Resonant Raman Measurements of an Organic Semiconducting Single
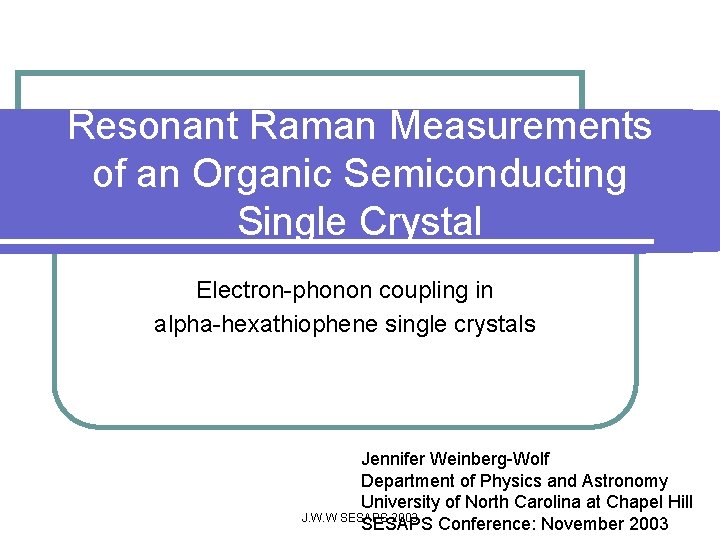
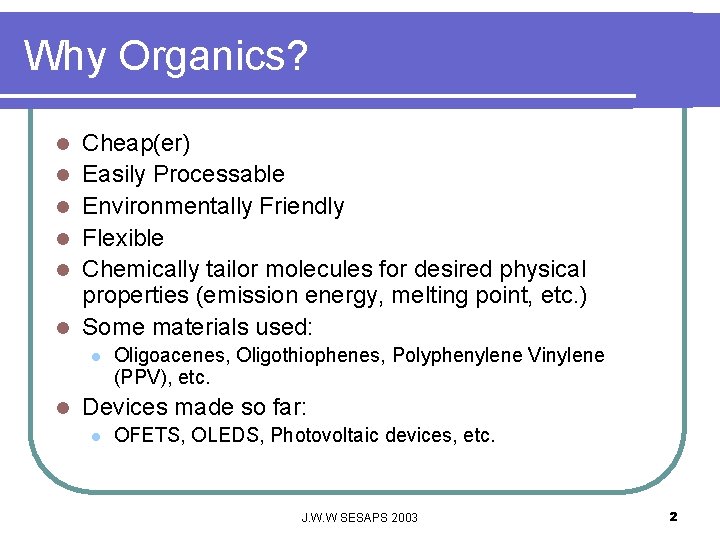
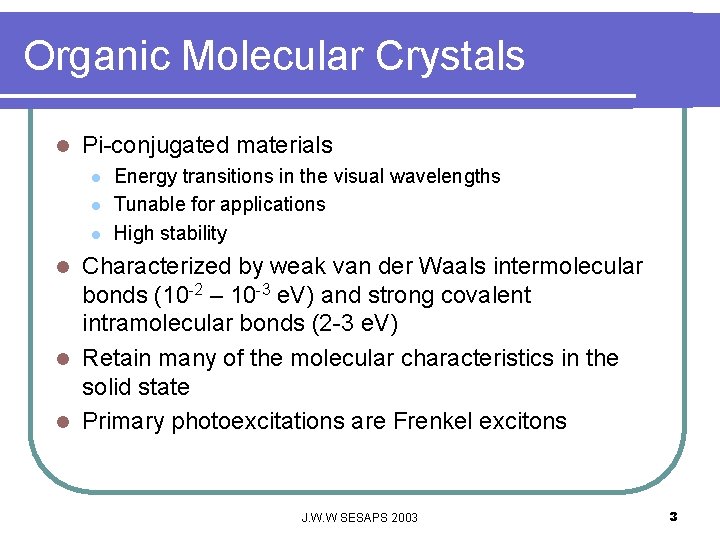
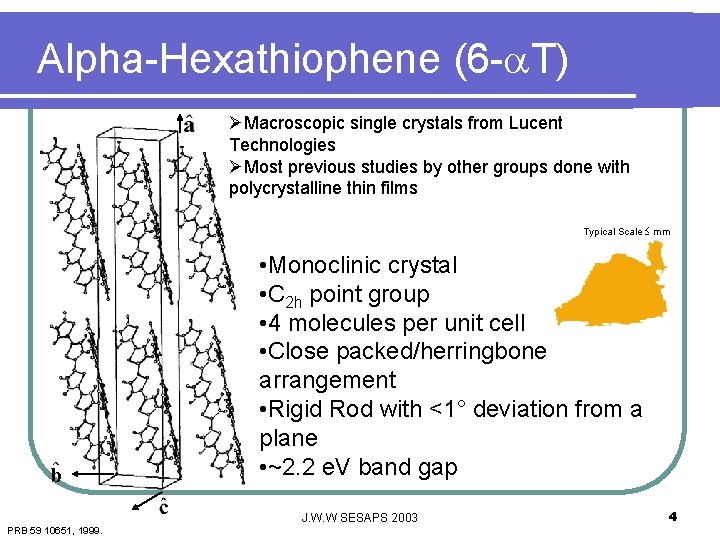
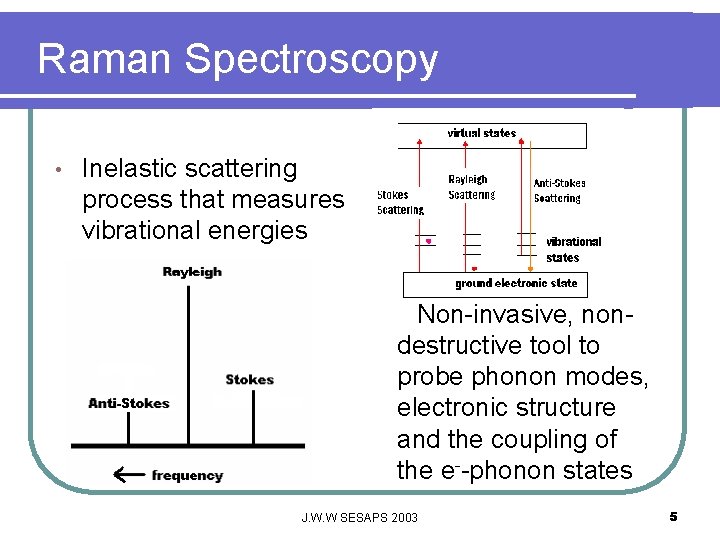
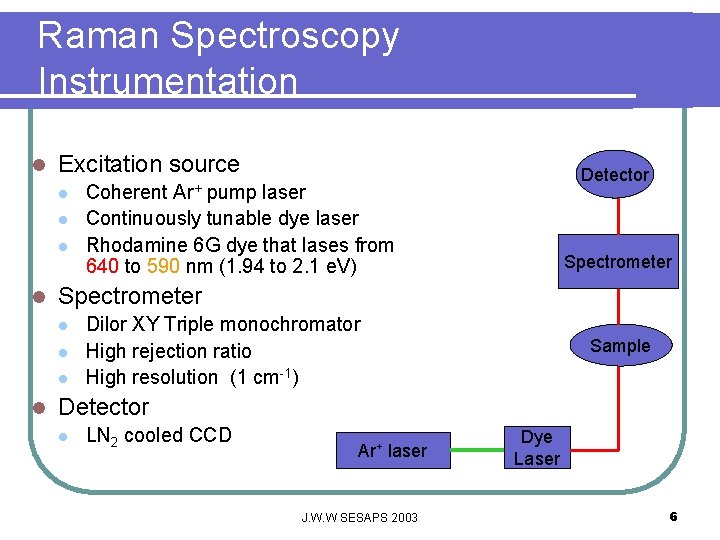
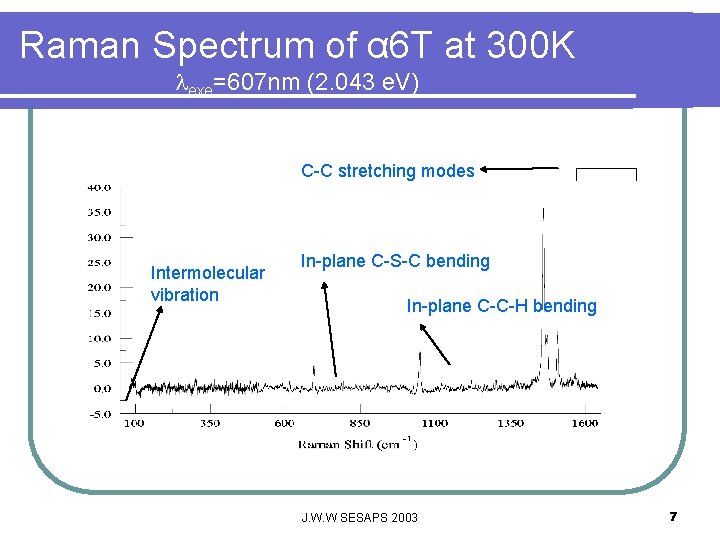
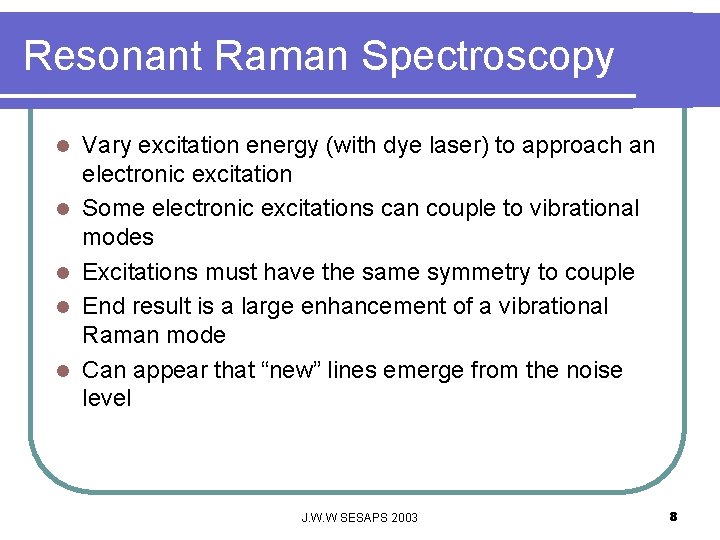
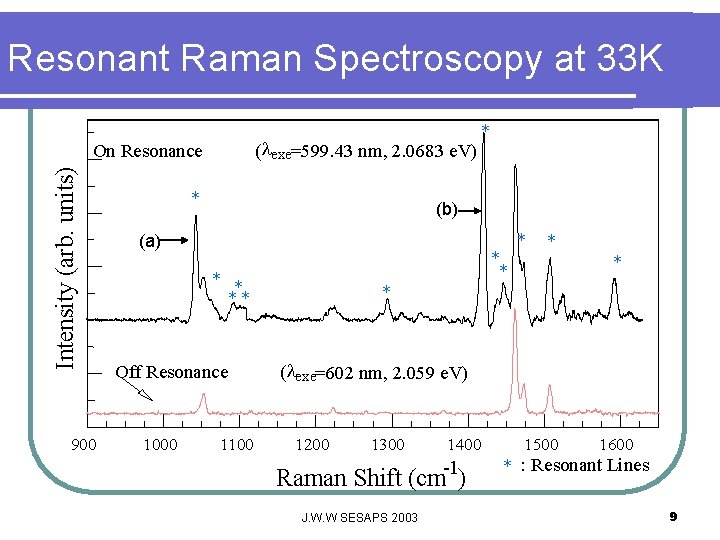
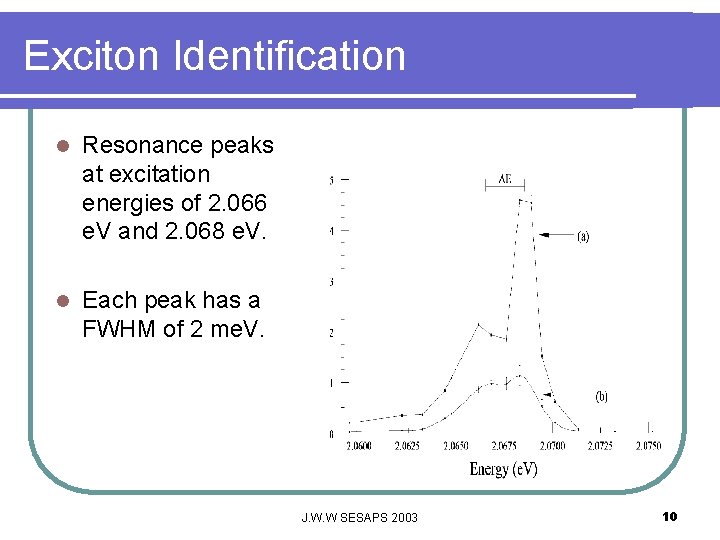
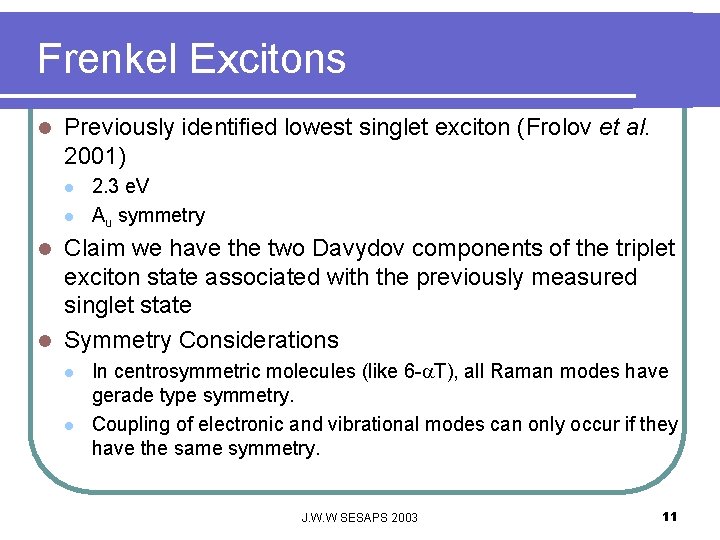
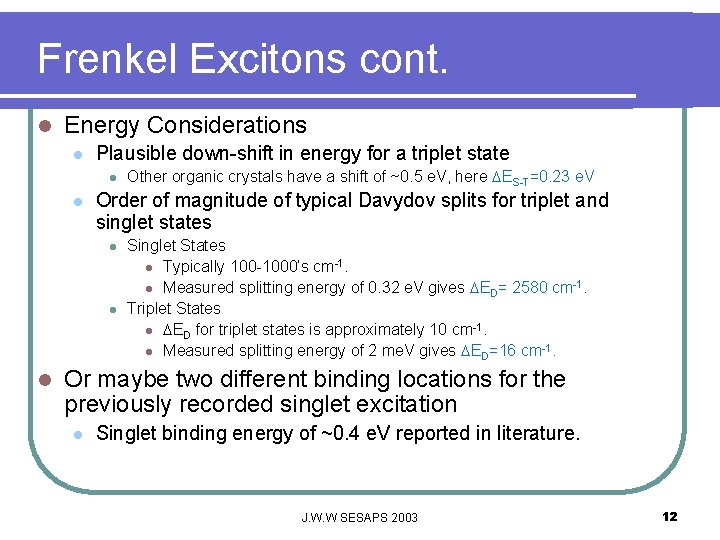
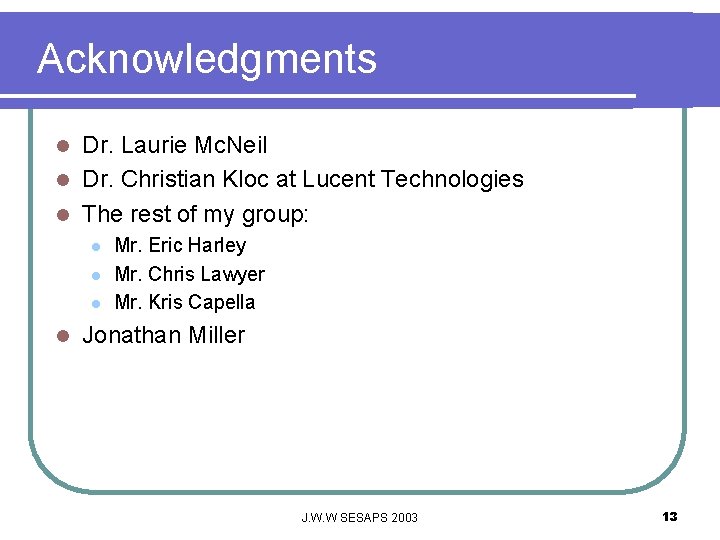
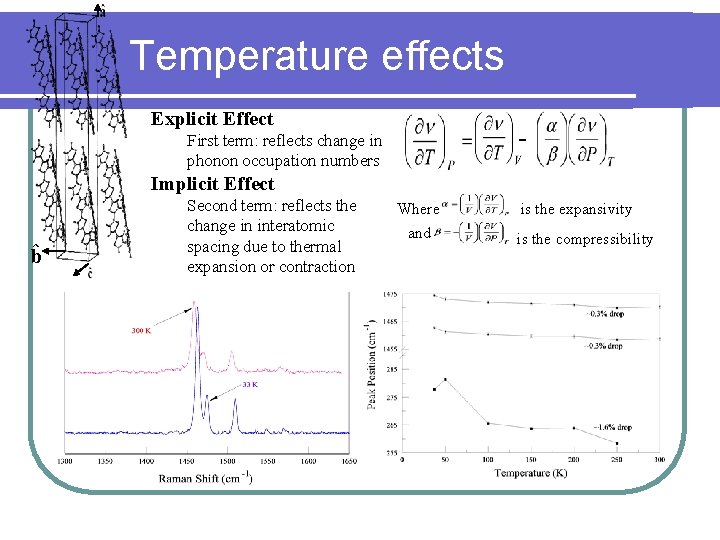
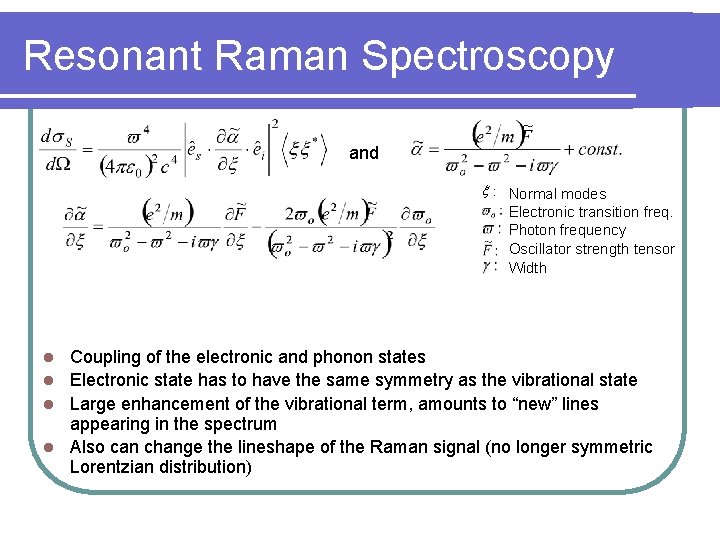
- Slides: 15
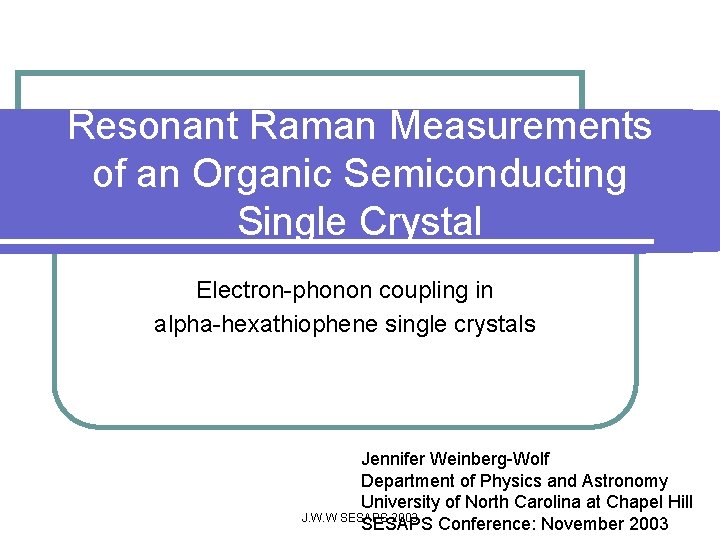
Resonant Raman Measurements of an Organic Semiconducting Single Crystal Electron-phonon coupling in alpha-hexathiophene single crystals Jennifer Weinberg-Wolf Department of Physics and Astronomy University of North Carolina at Chapel Hill J. W. W SESAPS 2003 SESAPS Conference: November 2003
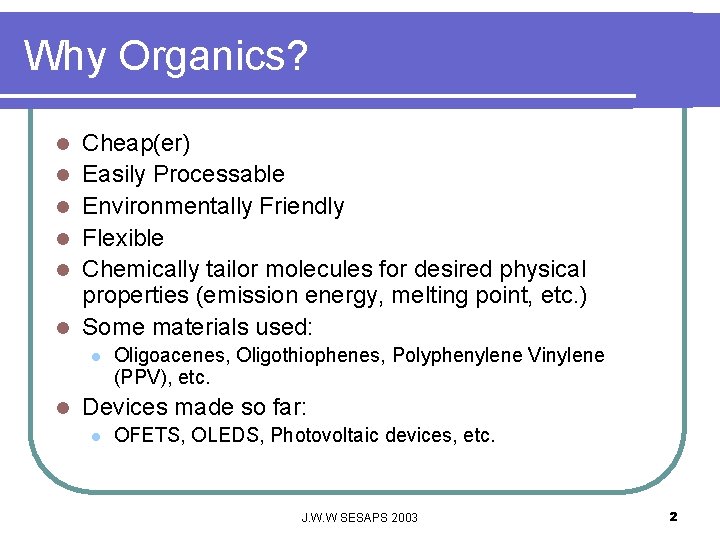
Why Organics? l l l Cheap(er) Easily Processable Environmentally Friendly Flexible Chemically tailor molecules for desired physical properties (emission energy, melting point, etc. ) Some materials used: l l Oligoacenes, Oligothiophenes, Polyphenylene Vinylene (PPV), etc. Devices made so far: l OFETS, OLEDS, Photovoltaic devices, etc. J. W. W SESAPS 2003 2
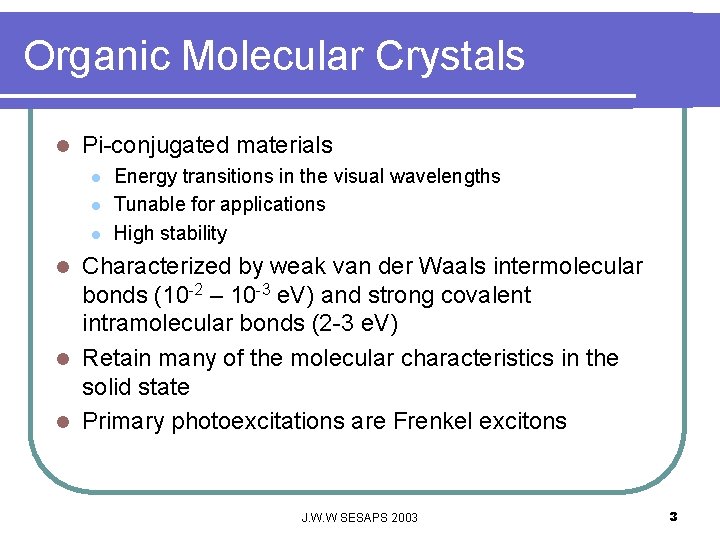
Organic Molecular Crystals l Pi-conjugated materials l l l Energy transitions in the visual wavelengths Tunable for applications High stability Characterized by weak van der Waals intermolecular bonds (10 -2 – 10 -3 e. V) and strong covalent intramolecular bonds (2 -3 e. V) l Retain many of the molecular characteristics in the solid state l Primary photoexcitations are Frenkel excitons l J. W. W SESAPS 2003 3
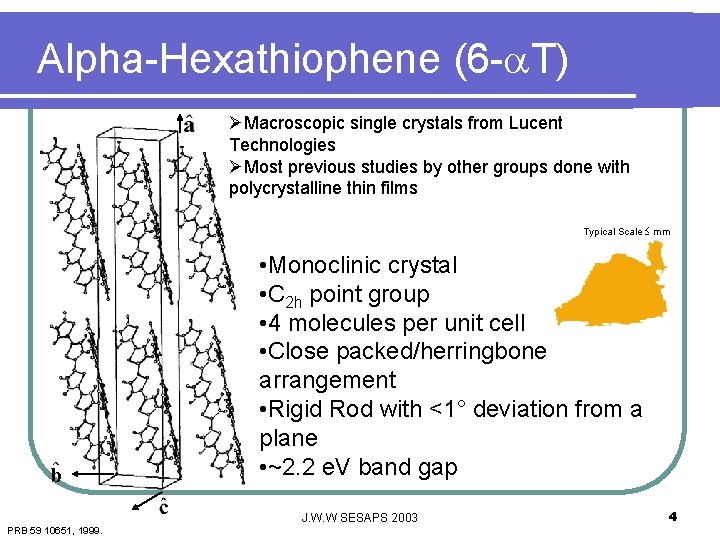
Alpha-Hexathiophene (6 -a. T) ØMacroscopic single crystals from Lucent Technologies ØMost previous studies by other groups done with polycrystalline thin films Typical Scale mm bˆ • Monoclinic crystal • C 2 h point group • 4 molecules per unit cell • Close packed/herringbone arrangement • Rigid Rod with <1° deviation from a plane • ~2. 2 e. V band gap J. W. W SESAPS 2003 PRB 59 10651, 1999. 4
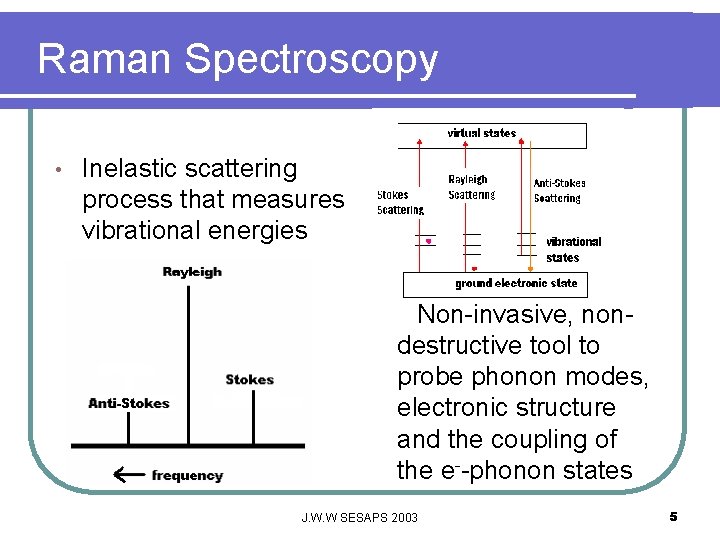
Raman Spectroscopy • Inelastic scattering process that measures vibrational energies Non-invasive, nondestructive tool to probe phonon modes, electronic structure and the coupling of the e--phonon states • J. W. W SESAPS 2003 5
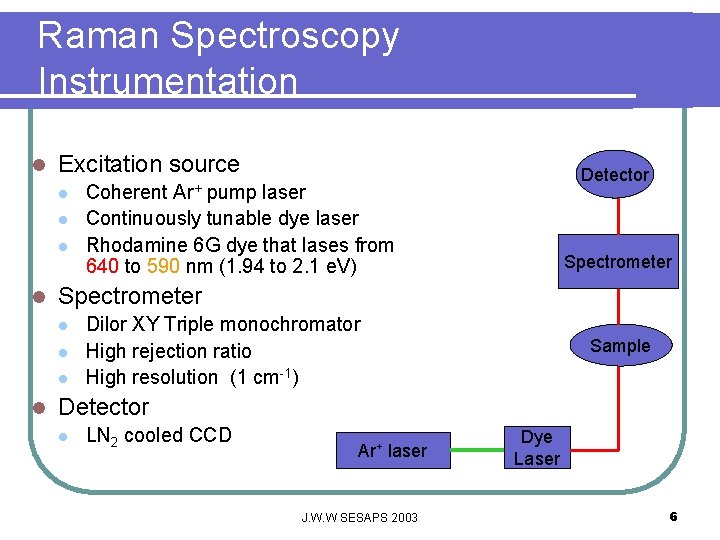
Raman Spectroscopy Instrumentation l Excitation source l l Coherent pump laser Continuously tunable dye laser Rhodamine 6 G dye that lases from 640 to 590 nm (1. 94 to 2. 1 e. V) Spectrometer l l Detector Ar+ Dilor XY Triple monochromator High rejection ratio High resolution (1 cm-1) Sample Detector l LN 2 cooled CCD Ar+ laser J. W. W SESAPS 2003 Dye Laser 6
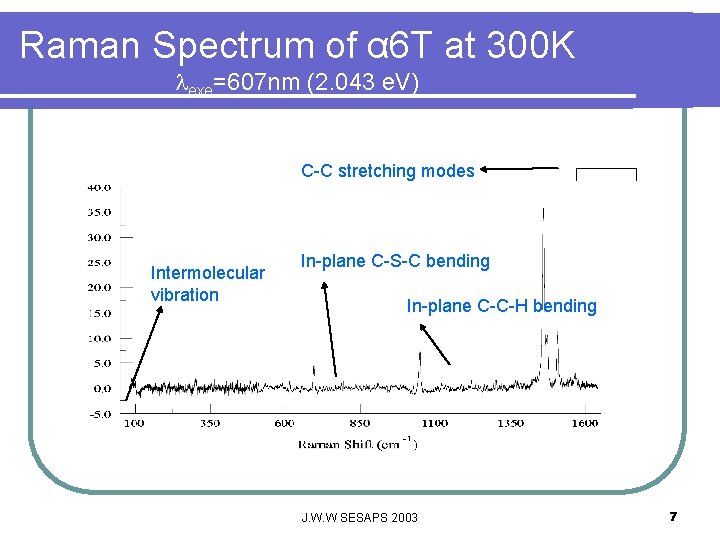
Raman Spectrum of α 6 T at 300 K lexe=607 nm (2. 043 e. V) C-C stretching modes Intermolecular vibration In-plane C-S-C bending In-plane C-C-H bending J. W. W SESAPS 2003 7
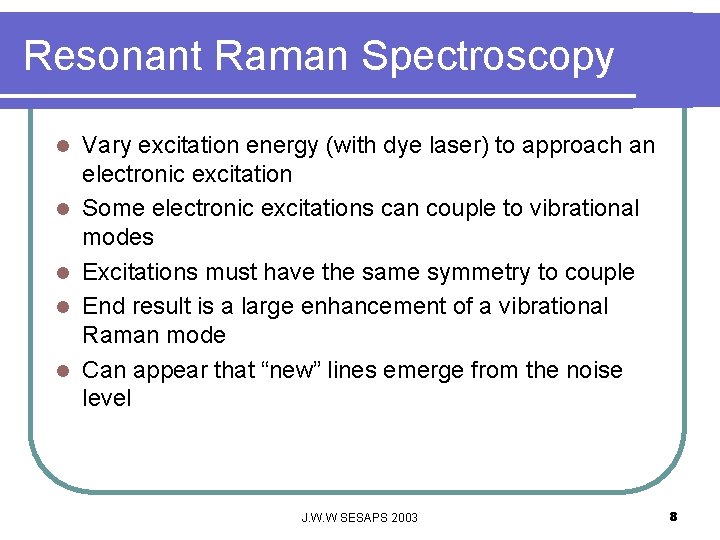
Resonant Raman Spectroscopy l l l Vary excitation energy (with dye laser) to approach an electronic excitation Some electronic excitations can couple to vibrational modes Excitations must have the same symmetry to couple End result is a large enhancement of a vibrational Raman mode Can appear that “new” lines emerge from the noise level J. W. W SESAPS 2003 8
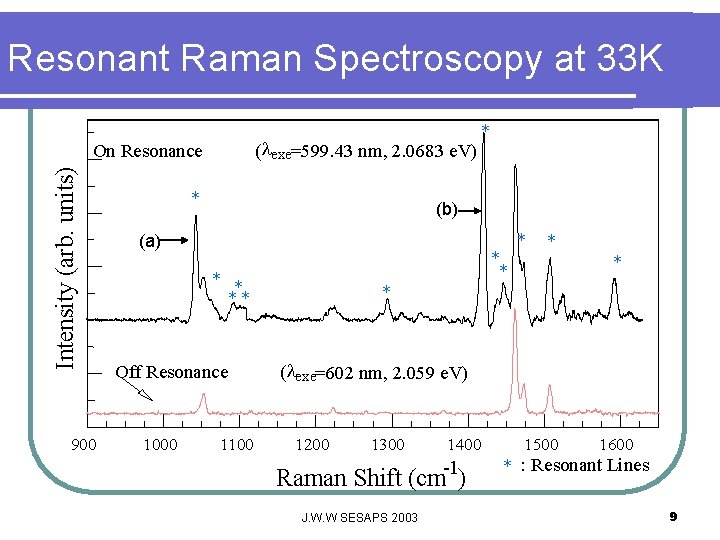
Resonant Raman Spectroscopy at 33 K (lexe=599. 43 nm, 2. 0683 e. V) Intensity (arb. units) On Resonance 900 * * (b) (a) * * ** Off Resonance 1000 1100 * * * (lexe=602 nm, 2. 059 e. V) 1200 1300 1400 -1 Raman Shift (cm ) J. W. W SESAPS 2003 1500 1600 * : Resonant Lines 9
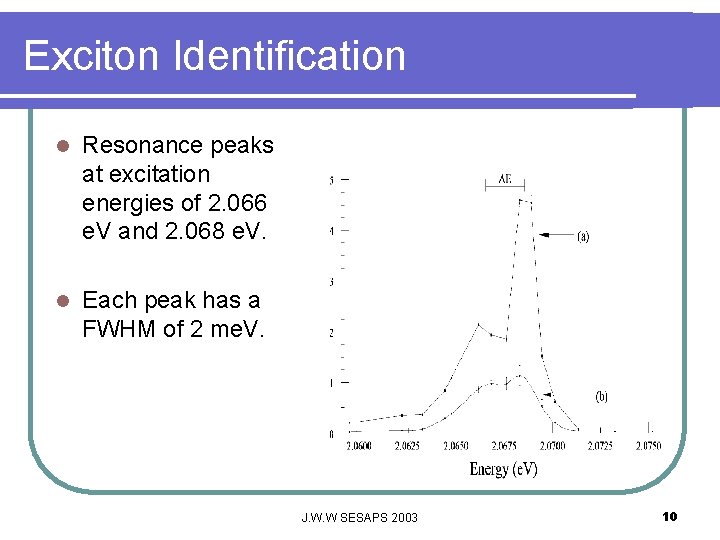
Exciton Identification l Resonance peaks at excitation energies of 2. 066 e. V and 2. 068 e. V. l Each peak has a FWHM of 2 me. V. J. W. W SESAPS 2003 10
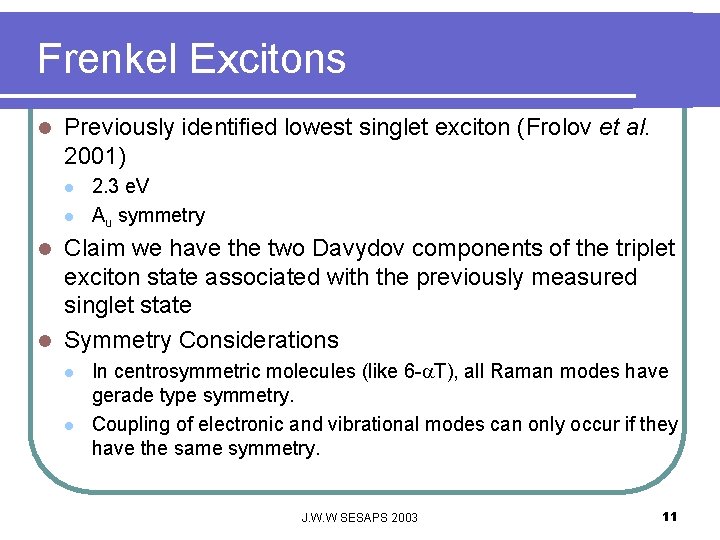
Frenkel Excitons l Previously identified lowest singlet exciton (Frolov et al. 2001) l l 2. 3 e. V Au symmetry Claim we have the two Davydov components of the triplet exciton state associated with the previously measured singlet state l Symmetry Considerations l l l In centrosymmetric molecules (like 6 -a. T), all Raman modes have gerade type symmetry. Coupling of electronic and vibrational modes can only occur if they have the same symmetry. J. W. W SESAPS 2003 11
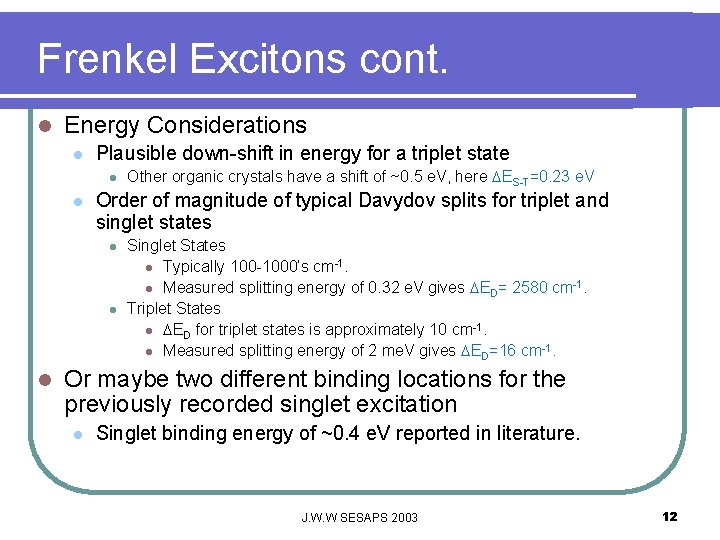
Frenkel Excitons cont. l Energy Considerations l Plausible down-shift in energy for a triplet state l l Order of magnitude of typical Davydov splits for triplet and singlet states l l l Other organic crystals have a shift of ~0. 5 e. V, here DES-T=0. 23 e. V Singlet States l Typically 100 -1000’s cm-1. l Measured splitting energy of 0. 32 e. V gives DED= 2580 cm-1. Triplet States l DED for triplet states is approximately 10 cm-1. l Measured splitting energy of 2 me. V gives DED=16 cm-1. Or maybe two different binding locations for the previously recorded singlet excitation l Singlet binding energy of ~0. 4 e. V reported in literature. J. W. W SESAPS 2003 12
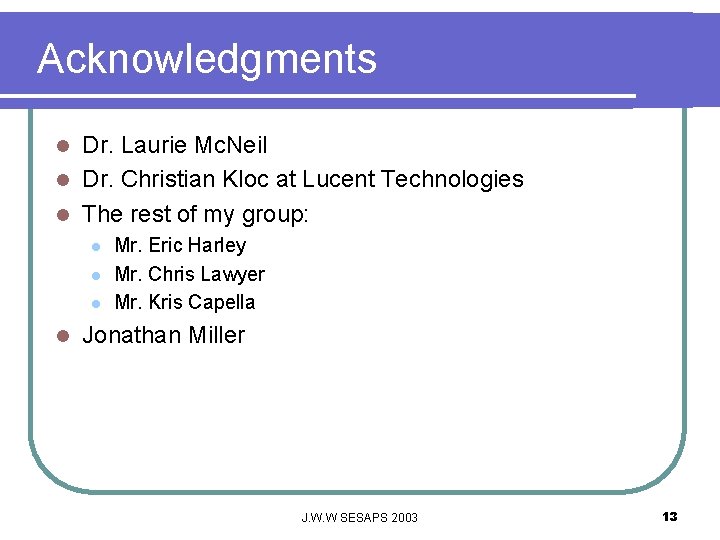
Acknowledgments Dr. Laurie Mc. Neil l Dr. Christian Kloc at Lucent Technologies l The rest of my group: l l l Mr. Eric Harley Mr. Chris Lawyer Mr. Kris Capella Jonathan Miller J. W. W SESAPS 2003 13
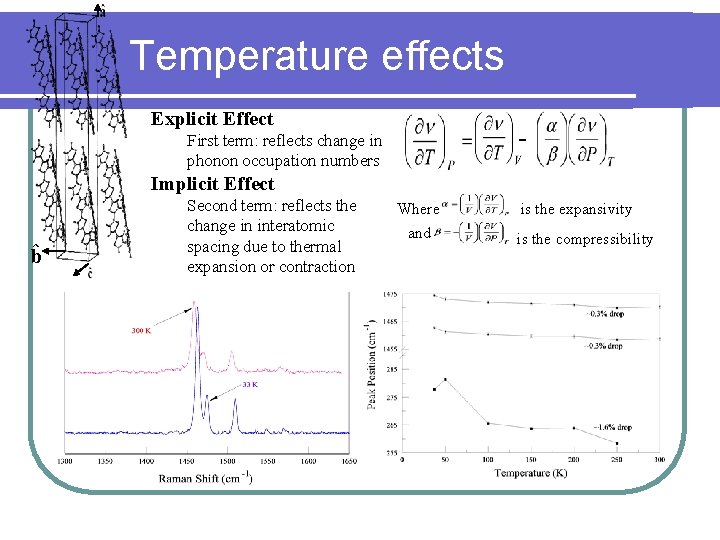
Temperature effects Explicit Effect - First term: reflects change in phonon occupation numbers Implicit Effect bˆ Second term: reflects the change in interatomic spacing due to thermal expansion or contraction Where and is the expansivity is the compressibility
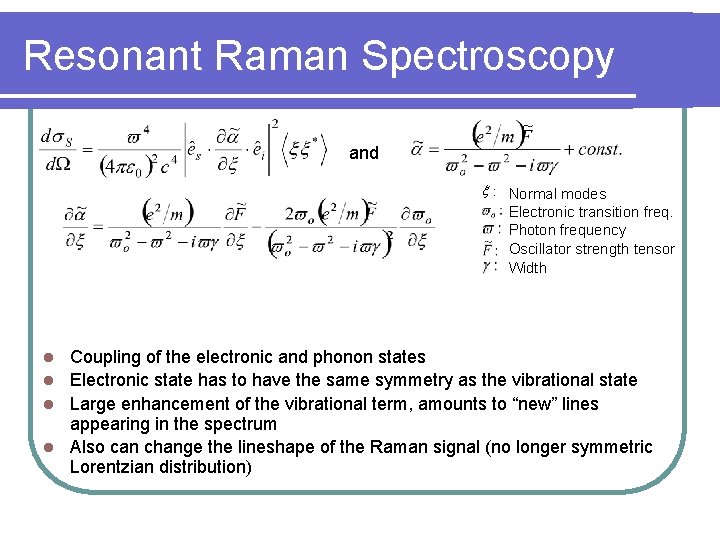
Resonant Raman Spectroscopy and x : Normal modes Electronic transition freq. Photon frequency Oscillator strength tensor Width Coupling of the electronic and phonon states l Electronic state has to have the same symmetry as the vibrational state l Large enhancement of the vibrational term, amounts to “new” lines appearing in the spectrum l Also can change the lineshape of the Raman signal (no longer symmetric Lorentzian distribution) l