Further Results of SoftInplane Tiltrotor Aeromechanics Investigation Using
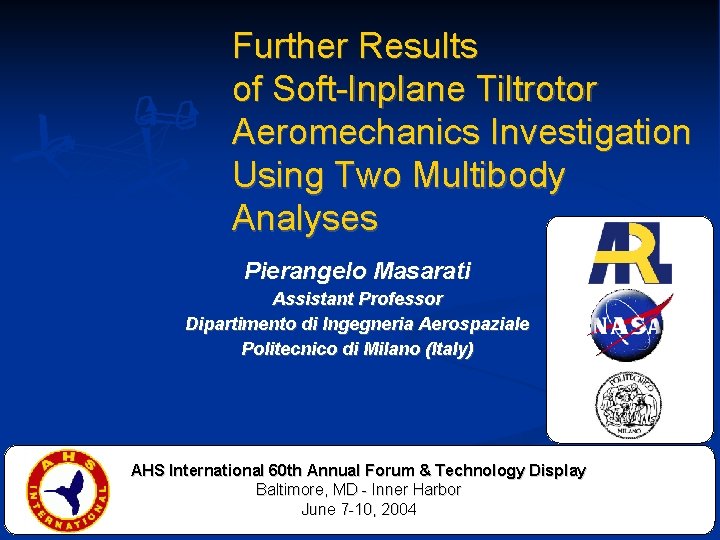
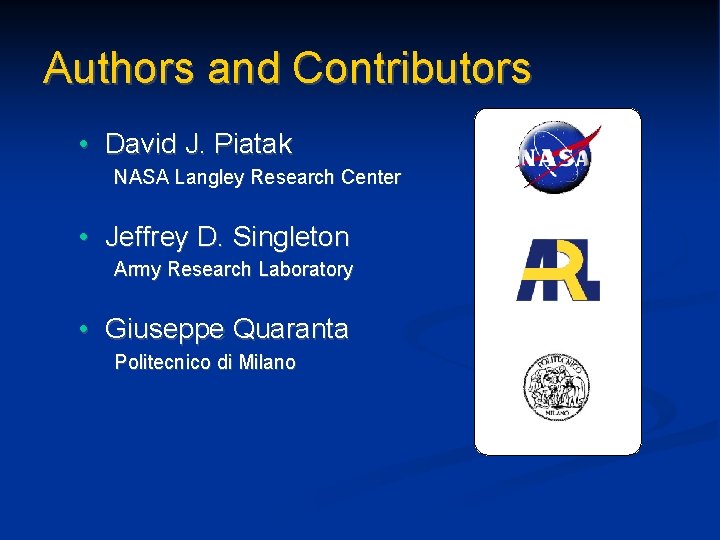
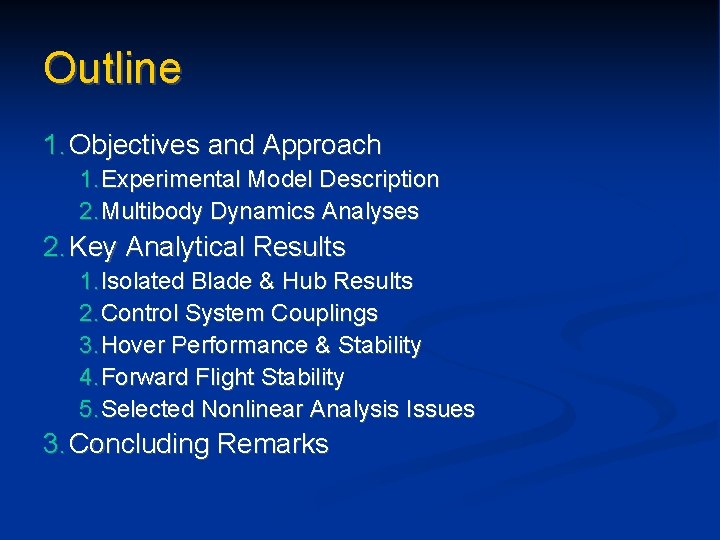
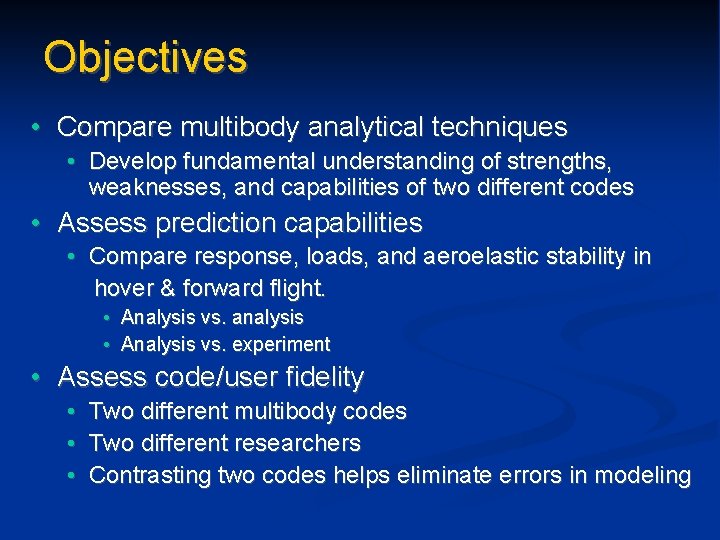
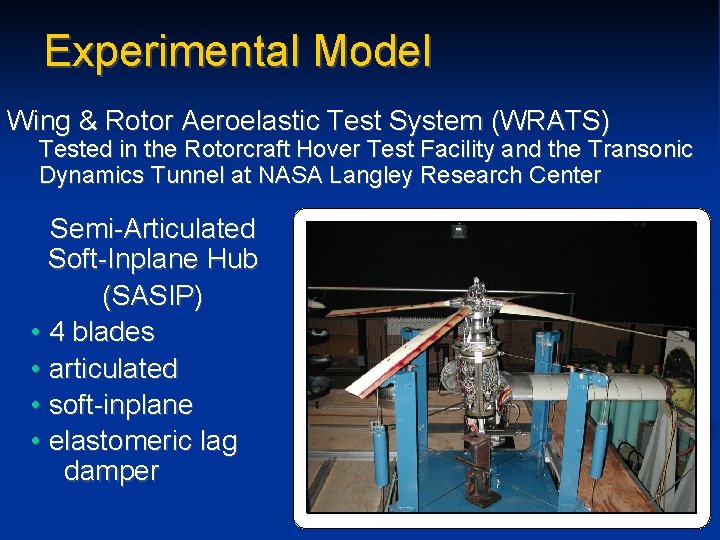
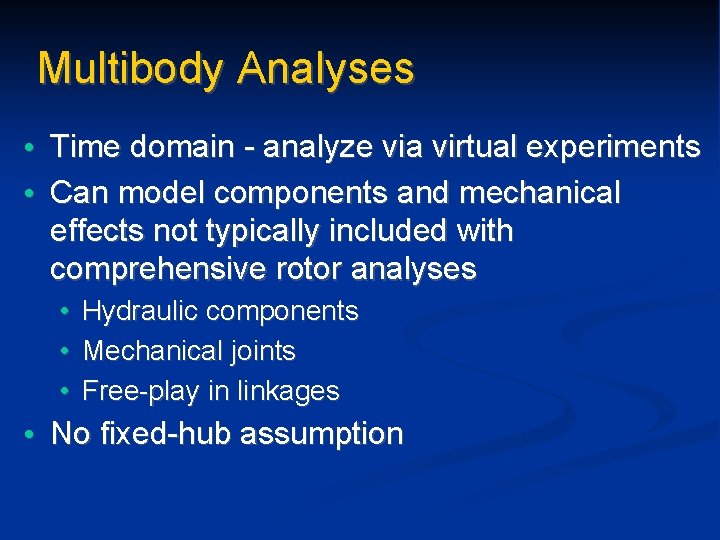
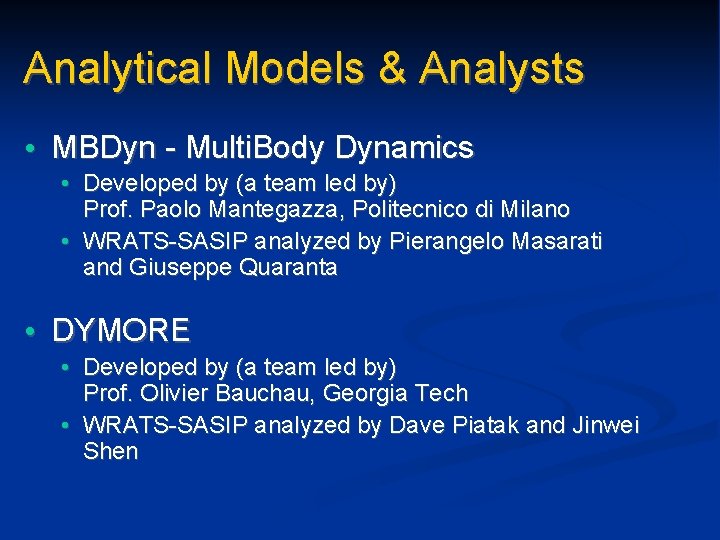
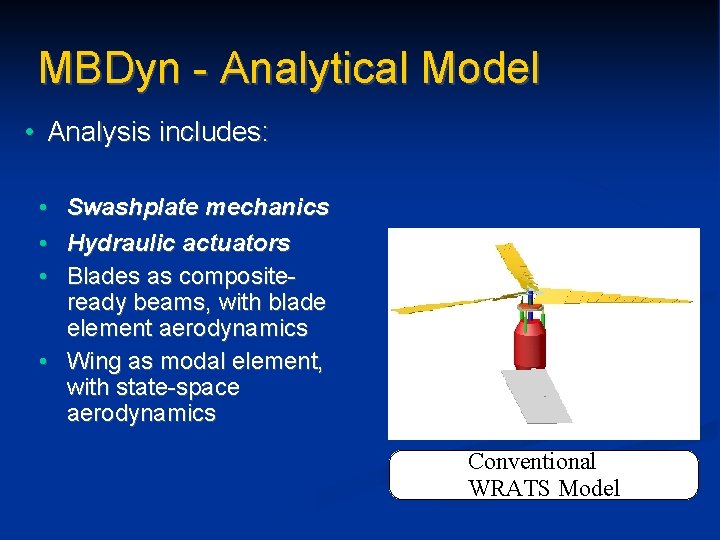
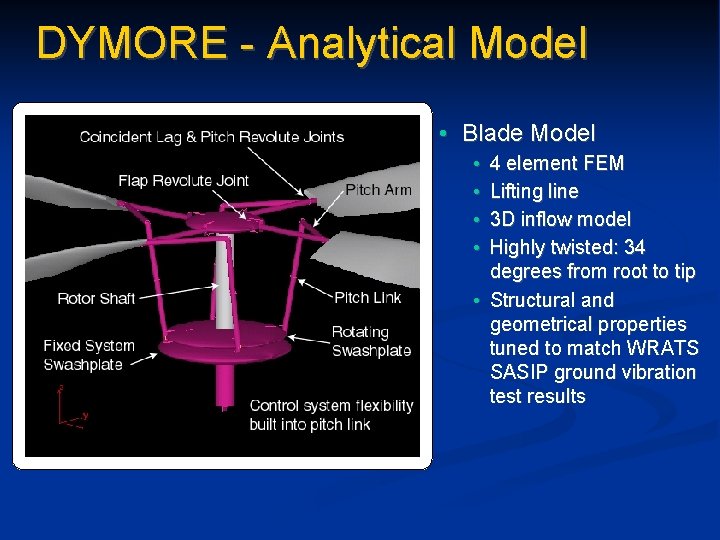
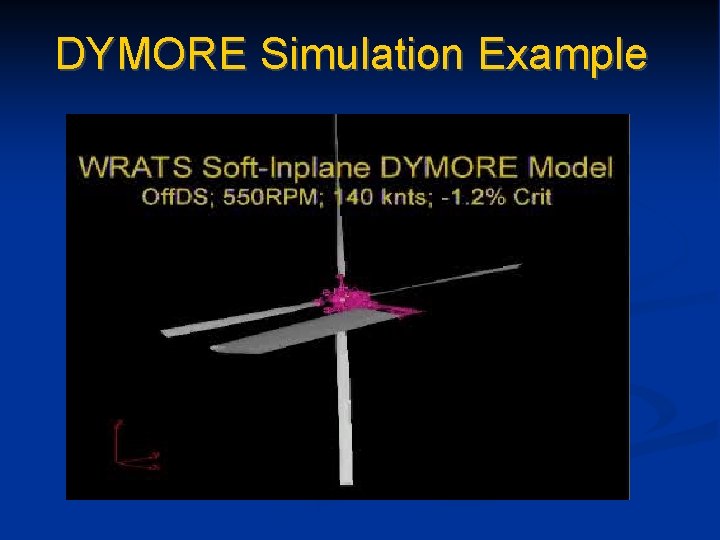
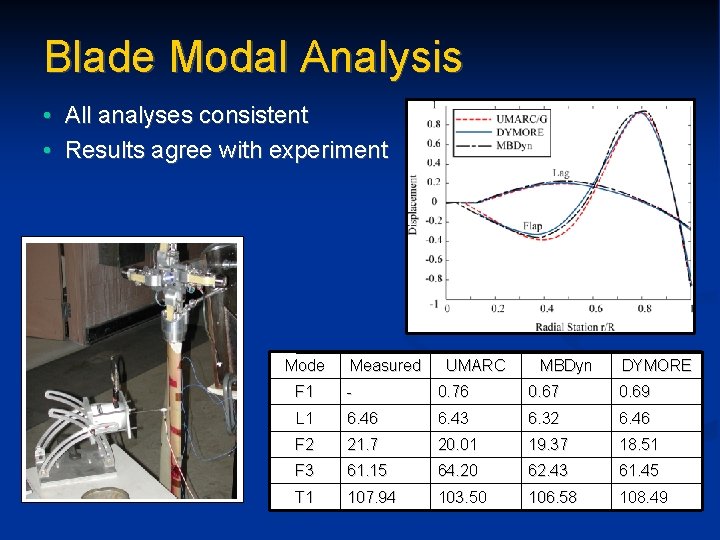
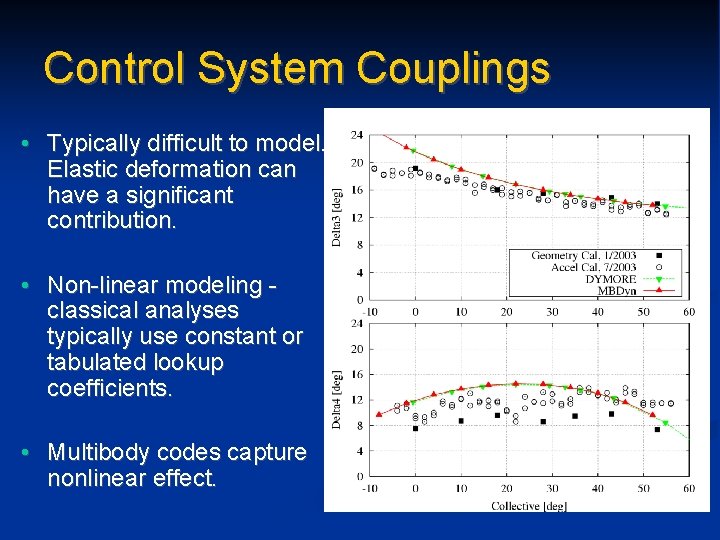
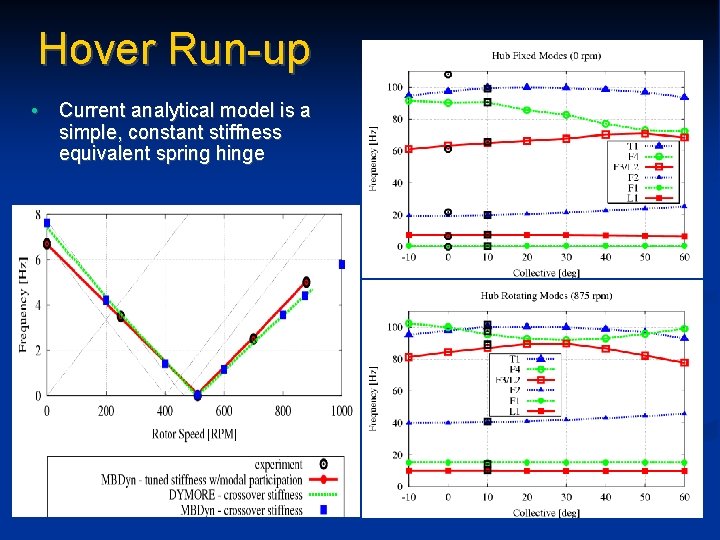
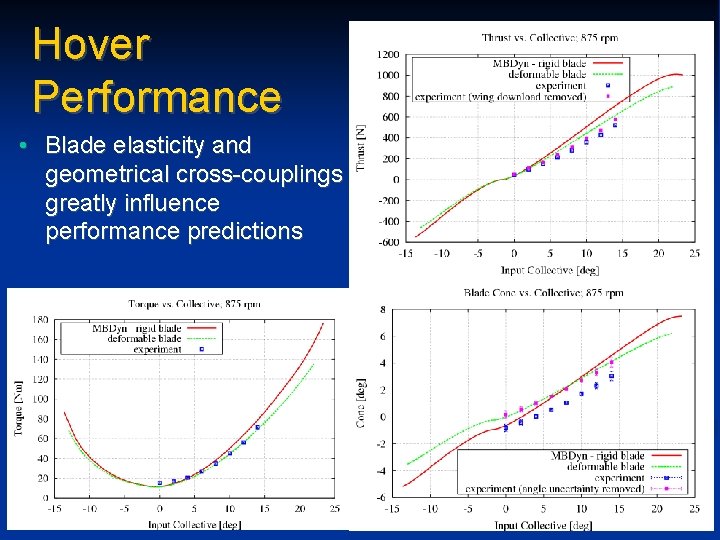
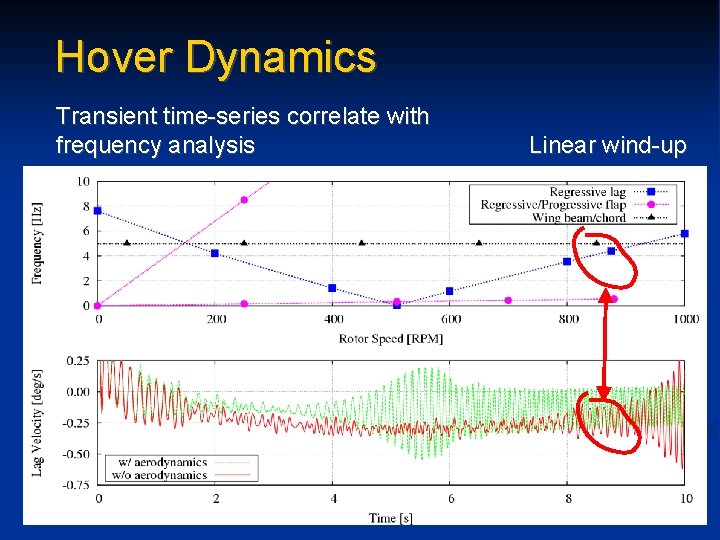
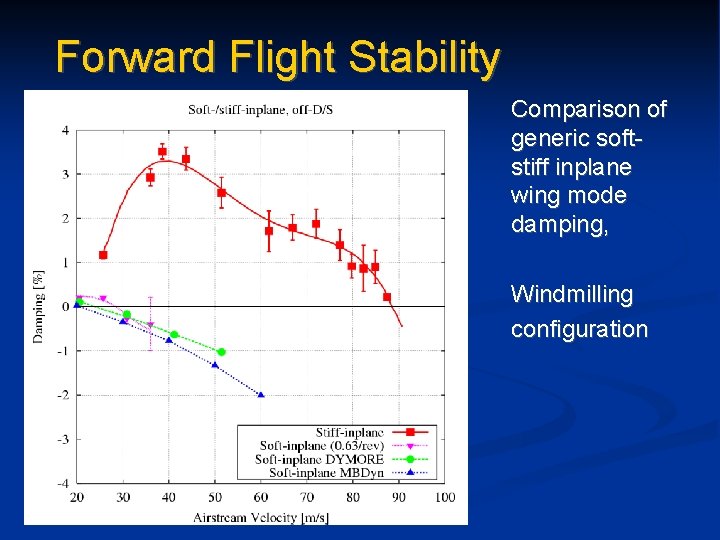
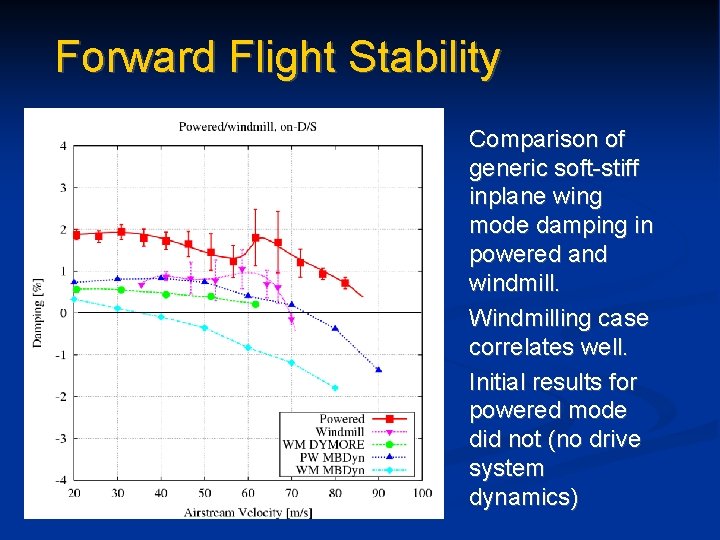
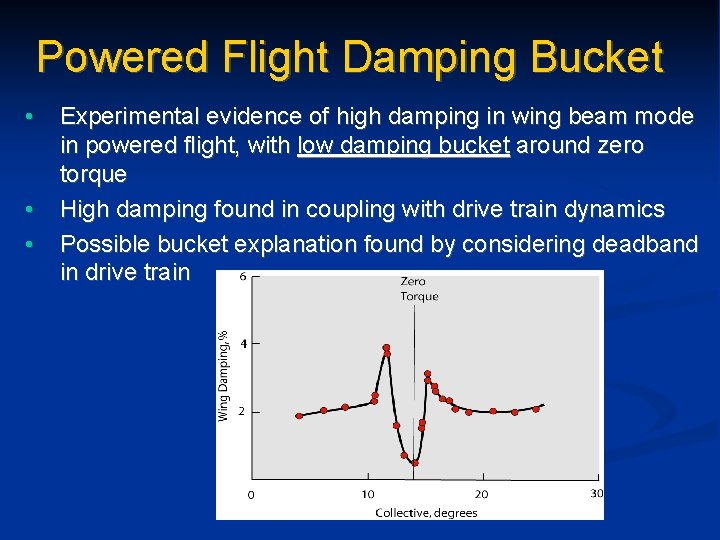
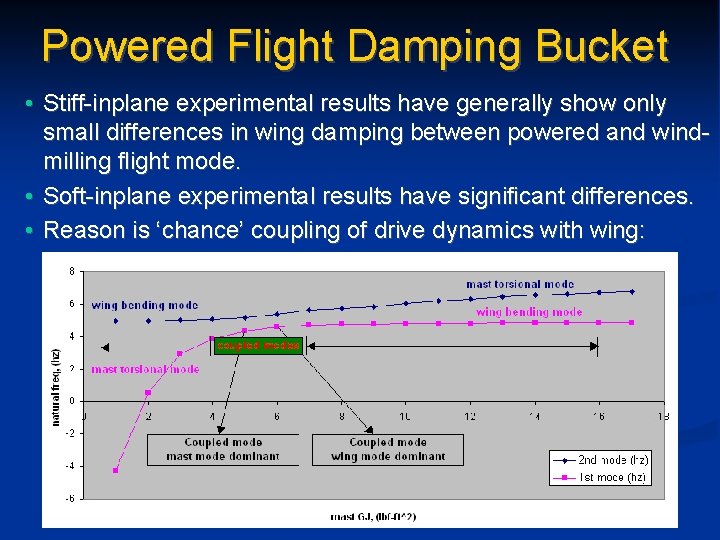
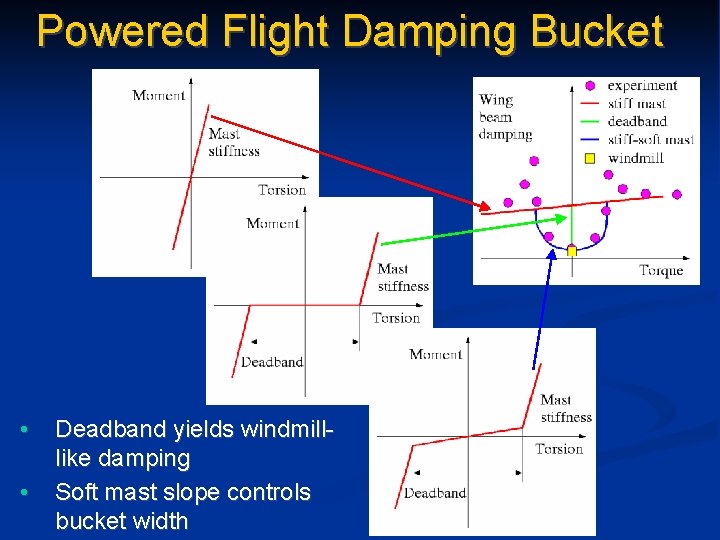
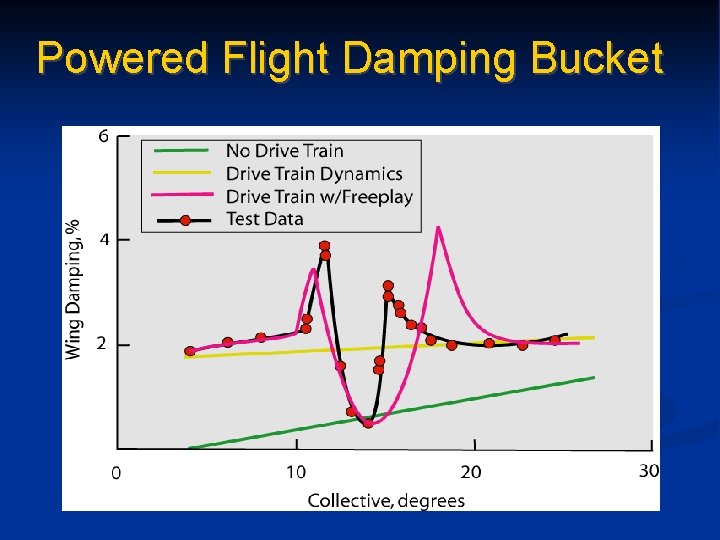
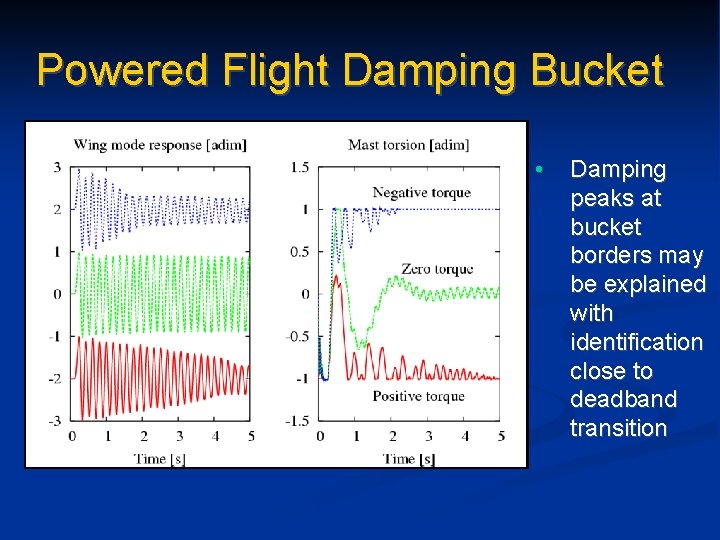
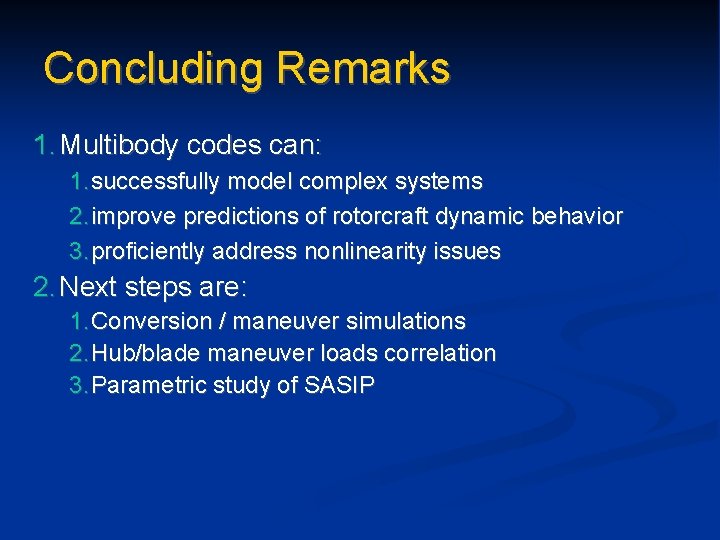
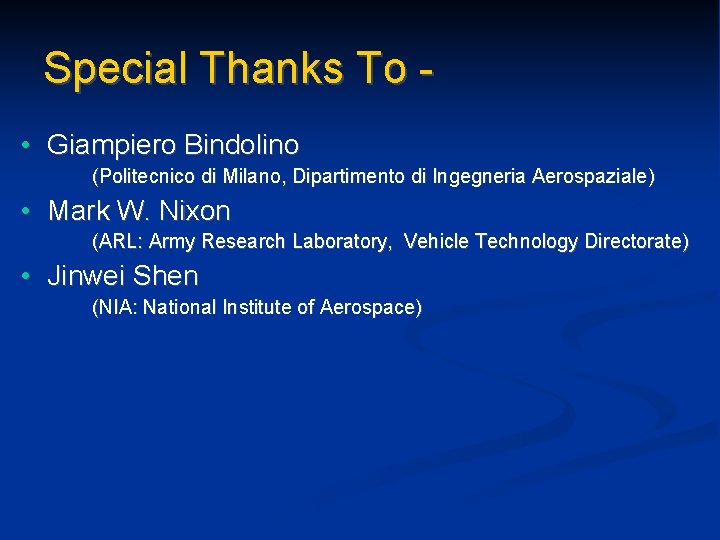
- Slides: 24
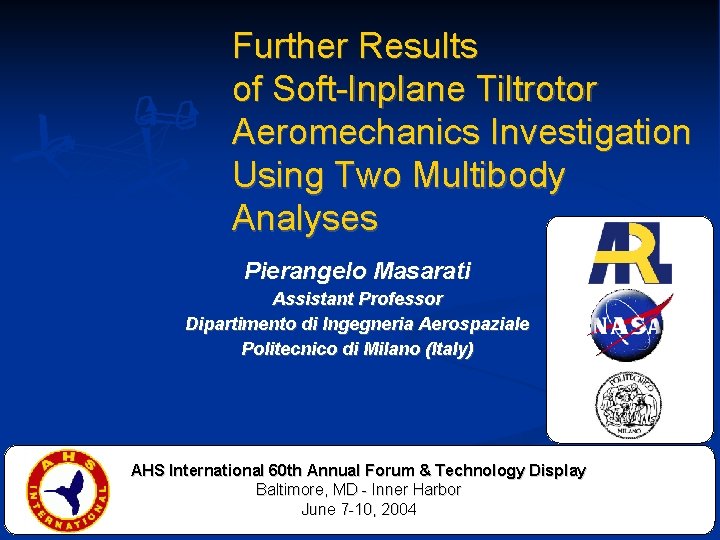
Further Results of Soft-Inplane Tiltrotor Aeromechanics Investigation Using Two Multibody Analyses Pierangelo Masarati Assistant Professor Dipartimento di Ingegneria Aerospaziale Politecnico di Milano (Italy) AHS International 60 th Annual Forum & Technology Display Baltimore, MD - Inner Harbor June 7 -10, 2004
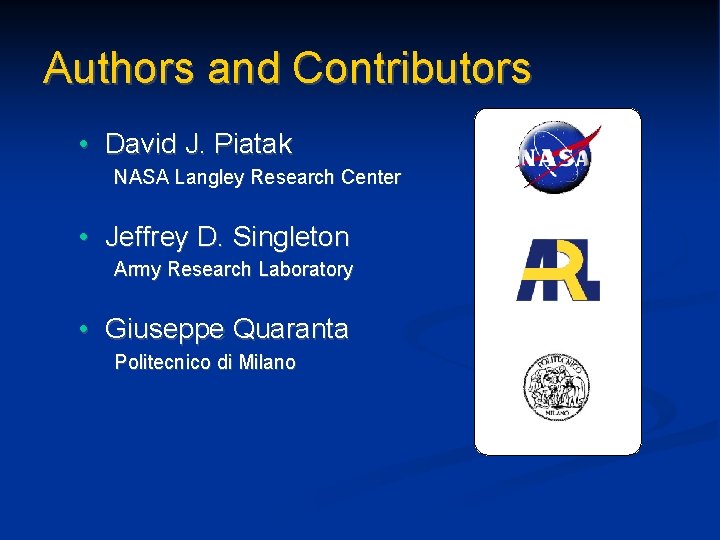
Authors and Contributors • David J. Piatak NASA Langley Research Center • Jeffrey D. Singleton Army Research Laboratory • Giuseppe Quaranta Politecnico di Milano
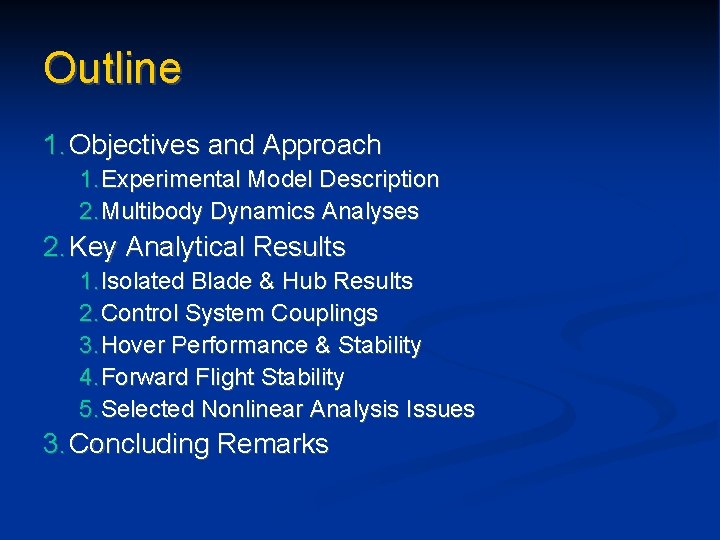
Outline 1. Objectives and Approach 1. Experimental Model Description 2. Multibody Dynamics Analyses 2. Key Analytical Results 1. Isolated Blade & Hub Results 2. Control System Couplings 3. Hover Performance & Stability 4. Forward Flight Stability 5. Selected Nonlinear Analysis Issues 3. Concluding Remarks
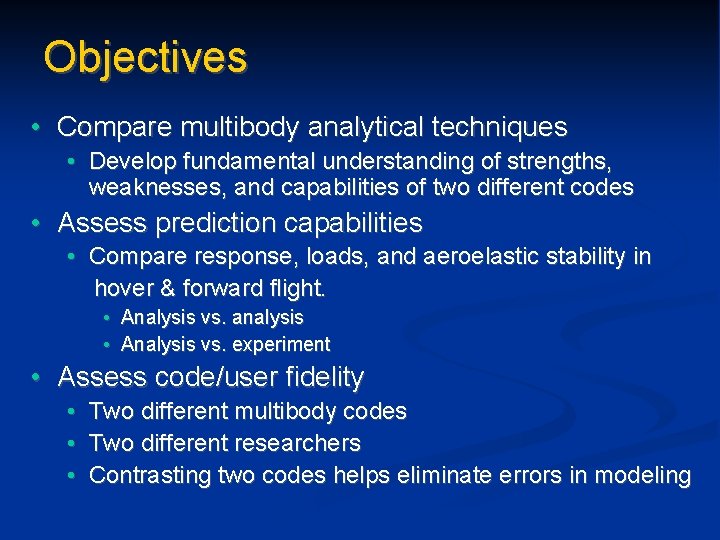
Objectives • Compare multibody analytical techniques • Develop fundamental understanding of strengths, weaknesses, and capabilities of two different codes • Assess prediction capabilities • Compare response, loads, and aeroelastic stability in hover & forward flight. • Analysis vs. analysis • Analysis vs. experiment • Assess code/user fidelity • • • Two different multibody codes Two different researchers Contrasting two codes helps eliminate errors in modeling
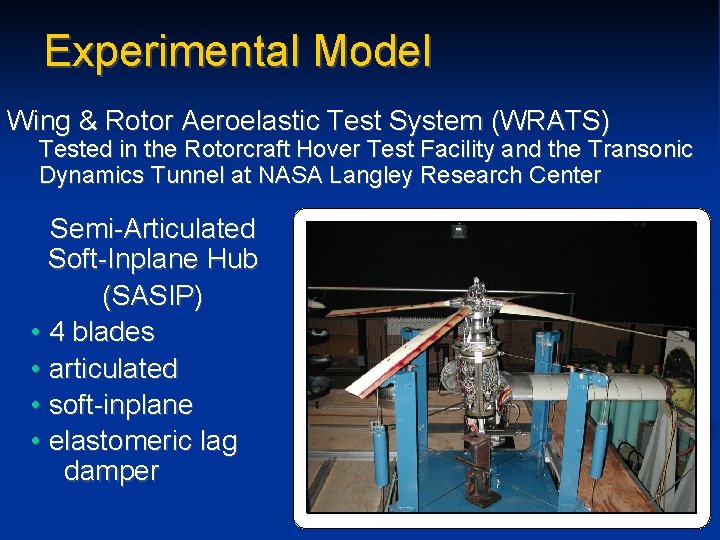
Experimental Model Wing & Rotor Aeroelastic Test System (WRATS) Tested in the Rotorcraft Hover Test Facility and the Transonic Dynamics Tunnel at NASA Langley Research Center Semi-Articulated Soft-Inplane Hub (SASIP) • 4 blades • articulated • soft-inplane • elastomeric lag damper
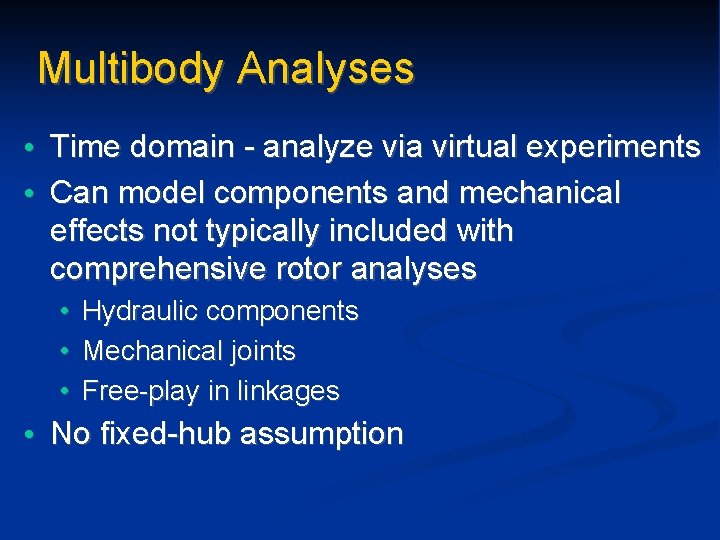
Multibody Analyses • Time domain - analyze via virtual experiments • Can model components and mechanical effects not typically included with comprehensive rotor analyses • • • Hydraulic components Mechanical joints Free-play in linkages • No fixed-hub assumption
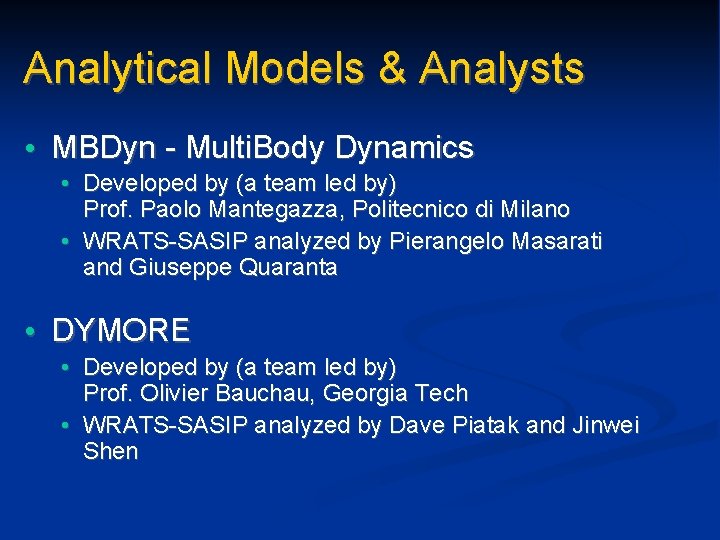
Analytical Models & Analysts • MBDyn - Multi. Body Dynamics • Developed by (a team led by) Prof. Paolo Mantegazza, Politecnico di Milano • WRATS-SASIP analyzed by Pierangelo Masarati and Giuseppe Quaranta • DYMORE • Developed by (a team led by) Prof. Olivier Bauchau, Georgia Tech • WRATS-SASIP analyzed by Dave Piatak and Jinwei Shen
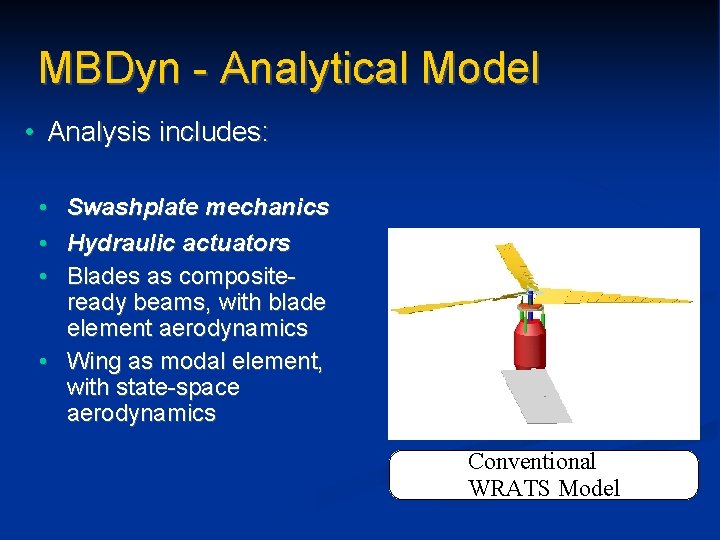
MBDyn - Analytical Model • Analysis includes: • Swashplate mechanics • Hydraulic actuators • Blades as compositeready beams, with blade element aerodynamics • Wing as modal element, with state-space aerodynamics Conventional WRATS Model
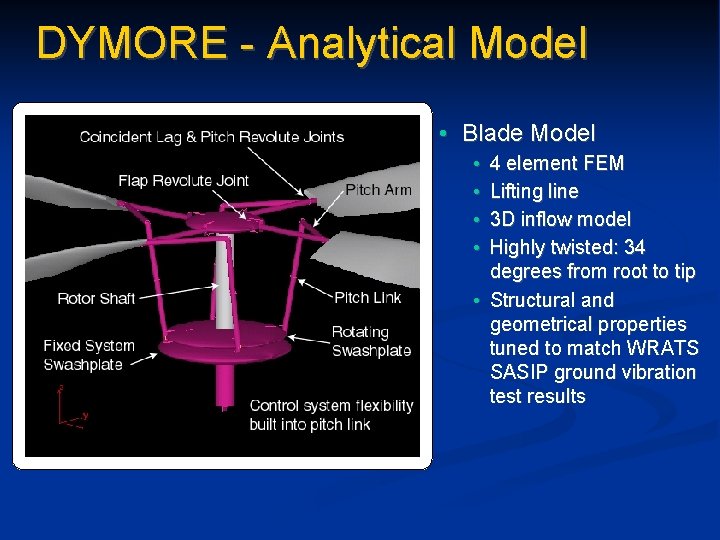
DYMORE - Analytical Model • Blade Model • • 4 element FEM Lifting line 3 D inflow model Highly twisted: 34 degrees from root to tip • Structural and geometrical properties tuned to match WRATS SASIP ground vibration test results
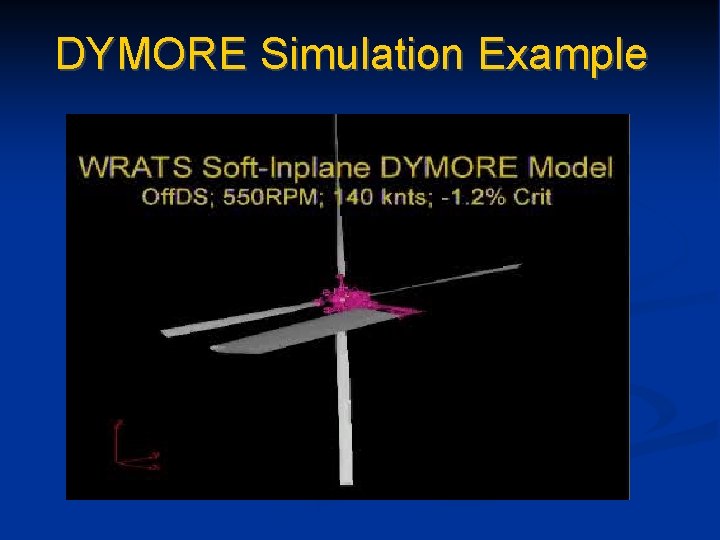
DYMORE Simulation Example
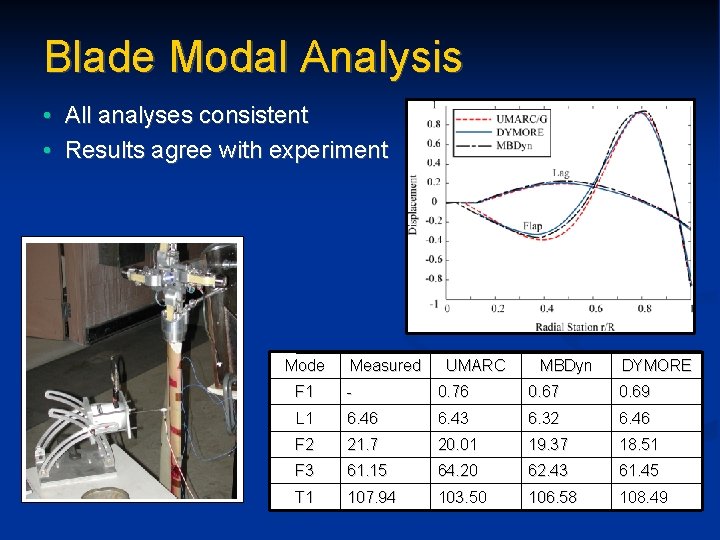
Blade Modal Analysis • All analyses consistent • Results agree with experiment Mode Measured UMARC MBDyn DYMORE F 1 - 0. 76 0. 67 0. 69 L 1 6. 46 6. 43 6. 32 6. 46 F 2 21. 7 20. 01 19. 37 18. 51 F 3 61. 15 64. 20 62. 43 61. 45 T 1 107. 94 103. 50 106. 58 108. 49
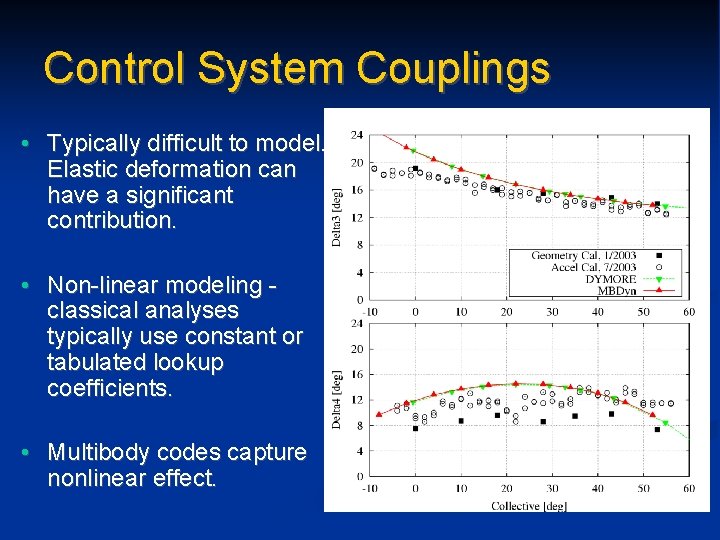
Control System Couplings • Typically difficult to model. Elastic deformation can have a significant contribution. • Non-linear modeling classical analyses typically use constant or tabulated lookup coefficients. • Multibody codes capture nonlinear effect.
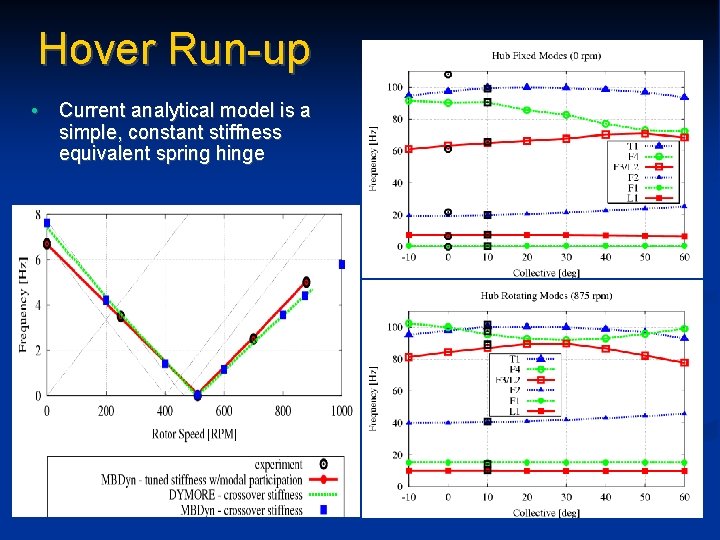
Hover Run-up • Current analytical model is a simple, constant stiffness equivalent spring hinge
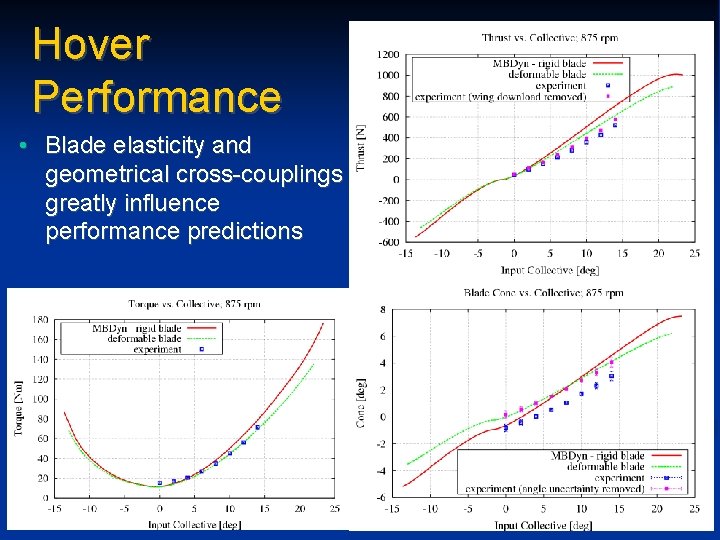
Hover Performance • Blade elasticity and geometrical cross-couplings greatly influence performance predictions
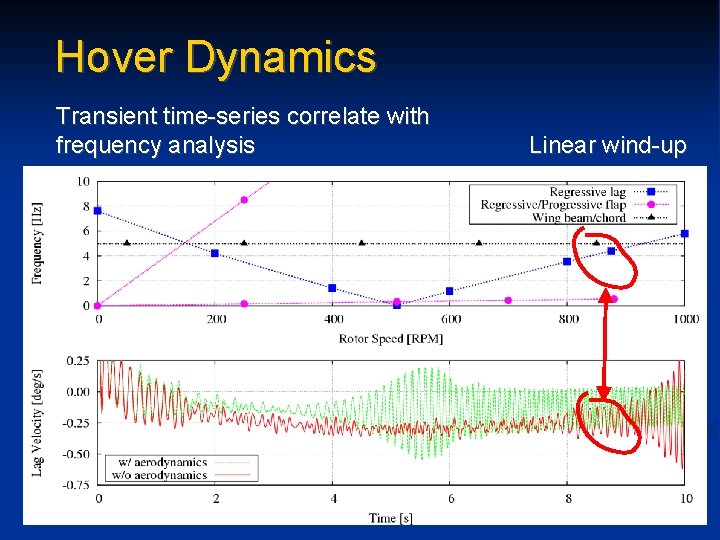
Hover Dynamics Transient time-series correlate with frequency analysis Linear wind-up
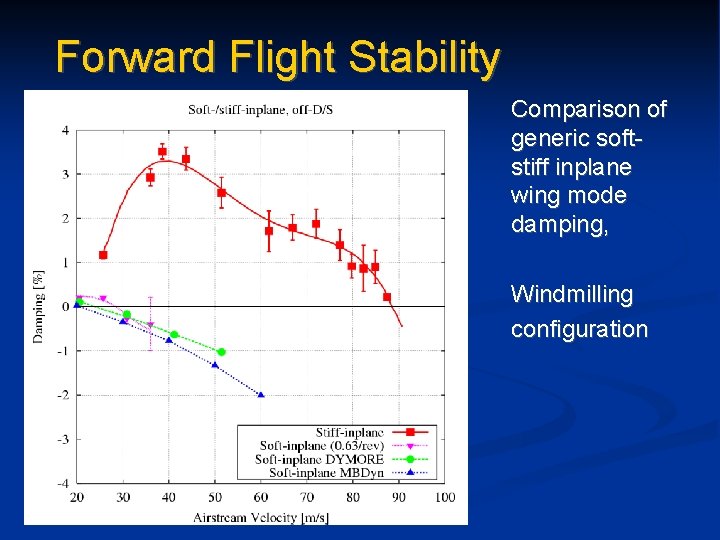
Forward Flight Stability Comparison of generic softstiff inplane wing mode damping, Windmilling configuration
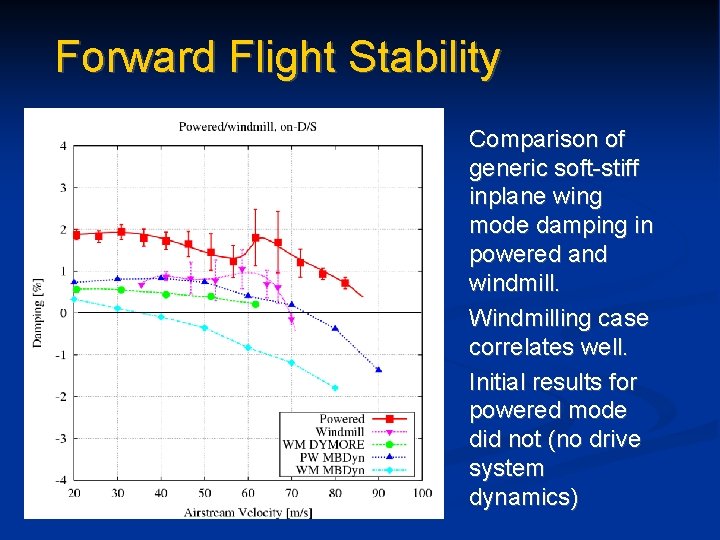
Forward Flight Stability Comparison of generic soft-stiff inplane wing mode damping in powered and windmill. Windmilling case correlates well. Initial results for powered mode did not (no drive system dynamics)
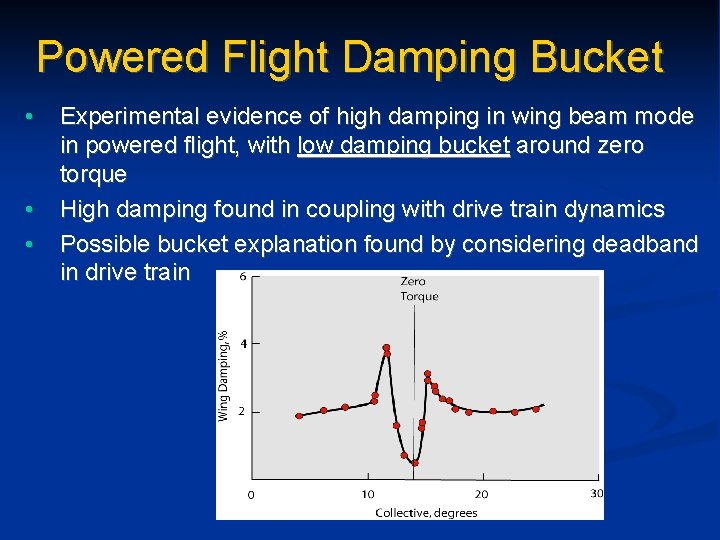
Powered Flight Damping Bucket • • • Experimental evidence of high damping in wing beam mode in powered flight, with low damping bucket around zero torque High damping found in coupling with drive train dynamics Possible bucket explanation found by considering deadband in drive train
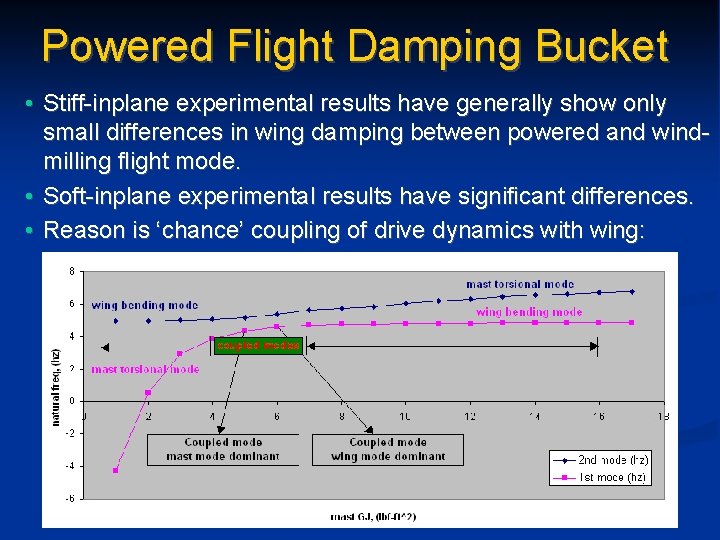
Powered Flight Damping Bucket • Stiff-inplane experimental results have generally show only small differences in wing damping between powered and windmilling flight mode. • Soft-inplane experimental results have significant differences. • Reason is ‘chance’ coupling of drive dynamics with wing:
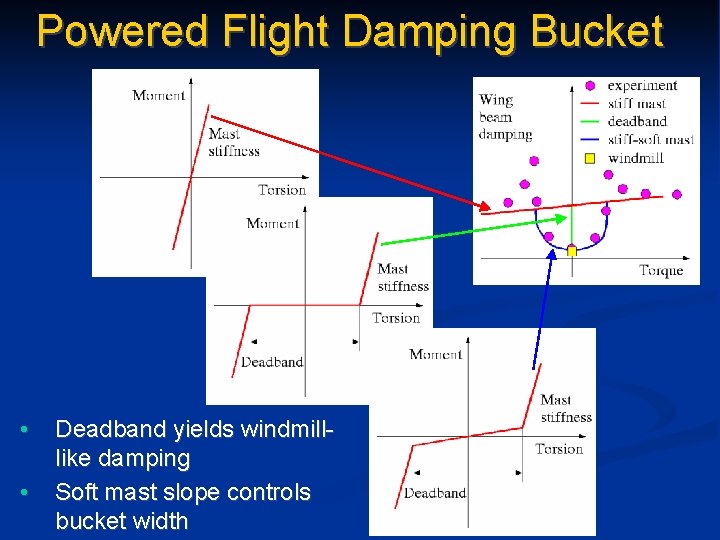
Powered Flight Damping Bucket • • Deadband yields windmilllike damping Soft mast slope controls bucket width
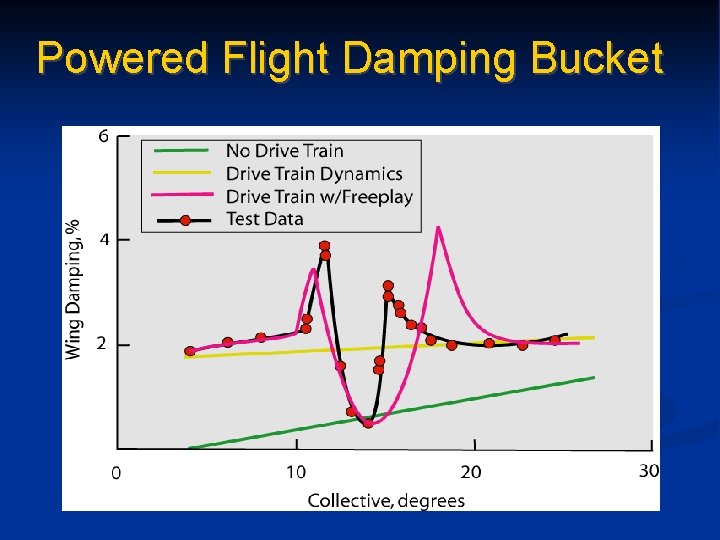
Powered Flight Damping Bucket
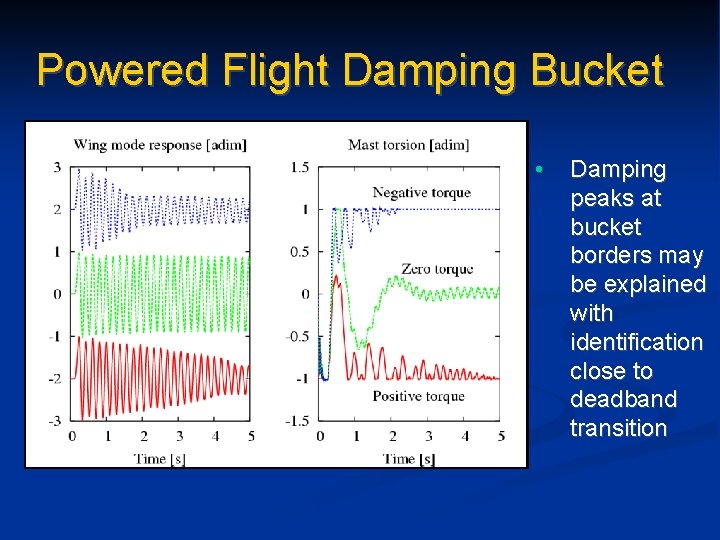
Powered Flight Damping Bucket • Damping peaks at bucket borders may be explained with identification close to deadband transition
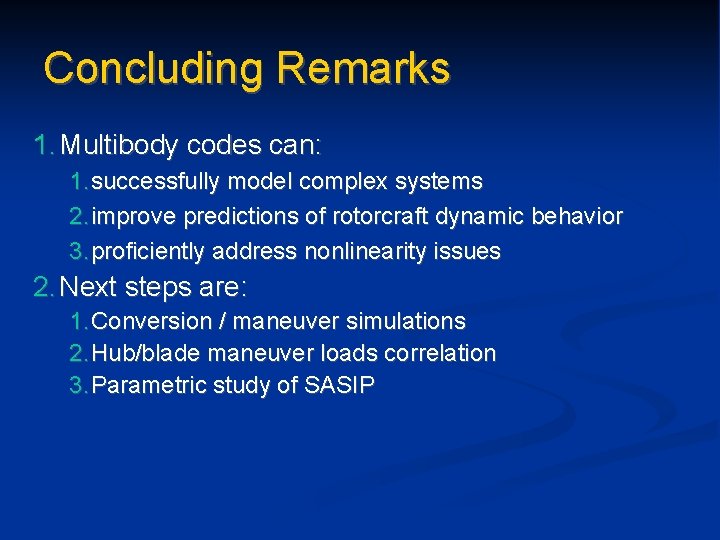
Concluding Remarks 1. Multibody codes can: 1. successfully model complex systems 2. improve predictions of rotorcraft dynamic behavior 3. proficiently address nonlinearity issues 2. Next steps are: 1. Conversion / maneuver simulations 2. Hub/blade maneuver loads correlation 3. Parametric study of SASIP
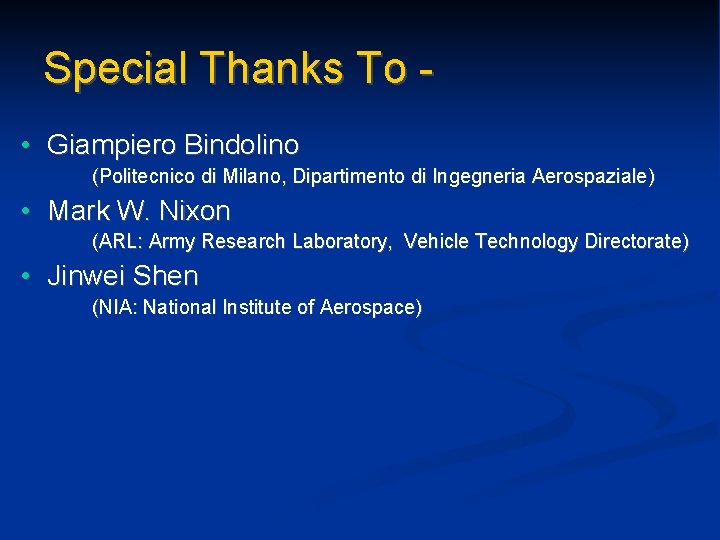
Special Thanks To • Giampiero Bindolino (Politecnico di Milano, Dipartimento di Ingegneria Aerospaziale) • Mark W. Nixon (ARL: Army Research Laboratory, Vehicle Technology Directorate) • Jinwei Shen (NIA: National Institute of Aerospace)
English for further studies
Not for further distribution
For details please contact
Further mechanics 1 unit test 1 momentum and impulse
Time series further maths
Further applications of integration
Ship terminology denoting toward the direction of the bow
Further applications of integration
This is to certify further
For further information please visit our website
Havering college of further and higher education
Further applications of integration
Dr frost further kinematics
Further simplification of a force and couple system
Requesting information letter
Further applications of integration
Further applications of integration
Difference between further and furthermore
A metaphor introduced and then further developed
Further mechanics elastic strings and springs
Further education support service
Sell or process further decision
Further applications of integration
Before we proceed further
Further study design