DIFFRACTION WHY IS IT INTERESTING E C ASCHENAUER
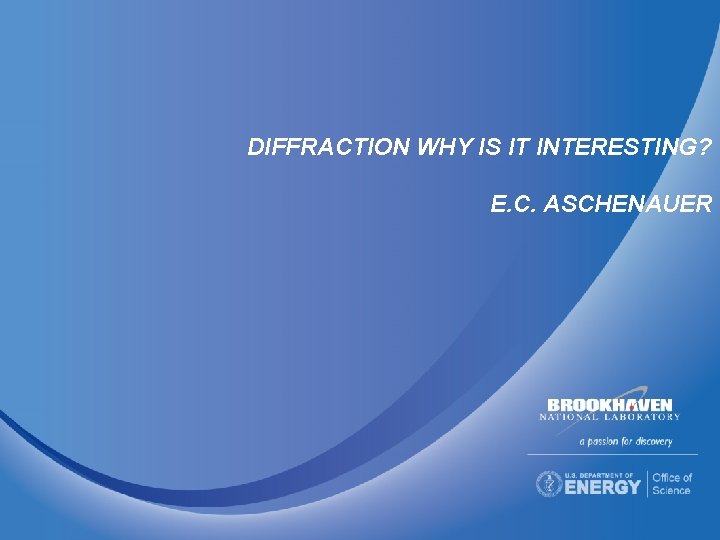
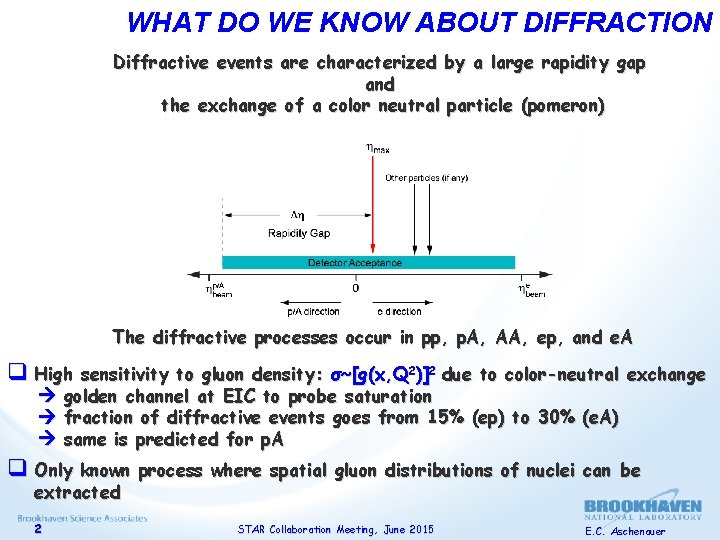
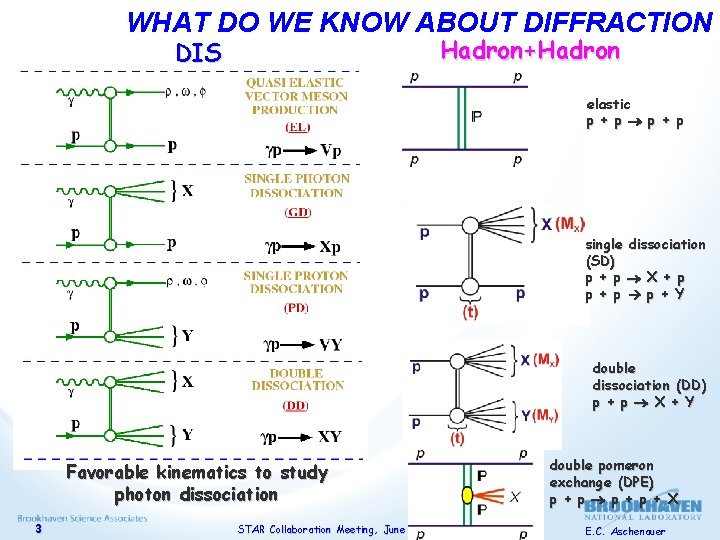
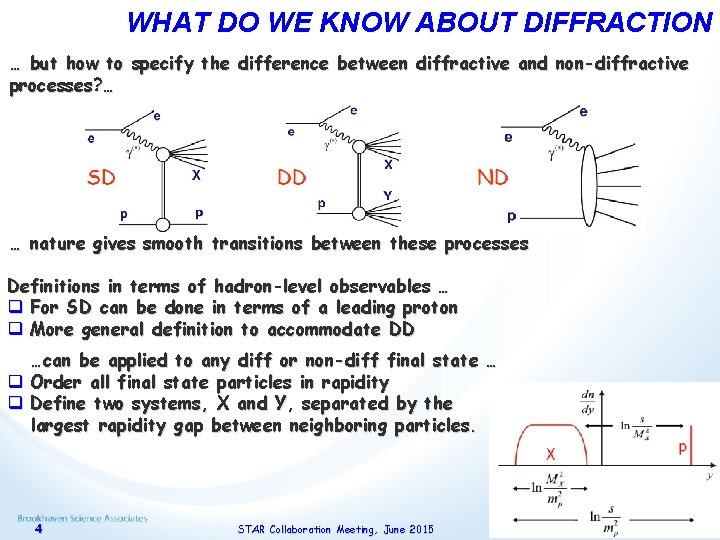
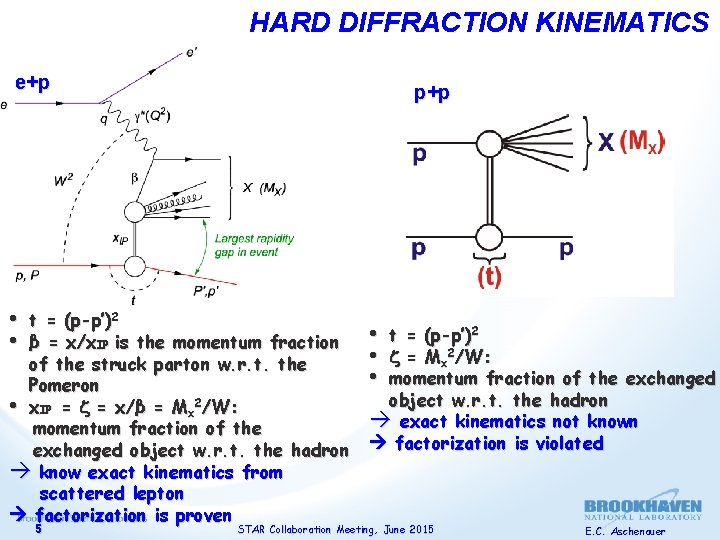
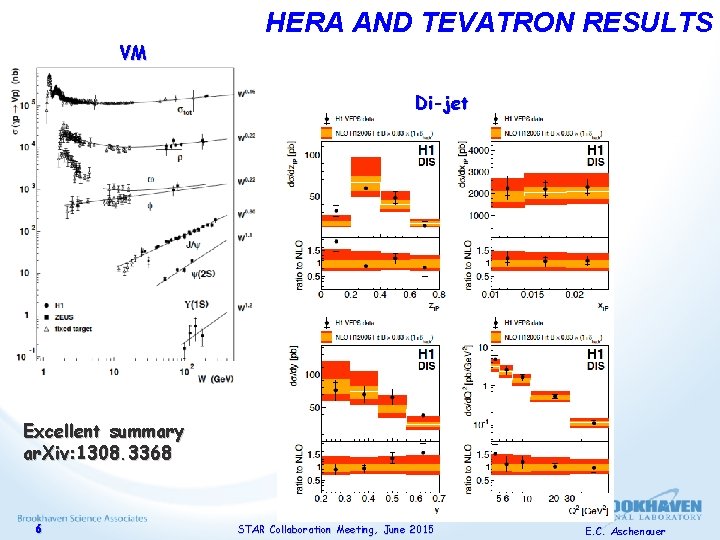
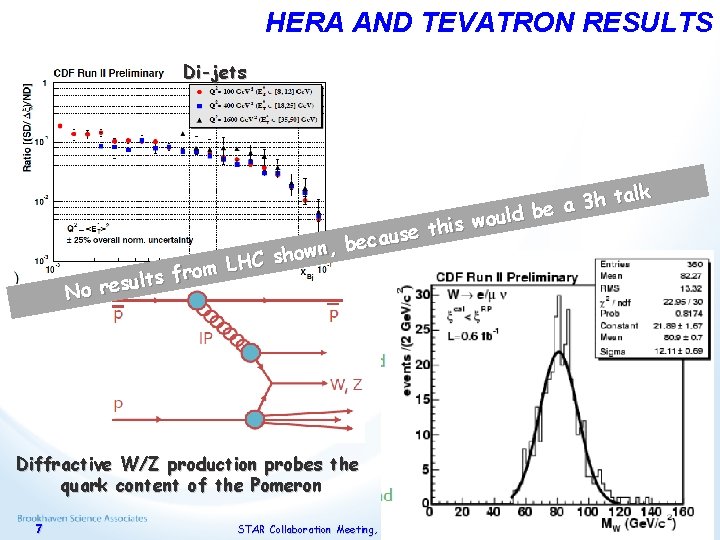
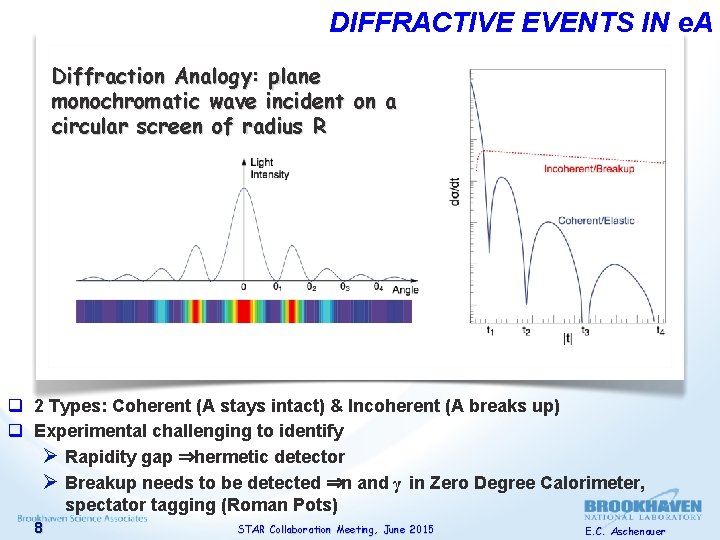
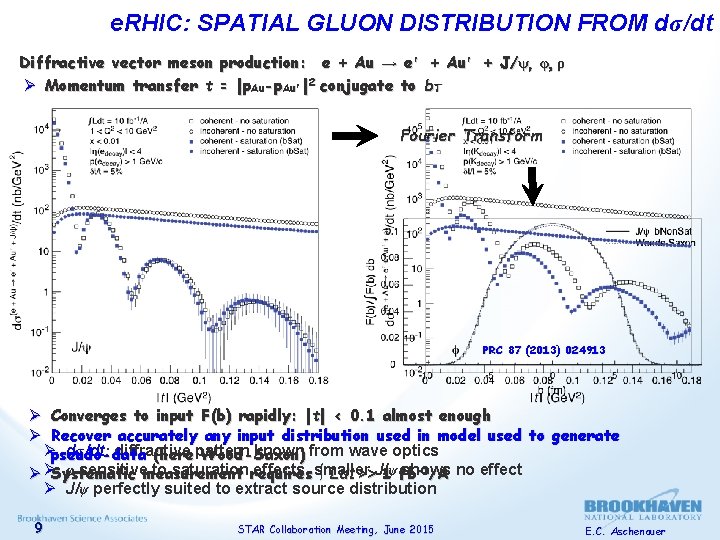
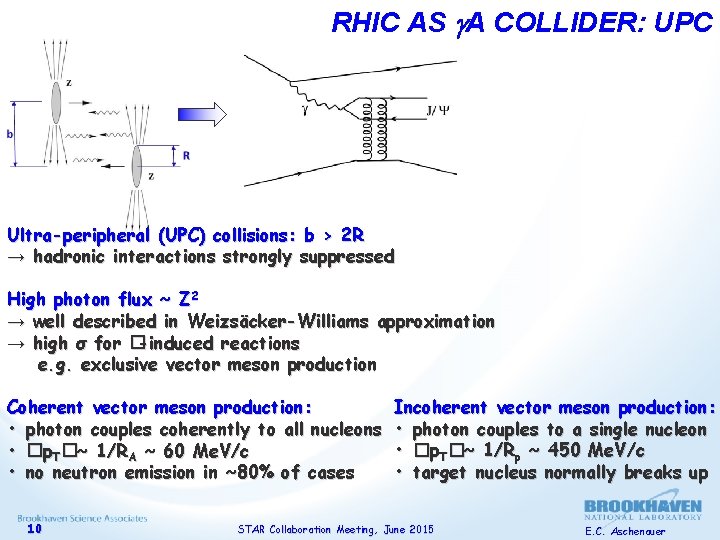
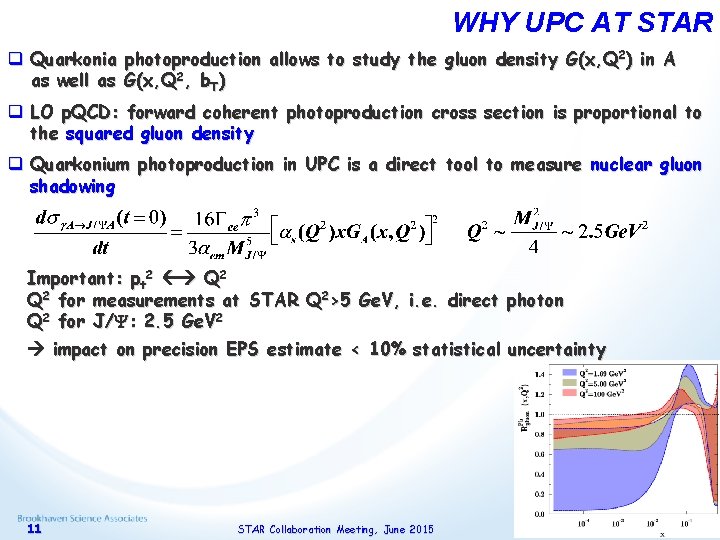
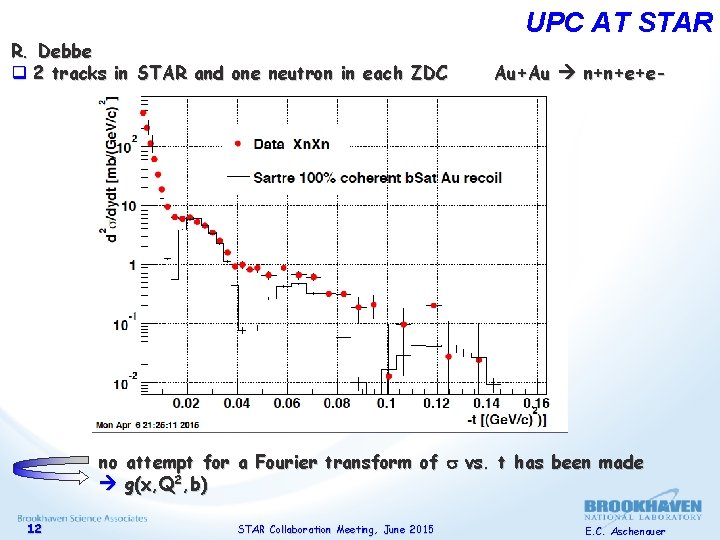
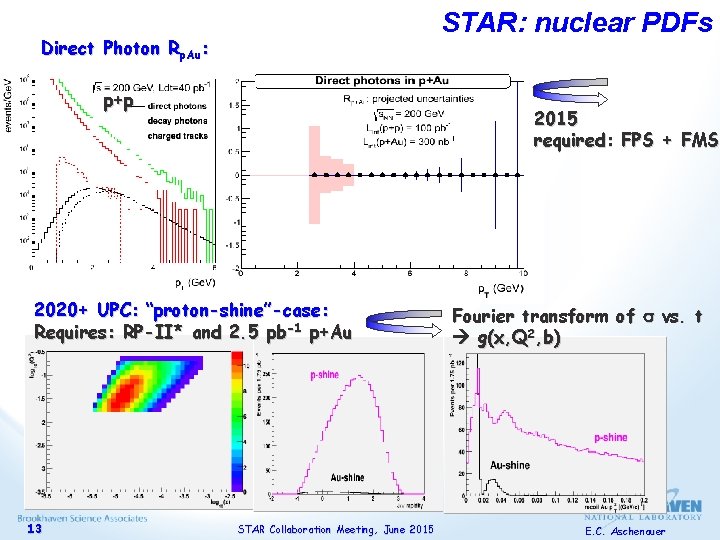
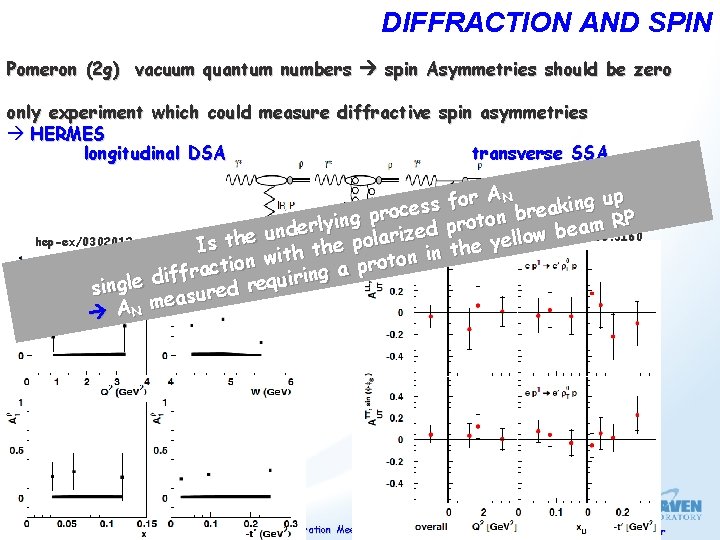
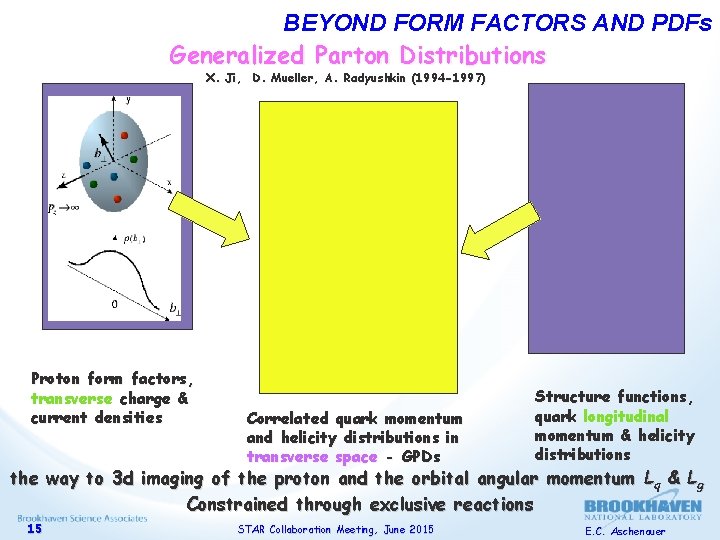
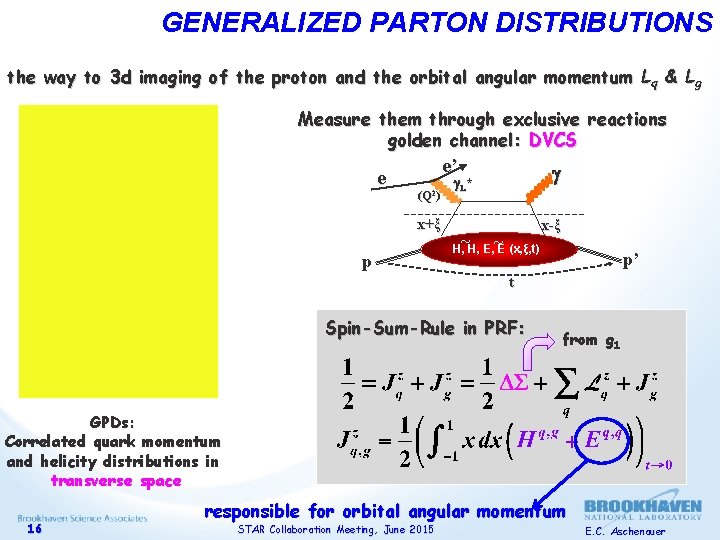
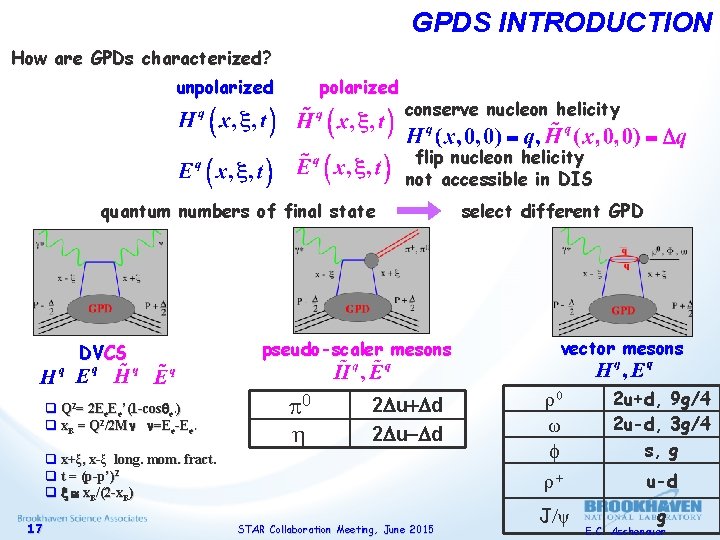
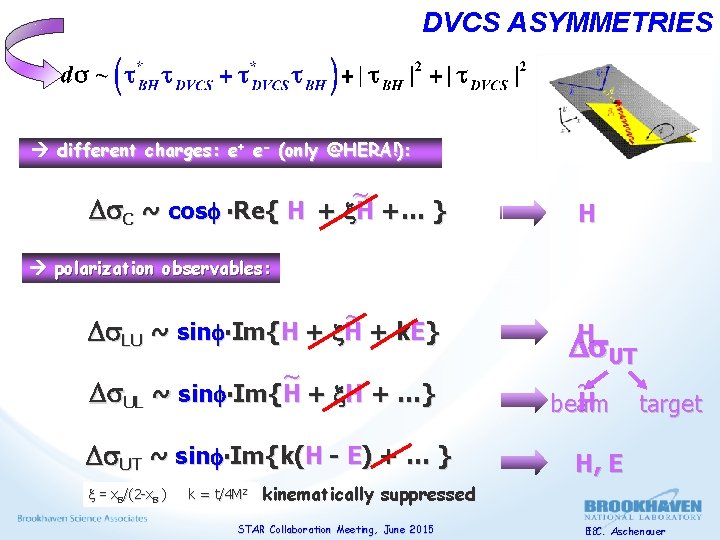
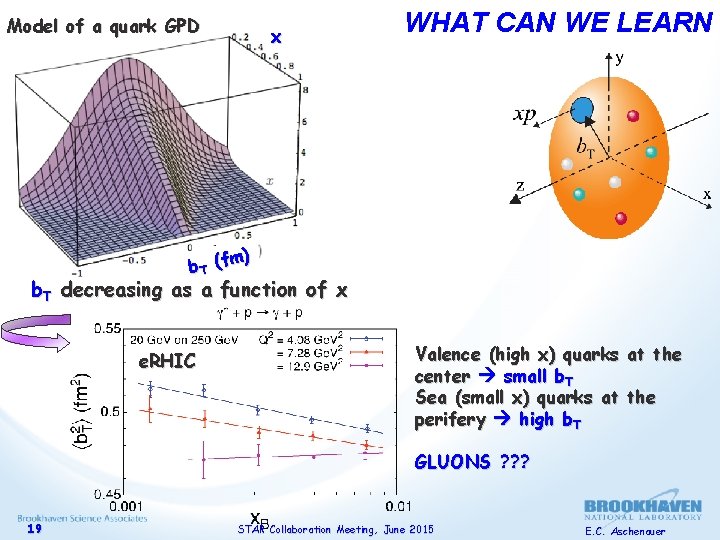
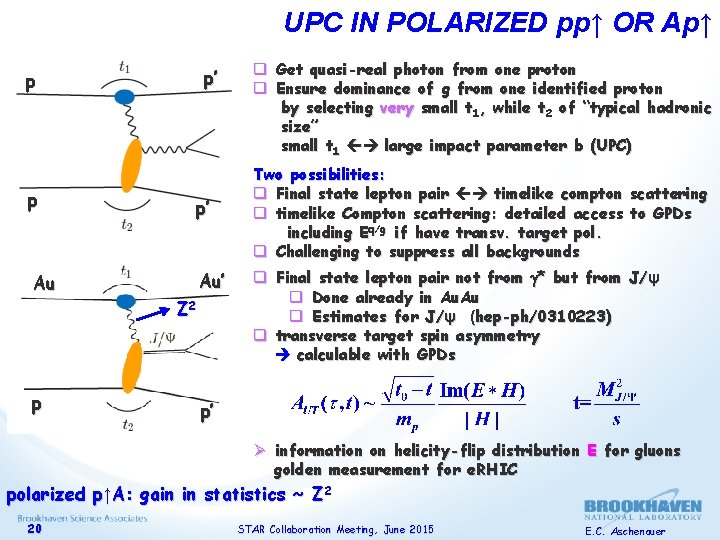
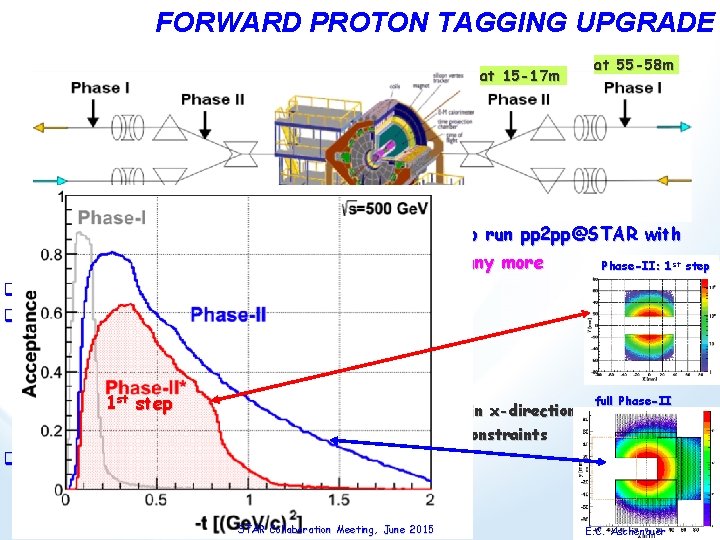
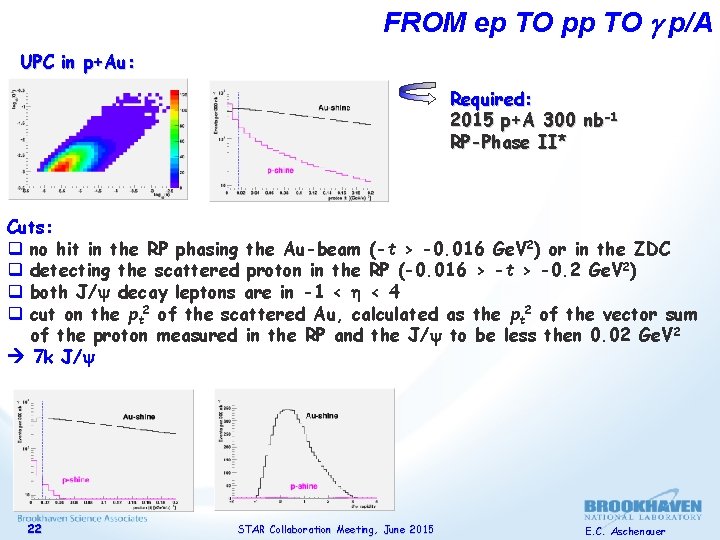
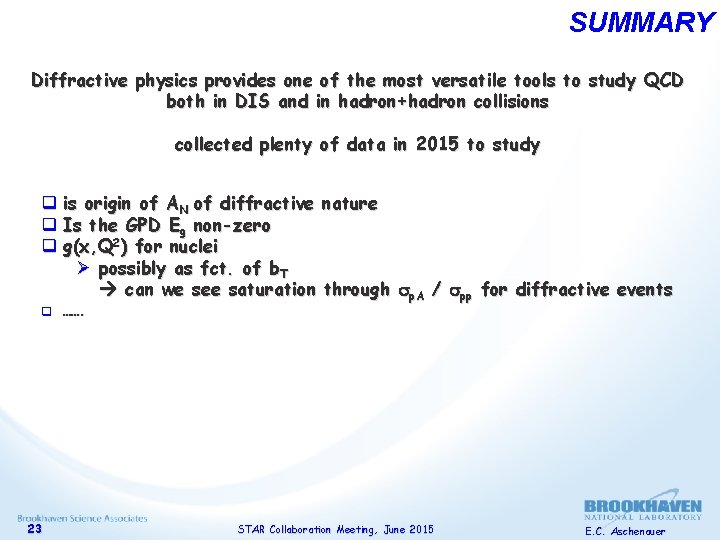
- Slides: 23
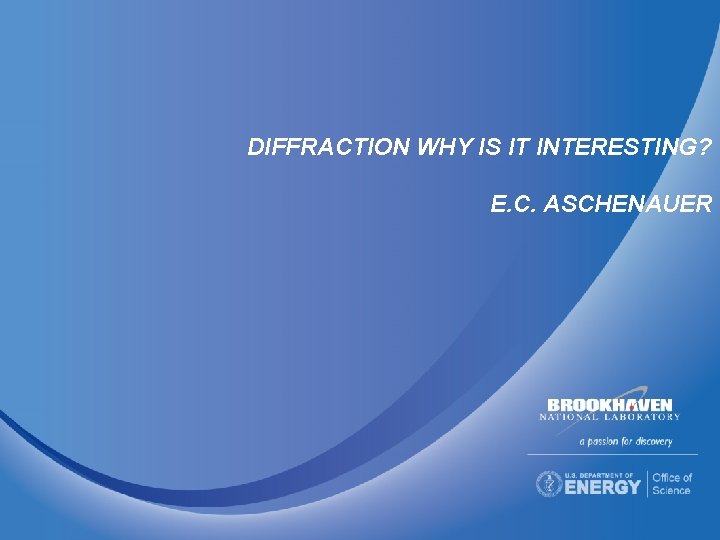
DIFFRACTION WHY IS IT INTERESTING? E. C. ASCHENAUER
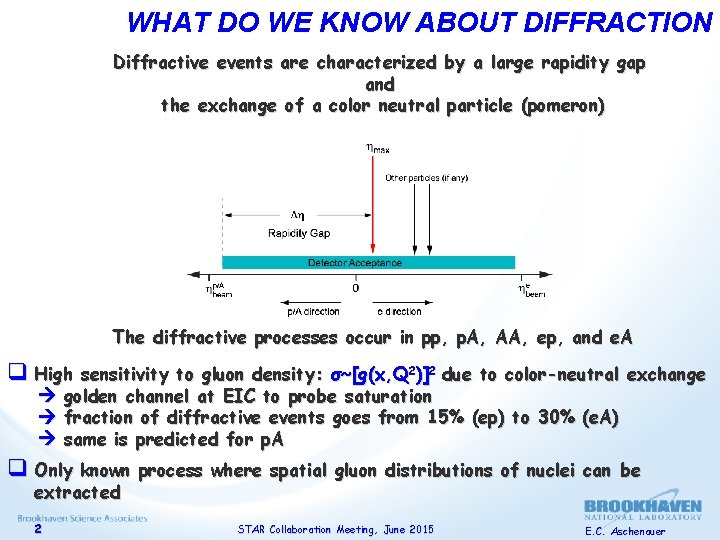
WHAT DO WE KNOW ABOUT DIFFRACTION Diffractive events are characterized by a large rapidity gap and the exchange of a color neutral particle (pomeron) The diffractive processes occur in pp, p. A, AA, ep, and e. A q High sensitivity to gluon density: σ~[g(x, Q 2)]2 due to color-neutral exchange golden channel at EIC to probe saturation fraction of diffractive events goes from 15% (ep) to 30% (e. A) same is predicted for p. A q Only known process where spatial gluon distributions of nuclei can be extracted 2 STAR Collaboration Meeting, June 2015 E. C. Aschenauer
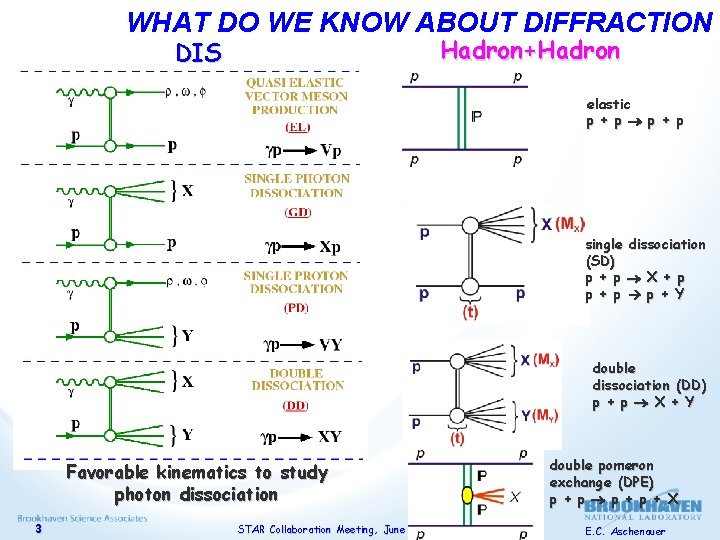
WHAT DO WE KNOW ABOUT DIFFRACTION Hadron+Hadron DIS elastic p + p single dissociation (SD) p + p X + p p + p p + Y double dissociation (DD) p + p X + Y Favorable kinematics to study photon dissociation 3 STAR Collaboration Meeting, June 2015 double pomeron exchange (DPE) p + p + X E. C. Aschenauer
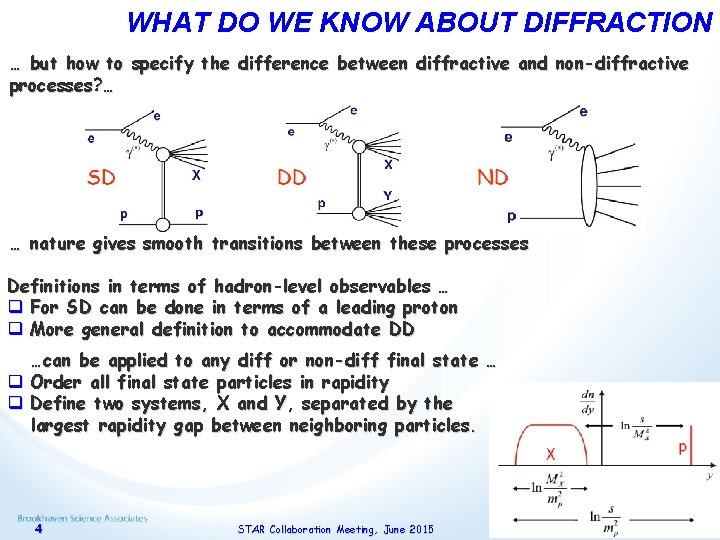
WHAT DO WE KNOW ABOUT DIFFRACTION … but how to specify the difference between diffractive and non-diffractive processes? … … nature gives smooth transitions between these processes Definitions in terms of hadron-level observables … q For SD can be done in terms of a leading proton q More general definition to accommodate DD …can be applied to any diff or non-diff final state … q Order all final state particles in rapidity q Define two systems, X and Y, separated by the largest rapidity gap between neighboring particles. 4 STAR Collaboration Meeting, June 2015 E. C. Aschenauer
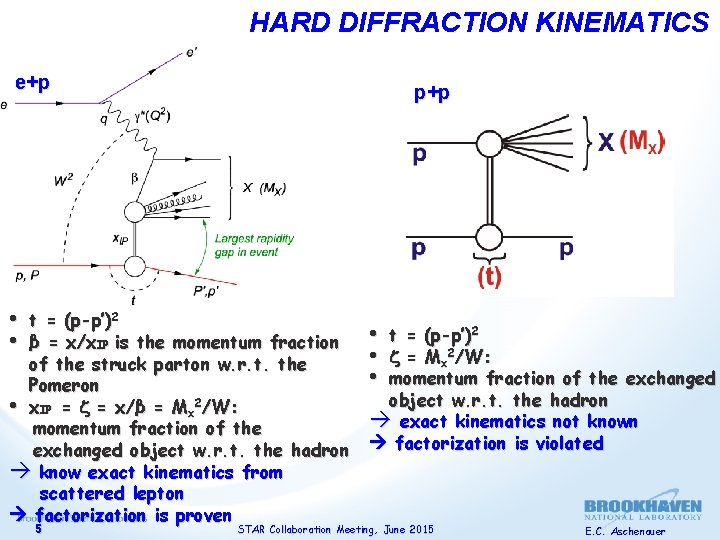
HARD DIFFRACTION KINEMATICS e+p • • p+p t = ( p - p ’) 2 β = x/x. IP is the momentum fraction of the struck parton w. r. t. the Pomeron • x. IP = z = x/β = Mx 2/W: momentum fraction of the exchanged object w. r. t. the hadron à know exact kinematics from scattered lepton factorization is proven 5 • • • t = ( p - p ’) 2 z = Mx 2/W: momentum fraction of the exchanged object w. r. t. the hadron à exact kinematics not known factorization is violated STAR Collaboration Meeting, June 2015 E. C. Aschenauer
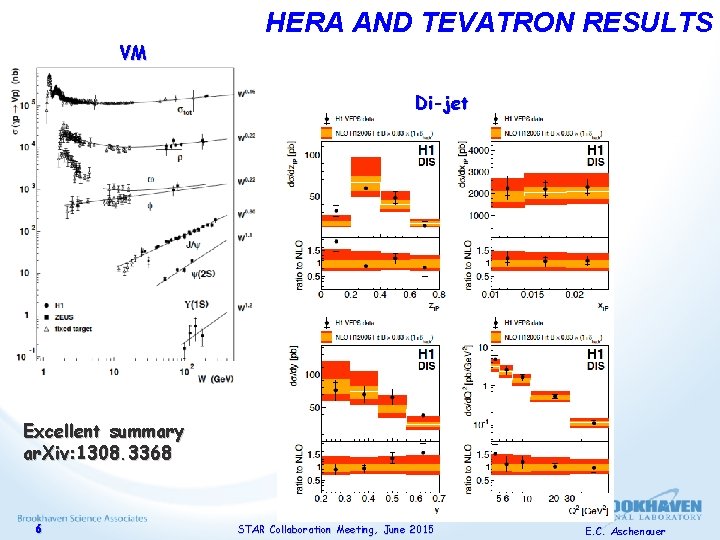
HERA AND TEVATRON RESULTS VM Di-jet Excellent summary ar. Xiv: 1308. 3368 6 STAR Collaboration Meeting, June 2015 E. C. Aschenauer
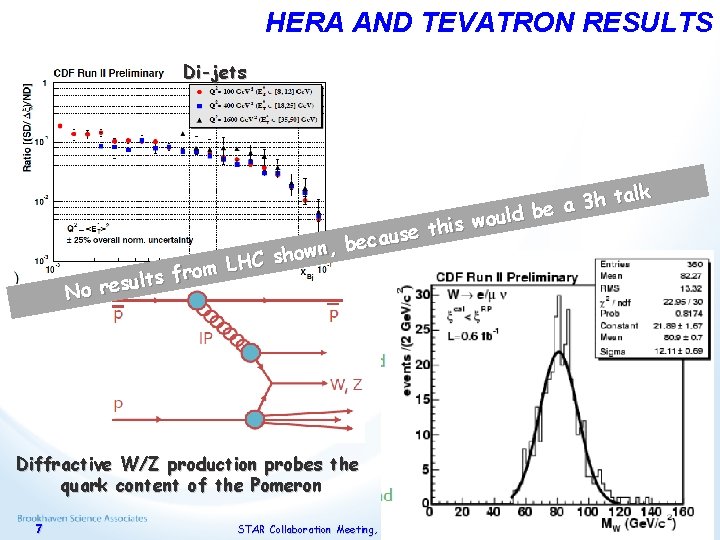
HERA AND TEVATRON RESULTS Di-jets alk t h 3 be a d l u o his w t e s u beca , n w o C sh H L m fro s t l u s No re Diffractive W/Z production probes the quark content of the Pomeron 7 STAR Collaboration Meeting, June 2015 E. C. Aschenauer
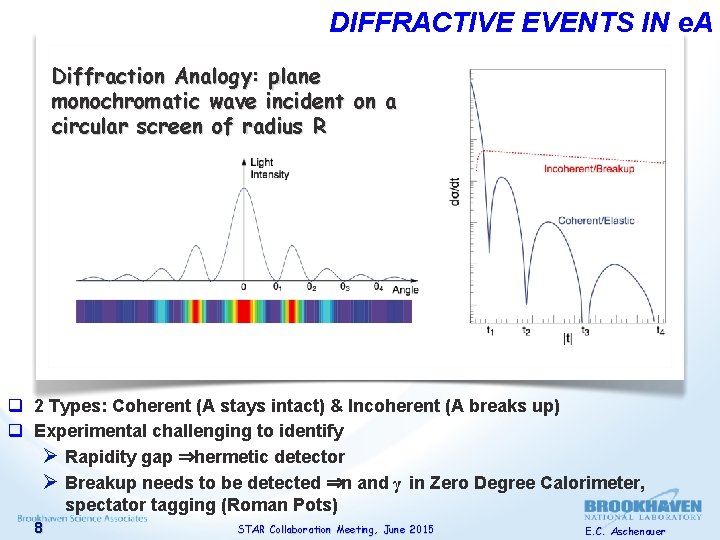
DIFFRACTIVE EVENTS IN e. A Diffraction Analogy: plane monochromatic wave incident on a circular screen of radius R q 2 Types: Coherent (A stays intact) & Incoherent (A breaks up) q Experimental challenging to identify Ø Rapidity gap ⇒hermetic detector Ø Breakup needs to be detected ⇒n and γ in Zero Degree Calorimeter, spectator tagging (Roman Pots) 8 STAR Collaboration Meeting, June 2015 E. C. Aschenauer
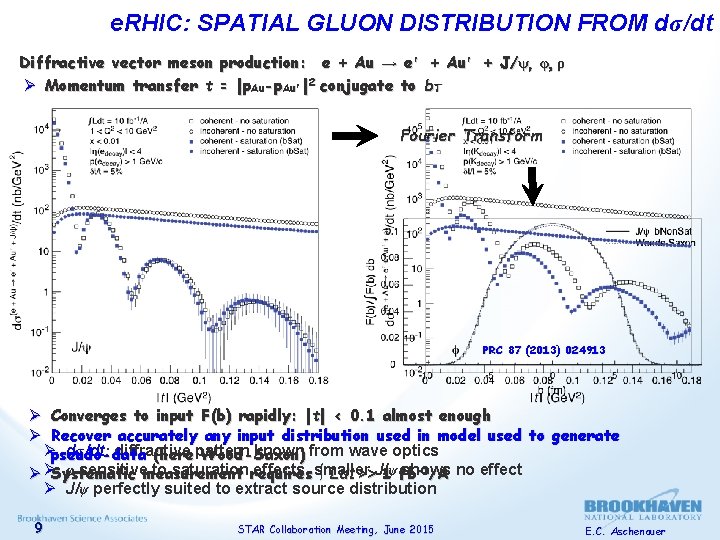
e. RHIC: SPATIAL GLUON DISTRIBUTION FROM dσ/dt Diffractive vector meson production: e + Au → e′ + Au′ + J/ψ, φ, ρ 2 Ø Momentum transfer t = |p. Au-p. Au′ | conjugate to b. T Fourier Transform PRC 87 (2013) 024913 Ø Converges to input F(b) rapidly: |t| < 0. 1 almost enough Ø Recover accurately any input distribution used in model used to generate Øpseudo-data dσ/dt: diffractive known from wave optics (here pattern Wood-Saxon) -1/A no effect φ sensitivemeasurement to saturation requires effects, smaller shows Ø ØSystematic ∫ Ldt >>J/ψ 1 fb Ø J/ψ perfectly suited to extract source distribution 9 STAR Collaboration Meeting, June 2015 E. C. Aschenauer
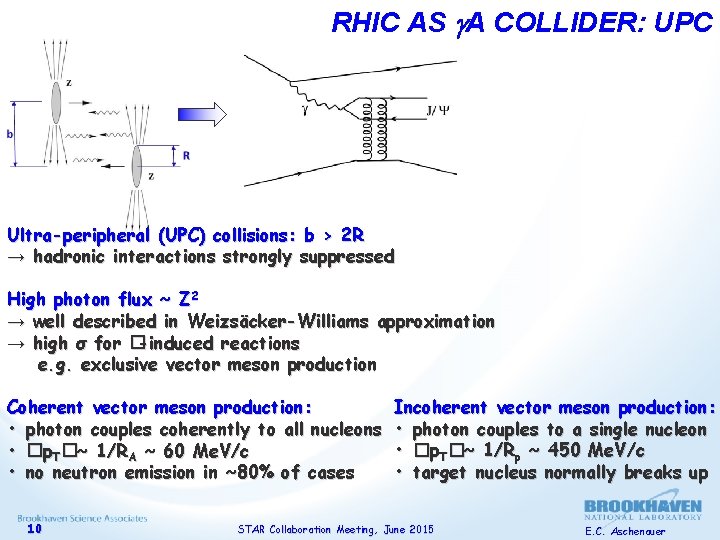
RHIC AS g. A COLLIDER: UPC Ultra-peripheral (UPC) collisions: b > 2 R → hadronic interactions strongly suppressed High photon flux ~ Z 2 → well described in Weizsäcker-Williams approximation → high σ for � -induced reactions e. g. exclusive vector meson production Coherent vector meson production: • photon couples coherently to all nucleons • �p. T�~ 1/RA ~ 60 Me. V/c • no neutron emission in ~80% of cases 10 Incoherent vector meson production: • photon couples to a single nucleon • �p. T�~ 1/Rp ~ 450 Me. V/c • target nucleus normally breaks up STAR Collaboration Meeting, June 2015 E. C. Aschenauer
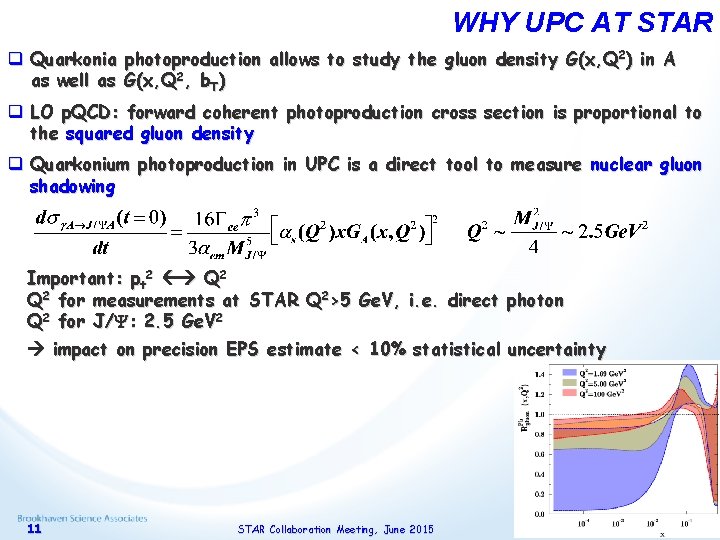
WHY UPC AT STAR q Quarkonia photoproduction allows to study the gluon density G(x, Q 2) in A as well as G(x, Q 2, b. T) q LO p. QCD: forward coherent photoproduction cross section is proportional to the squared gluon density q Quarkonium photoproduction in UPC is a direct tool to measure nuclear gluon shadowing Important: pt 2 Q 2 for measurements at STAR Q 2>5 Ge. V, i. e. direct photon Q 2 for J/Y: 2. 5 Ge. V 2 impact on precision EPS estimate < 10% statistical uncertainty 11 STAR Collaboration Meeting, June 2015 E. C. Aschenauer
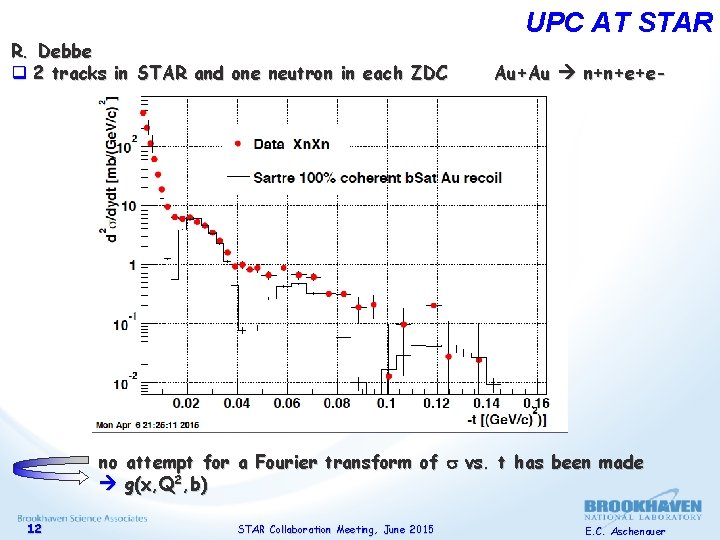
R. Debbe q 2 tracks in STAR and one neutron in each ZDC UPC AT STAR Au+Au n+n+e+e- no attempt for a Fourier transform of s vs. t has been made g(x, Q 2, b) 12 STAR Collaboration Meeting, June 2015 E. C. Aschenauer
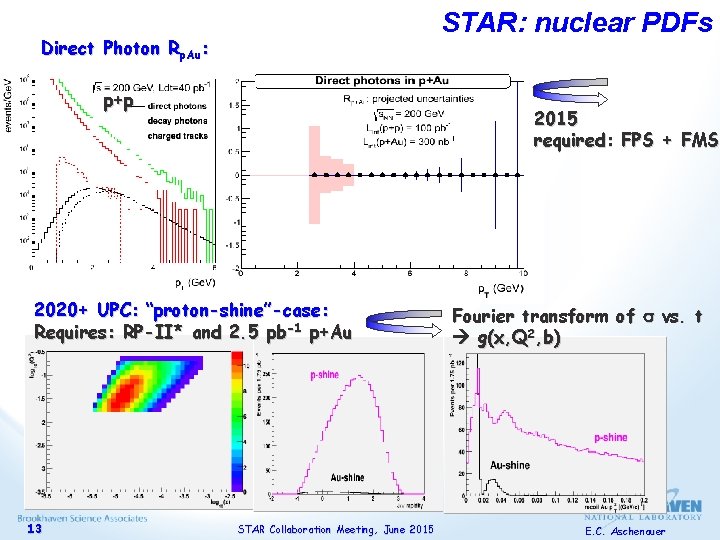
STAR: nuclear PDFs Direct Photon Rp. Au: p+p 2015 required: FPS + FMS 2020+ UPC: “proton-shine”-case: Requires: RP-II* and 2. 5 pb-1 p+Au 13 STAR Collaboration Meeting, June 2015 Fourier transform of s vs. t g(x, Q 2, b) E. C. Aschenauer
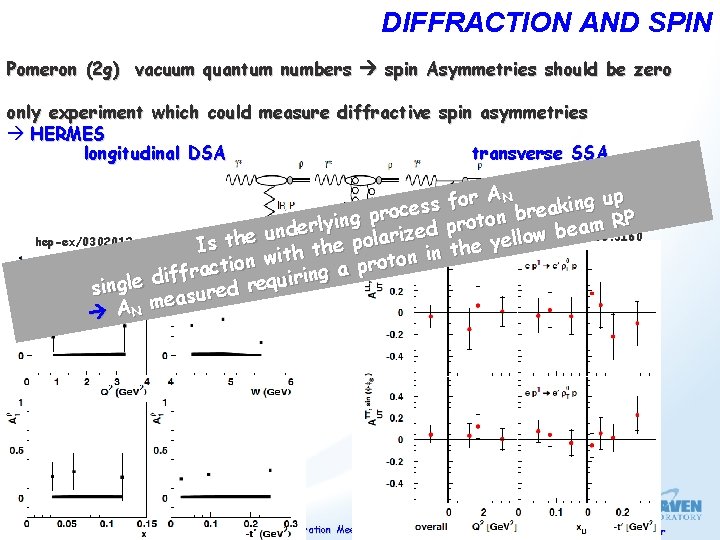
DIFFRACTION AND SPIN Pomeron (2 g) vacuum quantum numbers spin Asymmetries should be zero only experiment which could measure diffractive spin asymmetries à HERMES transverse SSA longitudinal DSA up or A N f g n i s s k e a bre proc n g o n t RP i o y r l m r p a e e d d b e n z u i war. Xiv: 0906. 5160 hep-ex/0302012 Is the th the polar in the yello wi on t n o o r i t p c a ing iffra r d i u e q l e g r sin red u s a e m AN 14 STAR Collaboration Meeting, June 2015 E. C. Aschenauer
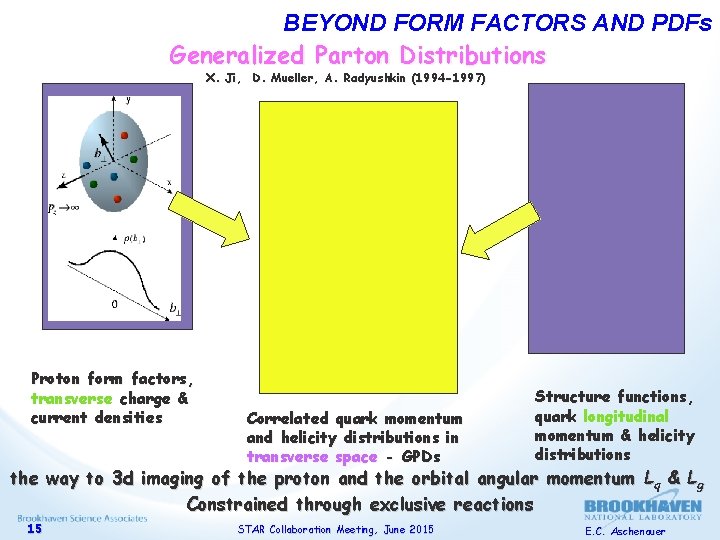
BEYOND FORM FACTORS AND PDFs Generalized Parton Distributions X. Ji, D. Mueller, A. Radyushkin (1994 -1997) Proton form factors, transverse charge & current densities Correlated quark momentum and helicity distributions in transverse space - GPDs Structure functions, quark longitudinal momentum & helicity distributions the way to 3 d imaging of the proton and the orbital angular momentum Lq & Lg Constrained through exclusive reactions 15 STAR Collaboration Meeting, June 2015 E. C. Aschenauer
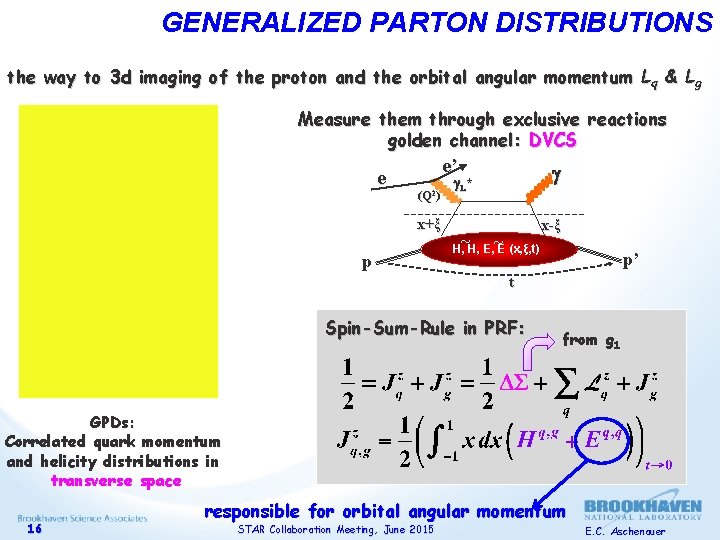
GENERALIZED PARTON DISTRIBUTIONS the way to 3 d imaging of the proton and the orbital angular momentum Lq & Lg Measure them through exclusive reactions golden channel: DVCS e e’ (Q 2) x+ξ p g g. L* ~ ~ H, H, E, ~E (x, ξ, t) x-ξ p’ t Spin-Sum-Rule in PRF: from g 1 GPDs: Correlated quark momentum and helicity distributions in transverse space 16 responsible for orbital angular momentum STAR Collaboration Meeting, June 2015 E. C. Aschenauer
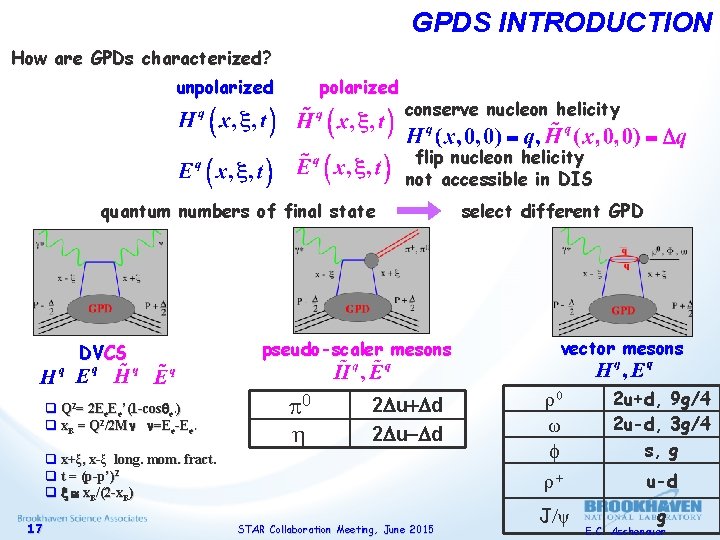
GPDS INTRODUCTION How are GPDs characterized? unpolarized conserve nucleon helicity flip nucleon helicity not accessible in DIS quantum numbers of final state DVCS q 2 Ee. Ee’(1 -cosqe’) q x. B = Q 2/2 Mn n=Ee-Ee’ Q 2= pseudo-scaler mesons p 0 h 2 Du+Dd 2 Du-Dd q x+ξ, x-ξ long. mom. fract. q t = (p-p’)2 q x x. B/(2 -x. B) 17 STAR Collaboration Meeting, June 2015 select different GPD vector mesons ρ0 ω f 2 u+d, 9 g/4 2 u-d, 3 g/4 s, g ρ+ u-d J/ψ g E. C. Aschenauer
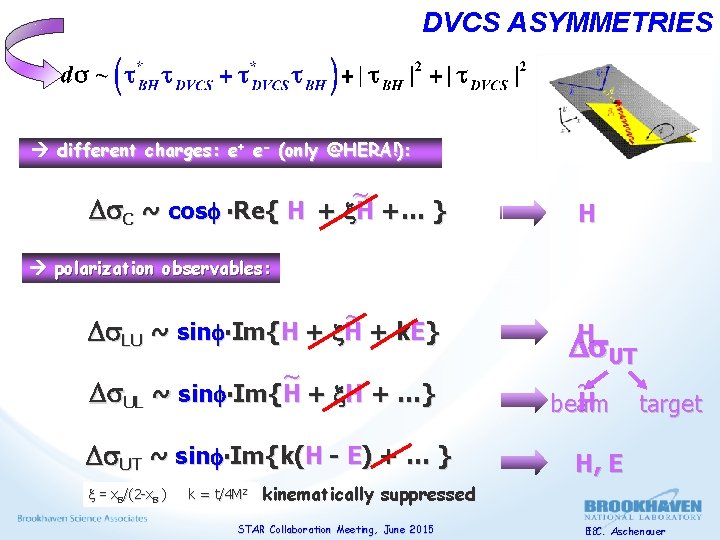
DVCS ASYMMETRIES different charges: e+ e- (only @HERA!): ~ Ds. C ~ cosf ∙Re{ H + x. H +… } H polarization observables: ~ Ds. LU ~ sinf∙Im{H + x. H + k. E} ~ Ds. UL ~ sinf∙Im{H + x. H + …} Ds. UT ~ sinf∙Im{k(H - E) + … } x = x. B/(2 -x. B ) k = t/4 M 2 H Ds UT ~ H beam target H, E kinematically suppressed STAR Collaboration Meeting, June 2015 E. C. Aschenauer 18
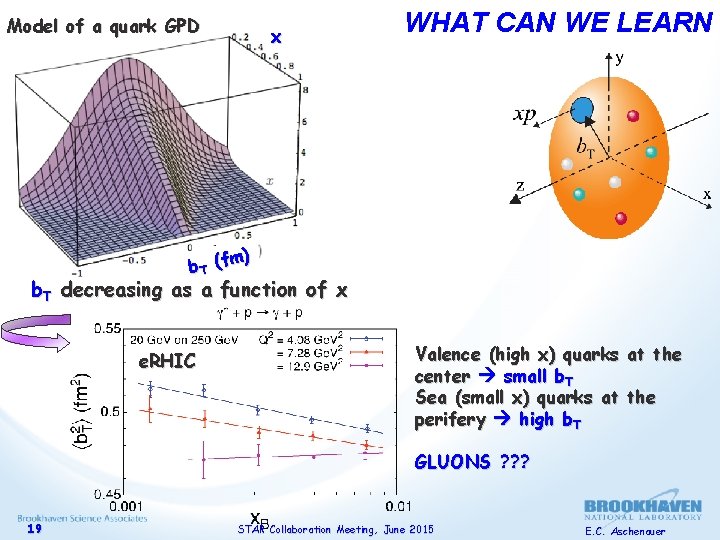
Model of a quark GPD x WHAT CAN WE LEARN ) b T (fm b. T decreasing as a function of x e. RHIC Valence (high x) quarks at the center small b. T Sea (small x) quarks at the perifery high b. T GLUONS ? ? ? 19 STAR Collaboration Meeting, June 2015 E. C. Aschenauer
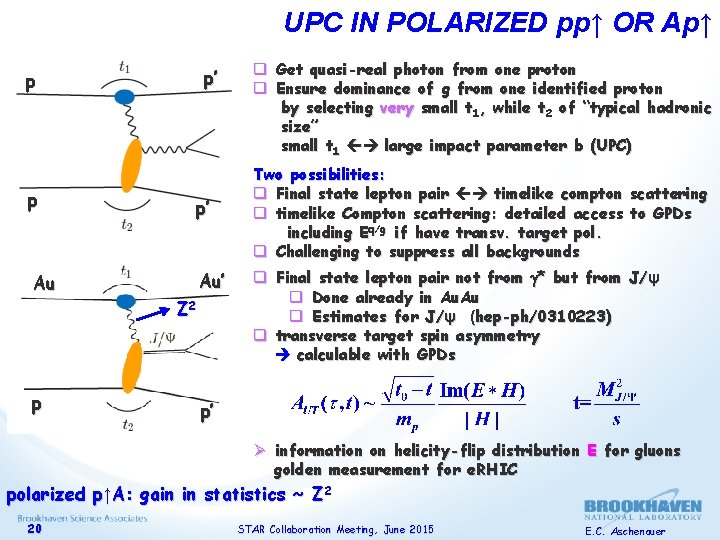
UPC IN POLARIZED pp↑ OR Ap↑ p’ p p p’ Au Au’ p Z 2 q Get quasi-real photon from one proton q Ensure dominance of g from one identified proton by selecting very small t 1, while t 2 of “typical hadronic size” small t 1 large impact parameter b (UPC) Two possibilities: q Final state lepton pair timelike compton scattering q timelike Compton scattering: detailed access to GPDs including Eq/g if have transv. target pol. q Challenging to suppress all backgrounds q Final state lepton pair not from g* but from J/ψ q Done already in Au. Au q Estimates for J/ψ (hep-ph/0310223) q transverse target spin asymmetry calculable with GPDs p’ Ø information on helicity-flip distribution E for gluons golden measurement for e. RHIC polarized p↑A: gain in statistics ~ Z 2 20 STAR Collaboration Meeting, June 2015 E. C. Aschenauer
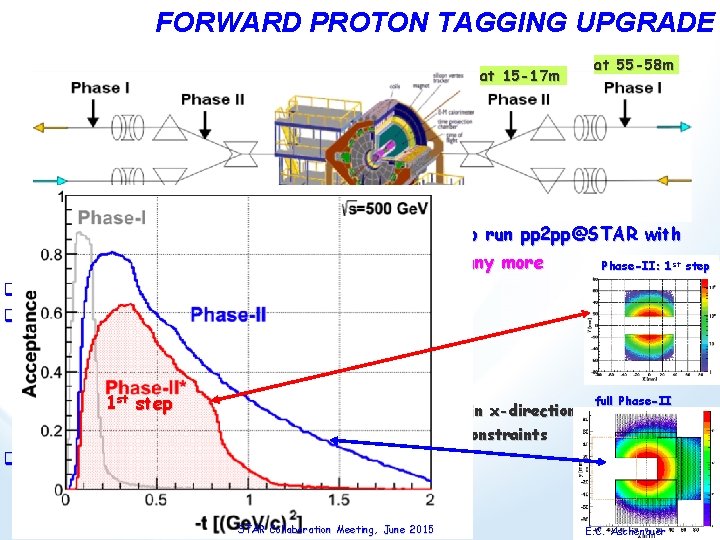
FORWARD PROTON TAGGING UPGRADE at 15 -17 m at 55 -58 m Follow PAC recommendation to develop a solution to run pp 2 pp@STAR with std. physics data taking No special b* running any more Phase-II: 1 st step q should cover wide range in t RPs at 15 m & 17 m q Staged implementation q q à à Phase I (currently installed): low-t coverage Phase II (proposed) : for larger-t coverage 1 st step reuse Phase I RP at new location only in y st step 1 full phase-II: new bigger acceptance RPs & add RP in x-direction à full coverage in φ not possible due to machine constraints full Phase-II q Good acceptance also for spectator protons from deuterium and He-3 collisions 21 STAR Collaboration Meeting, June 2015 E. C. Aschenauer
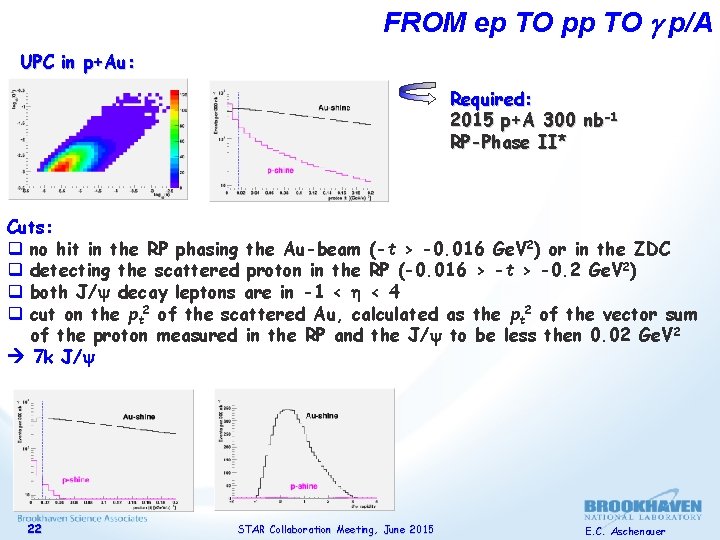
FROM ep TO pp TO g p/A UPC in p+Au: Required: 2015 p+A 300 nb-1 RP-Phase II* Cuts: q no hit in the RP phasing the Au-beam (-t > -0. 016 Ge. V 2) or in the ZDC q detecting the scattered proton in the RP (-0. 016 > -t > -0. 2 Ge. V 2) q both J/ decay leptons are in -1 < h < 4 q cut on the pt 2 of the scattered Au, calculated as the pt 2 of the vector sum of the proton measured in the RP and the J/ to be less then 0. 02 Ge. V 2 7 k J/ 22 STAR Collaboration Meeting, June 2015 E. C. Aschenauer
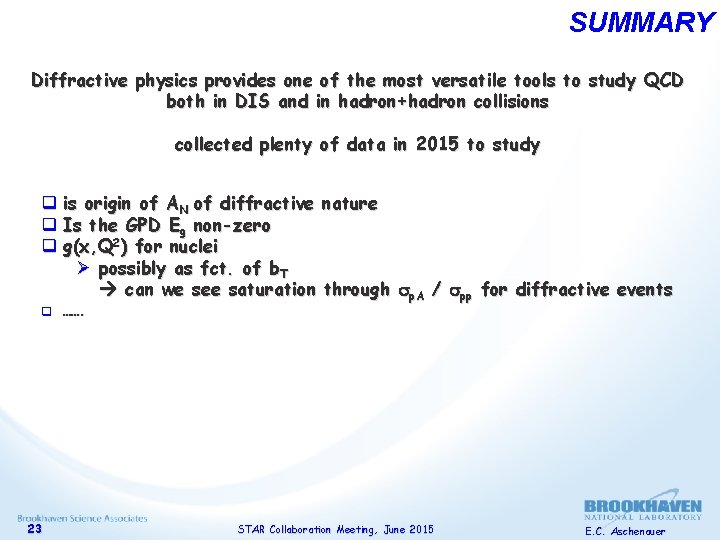
SUMMARY Diffractive physics provides one of the most versatile tools to study QCD both in DIS and in hadron+hadron collisions collected plenty of data in 2015 to study q is origin of AN of diffractive nature q Is the GPD Eg non-zero q g(x, Q 2) for nuclei Ø possibly as fct. of b. T can we see saturation through sp. A / spp for diffractive events q ……. 23 STAR Collaboration Meeting, June 2015 E. C. Aschenauer
Interesting more interesting the most interesting
Andreas carlsson bye bye bye
Don't ask why why why
Thin film formula
Reflection refraction diffraction interference
Refraction vs diffraction
Multiple slits diffraction
Magnets for neutron diffraction
Fraunhoffer diffraction
Define dispersive power of a grating
Difference between fresnel and fraunhofer diffraction
Sound diffraction
Fresnel and fraunhofer diffraction
Diffraction of light
Diffraction by circular aperature
Missing order in diffraction
Diffraction grating
Diffraction in a sentence
Von laue
Diffraction and polarization
Slit diffraction
Crystal diffraction and reciprocal lattice
Diffraction examples
When a wave strikes an object and bounces off