SmallScale Fading PROF MICHAEL TSAI 20150424 RX just
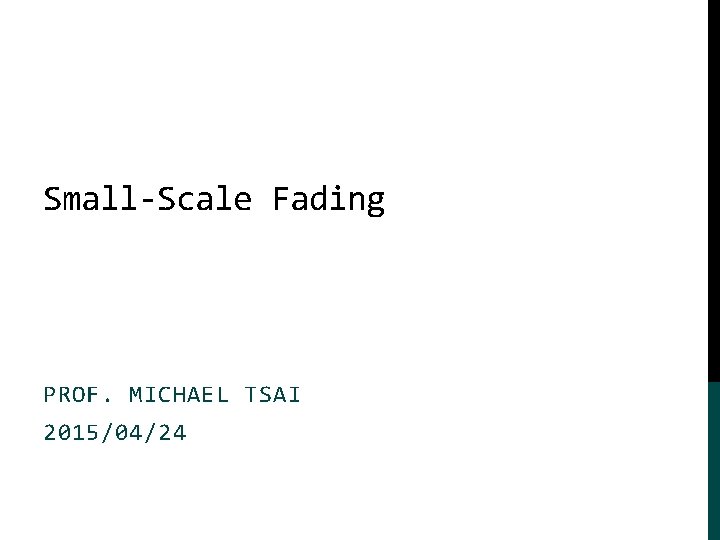
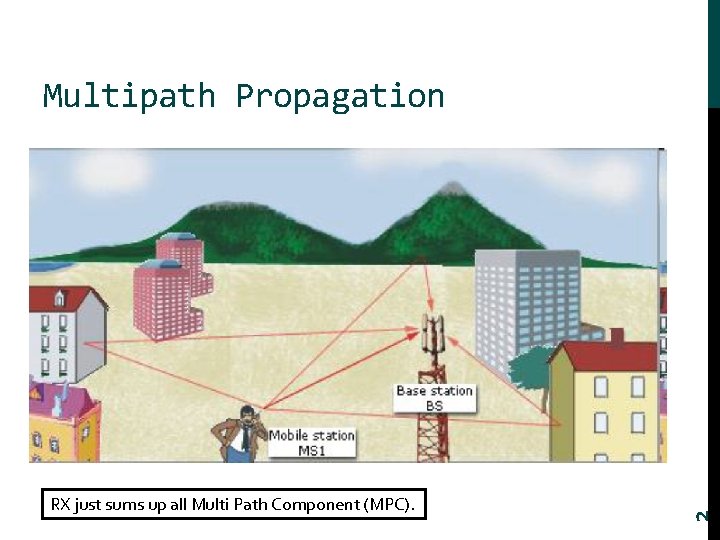
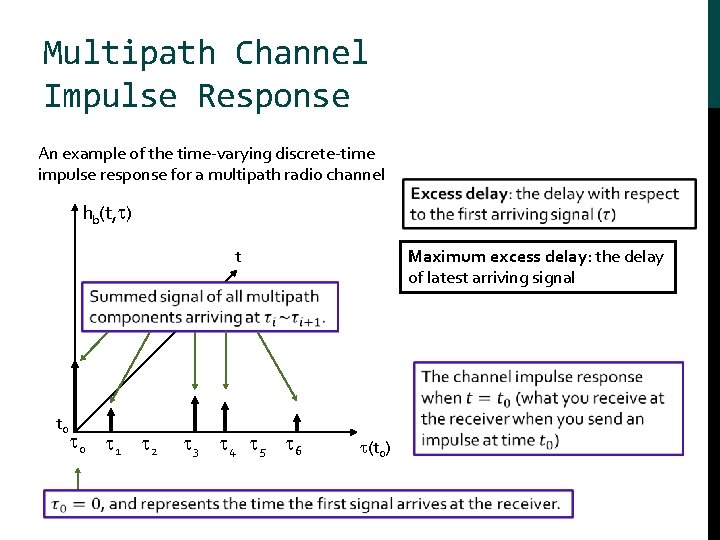
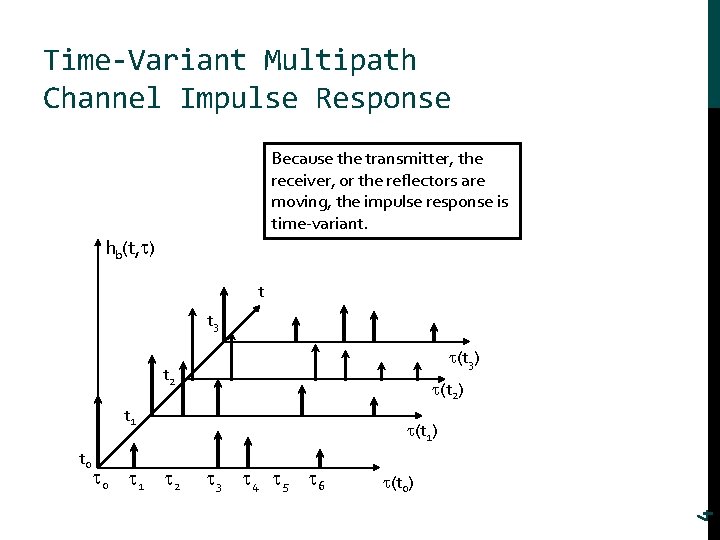
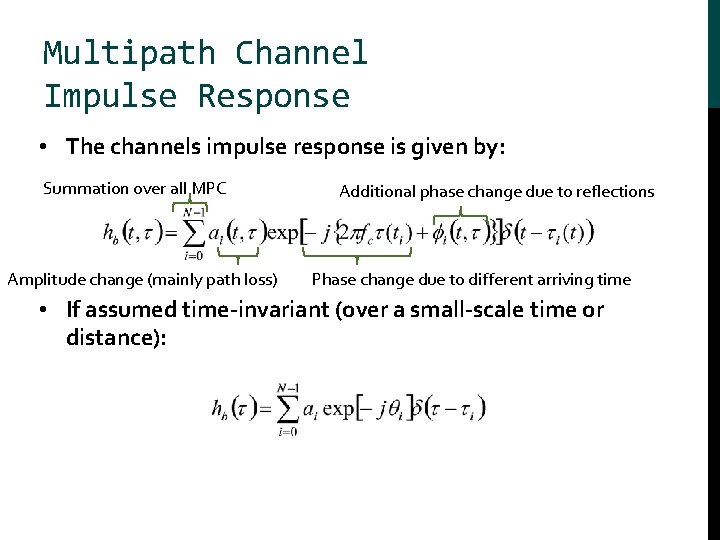
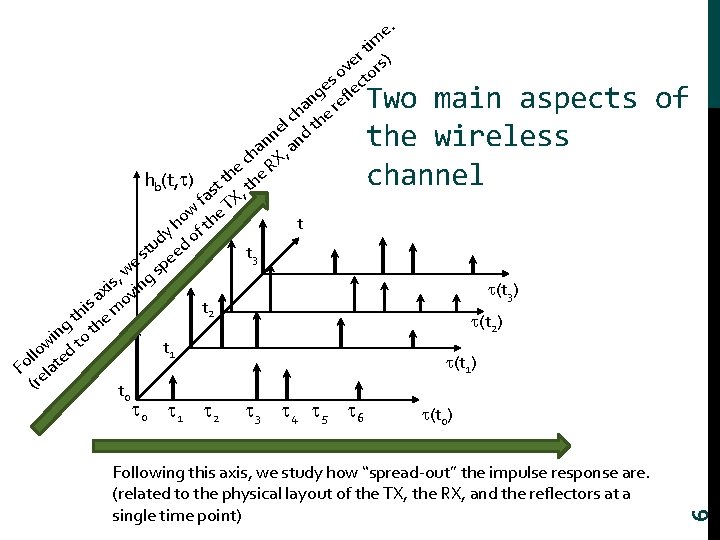
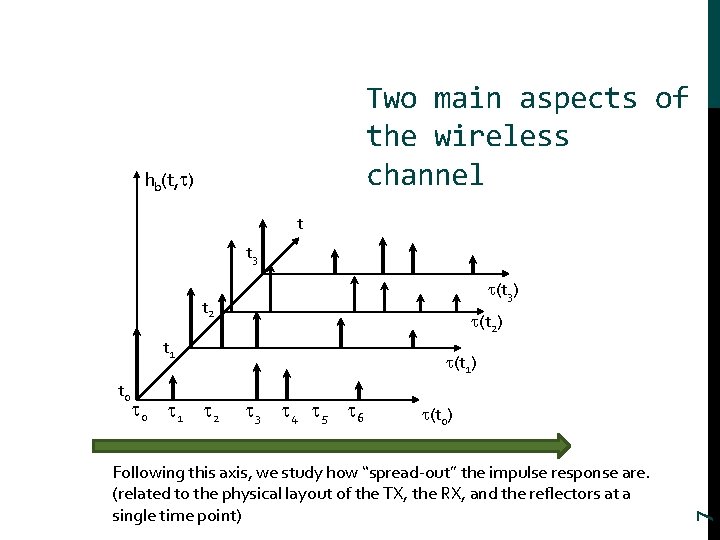
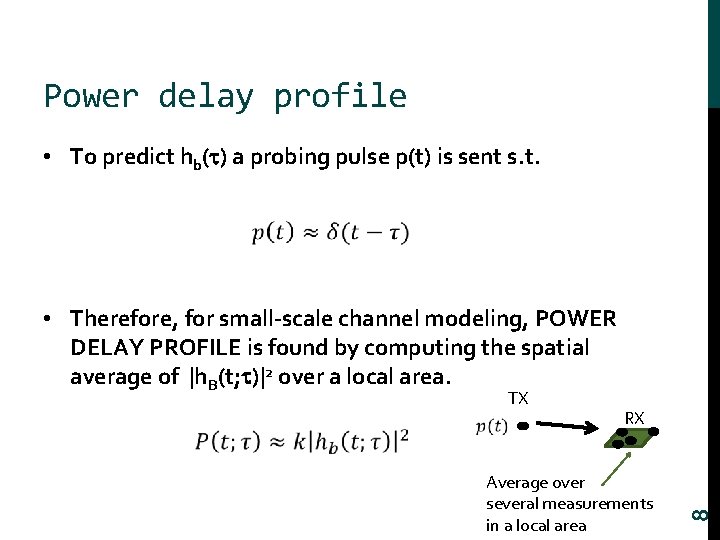
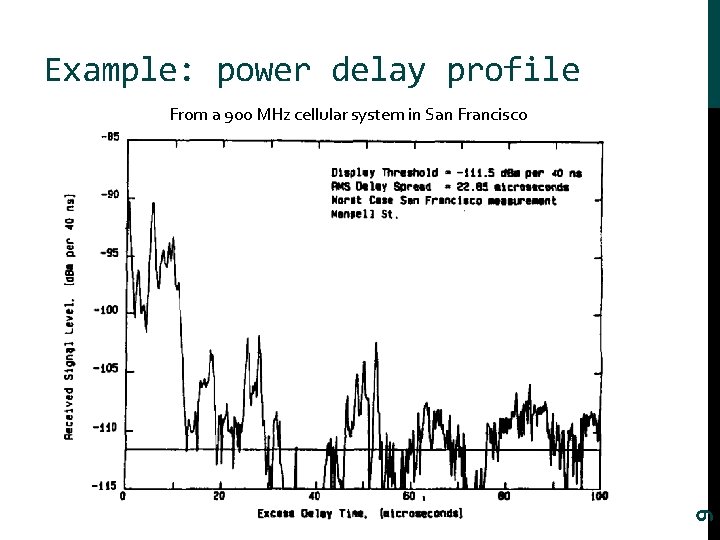
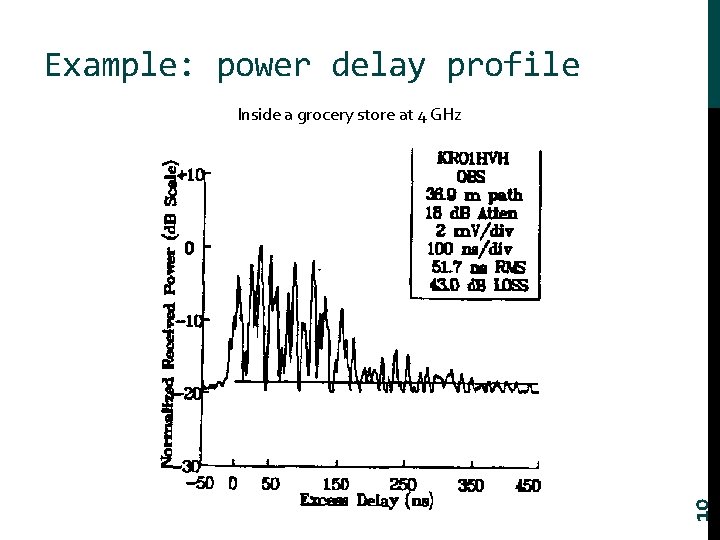
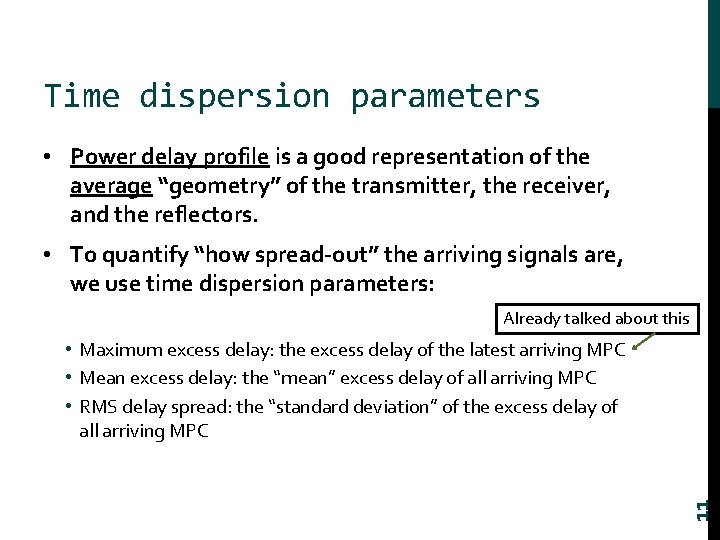
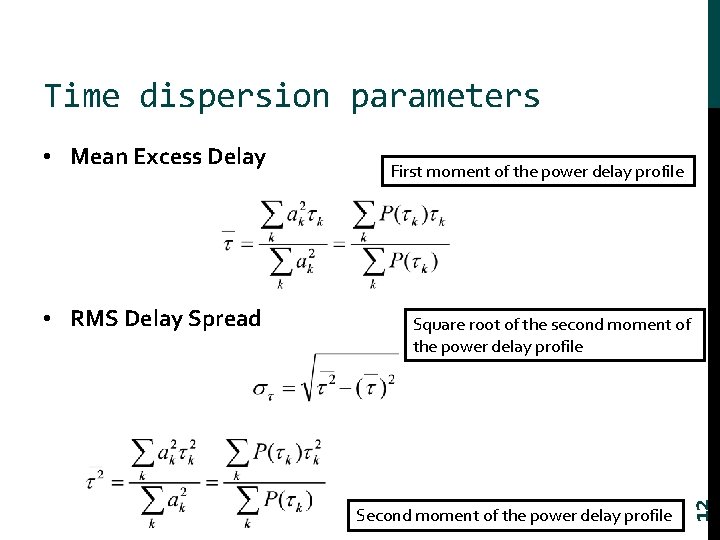
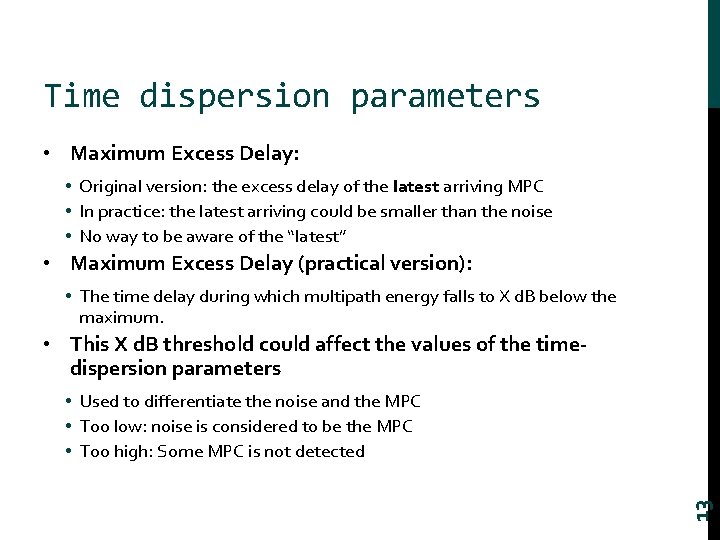
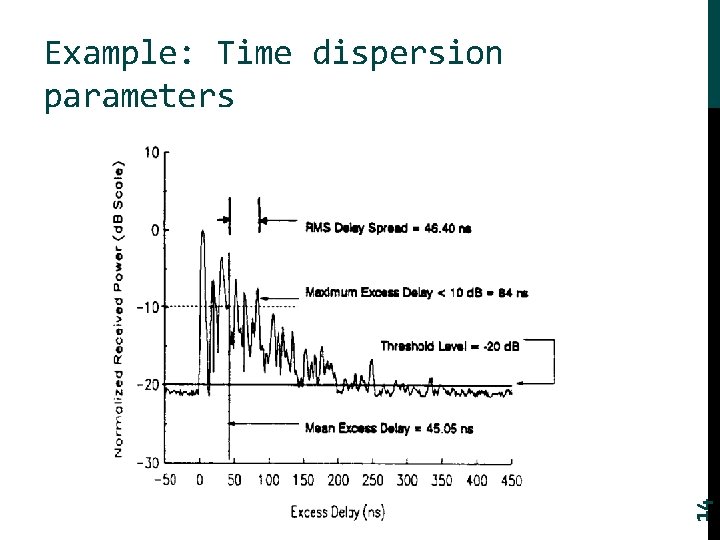
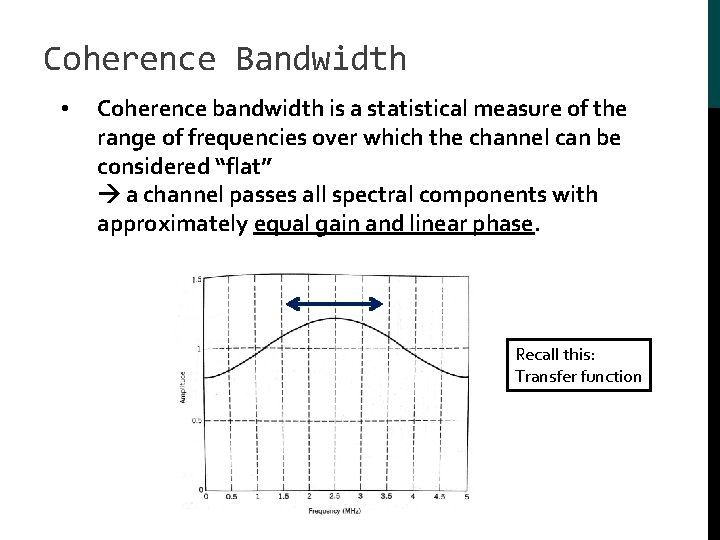
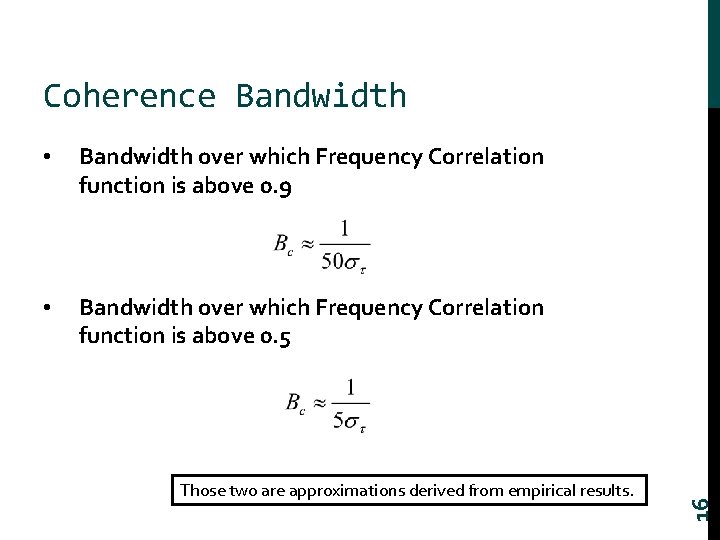
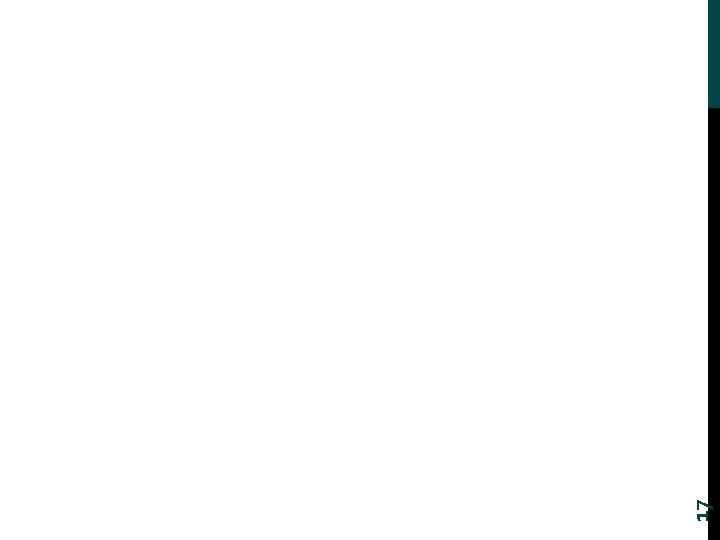
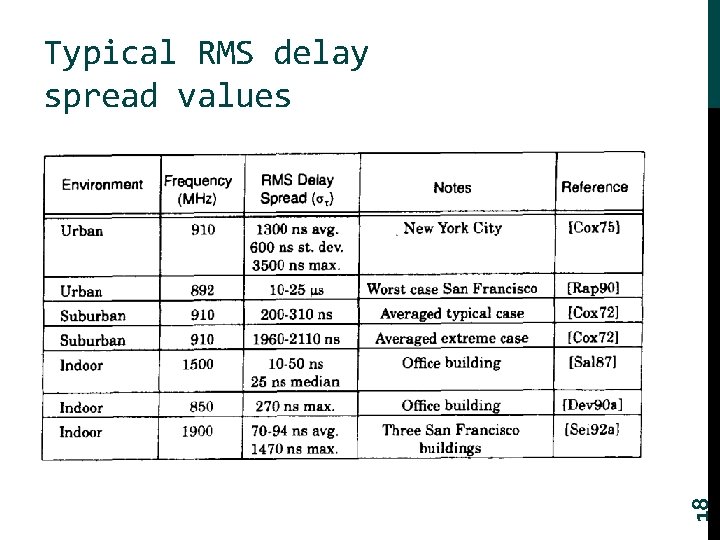
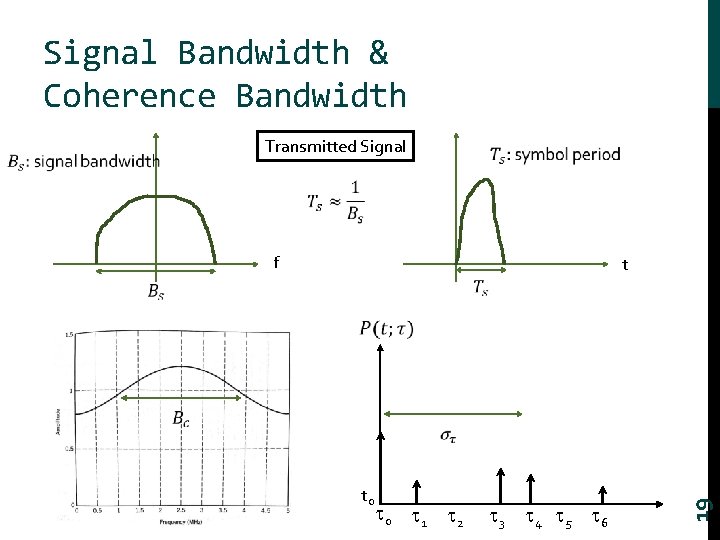
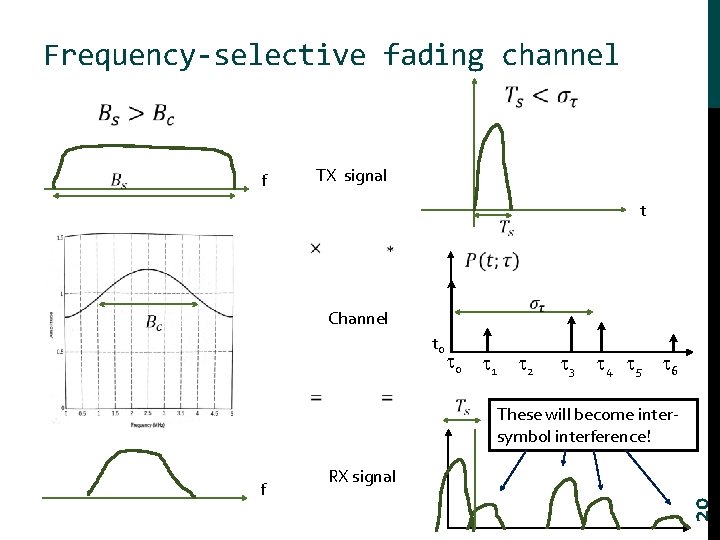
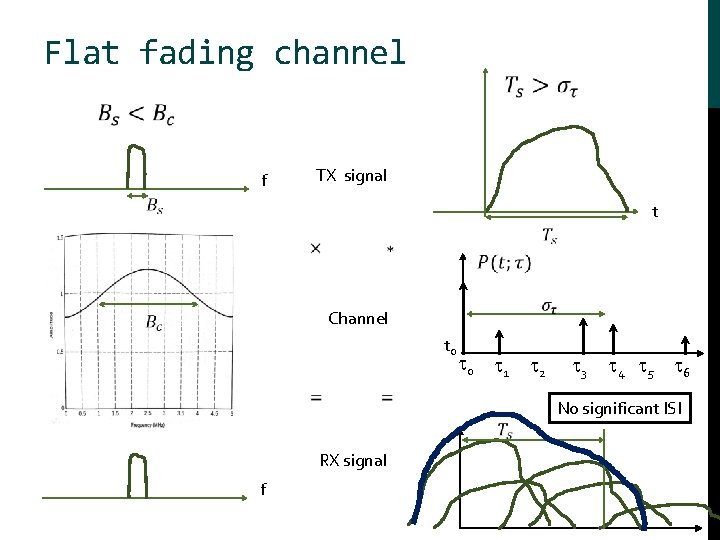
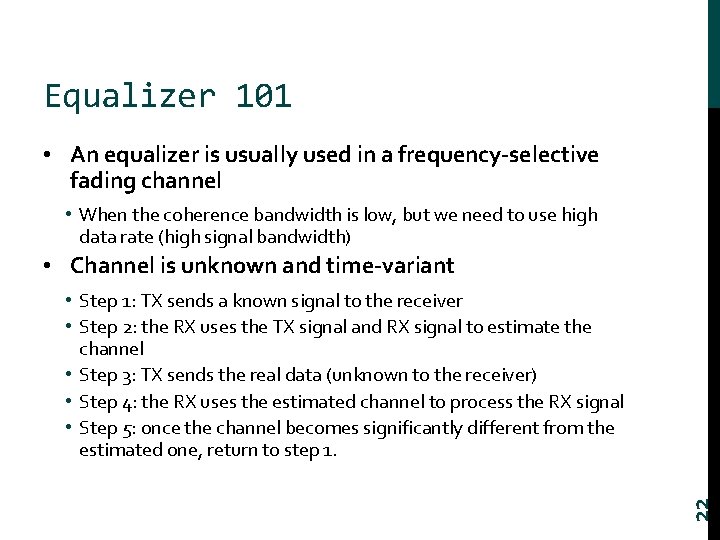
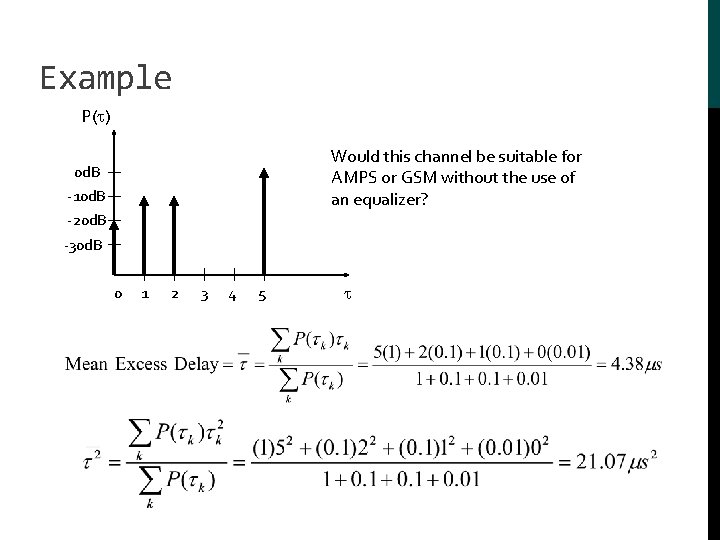
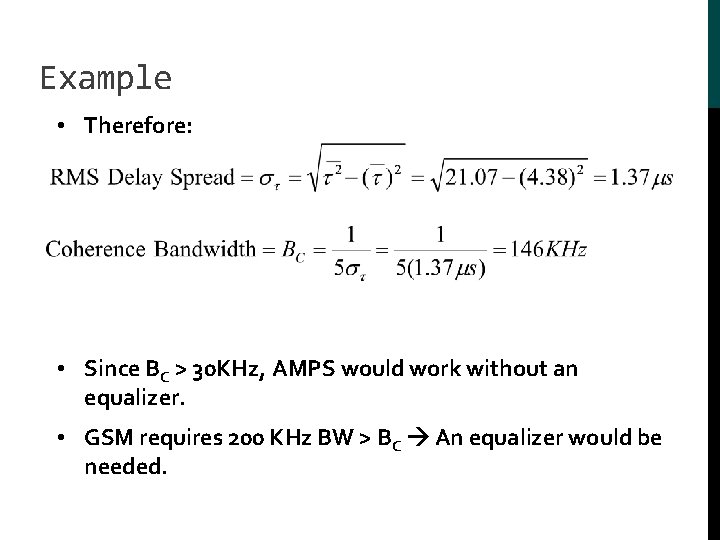
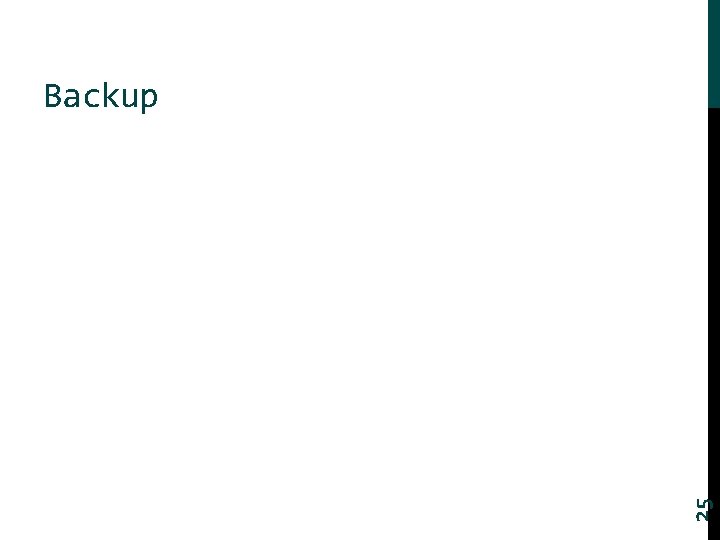
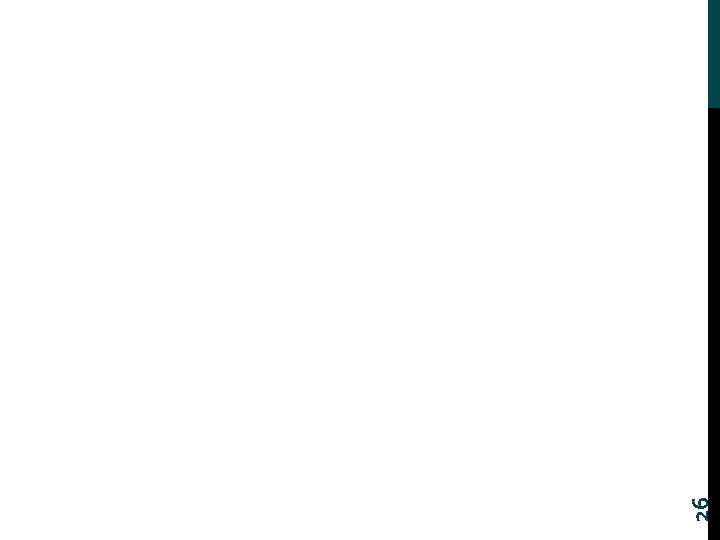
- Slides: 26
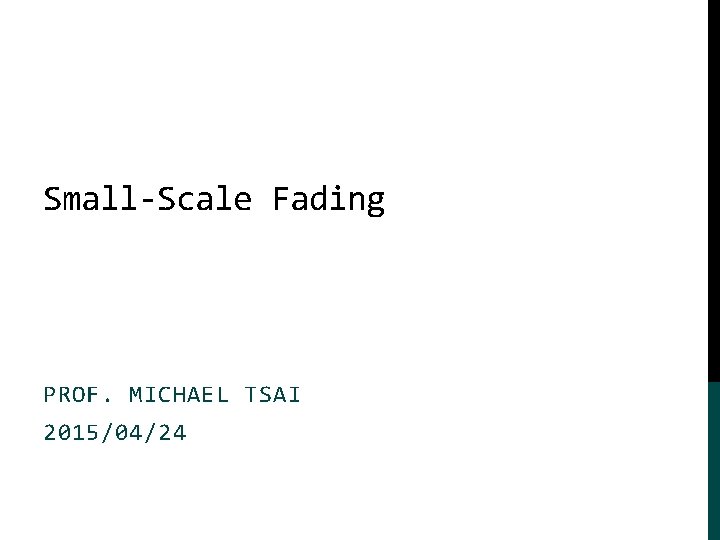
Small-Scale Fading PROF. MICHAEL TSAI 2015/04/24
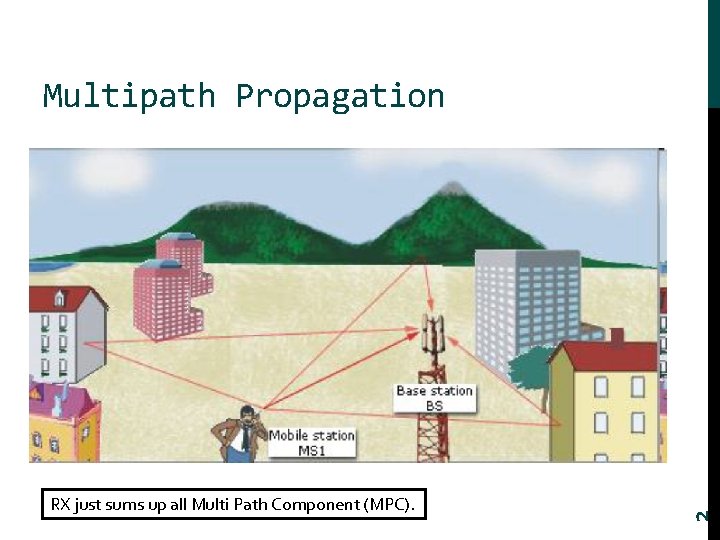
RX just sums up all Multi Path Component (MPC). 2 Multipath Propagation
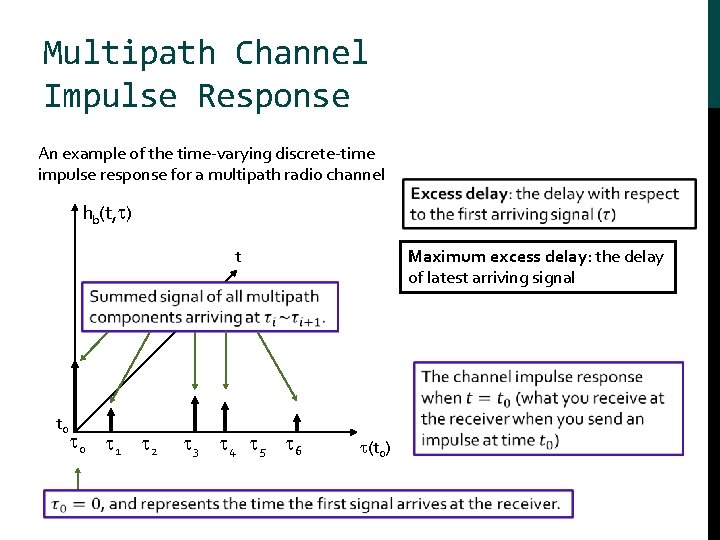
Multipath Channel Impulse Response An example of the time-varying discrete-time impulse response for a multipath radio channel hb(t, ) Maximum excess delay: the delay of latest arriving signal t t 0 0 1 2 3 4 5 6 (t 0)
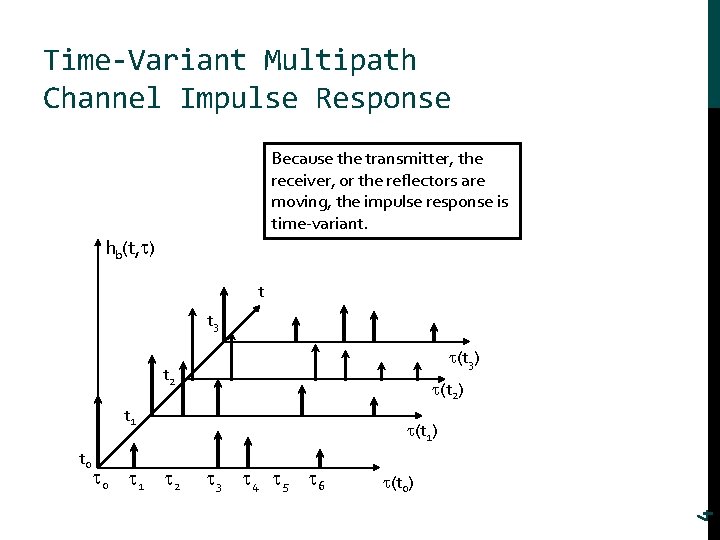
Time-Variant Multipath Channel Impulse Response Because the transmitter, the receiver, or the reflectors are moving, the impulse response is time-variant. hb(t, ) t t 3 (t 3) t 2 (t 2) t 1 0 1 2 3 4 5 6 (t 0) 4 t 0 (t 1)
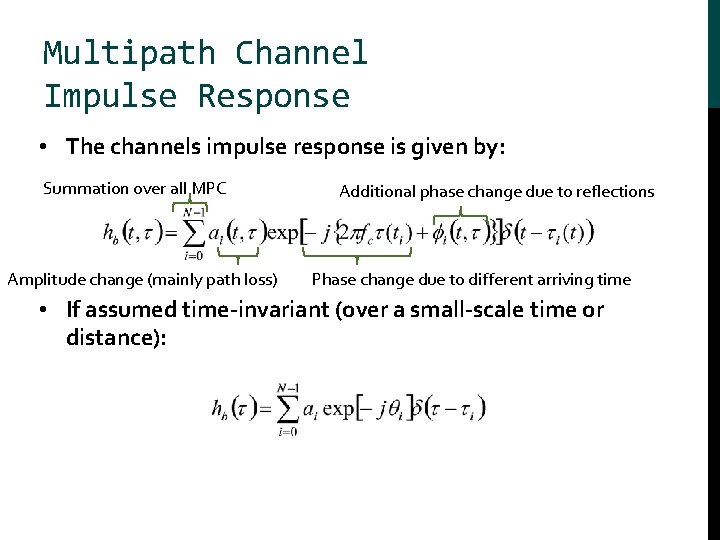
Multipath Channel Impulse Response • The channels impulse response is given by: Summation over all MPC Amplitude change (mainly path loss) Additional phase change due to reflections Phase change due to different arriving time • If assumed time-invariant (over a small-scale time or distance):
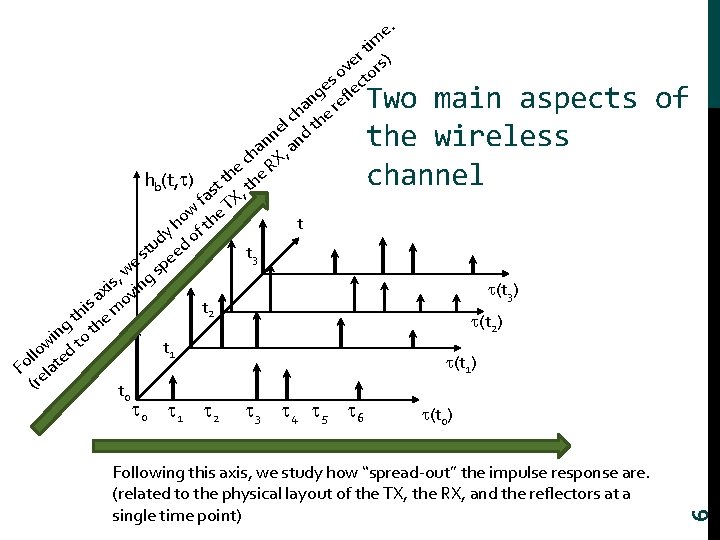
e. im t er rs) v o to s e lec g n ref a ch the l ne nd n ha X, a c e R hb(t, ) st th the fa TX, ow the t h f y o d tu ed t 3 s e e p w gs , s xi vin a is mo t 2 h t e ng o th i w t t 1 lo ted l Fo ela (r t 0 Two main aspects of the wireless channel 1 2 3 4 5 6 (t 2) (t 1) (t 0) Following this axis, we study how “spread-out” the impulse response are. (related to the physical layout of the TX, the RX, and the reflectors at a single time point) 6 0 (t 3)
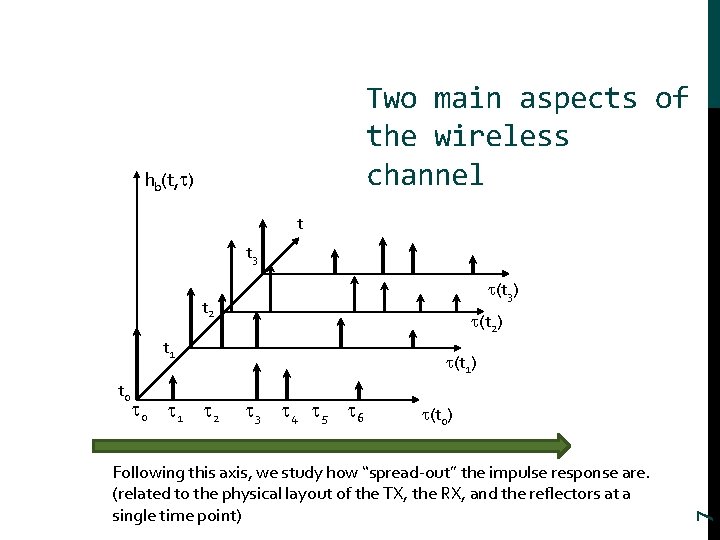
Two main aspects of the wireless channel hb(t, ) t t 3 (t 3) t 2 (t 2) t 1 0 1 2 3 4 5 6 (t 0) Following this axis, we study how “spread-out” the impulse response are. (related to the physical layout of the TX, the RX, and the reflectors at a single time point) 7 t 0 (t 1)
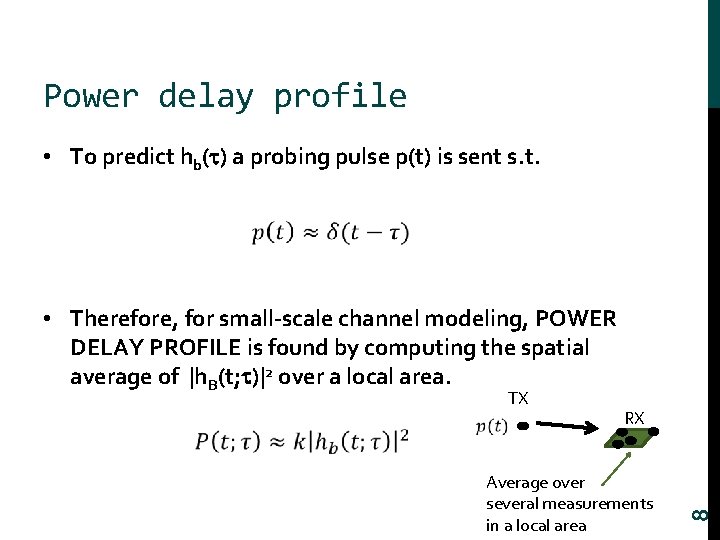
Power delay profile • To predict hb( ) a probing pulse p(t) is sent s. t. • Therefore, for small-scale channel modeling, POWER DELAY PROFILE is found by computing the spatial average of |h. B(t; )|2 over a local area. RX Average over several measurements in a local area 8 TX
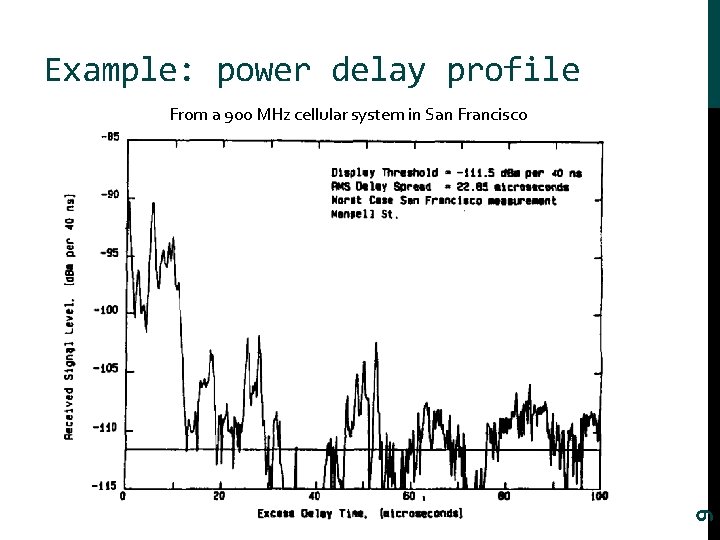
Example: power delay profile 9 From a 900 MHz cellular system in San Francisco
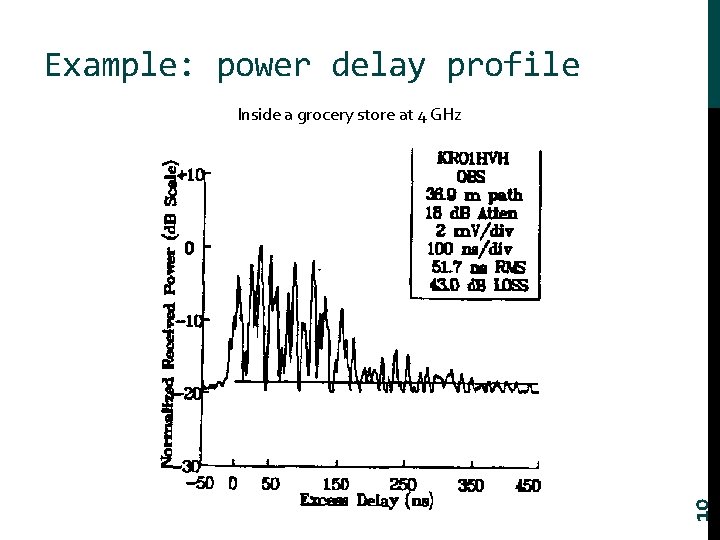
Example: power delay profile 10 Inside a grocery store at 4 GHz
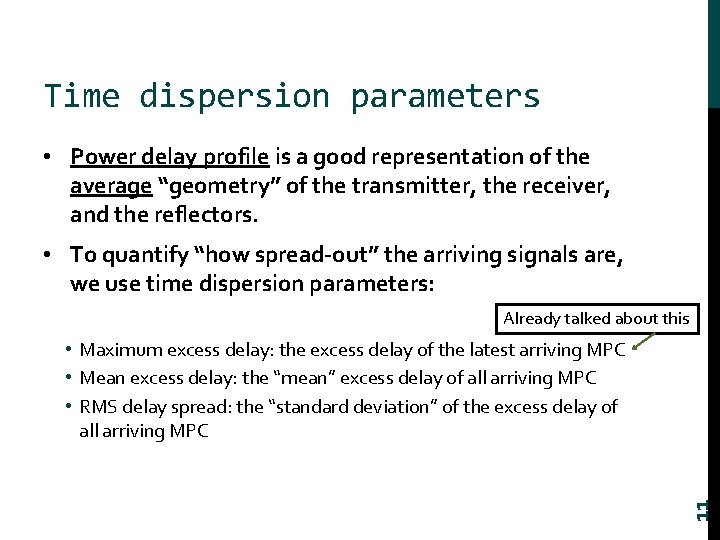
Time dispersion parameters • Power delay profile is a good representation of the average “geometry” of the transmitter, the receiver, and the reflectors. • To quantify “how spread-out” the arriving signals are, we use time dispersion parameters: Already talked about this 11 • Maximum excess delay: the excess delay of the latest arriving MPC • Mean excess delay: the “mean” excess delay of all arriving MPC • RMS delay spread: the “standard deviation” of the excess delay of all arriving MPC
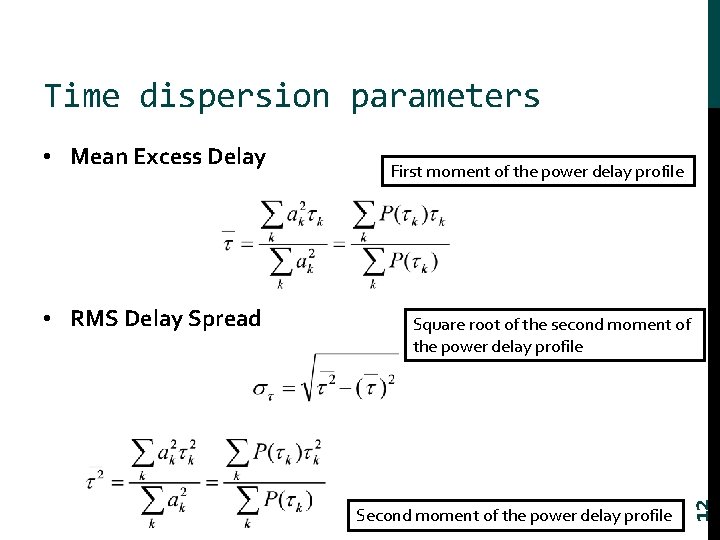
Time dispersion parameters • RMS Delay Spread First moment of the power delay profile Square root of the second moment of the power delay profile Second moment of the power delay profile 12 • Mean Excess Delay
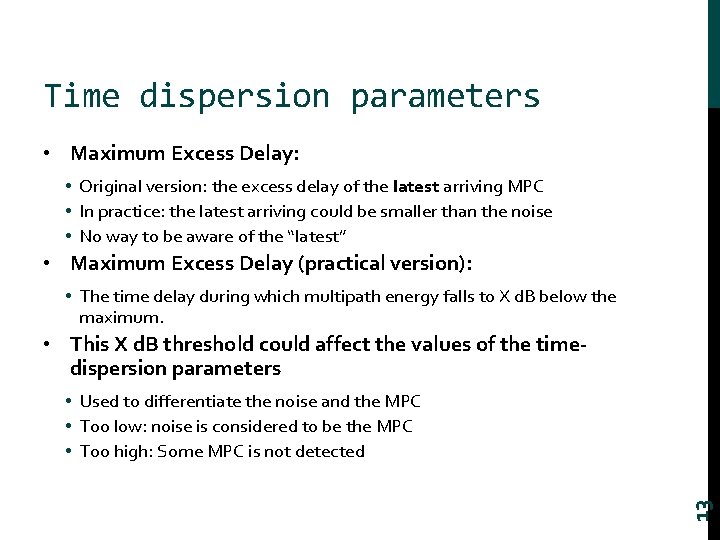
Time dispersion parameters • Maximum Excess Delay: • Original version: the excess delay of the latest arriving MPC • In practice: the latest arriving could be smaller than the noise • No way to be aware of the “latest” • Maximum Excess Delay (practical version): • The time delay during which multipath energy falls to X d. B below the maximum. • This X d. B threshold could affect the values of the timedispersion parameters 13 • Used to differentiate the noise and the MPC • Too low: noise is considered to be the MPC • Too high: Some MPC is not detected
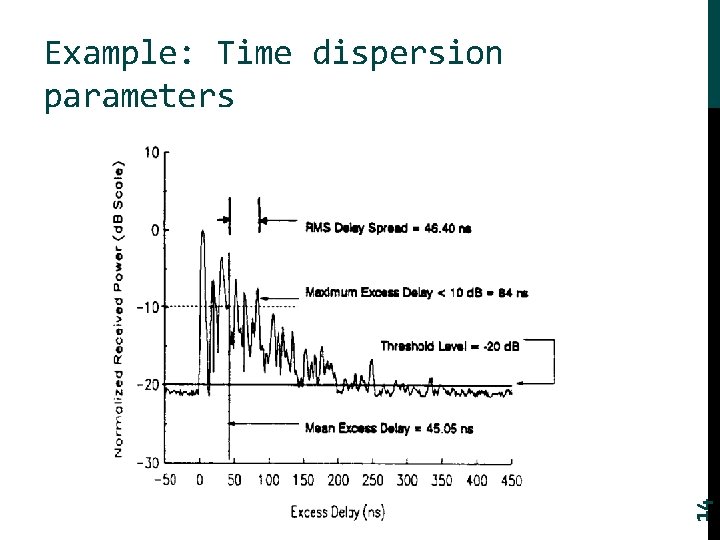
14 Example: Time dispersion parameters
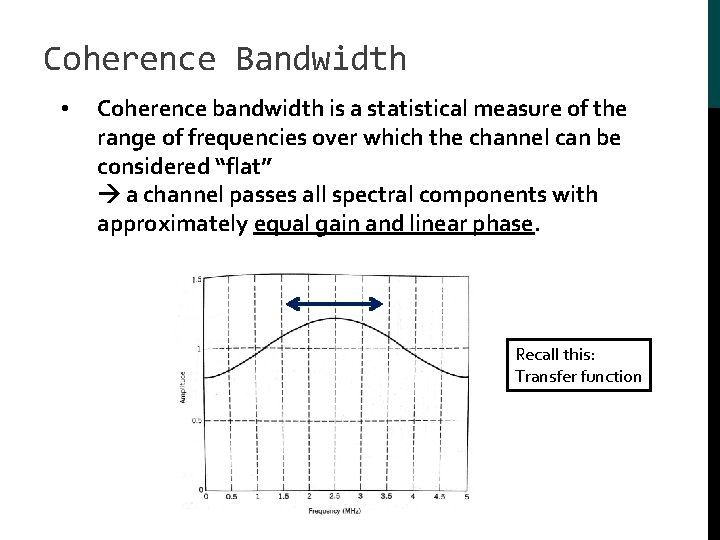
Coherence Bandwidth • Coherence bandwidth is a statistical measure of the range of frequencies over which the channel can be considered “flat” a channel passes all spectral components with approximately equal gain and linear phase. Recall this: Transfer function
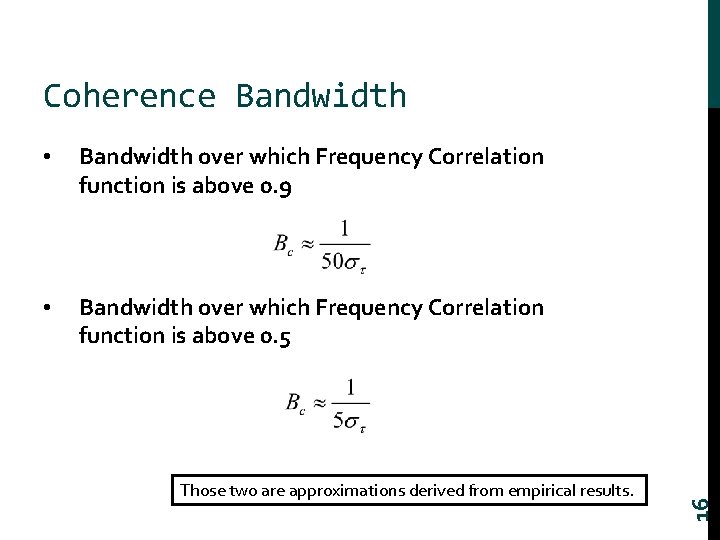
• Bandwidth over which Frequency Correlation function is above 0. 9 • Bandwidth over which Frequency Correlation function is above 0. 5 Those two are approximations derived from empirical results. 16 Coherence Bandwidth
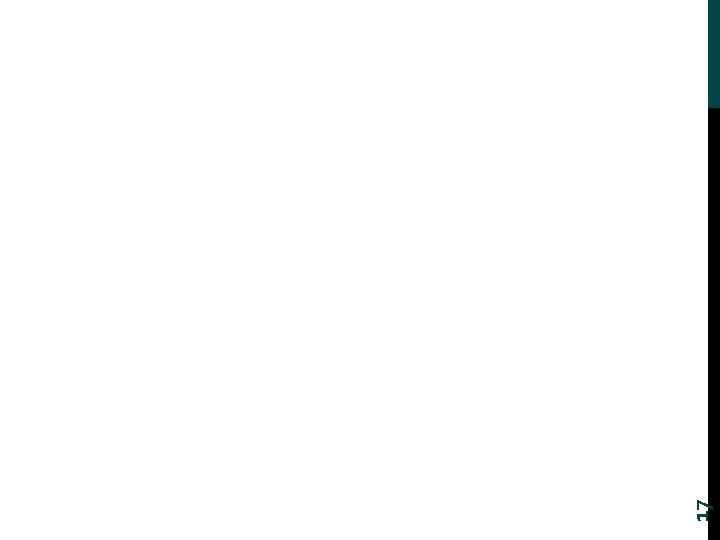
17
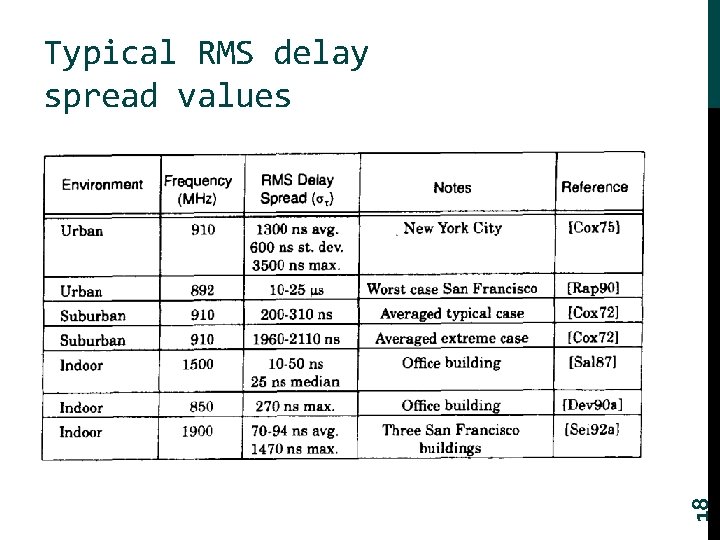
18 Typical RMS delay spread values
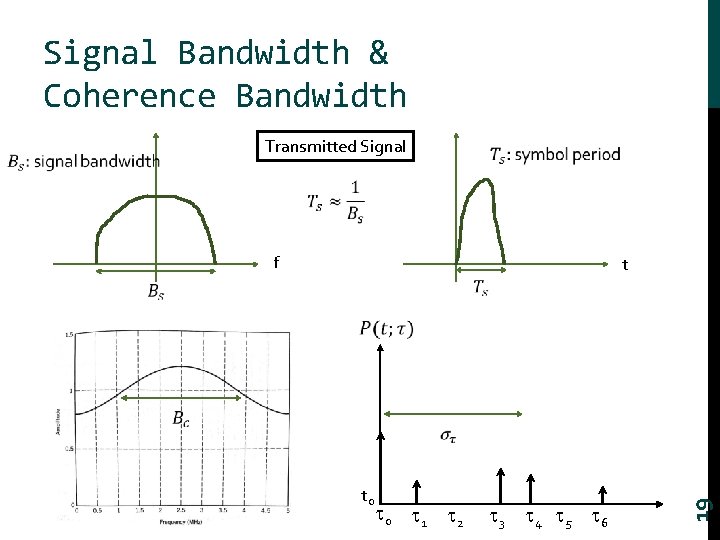
Signal Bandwidth & Coherence Bandwidth Transmitted Signal f t 0 0 1 2 3 4 5 6 19 t
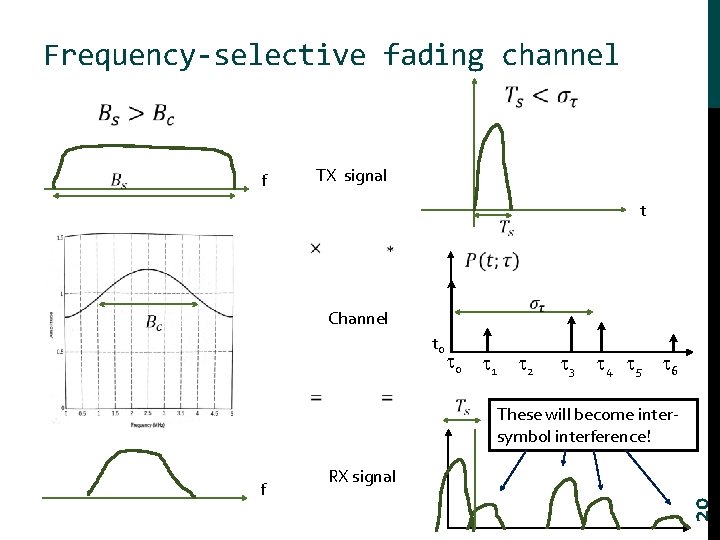
Frequency-selective fading channel f TX signal t Channel t 0 0 1 2 3 4 5 6 These will become intersymbol interference! RX signal 20 f
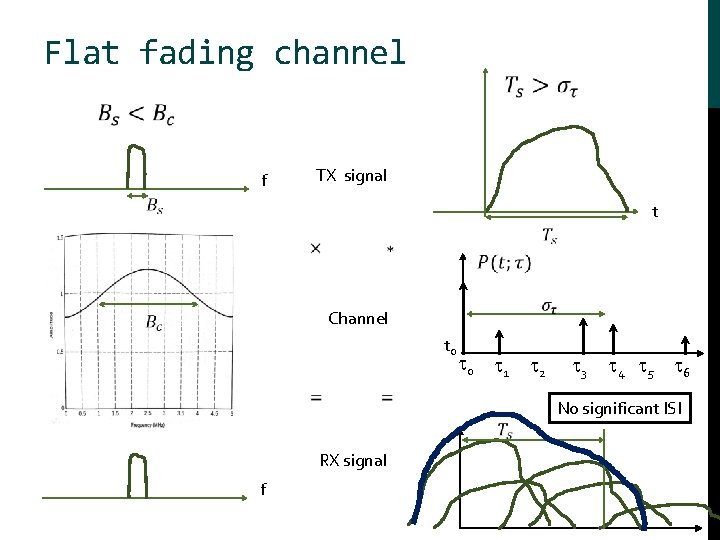
Flat fading channel f TX signal t Channel t 0 0 1 2 3 4 5 6 No significant ISI RX signal f
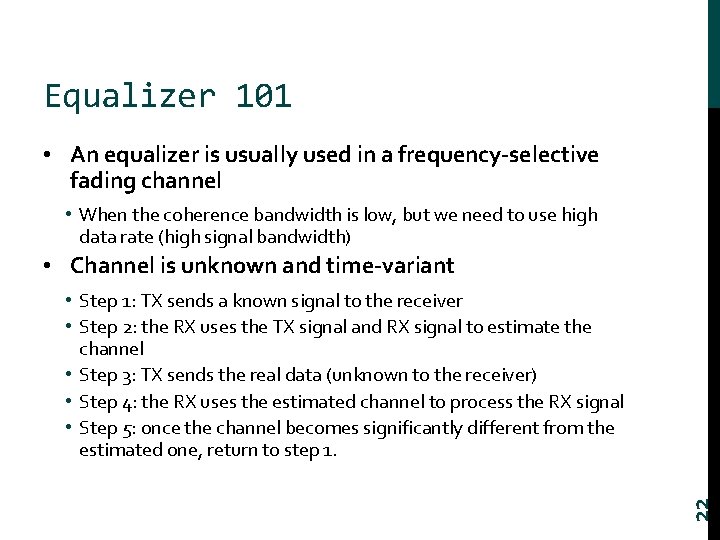
Equalizer 101 • An equalizer is usually used in a frequency-selective fading channel • When the coherence bandwidth is low, but we need to use high data rate (high signal bandwidth) • Channel is unknown and time-variant 22 • Step 1: TX sends a known signal to the receiver • Step 2: the RX uses the TX signal and RX signal to estimate the channel • Step 3: TX sends the real data (unknown to the receiver) • Step 4: the RX uses the estimated channel to process the RX signal • Step 5: once the channel becomes significantly different from the estimated one, return to step 1.
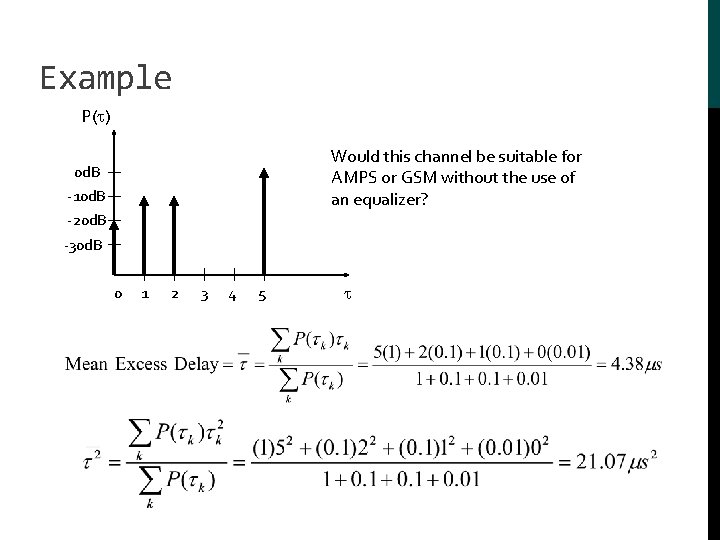
Example P( ) Would this channel be suitable for AMPS or GSM without the use of an equalizer? 0 d. B -10 d. B -20 d. B -30 d. B 0 1 2 3 4 5
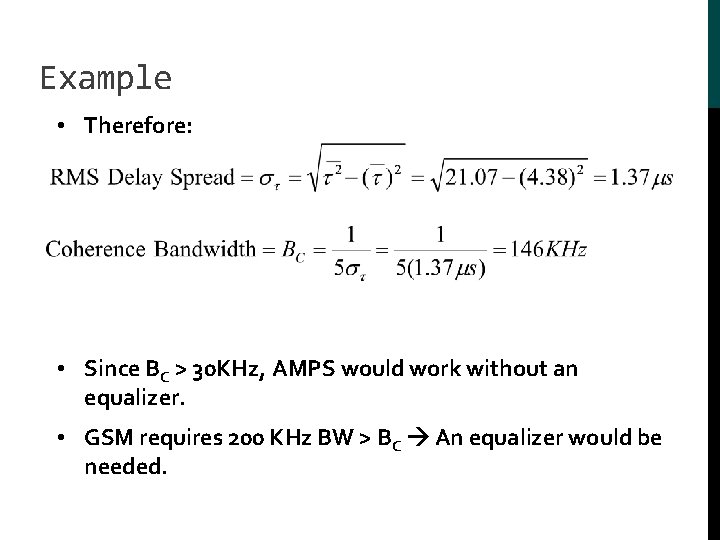
Example • Therefore: • Since BC > 30 KHz, AMPS would work without an equalizer. • GSM requires 200 KHz BW > BC An equalizer would be needed.
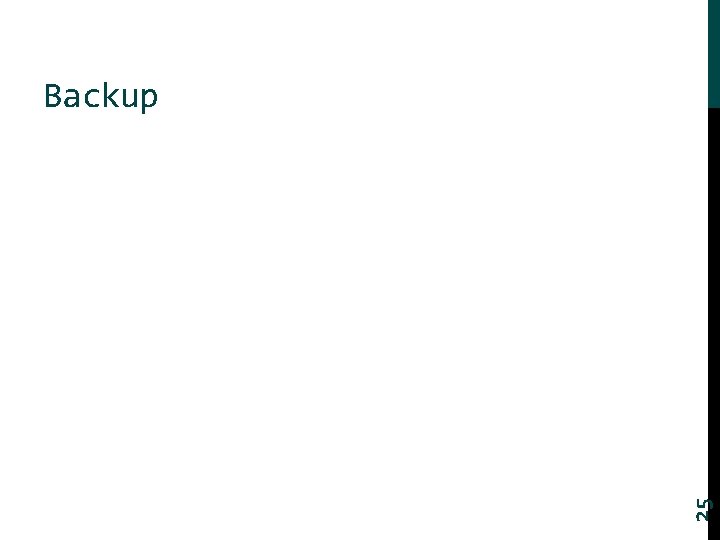
25 Backup
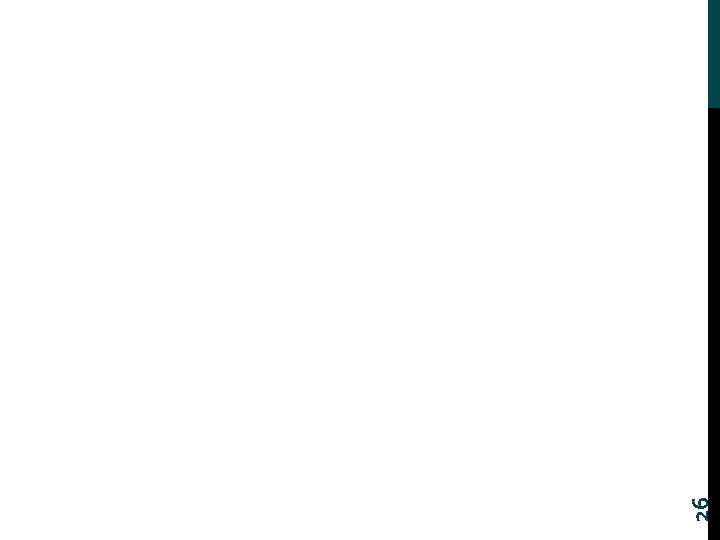
26
Types of small scale fading
Not because of who i am
Kinetics of crystal violet fading
Narayan mandayam
Henry peach robinson fading away
Philip larkin summer is fading
Fading
Narrow band fading
Fading psicologia
Small scale fading adalah
Henry peach robinson fading away
Large scale fading in wireless communication
Splunk ftr
Shannon tsai
Robin tsai
Introduction to snmp
Beth tsai
Charlene tsai
Fast radio burst
What does effective communication look like
Eddie tsai
Chin-chung tsai
Wen-hsuan tsai
Jerry tsai
Which two characters are detained by the watchmen
Professor michael nagel
Professor michael woodward