Ettore Majorana meets his shadow An introductory course
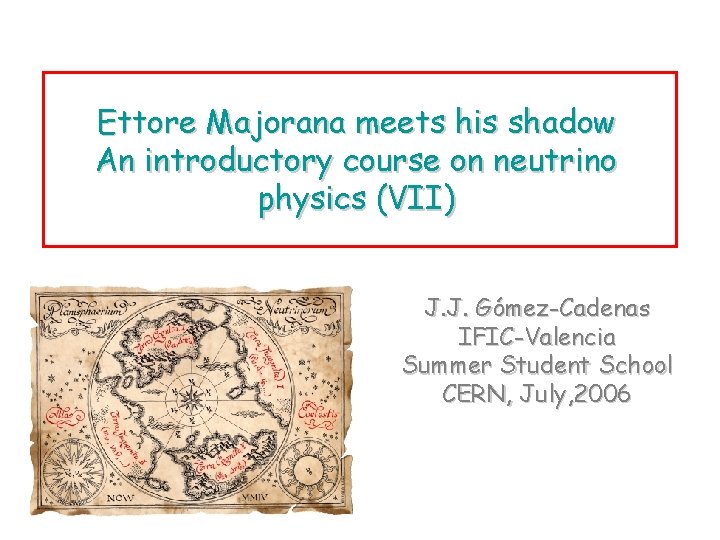
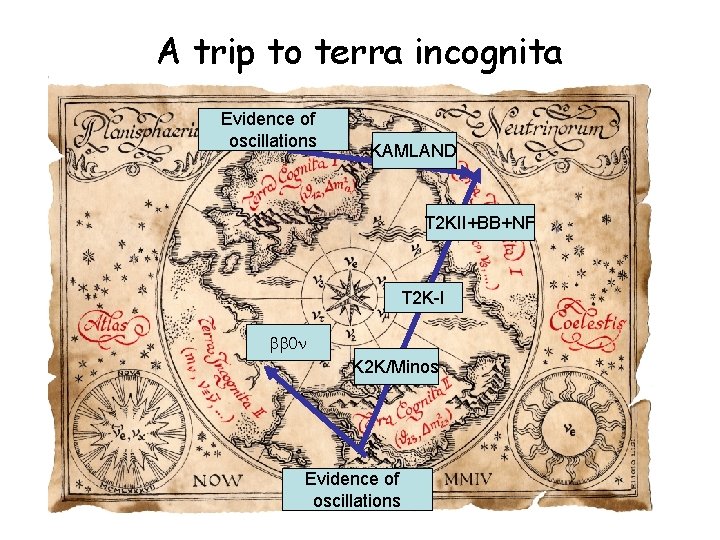
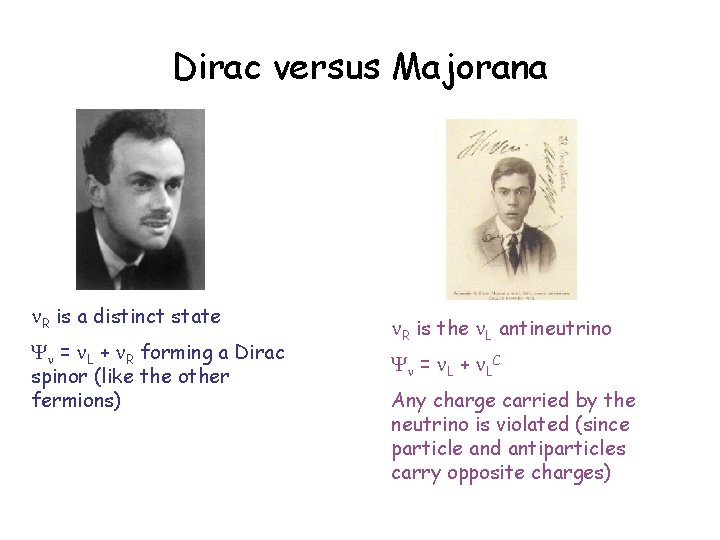
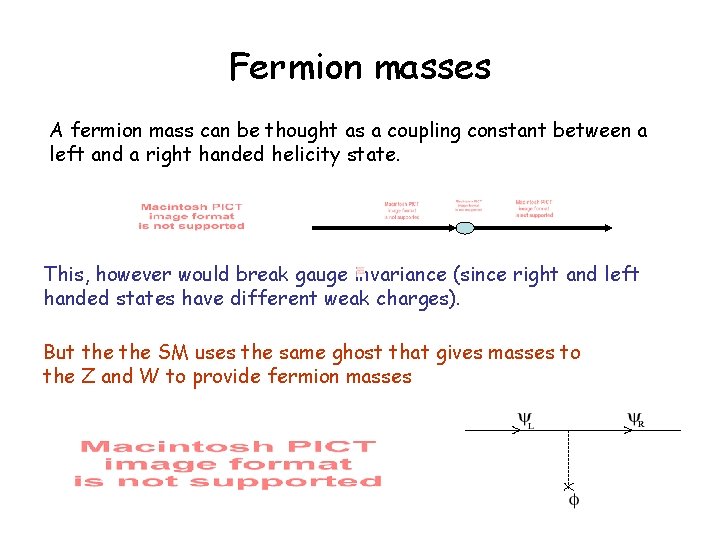
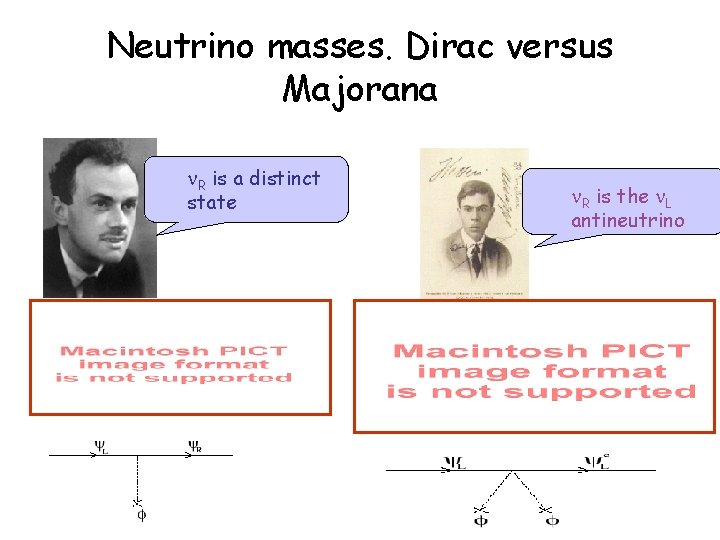
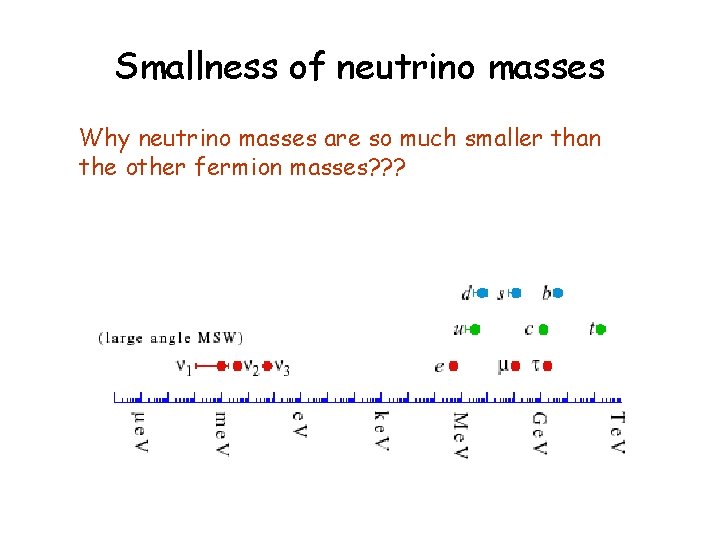
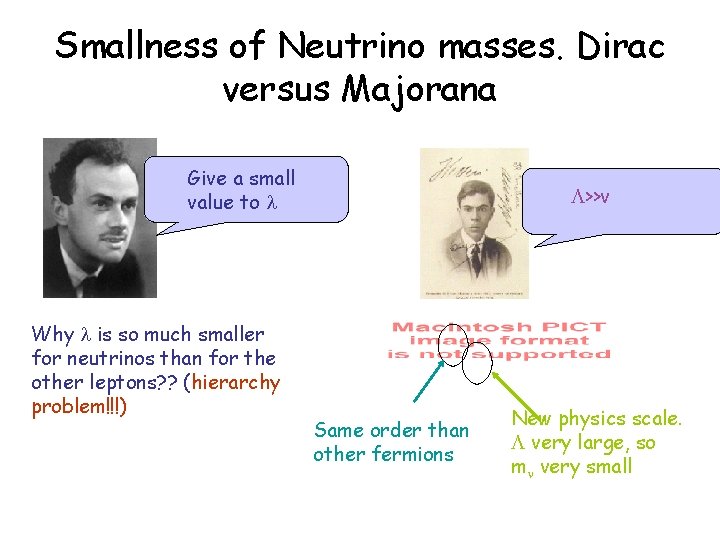
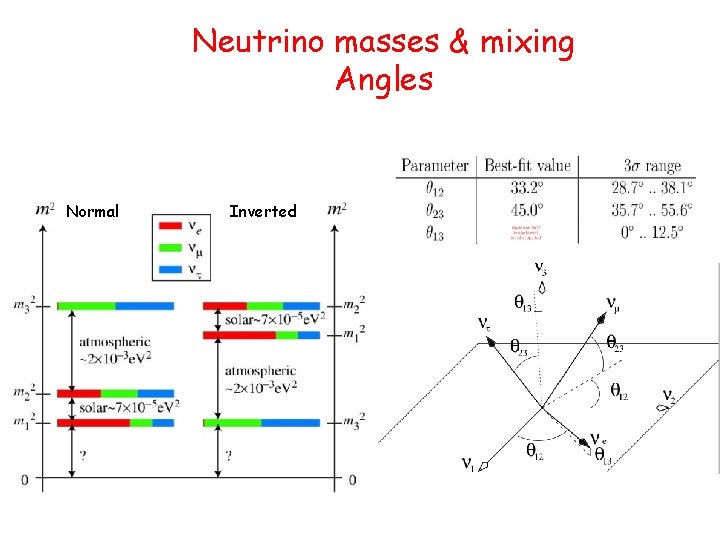
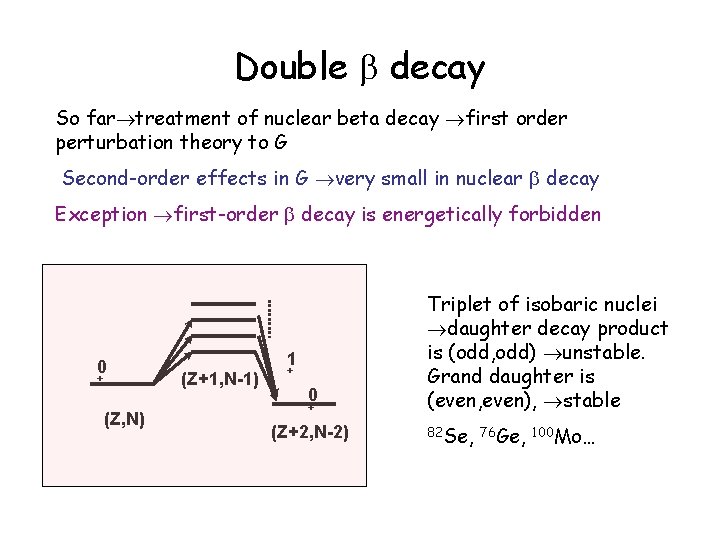
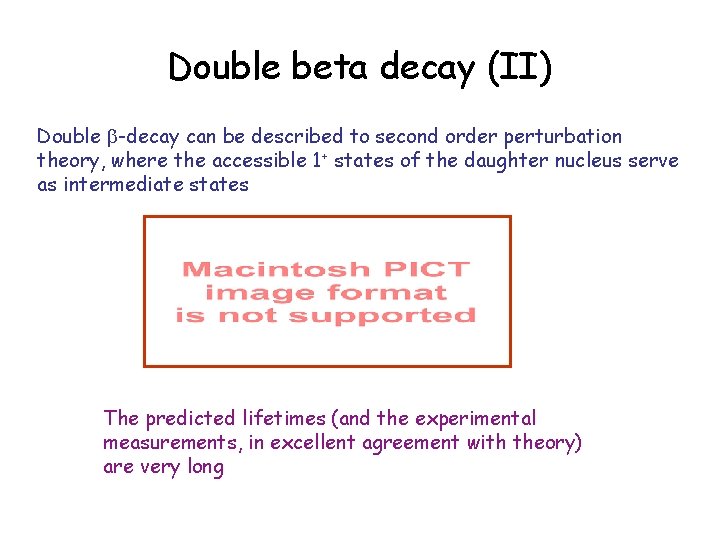
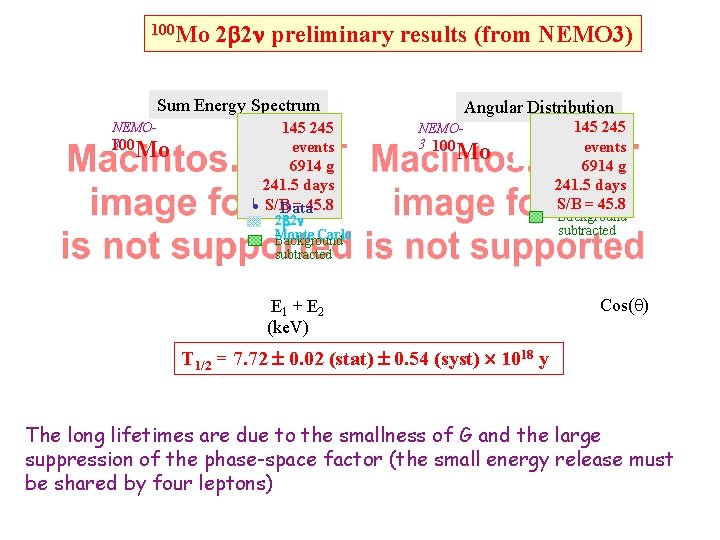
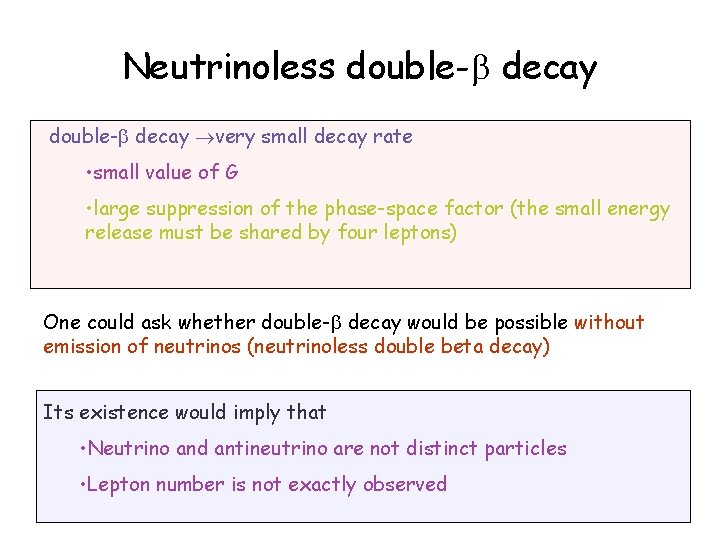
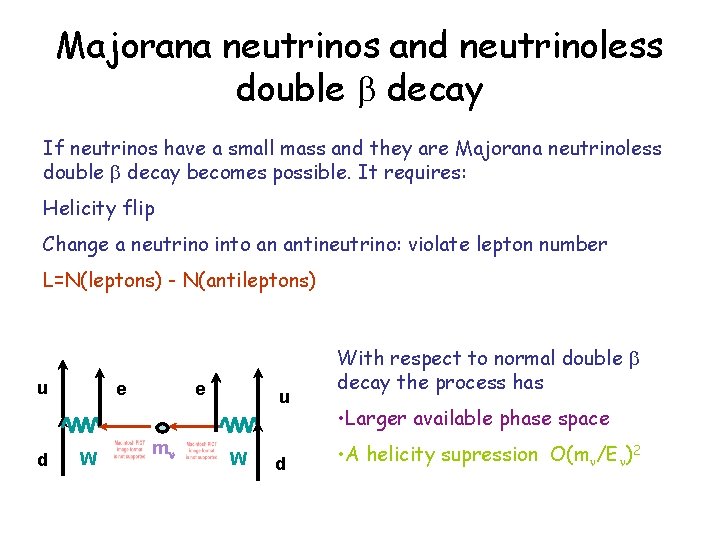
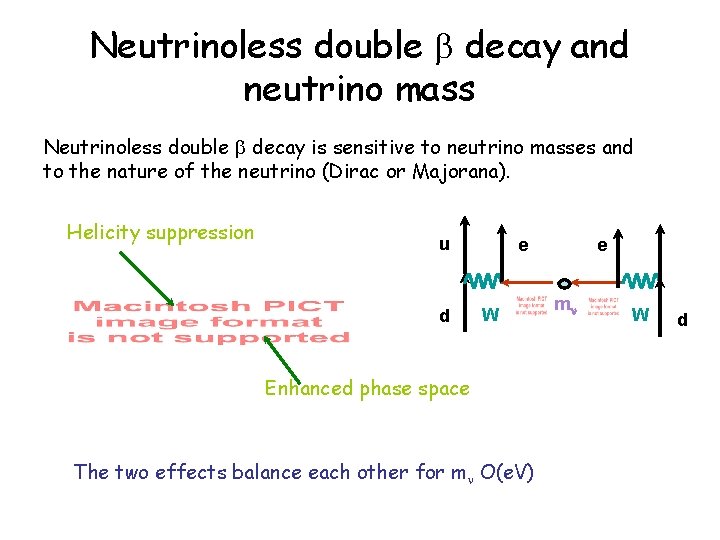
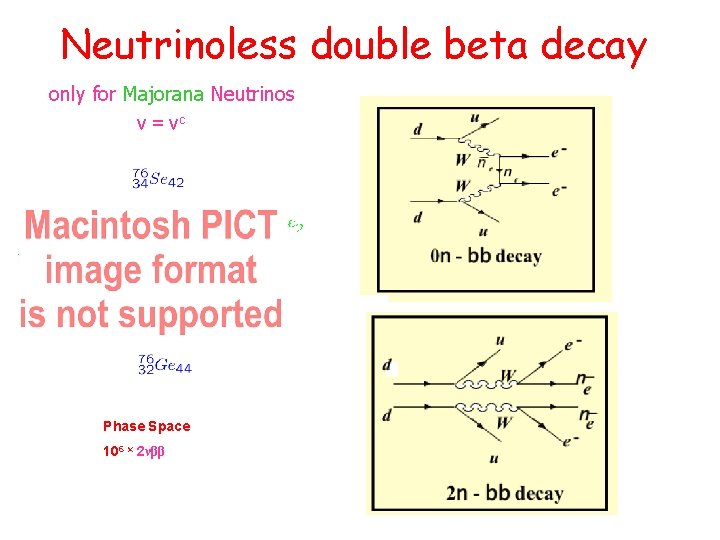
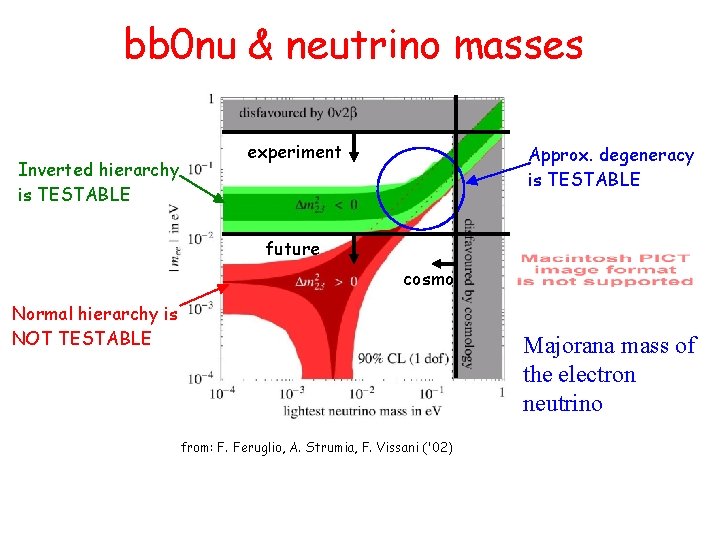
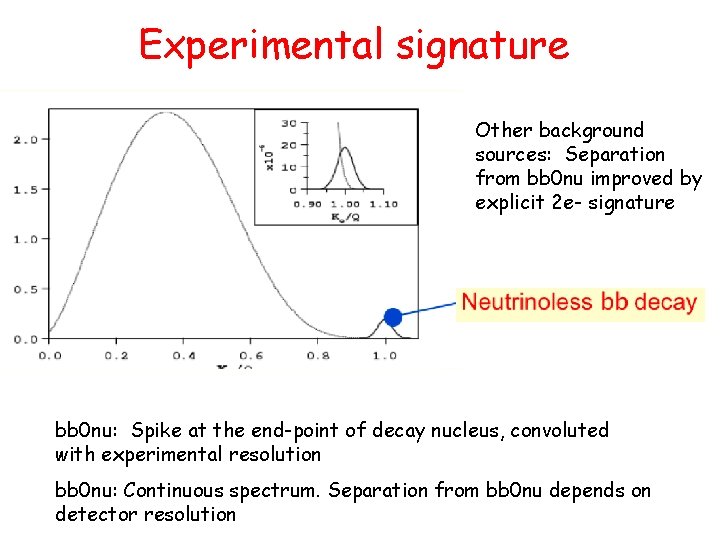
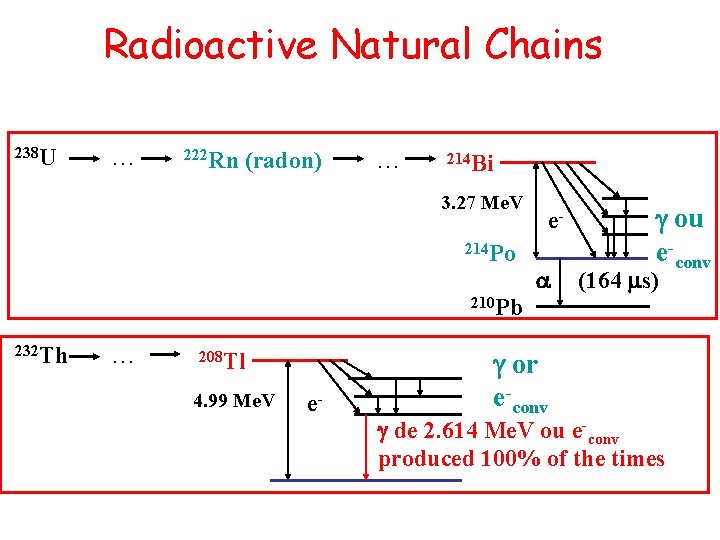
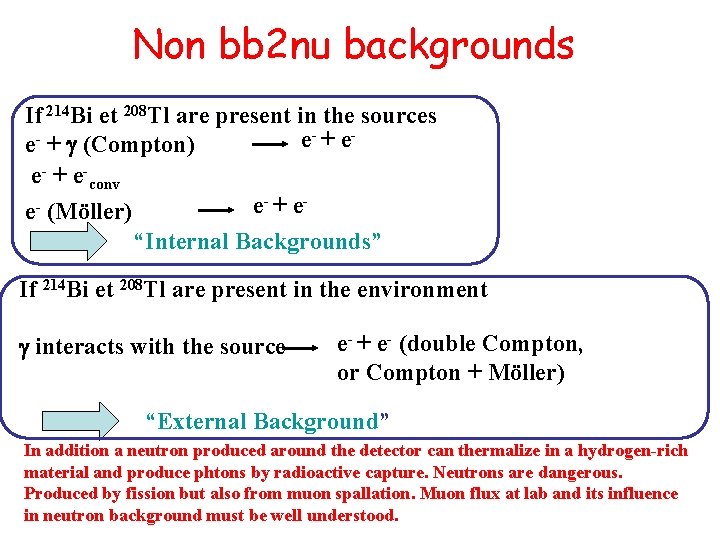
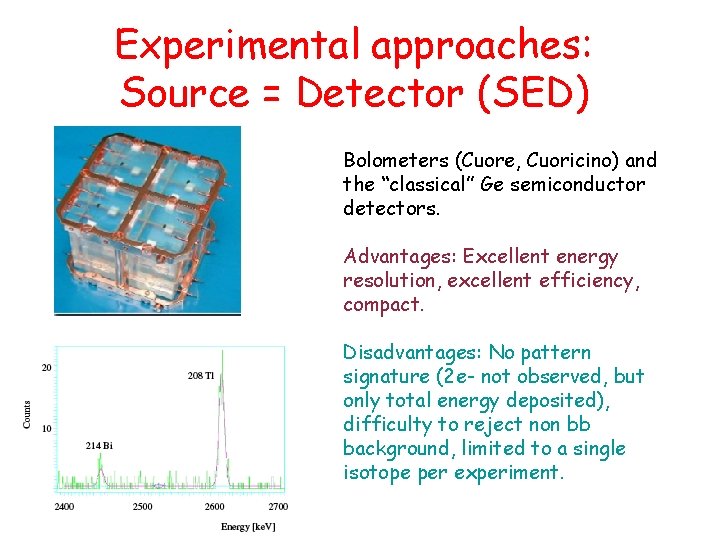
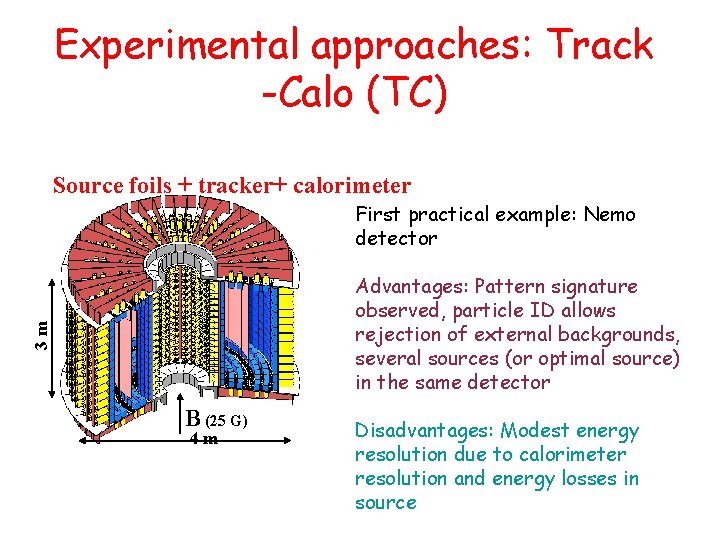
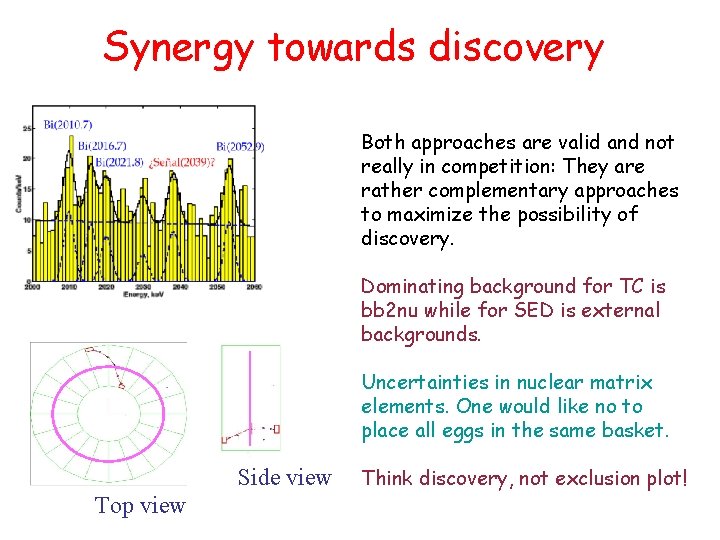
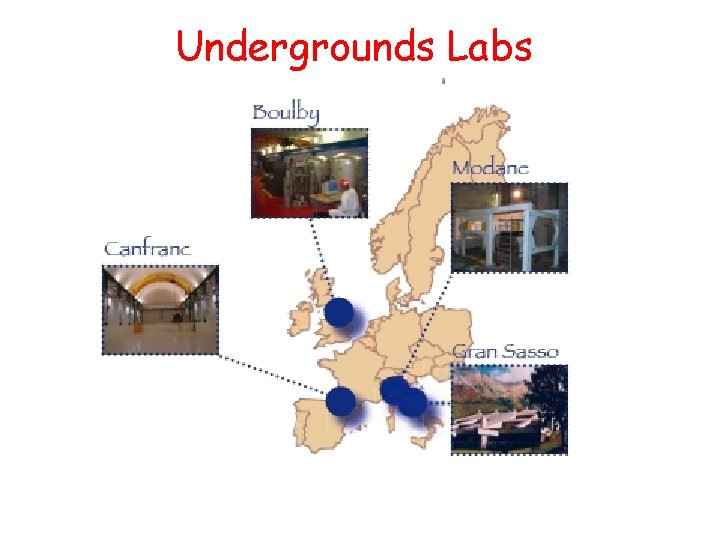
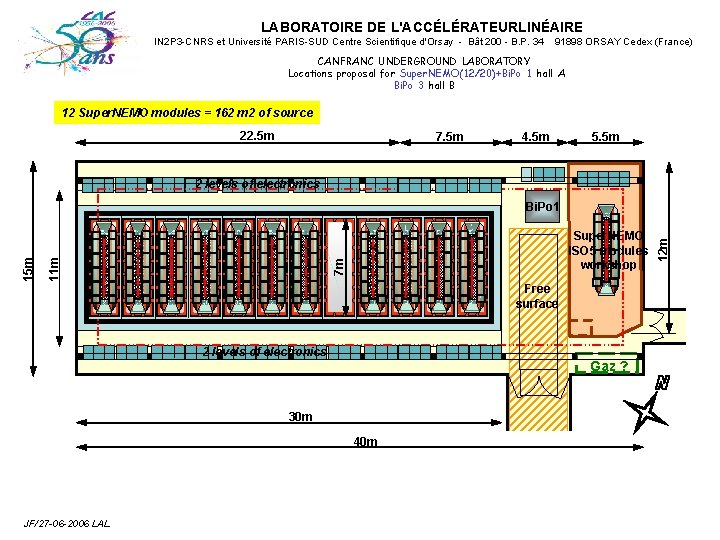
- Slides: 24
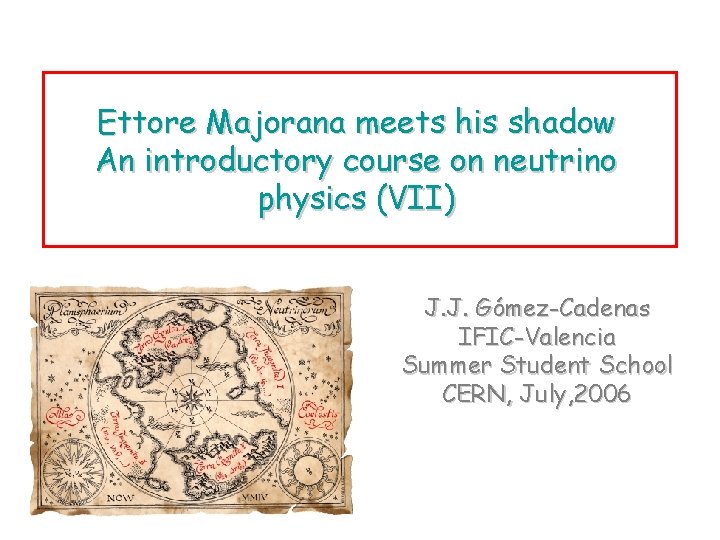
Ettore Majorana meets his shadow An introductory course on neutrino physics (VII) J. J. Gómez-Cadenas IFIC-Valencia Summer Student School CERN, July, 2006
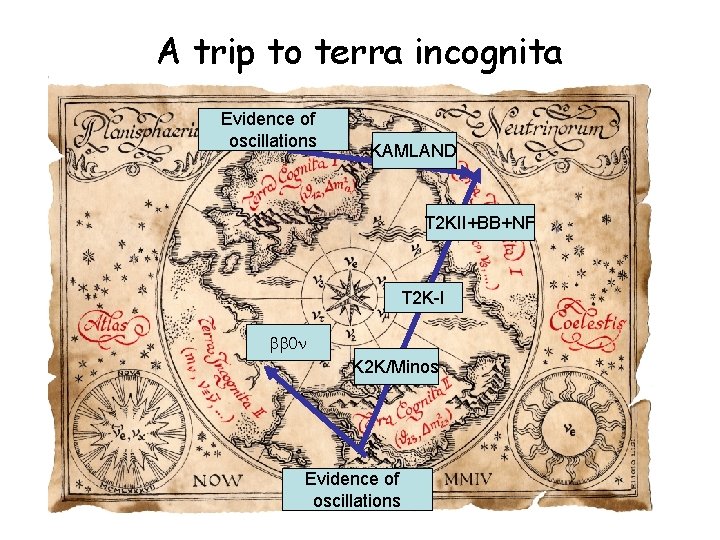
A trip to terra incognita Evidence of oscillations KAMLAND T 2 KII+BB+NF T 2 K-I K 2 K/Minos Evidence of oscillations
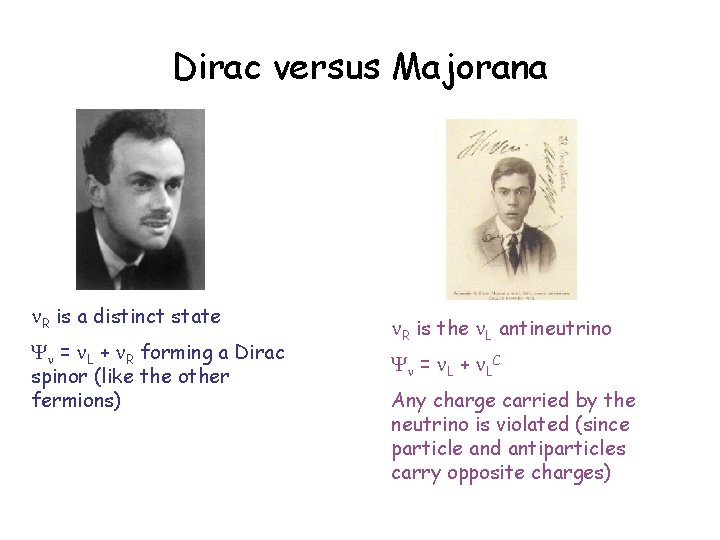
Dirac versus Majorana R is a distinct state Y = L + R forming a Dirac spinor (like the other fermions) R is the L antineutrino Y = L + LC Any charge carried by the neutrino is violated (since particle and antiparticles carry opposite charges)
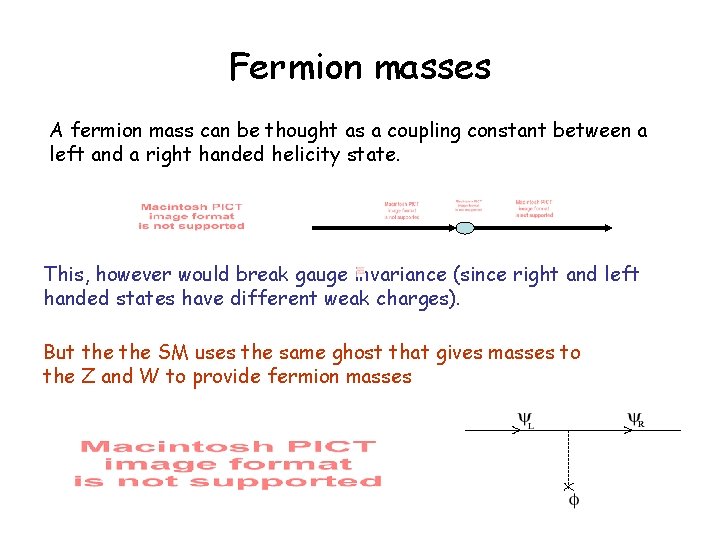
Fermion masses A fermion mass can be thought as a coupling constant between a left and a right handed helicity state. This, however would break gauge invariance (since right and left handed states have different weak charges). But the SM uses the same ghost that gives masses to the Z and W to provide fermion masses
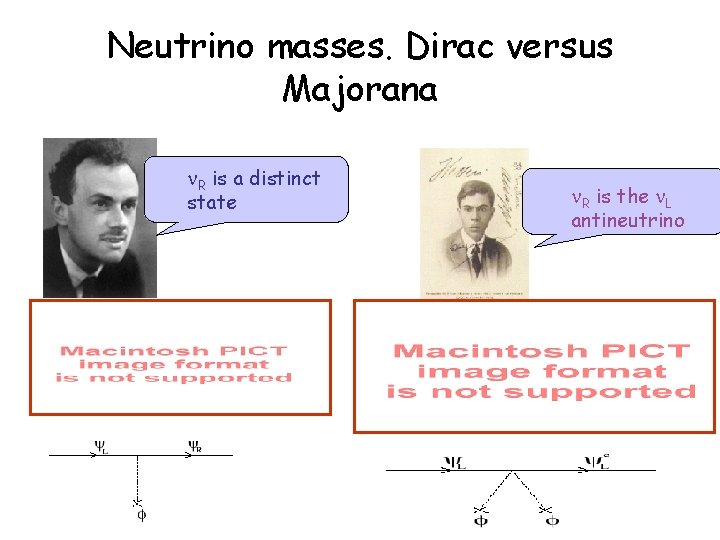
Neutrino masses. Dirac versus Majorana R is a distinct state R is the L antineutrino
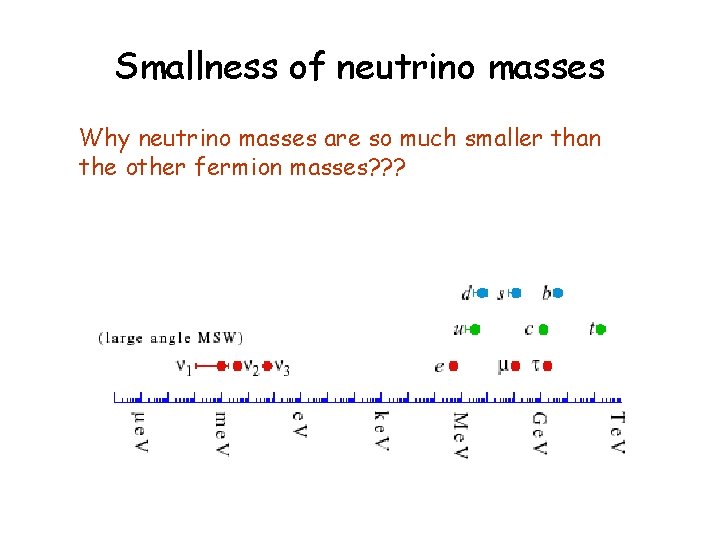
Smallness of neutrino masses Why neutrino masses are so much smaller than the other fermion masses? ? ?
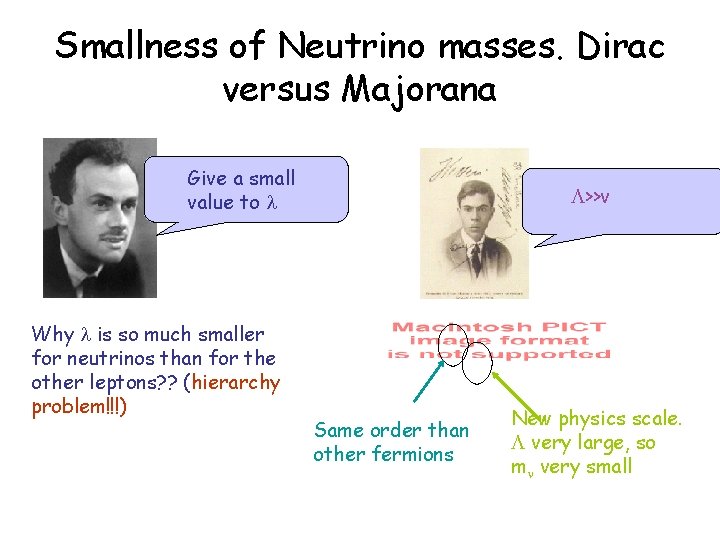
Smallness of Neutrino masses. Dirac versus Majorana Give a small value to l Why l is so much smaller for neutrinos than for the other leptons? ? (hierarchy problem!!!) L>>v Same order than other fermions New physics scale. L very large, so m very small
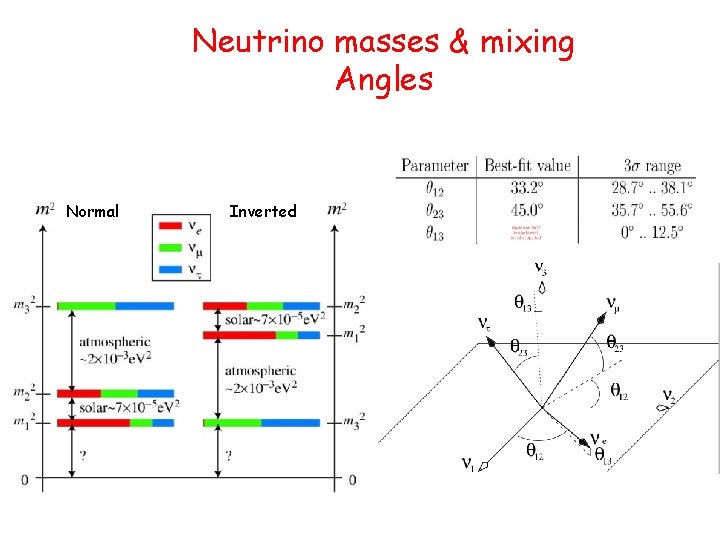
Neutrino masses & mixing Angles Normal Inverted
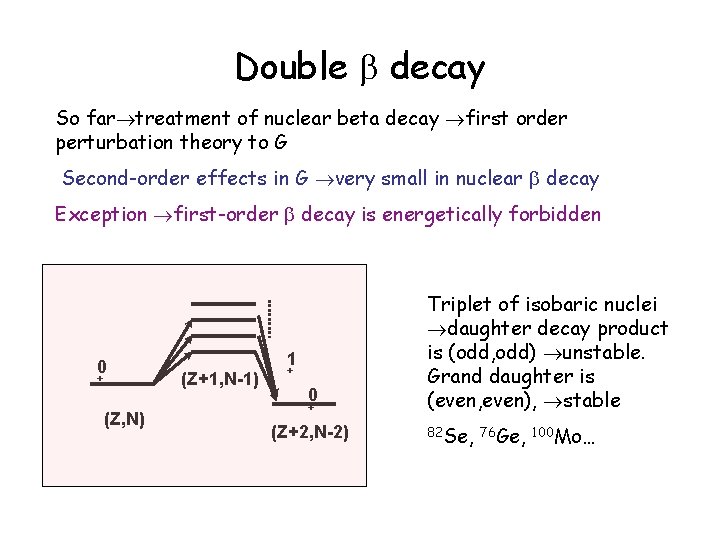
Double decay So far treatment of nuclear beta decay first order perturbation theory to G Second-order effects in G very small in nuclear decay Exception first-order decay is energetically forbidden 0 + (Z, N) 1 (Z+1, N-1) + 0 + (Z+2, N-2) Triplet of isobaric nuclei daughter decay product is (odd, odd) unstable. Grand daughter is (even, even), stable 82 Se, 76 Ge, 100 Mo…
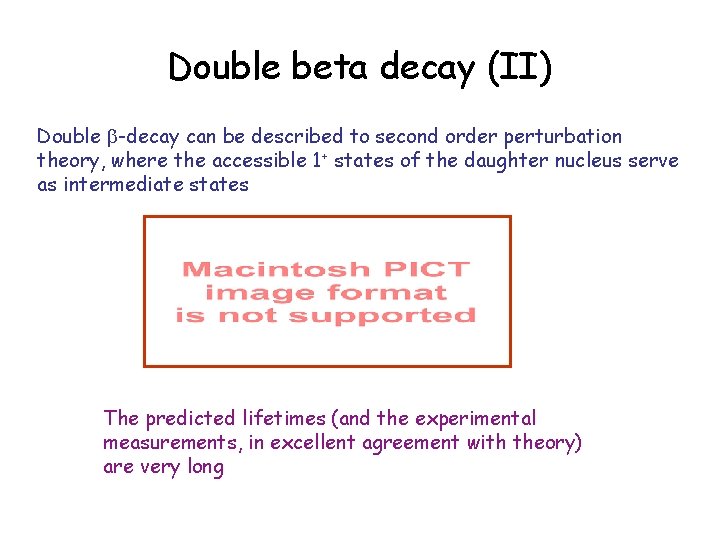
Double beta decay (II) Double -decay can be described to second order perturbation theory, where the accessible 1+ states of the daughter nucleus serve as intermediate states The predicted lifetimes (and the experimental measurements, in excellent agreement with theory) are very long
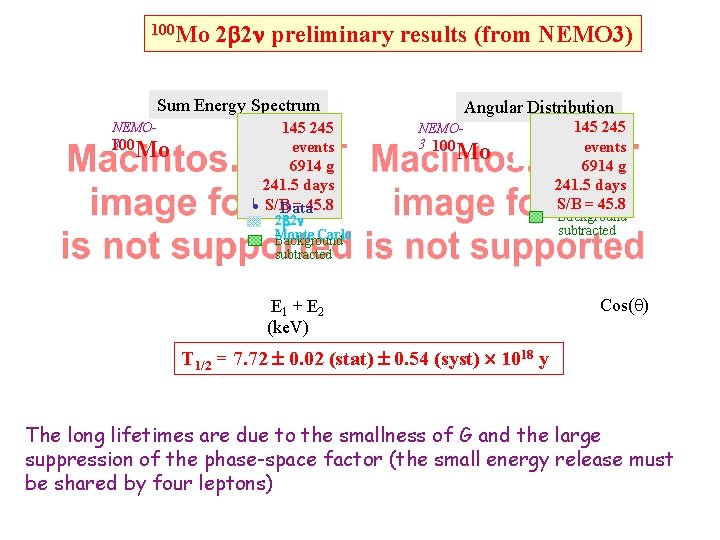
100 Mo 2 2 preliminary results (from NEMO 3) Sum Energy Spectrum NEMO 3 100 Mo 145 245 events 6914 g 241. 5 days = 45. 8 • S/BData Angular Distribution NEMO 3 100 Mo • 2 2 Monte Carlo Background subtracted E 1 + E 2 (ke. V) 145 245 events 6914 g 241. 5 Data days 2 2 S/B = 45. 8 Monte Carlo Background subtracted Cos( ) T 1/2 = 7. 72 ± 0. 02 (stat) ± 0. 54 (syst) 1018 y The long lifetimes are due to the smallness of G and the large suppression of the phase-space factor (the small energy release must be shared by four leptons)
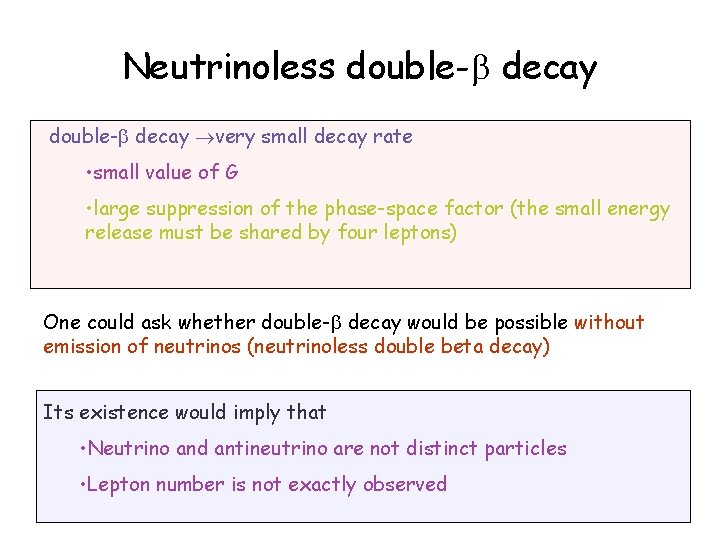
Neutrinoless double- decay very small decay rate • small value of G • large suppression of the phase-space factor (the small energy release must be shared by four leptons) One could ask whether double- decay would be possible without emission of neutrinos (neutrinoless double beta decay) Its existence would imply that • Neutrino and antineutrino are not distinct particles • Lepton number is not exactly observed
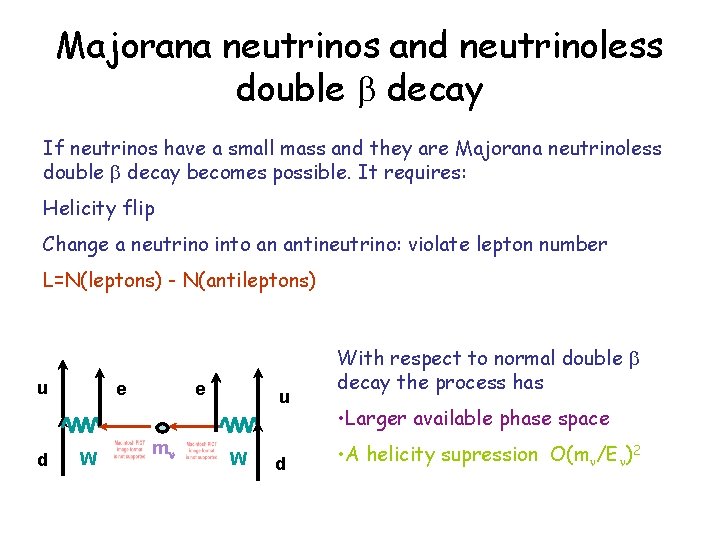
Majorana neutrinos and neutrinoless double decay If neutrinos have a small mass and they are Majorana neutrinoless double decay becomes possible. It requires: Helicity flip Change a neutrino into an antineutrino: violate lepton number L=N(leptons) - N(antileptons) u d e W e m u W d With respect to normal double decay the process has • Larger available phase space • A helicity supression O(m /E )2
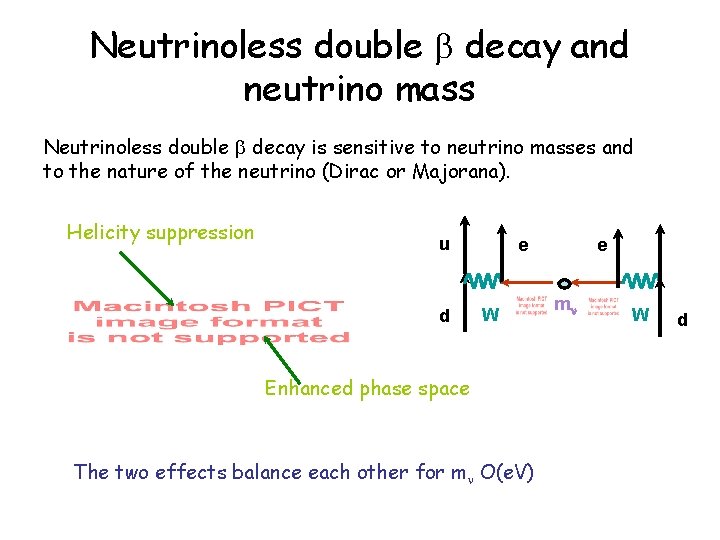
Neutrinoless double decay and neutrino mass Neutrinoless double decay is sensitive to neutrino masses and to the nature of the neutrino (Dirac or Majorana). Helicity suppression u d e W Enhanced phase space The two effects balance each other for m O(e. V) e m W d
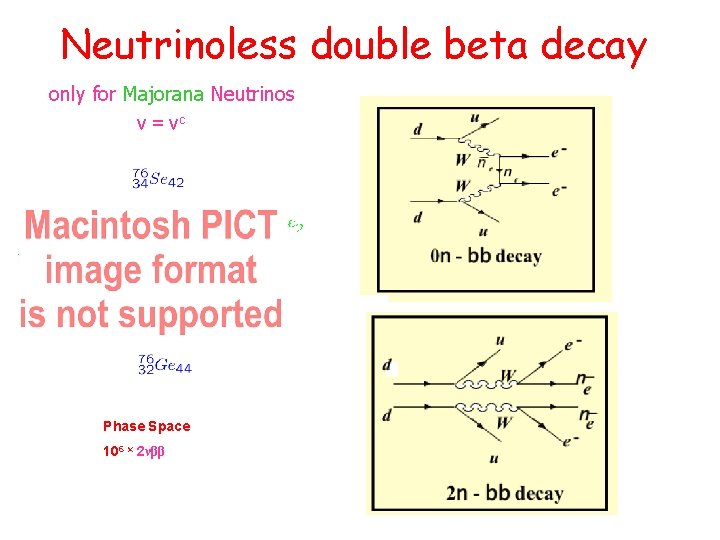
Neutrinoless double beta decay only for Majorana Neutrinos ν = νc P P Left ν n n Left Phase Space 106 x 2νββ
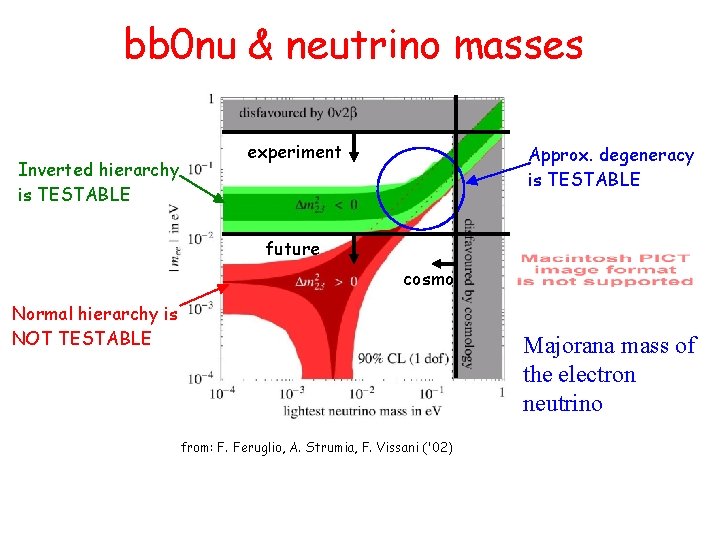
bb 0 nu & neutrino masses Inverted hierarchy is TESTABLE experiment Approx. degeneracy is TESTABLE future cosmo Normal hierarchy is NOT TESTABLE Majorana mass of the electron neutrino from: F. Feruglio, A. Strumia, F. Vissani ('02)
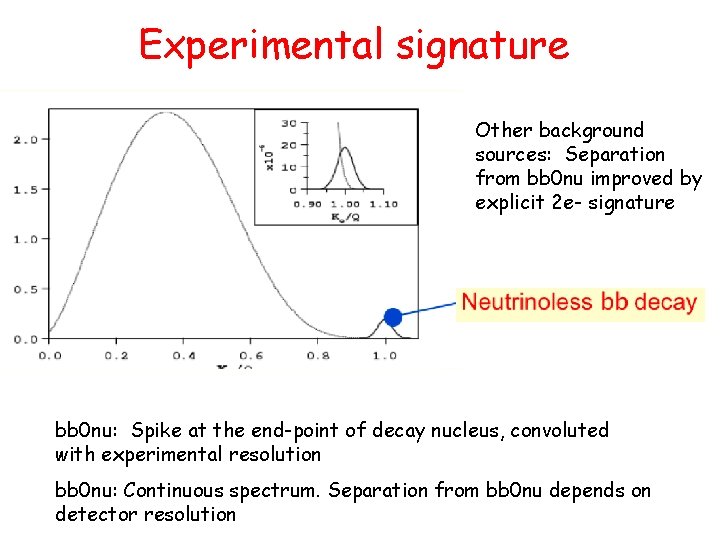
Experimental signature Other background sources: Separation from bb 0 nu improved by explicit 2 e- signature bb 0 nu: Spike at the end-point of decay nucleus, convoluted with experimental resolution bb 0 nu: Continuous spectrum. Separation from bb 0 nu depends on detector resolution
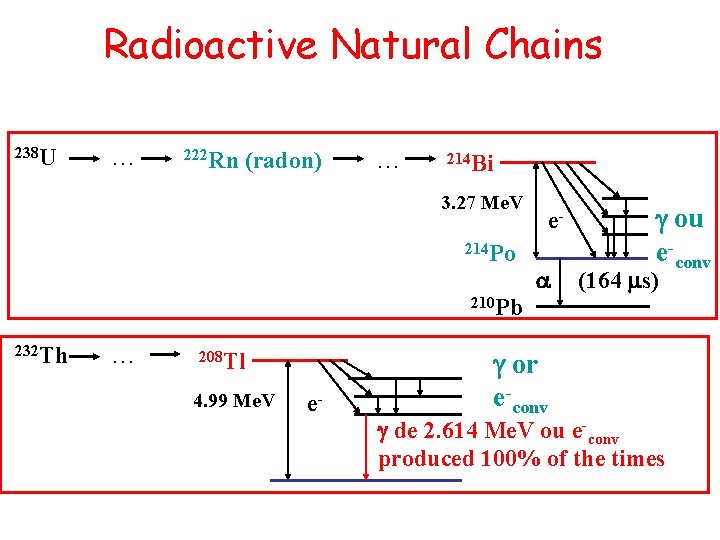
Radioactive Natural Chains 238 U … 222 Rn (radon) … 214 Bi 3. 27 Me. V 214 Po ea g ou e-conv (164 ms) 210 Pb 232 Th … 208 Tl 4. 99 Me. V e- g or e-conv g de 2. 614 Me. V ou e-conv produced 100% of the times
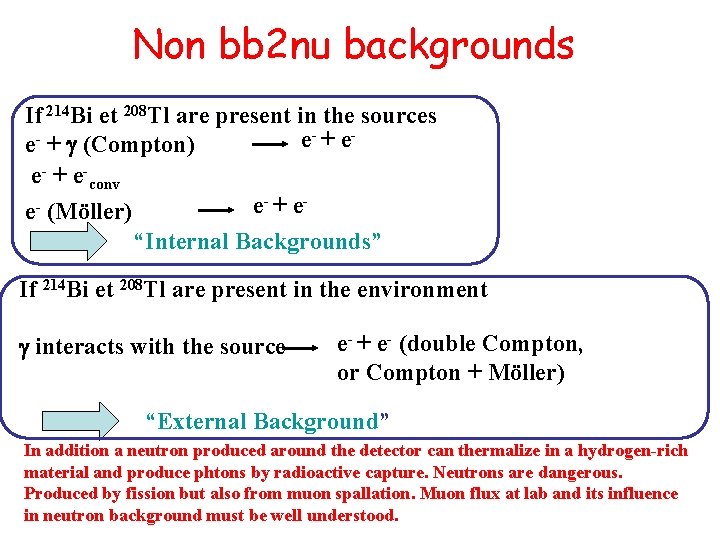
Non bb 2 nu backgrounds If 214 Bi et 208 Tl are present in the sources e- + ee- + g (Compton) e- + e-conv e- + e e- (Möller) “Internal Backgrounds” If 214 Bi et 208 Tl are present in the environment g interacts with the source e- + e- (double Compton, or Compton + Möller) “External Background” In addition a neutron produced around the detector can thermalize in a hydrogen-rich material and produce phtons by radioactive capture. Neutrons are dangerous. Produced by fission but also from muon spallation. Muon flux at lab and its influence in neutron background must be well understood.
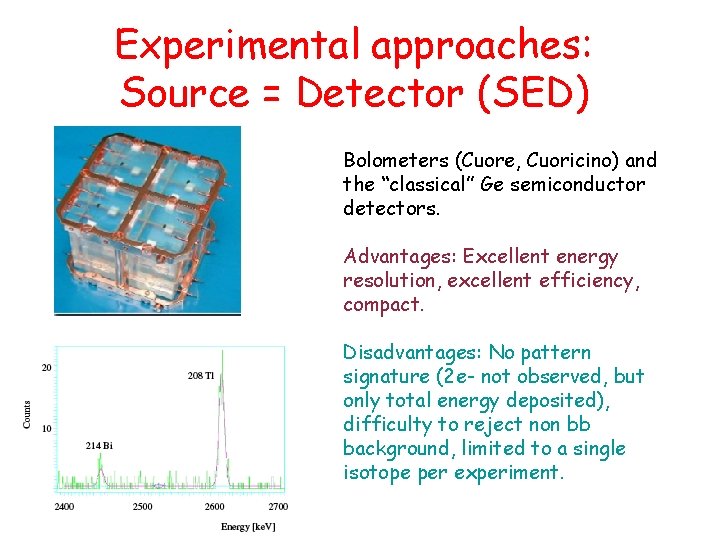
Experimental approaches: Source = Detector (SED) Bolometers (Cuore, Cuoricino) and the “classical” Ge semiconductor detectors. Advantages: Excellent energy resolution, excellent efficiency, compact. Disadvantages: No pattern signature (2 e- not observed, but only total energy deposited), difficulty to reject non bb background, limited to a single isotope per experiment.
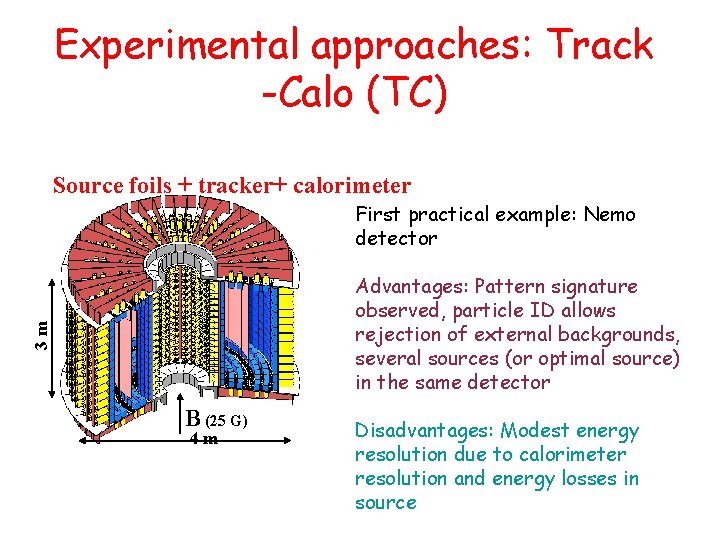
Experimental approaches: Track -Calo (TC) Source foils + tracker+ calorimeter First practical example: Nemo detector 3 m Advantages: Pattern signature observed, particle ID allows rejection of external backgrounds, several sources (or optimal source) in the same detector B (25 G) 4 m Disadvantages: Modest energy resolution due to calorimeter resolution and energy losses in source
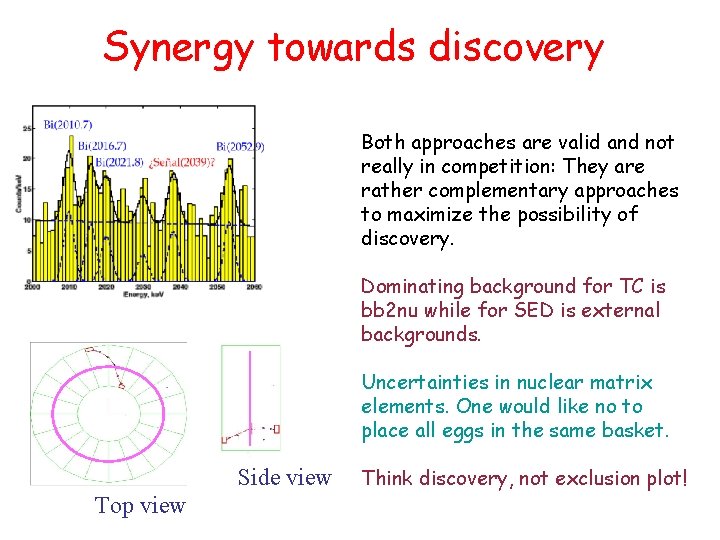
Synergy towards discovery Both approaches are valid and not really in competition: They are rather complementary approaches to maximize the possibility of discovery. Dominating background for TC is bb 2 nu while for SED is external backgrounds. Uncertainties in nuclear matrix elements. One would like no to place all eggs in the same basket. Side view Top view Think discovery, not exclusion plot!
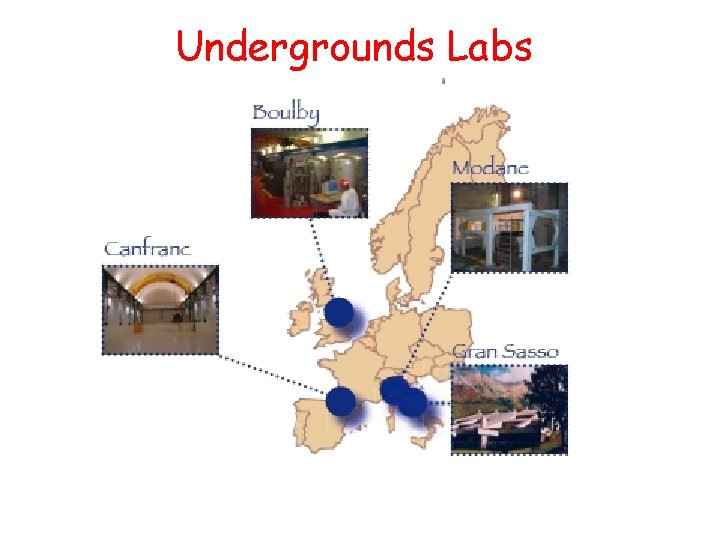
Undergrounds Labs
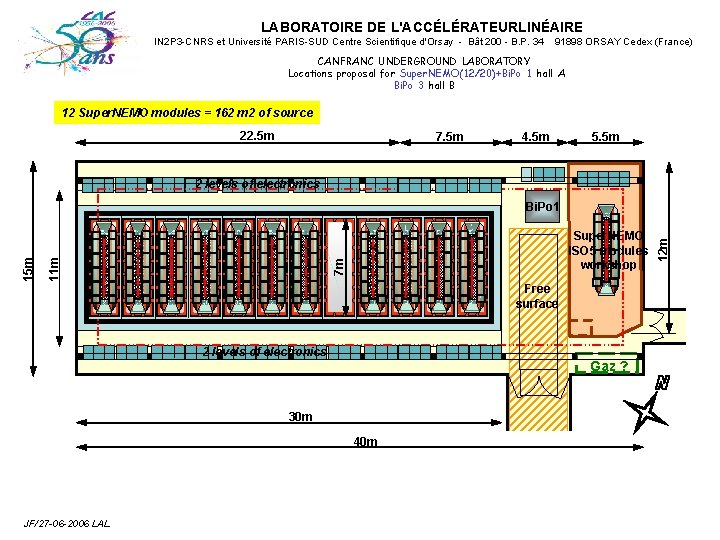
LABORATOIRE DE L'ACCÉLÉRATEURLINÉAIRE IN 2 P 3 -CNRS et Université PARIS-SUD Centre Scientifique d'Orsay - Bât 200 - B. P. 34 91898 ORSAY Cedex (France) CANFRANC UNDERGROUND LABORATORY Locations proposal for Super. NEMO(12/20)+Bi. Po 1 hall A Bi. Po 3 hall B 12 Super. NEMO modules = 162 m 2 of source 22. 5 m 7. 5 m 4. 5 m 5. 5 m 2 levels of electronics Free surface 2 levels of electronics Gaz ? 30 m 40 m JF/27 -06 -2006 LAL 12 m Super. NEMO ISO 5 modules workshop 7 m 11 m 15 m Bi. Po 1
Ettore majorana scomparsa
Istituto ettore majorana seriate
Liceo scientifico ettore majorana desio
Ettore majorana foundation
Iti majorana
Majorana bachelet
Liceo desio spark
Liceo scientifico ettore majorana desio
Liceo scientifico ettore majorana desio
Army traffic safety introductory course
Army traffic safety introductory course
Www.atlasti.com
Introductory maxqda course
Remains poem armitage
Bars of rage
Cosa è l epica
Ettore lanzarone
Duello tra ettore e achille
Un'intera nottata buttato vicino a un compagno massacrato
Ettore ferrari franciulli
Il pomo della discordia scuola primaria
Cesaris casalpusterlengo classi prime
Majorana di grugliasco
Majorana avezzano
Itis majorana grugliasco