Test of Variation in mpme using 40 Ca
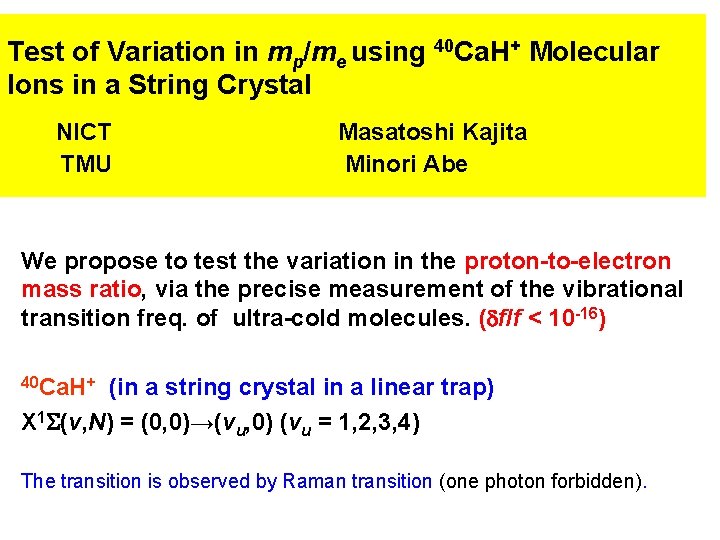
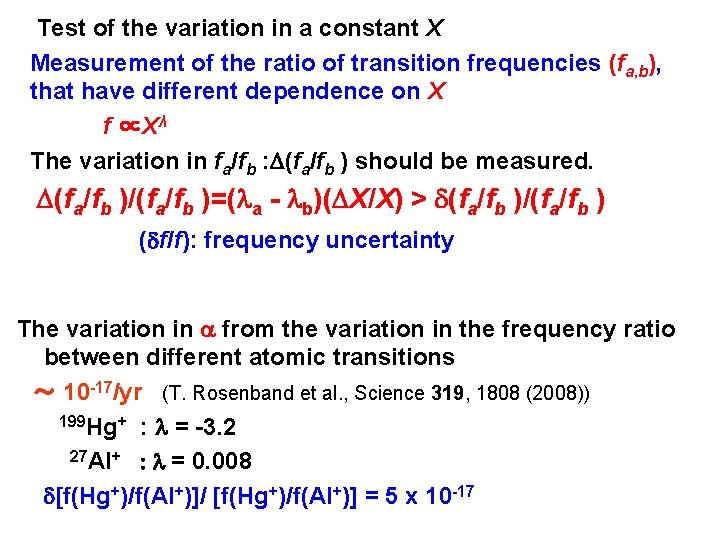
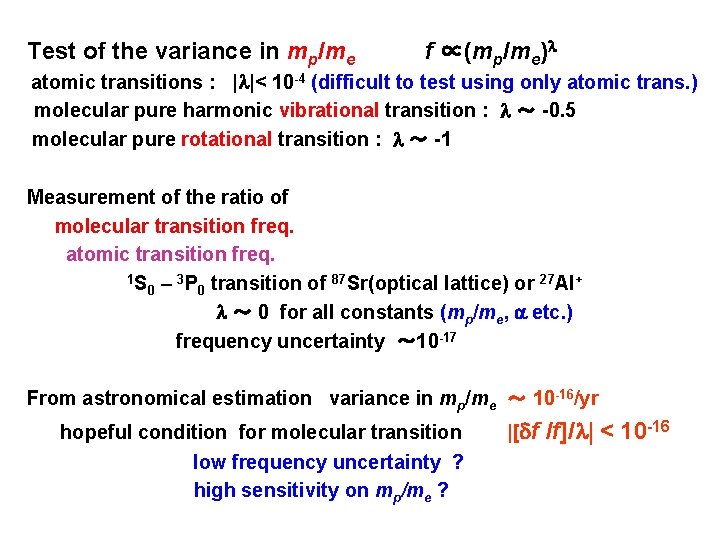
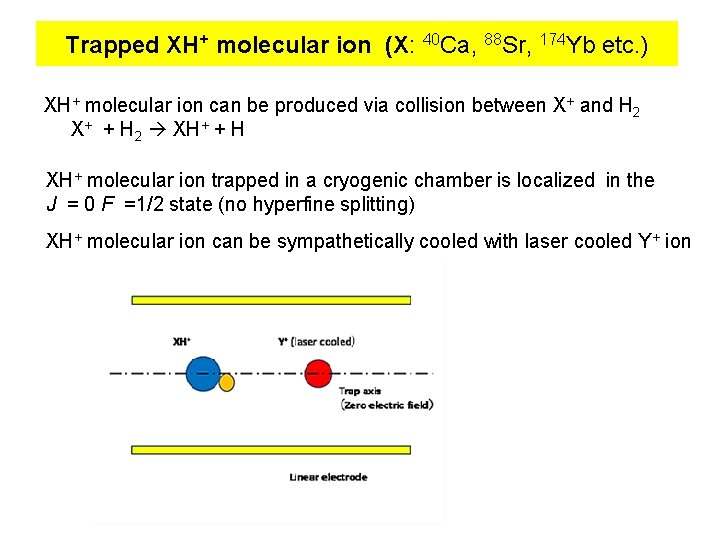
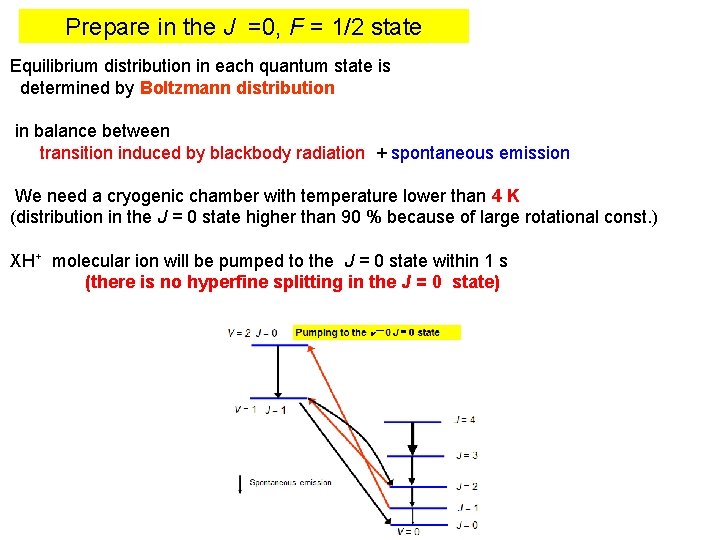
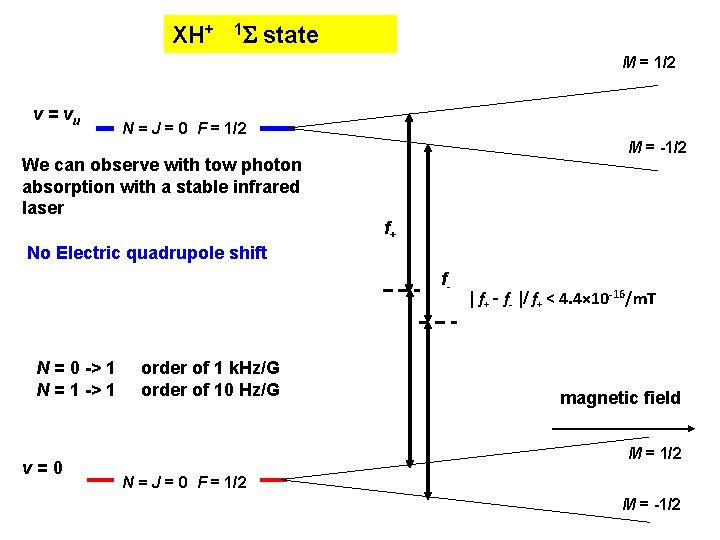
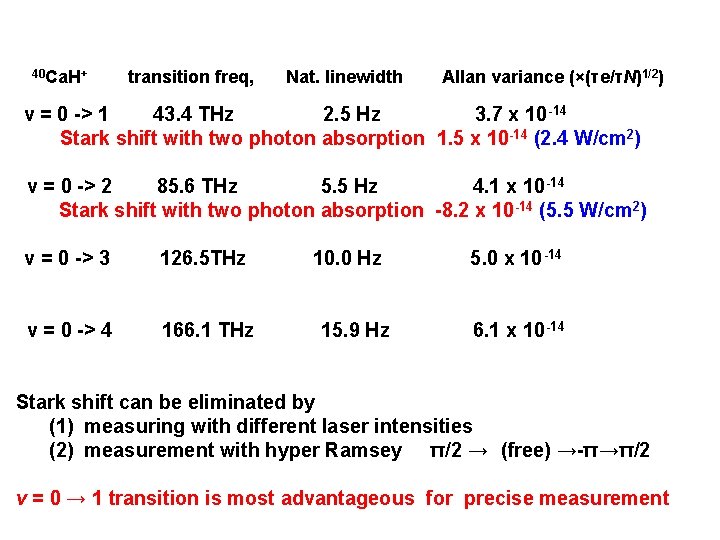
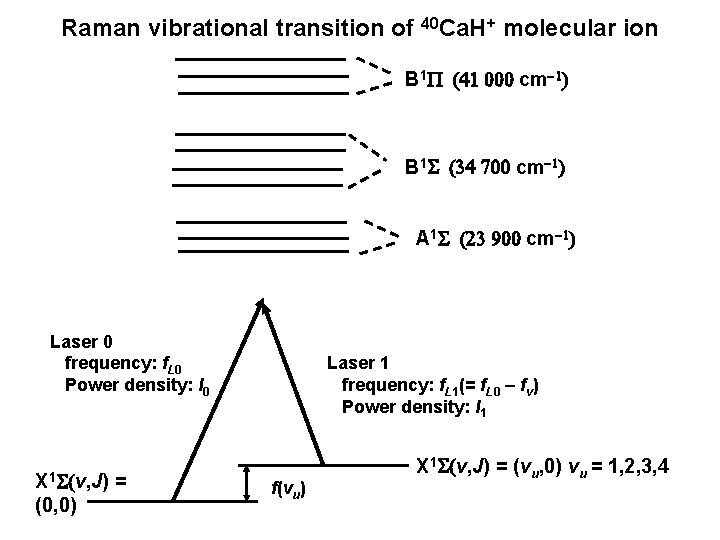
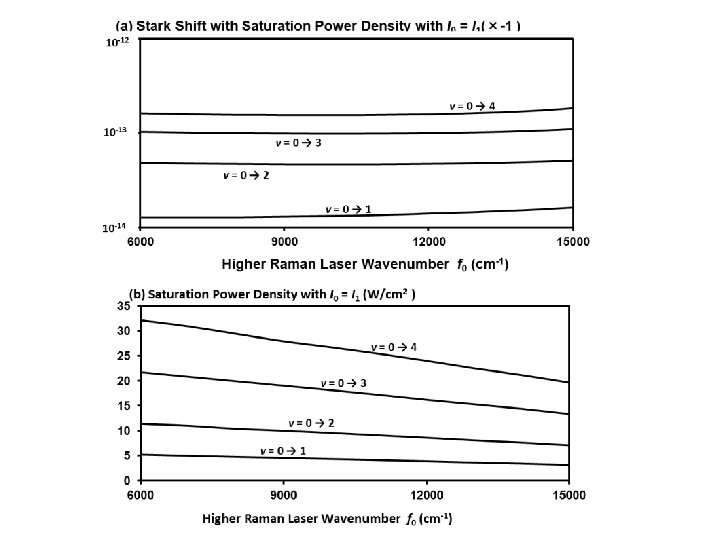
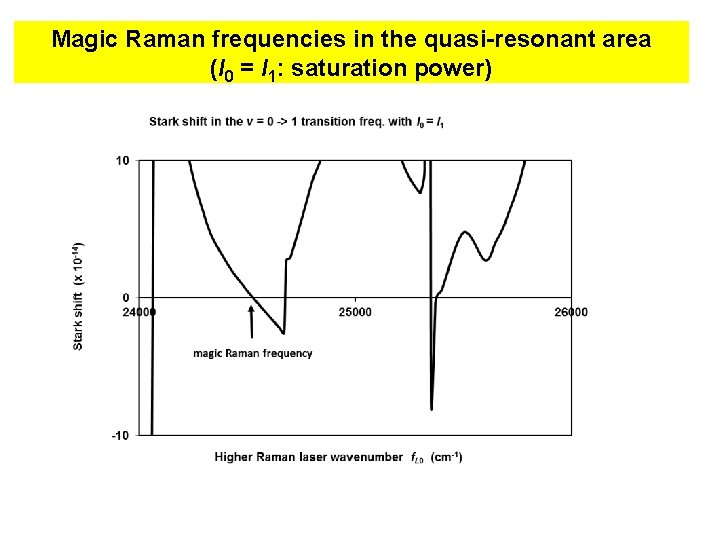
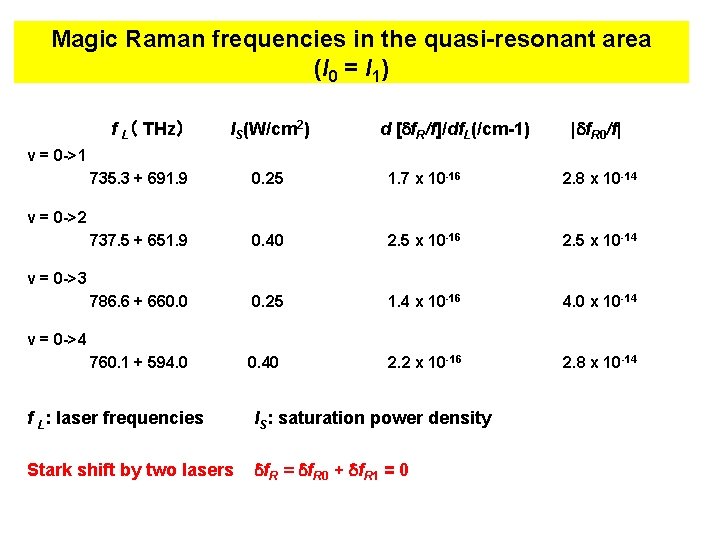
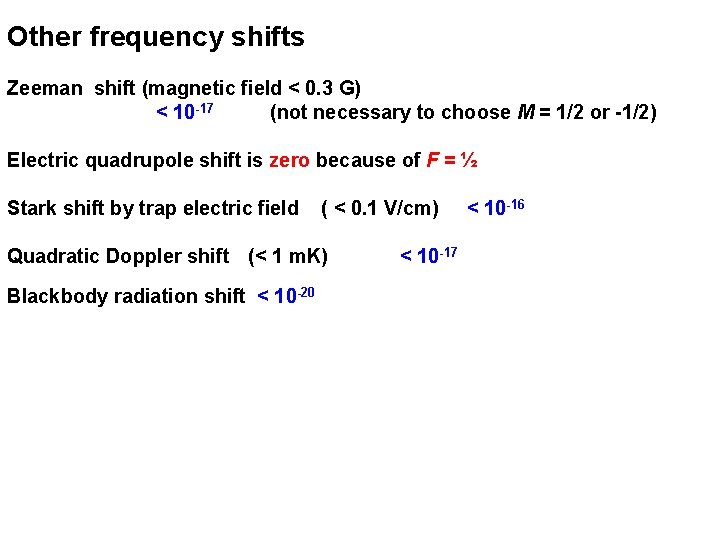
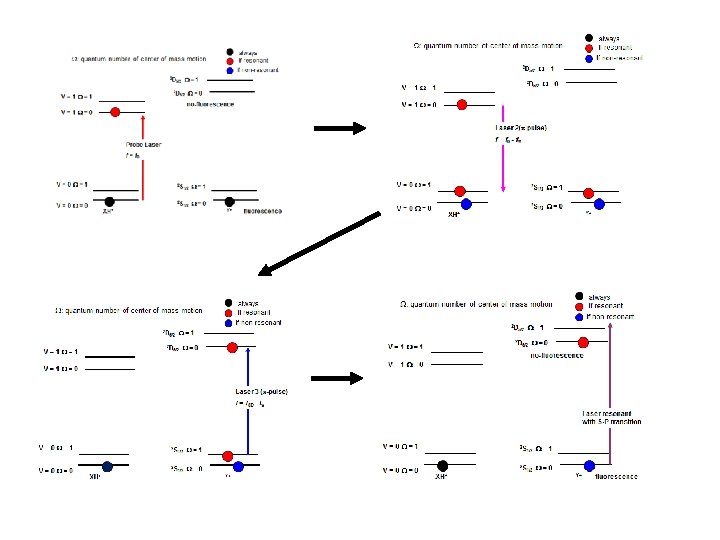
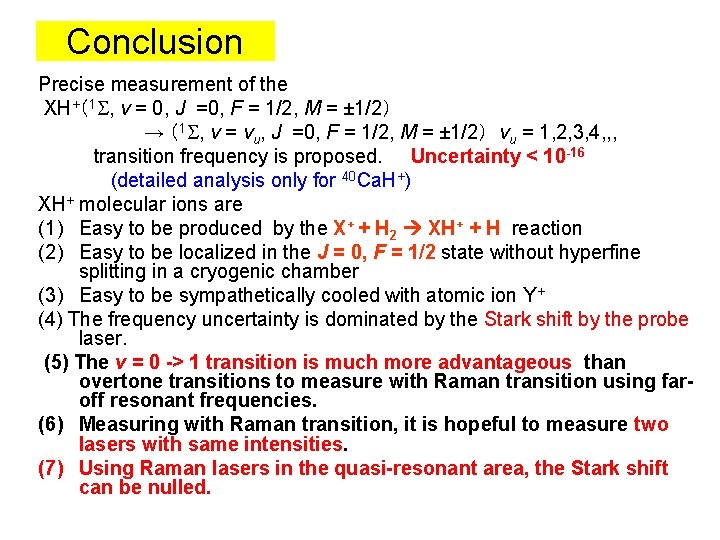
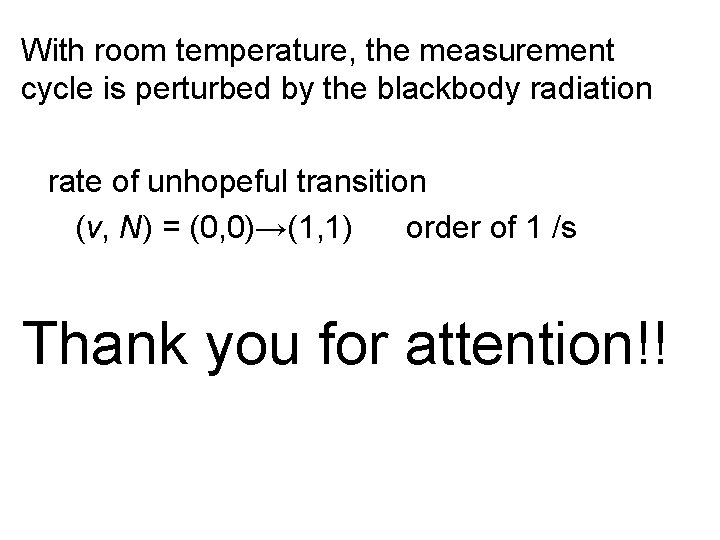
- Slides: 15
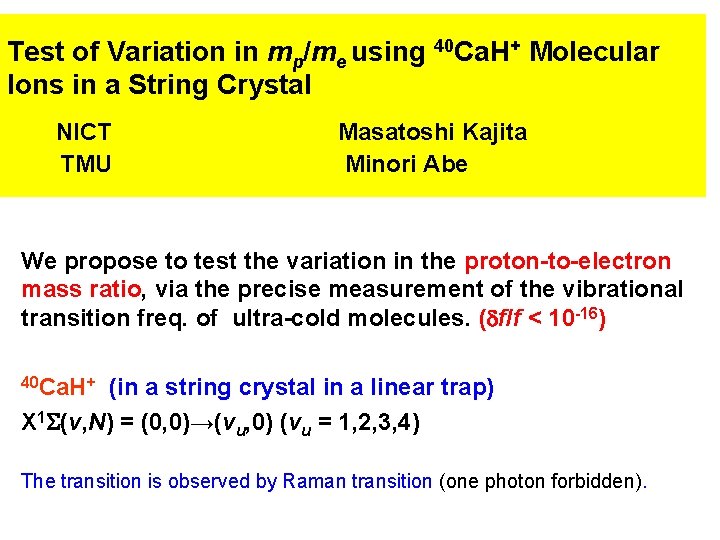
Test of Variation in mp/me using 40 Ca. H+ Molecular Ions in a String Crystal NICT TMU Masatoshi Kajita Minori Abe We propose to test the variation in the proton-to-electron mass ratio, via the precise measurement of the vibrational transition freq. of ultra-cold molecules. (df/f < 10 -16) 40 Ca. H+ (in a string crystal in a linear trap) X 1 S(v, N) = (0, 0)→(vu, 0) (vu = 1, 2, 3, 4) The transition is observed by Raman transition (one photon forbidden).
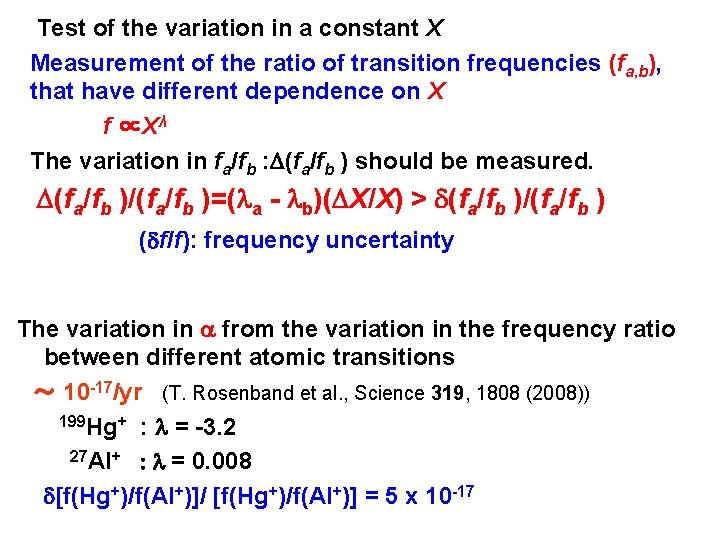
Test of the variation in a constant X Measurement of the ratio of transition frequencies (fa, b), that have different dependence on X f ∝Xλ The variation in fa/fb : D(fa/fb ) should be measured. D(fa/fb )/(fa/fb )=(la - lb)(DX/X) > d(fa/fb )/(fa/fb ) (df/f): frequency uncertainty The variation in a from the variation in the frequency ratio between different atomic transitions ~ 10 -17/yr (T. Rosenband et al. , Science 319, 1808 (2008)) 199 Hg+ : l = -3. 2 27 Al+ : l = 0. 008 d[f(Hg+)/f(Al+)]/ [f(Hg+)/f(Al+)] = 5 x 10 -17
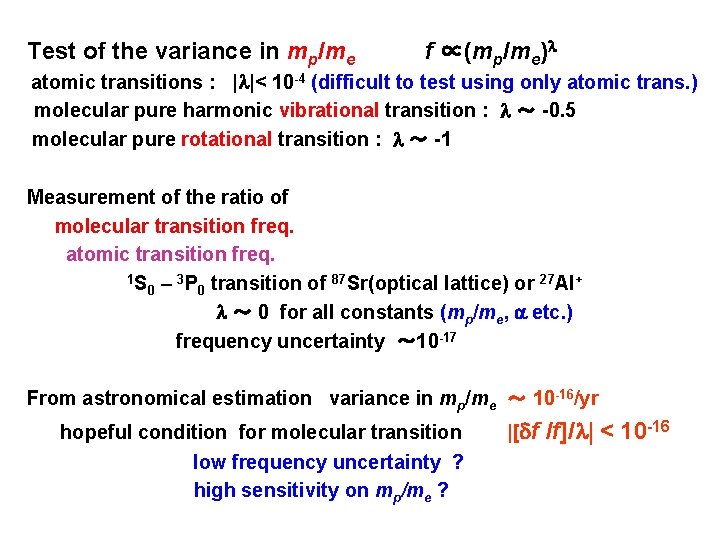
Test of the variance in mp/me f ∝(mp/me)l atomic transitions : |l|< 10 -4 (difficult to test using only atomic trans. ) molecular pure harmonic vibrational transition : l ~ -0. 5 molecular pure rotational transition : l ~ -1 Measurement of the ratio of molecular transition freq. atomic transition freq. 1 S – 3 P transition of 87 Sr(optical lattice) or 27 Al+ 0 0 l ~ 0 for all constants (mp/me, a etc. ) frequency uncertainty ~ 10 -17 From astronomical estimation variance in mp/me ~ 10 -16/yr hopeful condition for molecular transition low frequency uncertainty ? high sensitivity on mp/me ? |[df /f]/l| < 10 -16
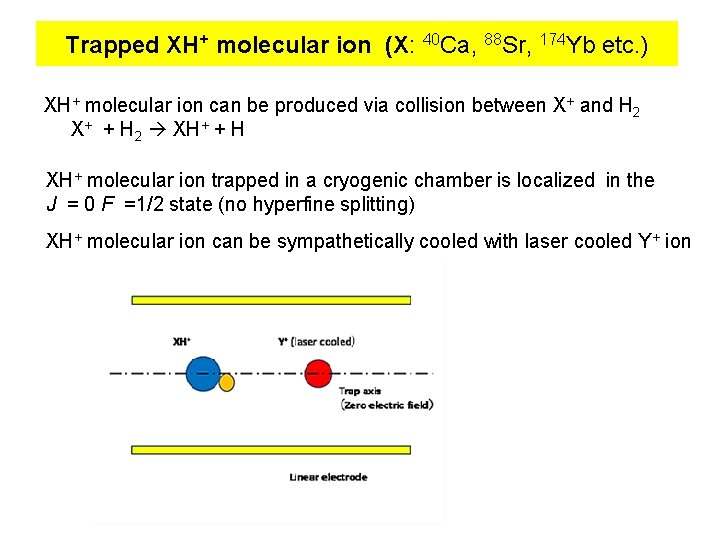
Trapped XH+ molecular ion (X: 40 Ca, 88 Sr, 174 Yb etc. ) XH+ molecular ion can be produced via collision between X+ and H 2 X+ + H 2 XH+ + H XH+ molecular ion trapped in a cryogenic chamber is localized in the J = 0 F =1/2 state (no hyperfine splitting) XH+ molecular ion can be sympathetically cooled with laser cooled Y+ ion
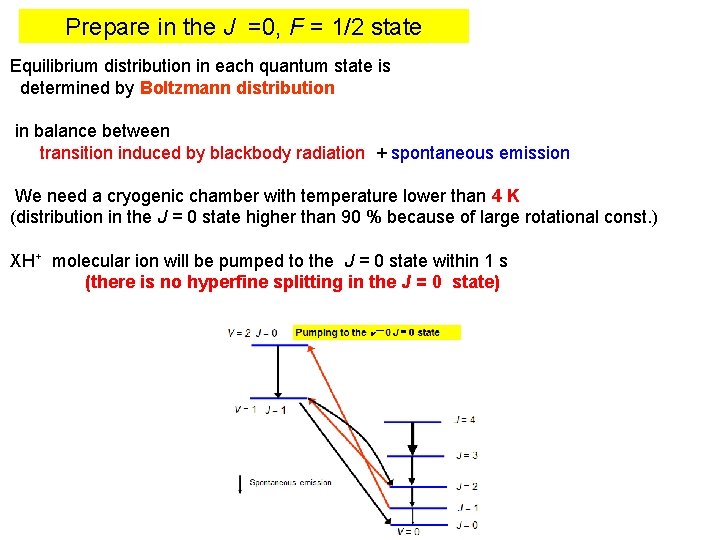
Prepare in the J =0, F = 1/2 state Equilibrium distribution in each quantum state is determined by Boltzmann distribution in balance between transition induced by blackbody radiation + spontaneous emission We need a cryogenic chamber with temperature lower than 4 K (distribution in the J = 0 state higher than 90 % because of large rotational const. ) XH+ molecular ion will be pumped to the J = 0 state within 1 s (there is no hyperfine splitting in the J = 0 state)
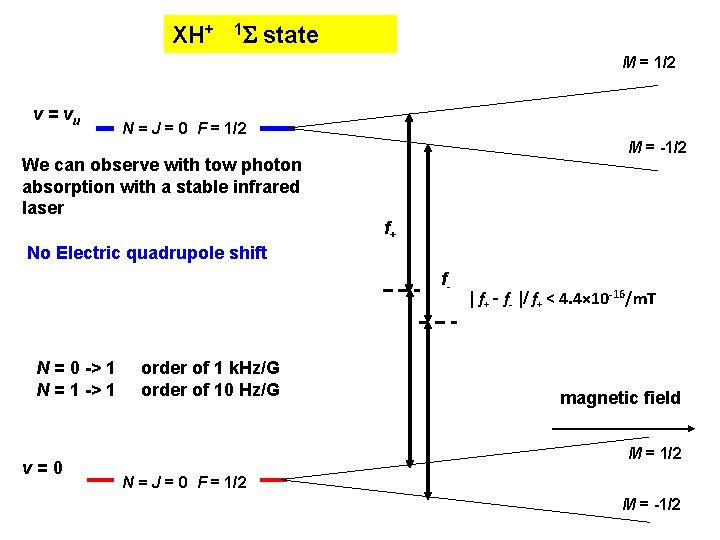
XH+ 1 S state M = 1/2 v = vu N = J = 0 F = 1/2 M = -1/2 We can observe with tow photon absorption with a stable infrared laser f+ No Electric quadrupole shift f- N = 0 -> 1 N = 1 -> 1 v=0 order of 1 k. Hz/G order of 10 Hz/G | f+ - f- |/ f+ < 4. 4× 10 -16/m. T magnetic field M = 1/2 N = J = 0 F = 1/2 M = -1/2
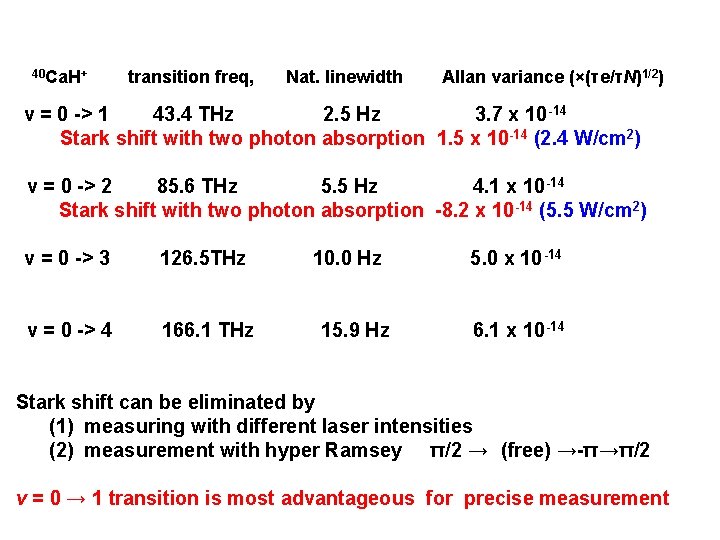
40 Ca. H+ transition freq, Nat. linewidth Allan variance (×(τe/τN)1/2) v = 0 -> 1 43. 4 THz 2. 5 Hz 3. 7 x 10 -14 Stark shift with two photon absorption 1. 5 x 10 -14 (2. 4 W/cm 2) v = 0 -> 2 85. 6 THz 5. 5 Hz 4. 1 x 10 -14 Stark shift with two photon absorption -8. 2 x 10 -14 (5. 5 W/cm 2) v = 0 -> 3 126. 5 THz v = 0 -> 4 166. 1 THz 10. 0 Hz 15. 9 Hz 5. 0 x 10 -14 6. 1 x 10 -14 Stark shift can be eliminated by (1) measuring with different laser intensities (2) measurement with hyper Ramsey π/2 → (free) →-π→π/2 v = 0 → 1 transition is most advantageous for precise measurement
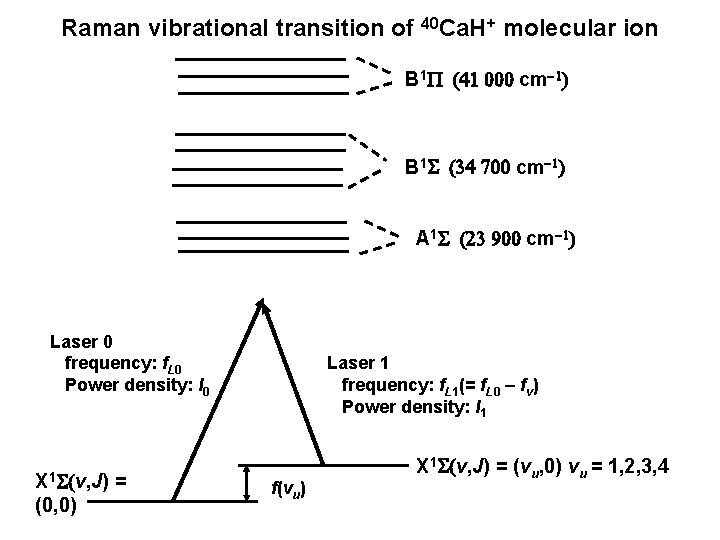
Raman vibrational transition of 40 Ca. H+ molecular ion B 1 P (41 000 cm-1) B 1 S (34 700 cm-1) A 1 S (23 900 cm-1) Laser 0 frequency: f. L 0 Power density: I 0 X 1 S(v, J) = (0, 0) Laser 1 frequency: f. L 1(= f. L 0 – fv) Power density: I 1 f(vu) X 1 S(v, J) = (vu, 0) vu = 1, 2, 3, 4
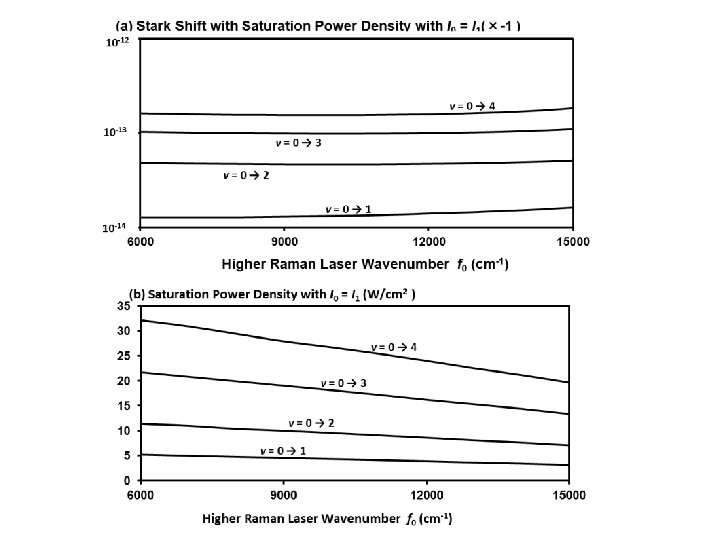
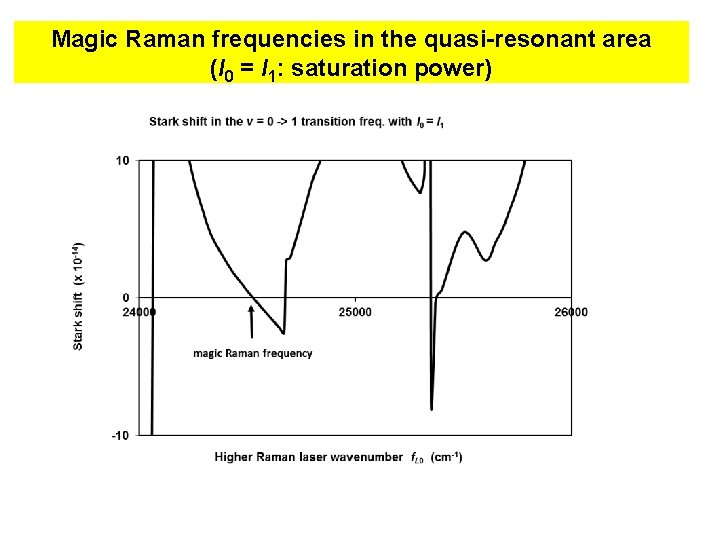
Magic Raman frequencies in the quasi-resonant area (I 0 = I 1: saturation power)
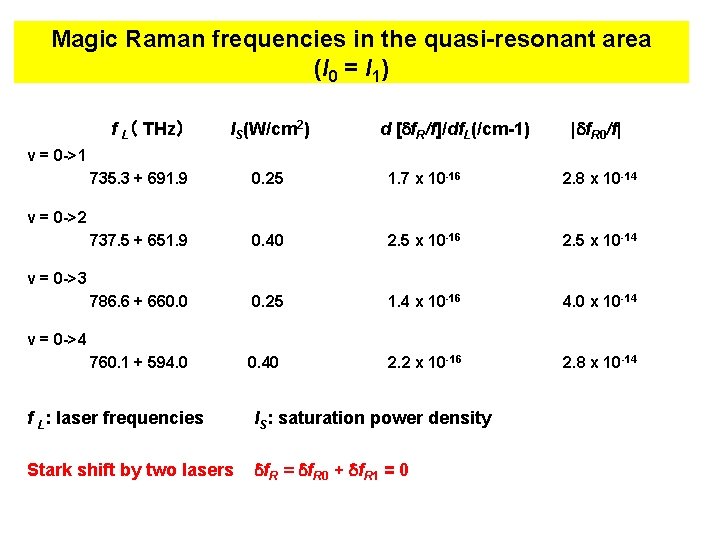
Magic Raman frequencies in the quasi-resonant area (I 0 = I 1) f L( THz) IS(W/cm 2) d [df. R/f]/df. L(/cm-1) |df. R 0/f| v = 0 ->1 735. 3 + 691. 9 0. 25 1. 7 x 10 -16 2. 8 x 10 -14 737. 5 + 651. 9 0. 40 2. 5 x 10 -16 2. 5 x 10 -14 786. 6 + 660. 0 0. 25 1. 4 x 10 -16 4. 0 x 10 -14 760. 1 + 594. 0 0. 40 2. 2 x 10 -16 2. 8 x 10 -14 v = 0 ->2 v = 0 ->3 v = 0 ->4 f L: laser frequencies IS: saturation power density Stark shift by two lasers df. R = df. R 0 + df. R 1 = 0
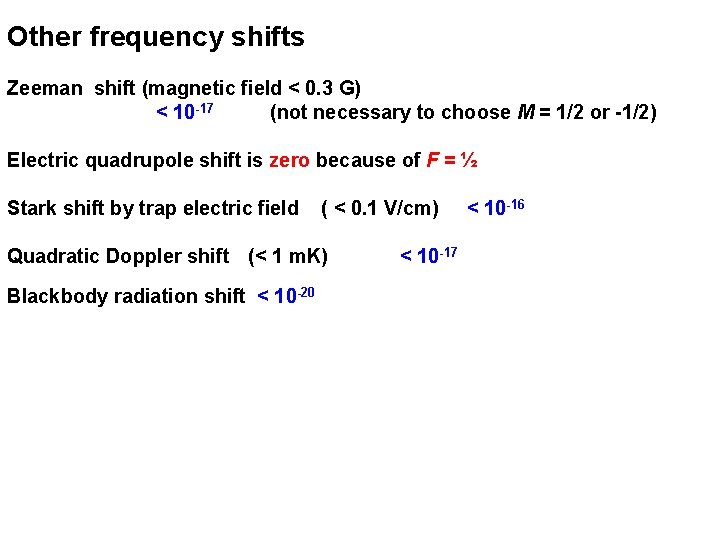
Other frequency shifts Zeeman shift (magnetic field < 0. 3 G) < 10 -17 (not necessary to choose M = 1/2 or -1/2) Electric quadrupole shift is zero because of F = ½ Stark shift by trap electric field ( < 0. 1 V/cm) Quadratic Doppler shift (< 1 m. K) Blackbody radiation shift < 10 -20 < 10 -17 < 10 -16
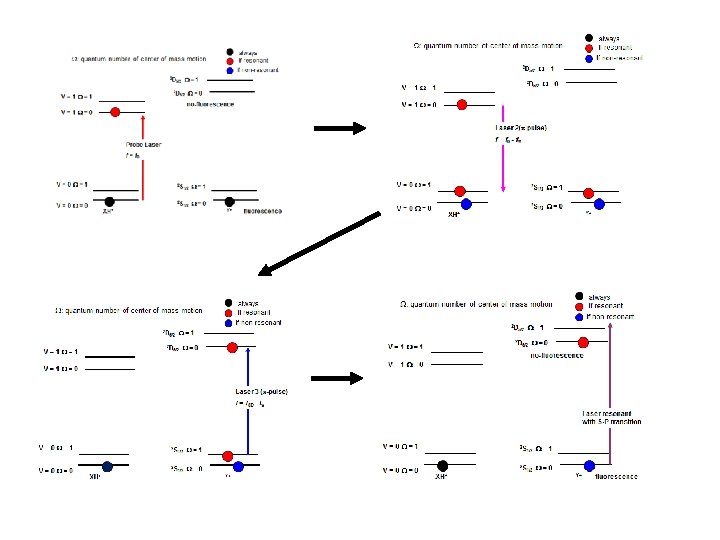
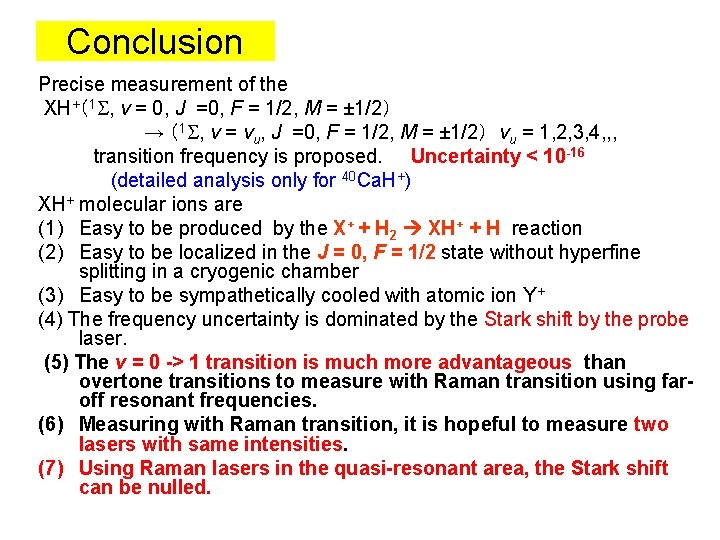
Conclusion Precise measurement of the XH+(1 S, v = 0, J =0, F = 1/2, M = ± 1/2) → (1 S, v = vu, J =0, F = 1/2, M = ± 1/2) vu = 1, 2, 3, 4, , , transition frequency is proposed. Uncertainty < 10 -16 (detailed analysis only for 40 Ca. H+) XH+ molecular ions are (1) Easy to be produced by the X+ + H 2 XH+ + H reaction (2) Easy to be localized in the J = 0, F = 1/2 state without hyperfine splitting in a cryogenic chamber (3) Easy to be sympathetically cooled with atomic ion Y+ (4) The frequency uncertainty is dominated by the Stark shift by the probe laser. (5) The v = 0 -> 1 transition is much more advantageous than overtone transitions to measure with Raman transition using faroff resonant frequencies. (6) Measuring with Raman transition, it is hopeful to measure two lasers with same intensities. (7) Using Raman lasers in the quasi-resonant area, the Stark shift can be nulled.
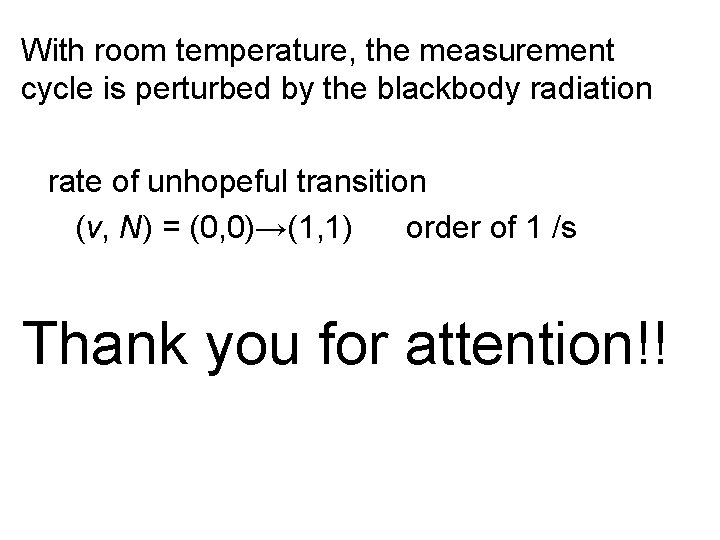
With room temperature, the measurement cycle is perturbed by the blackbody radiation rate of unhopeful transition (v, N) = (0, 0)→(1, 1) order of 1 /s Thank you for attention!!
Constant of variation
Examples of direct variation graphs
How to calculate sst in regression
Modeling using variation
Using system using system.collections.generic
Unit 25 special refrigeration system components
Chapter 20:11 using reagent strips to test urine
Test blueprint template for nursing
Variation of light
Equation of direct variation
Kinds of variation
Secular trend in time series
How to calculate average seasonal variation
Which of the following functions is a direct variation
Glidant excipient pricelist
What is sociolinguistics