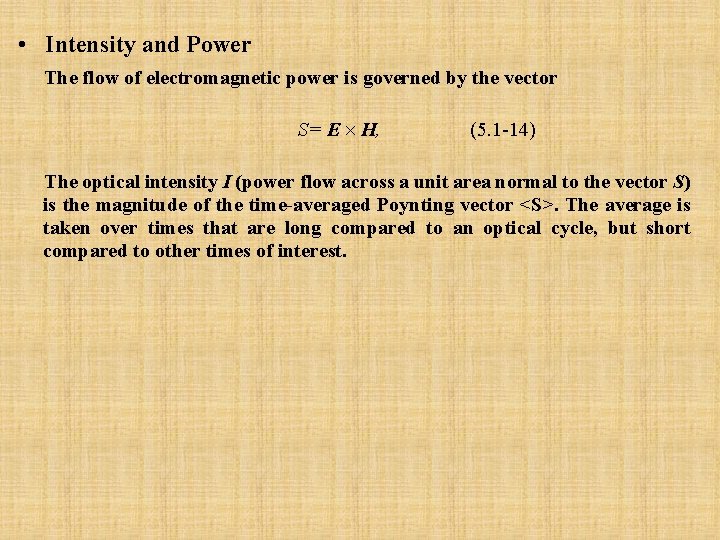
• Intensity and Power The flow of electromagnetic power is governed by the vector S= E H, (5. 1 14) The optical intensity I (power flow across a unit area normal to the vector S) is the magnitude of the time averaged Poynting vector <S>. The average is taken over times that are long compared to an optical cycle, but short compared to other times of interest.
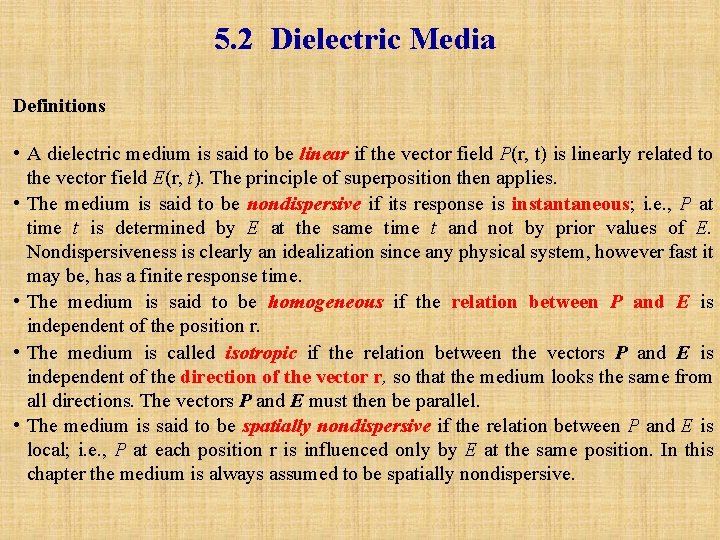
5. 2 Dielectric Media Definitions • A dielectric medium is said to be linear if the vector field P(r, t) is linearly related to the vector field E(r, t). The principle of superposition then applies. • The medium is said to be nondispersive if its response is instantaneous; i. e. , P at time t is determined by E at the same time t and not by prior values of E. Nondispersiveness is clearly an idealization since any physical system, however fast it may be, has a finite response time. • The medium is said to be homogeneous if the relation between P and E is independent of the position r. • The medium is called isotropic if the relation between the vectors P and E is independent of the direction of the vector r, so that the medium looks the same from all directions. The vectors P and E must then be parallel. • The medium is said to be spatially nondispersive if the relation between P and E is local; i. e. , P at each position r is influenced only by E at the same position. In this chapter the medium is always assumed to be spatially nondispersive.
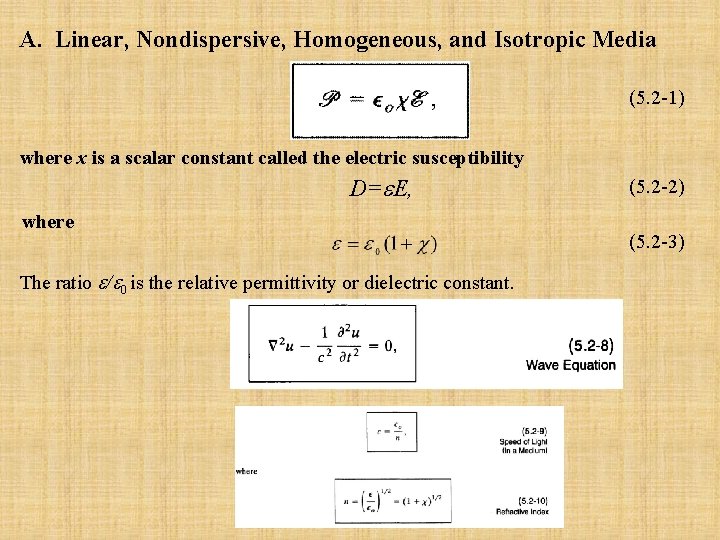
A. Linear, Nondispersive, Homogeneous, and Isotropic Media (5. 2 1) where x is a scalar constant called the electric susceptibility D= E, where The ratio / 0 is the relative permittivity or dielectric constant. (5. 2 2) (5. 2 3)
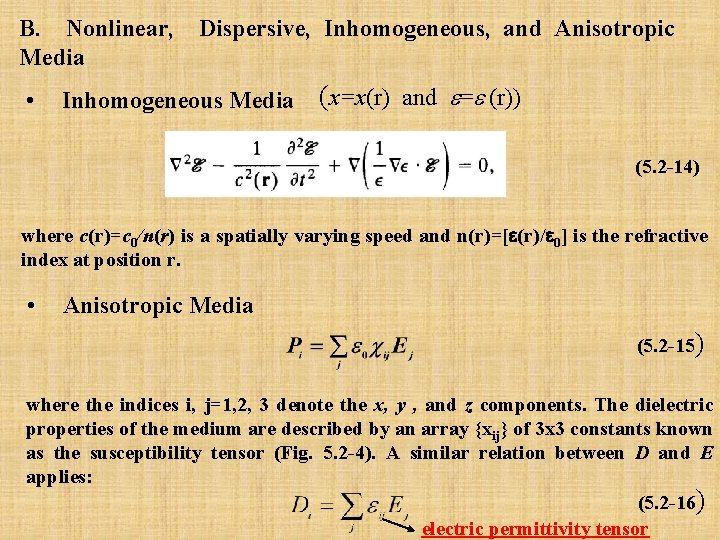
B. Nonlinear, Media • Dispersive, Inhomogeneous, and Anisotropic Inhomogeneous Media (x=x(r) and = (r)) (5. 2 14) where c(r)=c 0/n(r) is a spatially varying speed and n(r)=[ (r)/ 0] is the refractive index at position r. • Anisotropic Media (5. 2 15) where the indices i, j=1, 2, 3 denote the x, y , and z components. The dielectric properties of the medium are described by an array {xij} of 3 x 3 constants known as the susceptibility tensor (Fig. 5. 2 4). A similar relation between D and E applies: (5. 2 16) electric permittivity tensor
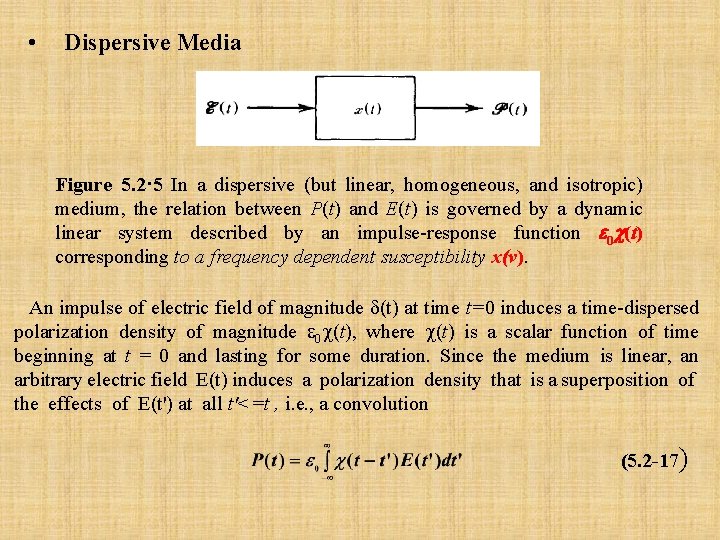
• Dispersive Media Figure 5. 2· 5 In a dispersive (but linear, homogeneous, and isotropic) medium, the relation between P(t) and E(t) is governed by a dynamic linear system described by an impulse response function 0 (t) corresponding to a frequency dependent susceptibility x(v). An impulse of electric field of magnitude (t) at time t=0 induces a time dispersed polarization density of magnitude 0 (t), where (t) is a scalar function of time beginning at t = 0 and lasting for some duration. Since the medium is linear, an arbitrary electric field E(t) induces a polarization density that is a superposition of the effects of E(t') at all t'<=t , i. e. , a convolution (5. 2 17)
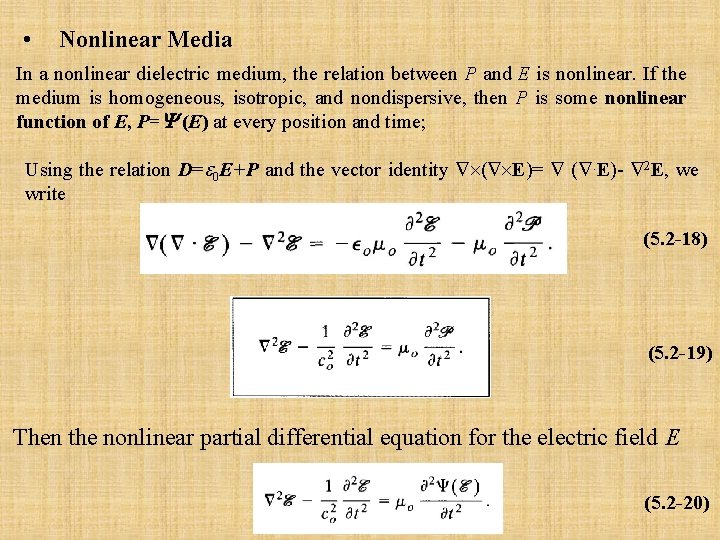
• Nonlinear Media In a nonlinear dielectric medium, the relation between P and E is nonlinear. If the medium is homogeneous, isotropic, and nondispersive, then P is some nonlinear function of E, P= (E) at every position and time; Using the relation D= 0 E+P and the vector identity ( E)= ( ·E) 2 E, we write (5. 2 18) (5. 2 19) Then the nonlinear partial differential equation for the electric field E (5. 2 20)
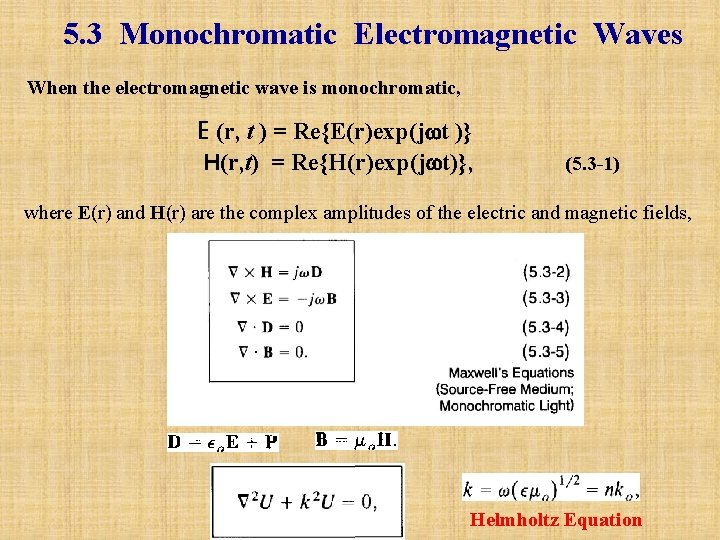
5. 3 Monochromatic Electromagnetic Waves When the electromagnetic wave is monochromatic, E (r, t ) = Re{E(r)exp(j t )} H(r, t) = Re{H(r)exp(j t)}, (5. 3 1) where E(r) and H(r) are the complex amplitudes of the electric and magnetic fields, Helmholtz Equation
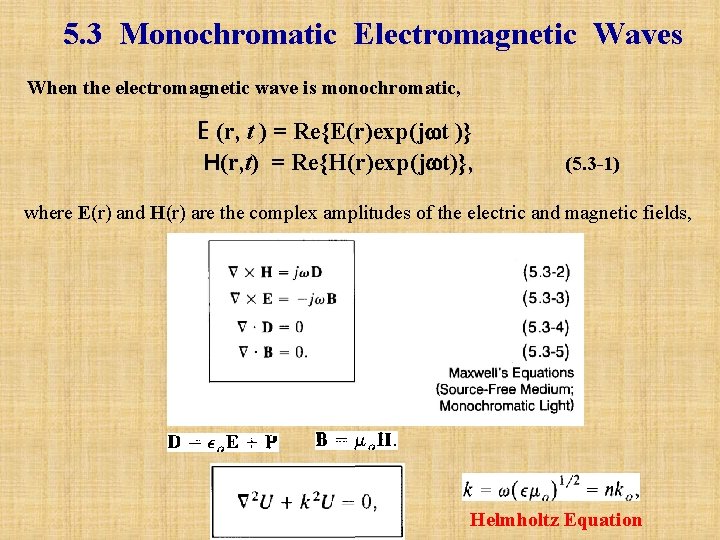
5. 3 Monochromatic Electromagnetic Waves When the electromagnetic wave is monochromatic, E (r, t ) = Re{E(r)exp(j t )} H(r, t) = Re{H(r)exp(j t)}, (5. 3 1) where E(r) and H(r) are the complex amplitudes of the electric and magnetic fields, Helmholtz Equation
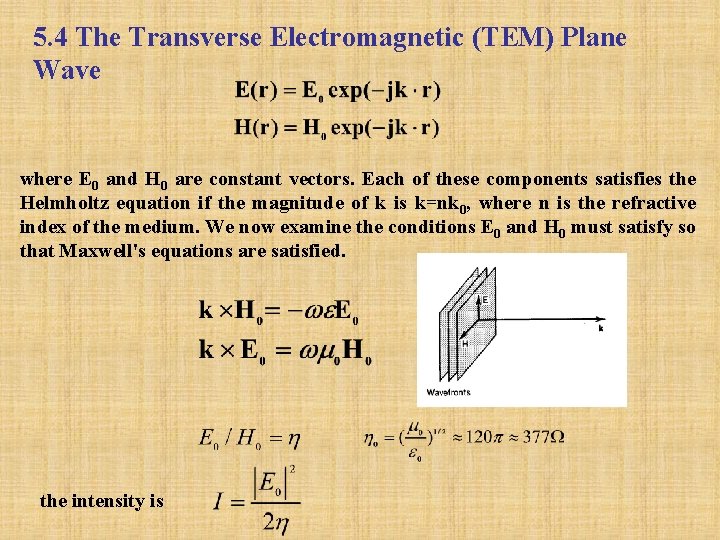
5. 4 The Transverse Electromagnetic (TEM) Plane Wave where E 0 and H 0 are constant vectors. Each of these components satisfies the Helmholtz equation if the magnitude of k is k=nk 0, where n is the refractive index of the medium. We now examine the conditions E 0 and H 0 must satisfy so that Maxwell's equations are satisfied. the intensity is
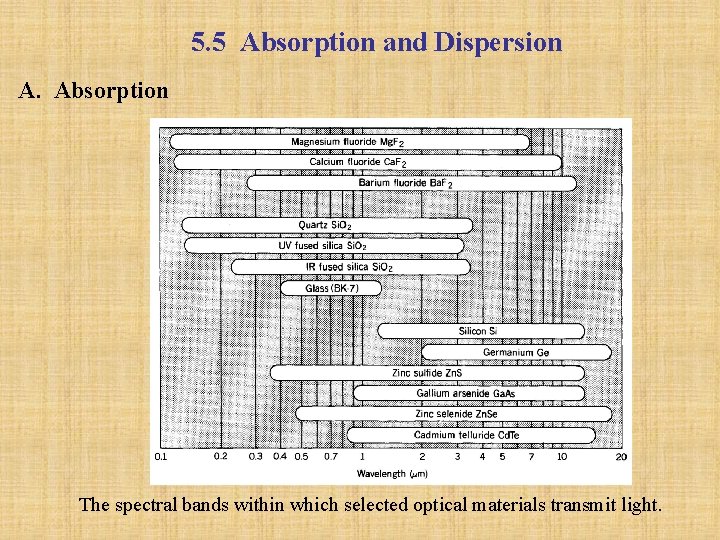
5. 5 Absorption and Dispersion A. Absorption The spectral bands within which selected optical materials transmit light.
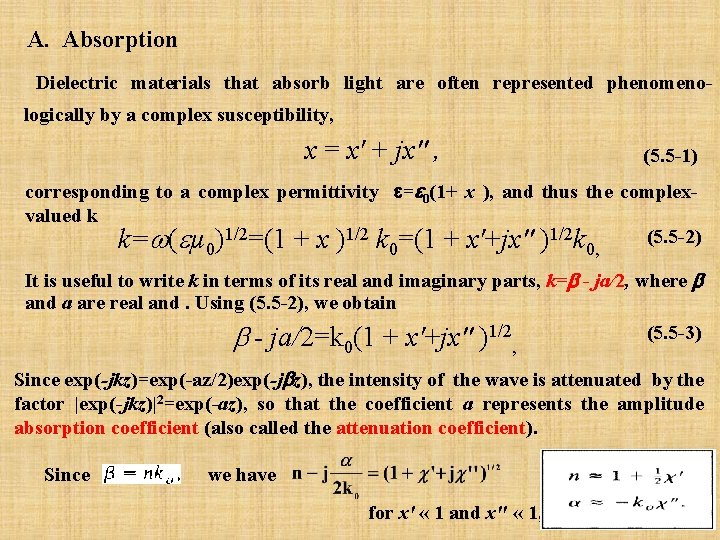
A. Absorption Dielectric materials that absorb light are often represented phenomeno logically by a complex susceptibility, x = x' + jx" , (5. 5 1) corresponding to a complex permittivity = 0(1+ x ), and thus the complex valued k (5. 5 2) k= ( µ )1/2=(1 + x )1/2 k =(1 + x'+jx" )1/2 k 0 0 0, It is useful to write k in terms of its real and imaginary parts, k= ja/2, where and a are real and. Using (5. 5 2), we obtain ja/2=k 0(1 + x'+jx" )1/2, (5. 5 3) Since exp(-jkz)=exp( az/2)exp(-j z), the intensity of the wave is attenuated by the factor |exp( jkz)|2=exp( az), so that the coefficient a represents the amplitude absorption coefficient (also called the attenuation coefficient). Since we have for x' « 1 and x" « 1,
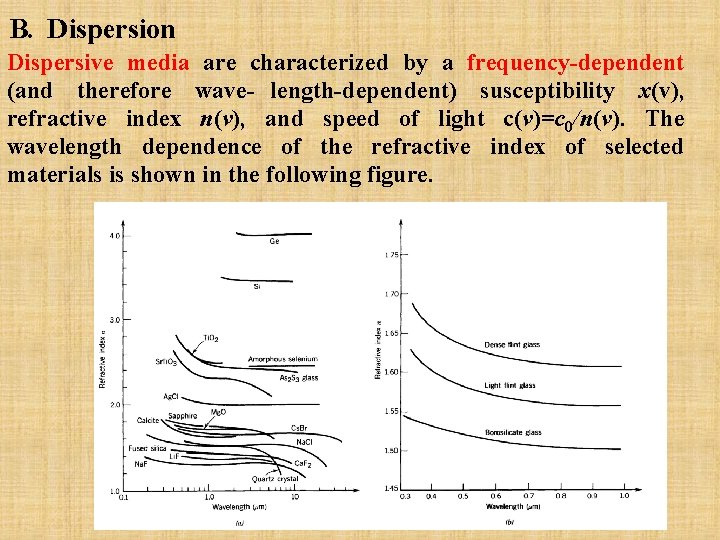
B. Dispersion Dispersive media are characterized by a frequency dependent (and therefore wave length dependent) susceptibility x(v), refractive index n(v), and speed of light c(v)=c 0/n(v). The wavelength dependence of the refractive index of selected materials is shown in the following figure.
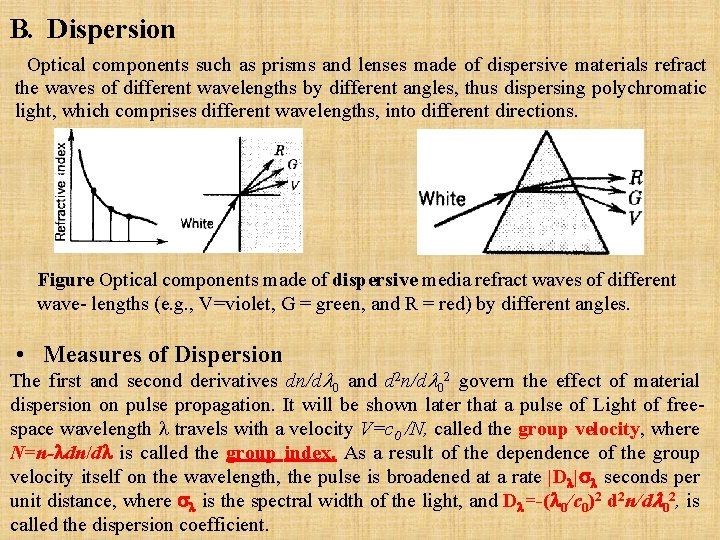
B. Dispersion Optical components such as prisms and lenses made of dispersive materials refract the waves of different wavelengths by different angles, thus dispersing polychromatic light, which comprises different wavelengths, into different directions. Figure Optical components made of dispersive media refract waves of different wave lengths (e. g. , V=violet, G = green, and R = red) by different angles. • Measures of Dispersion The first and second derivatives dn/d 0 and d 2 n/d 02 govern the effect of material dispersion on pulse propagation. It will be shown later that a pulse of Light of free space wavelength travels with a velocity V=c 0 /N, called the group velocity, where N=n- dn/d is called the group index. As a result of the dependence of the group velocity itself on the wavelength, the pulse is broadened at a rate |D | seconds per unit distance, where is the spectral width of the light, and D = ( 0/c 0)2 d 2 n/d 02, is called the dispersion coefficient.
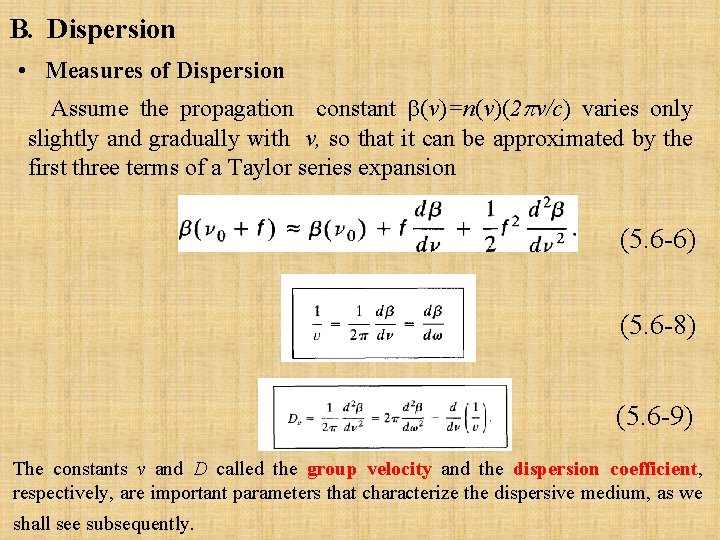
B. Dispersion • Measures of Dispersion Assume the propagation constant (v)=n(v)(2 v/c) varies only slightly and gradually with v, so that it can be approximated by the first three terms of a Taylor series expansion (5. 6 6) (5. 6 8) (5. 6 9) The constants v and D called the group velocity and the dispersion coefficient, respectively, are important parameters that characterize the dispersive medium, as we shall see subsequently.
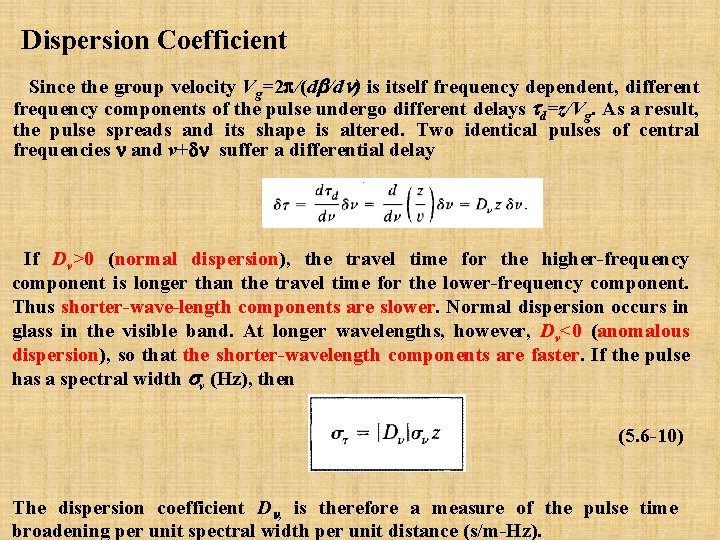
Dispersion Coefficient Since the group velocity Vg=2 /(d /d ) is itself frequency dependent, different frequency components of the pulse undergo different delays d=z/Vg. As a result, the pulse spreads and its shape is altered. Two identical pulses of central frequencies and v+ suffer a differential delay If Dv>0 (normal dispersion), the travel time for the higher frequency component is longer than the travel time for the lower frequency component. Thus shorter wave length components are slower. Normal dispersion occurs in glass in the visible band. At longer wavelengths, however, Dv<0 (anomalous dispersion), so that the shorter wavelength components are faster. If the pulse has a spectral width v (Hz), then (5. 6 10) The dispersion coefficient D , is therefore a measure of the pulse time broadening per unit spectral width per unit distance (s/m Hz).
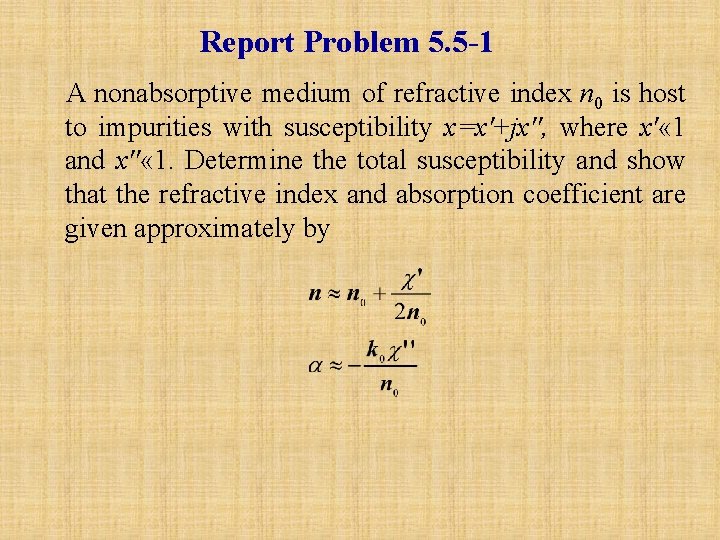
Report Problem 5. 5 1 A nonabsorptive medium of refractive index n 0 is host to impurities with susceptibility x=x'+jx'', where x' « 1 and x'' « 1. Determine the total susceptibility and show that the refractive index and absorption coefficient are given approximately by
Difference between ray optics and wave optics
Reflection and refraction venn diagram
Electromagnetic and mechanical waves
What are the mechanical waves
Longitudinal wave vs transverse wave
Orbital notation chlorine
Wave optics topics
Wave optics b.sc physics
Bill nye light energy
Light and optics notes
Bill nye reflection and refraction
Difference between luminous and non luminous
Into the light chapter 22
Chapter 22
Light light light chapter 23
Pie graph of electromagnetic waves
Em waves formulas
Em spectrum frequency
Intensity of electromagnetic wave
Radio waves, microwaves song lyrics
Intensity waves