Detection of Protein Conformational Changes with a Nanogap
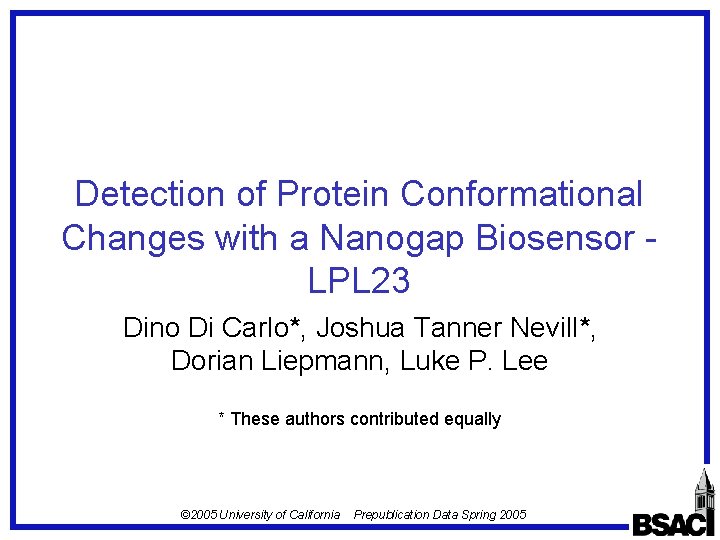
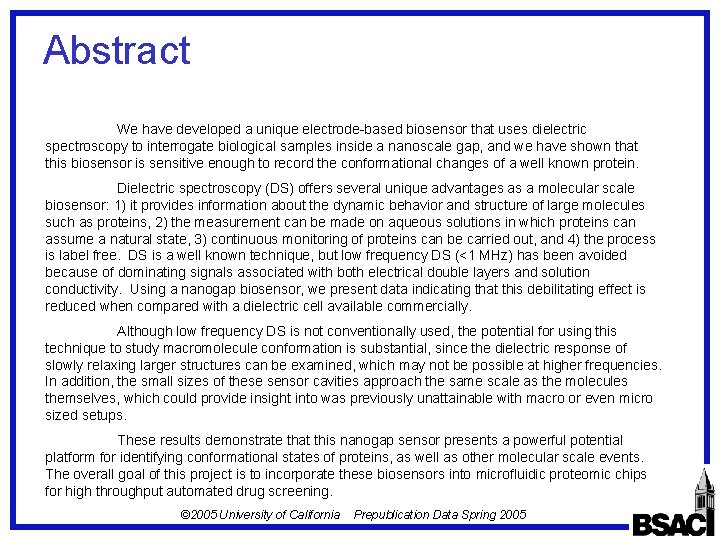
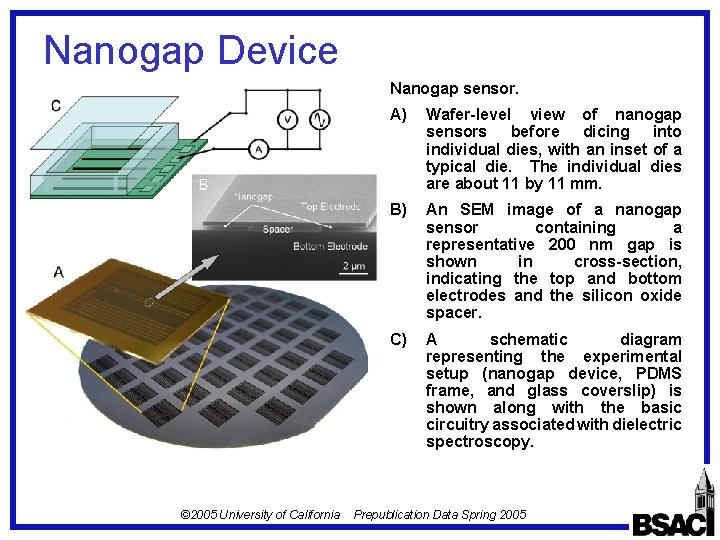
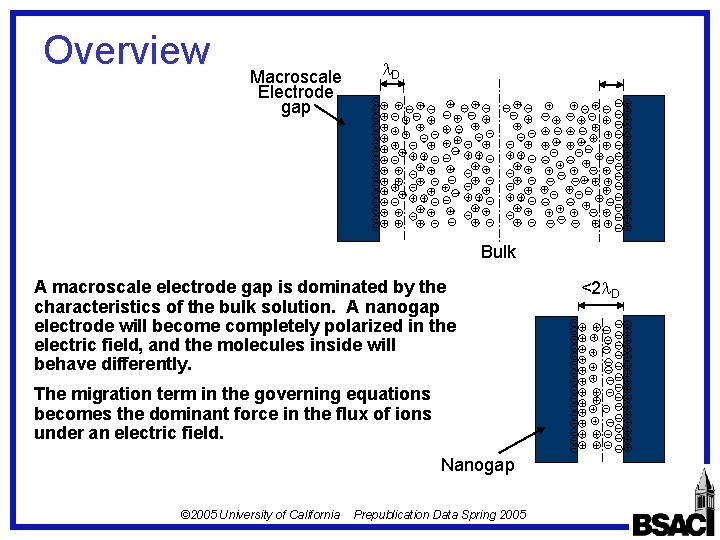
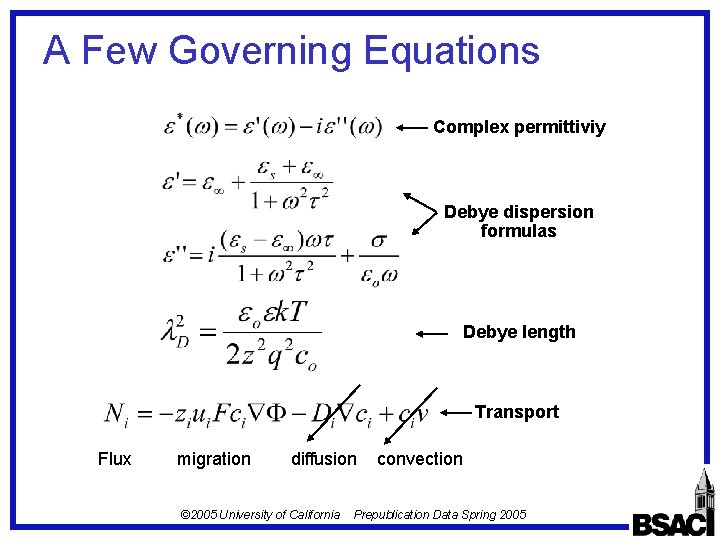
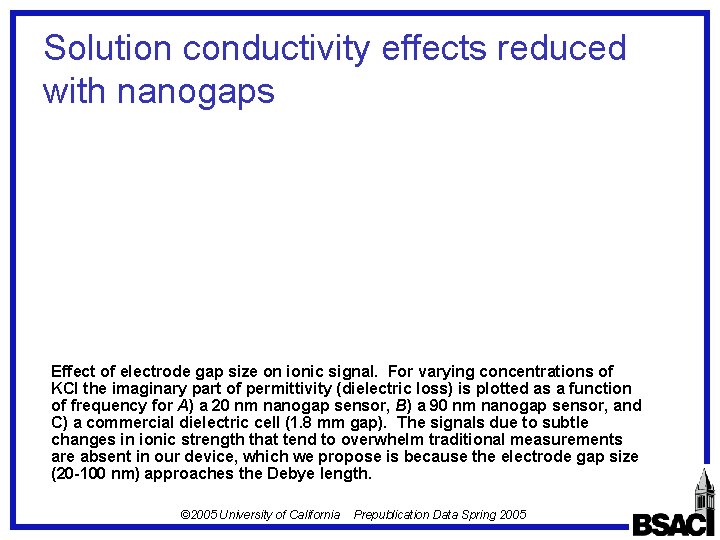
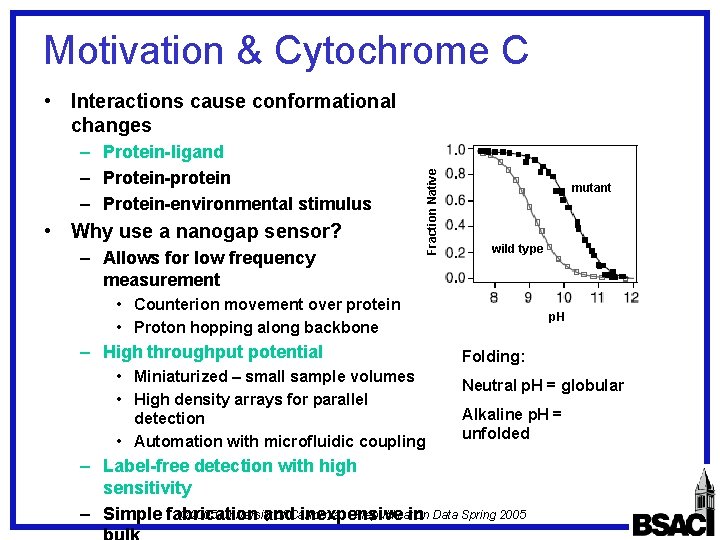
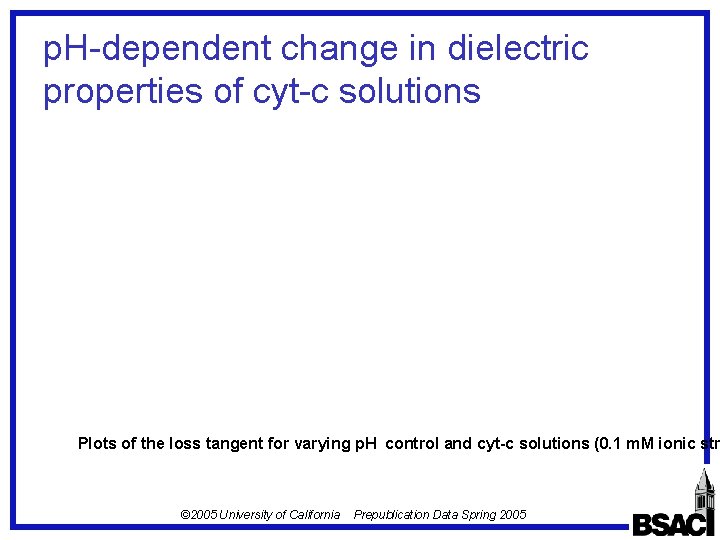
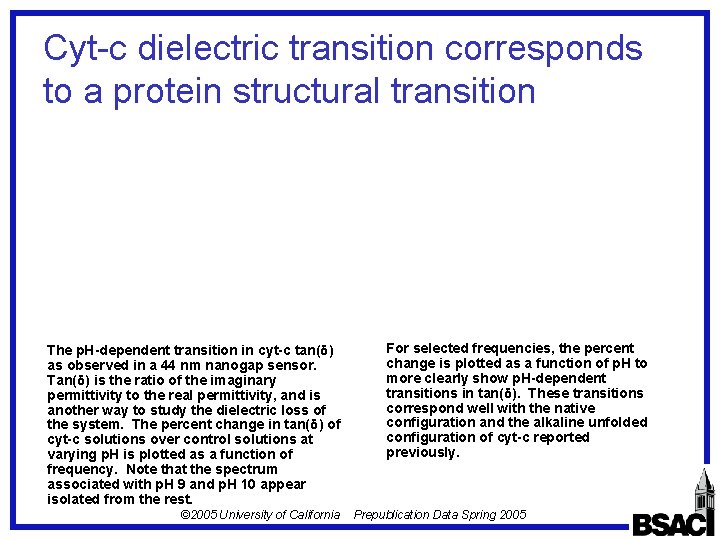
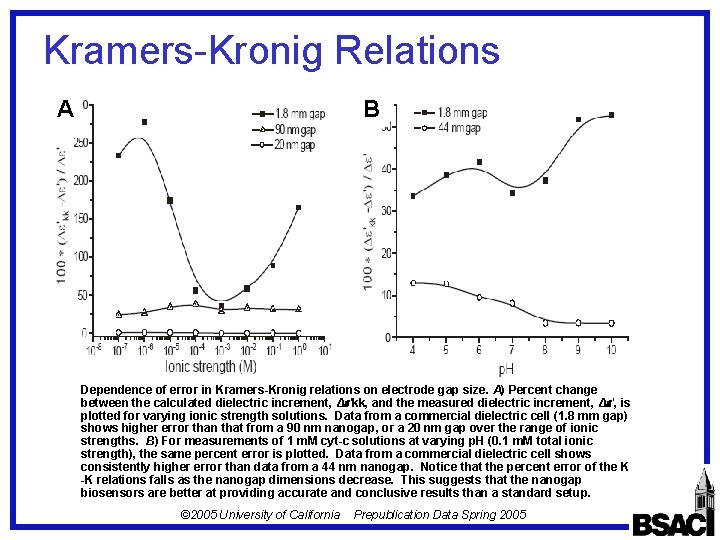
- Slides: 10
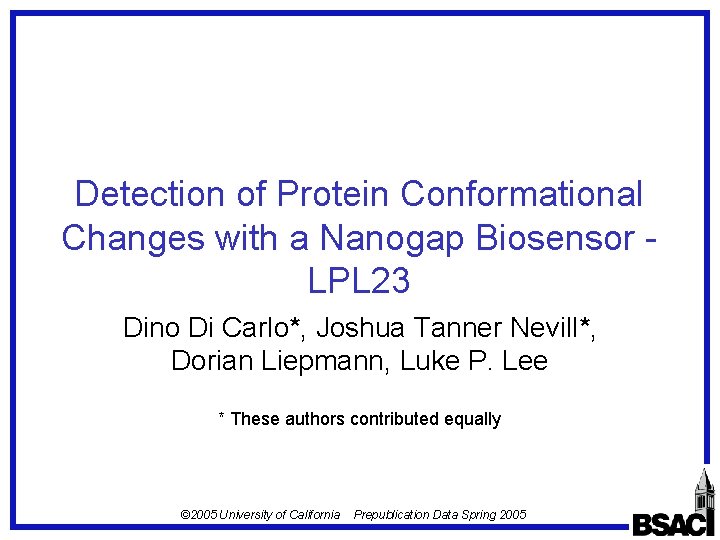
Detection of Protein Conformational Changes with a Nanogap Biosensor LPL 23 Dino Di Carlo*, Joshua Tanner Nevill*, Dorian Liepmann, Luke P. Lee * These authors contributed equally © 2005 University of California Prepublication Data Spring 2005
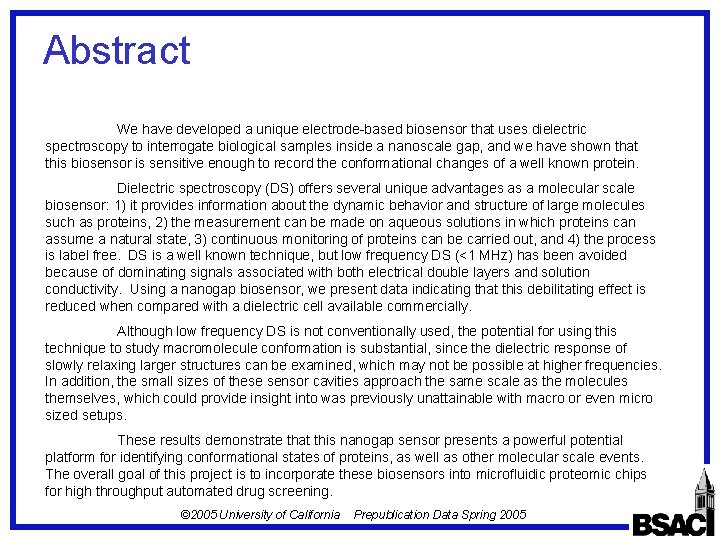
Abstract We have developed a unique electrode-based biosensor that uses dielectric spectroscopy to interrogate biological samples inside a nanoscale gap, and we have shown that this biosensor is sensitive enough to record the conformational changes of a well known protein. Dielectric spectroscopy (DS) offers several unique advantages as a molecular scale biosensor: 1) it provides information about the dynamic behavior and structure of large molecules such as proteins, 2) the measurement can be made on aqueous solutions in which proteins can assume a natural state, 3) continuous monitoring of proteins can be carried out, and 4) the process is label free. DS is a well known technique, but low frequency DS (<1 MHz) has been avoided because of dominating signals associated with both electrical double layers and solution conductivity. Using a nanogap biosensor, we present data indicating that this debilitating effect is reduced when compared with a dielectric cell available commercially. Although low frequency DS is not conventionally used, the potential for using this technique to study macromolecule conformation is substantial, since the dielectric response of slowly relaxing larger structures can be examined, which may not be possible at higher frequencies. In addition, the small sizes of these sensor cavities approach the same scale as the molecules themselves, which could provide insight into was previously unattainable with macro or even micro sized setups. These results demonstrate that this nanogap sensor presents a powerful potential platform for identifying conformational states of proteins, as well as other molecular scale events. The overall goal of this project is to incorporate these biosensors into microfluidic proteomic chips for high throughput automated drug screening. © 2005 University of California Prepublication Data Spring 2005
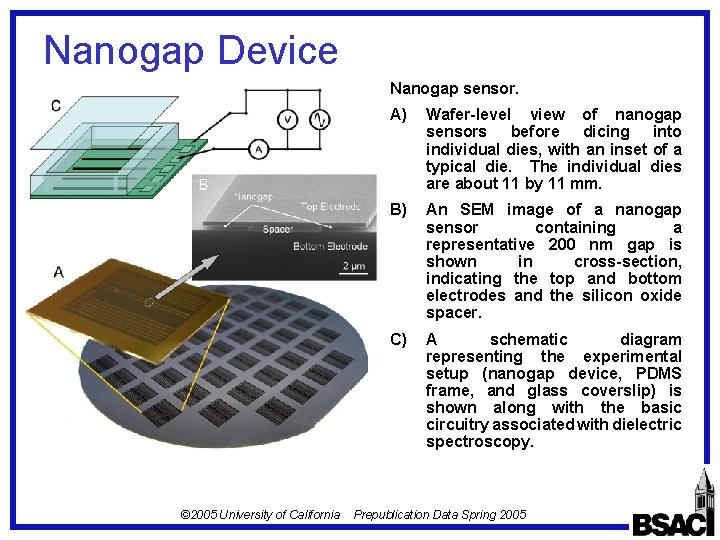
Nanogap Device Nanogap sensor. © 2005 University of California A) Wafer-level view of nanogap sensors before dicing into individual dies, with an inset of a typical die. The individual dies are about 11 by 11 mm. B) An SEM image of a nanogap sensor containing a representative 200 nm gap is shown in cross-section, indicating the top and bottom electrodes and the silicon oxide spacer. C) A schematic diagram representing the experimental setup (nanogap device, PDMS frame, and glass coverslip) is shown along with the basic circuitry associated with dielectric spectroscopy. Prepublication Data Spring 2005
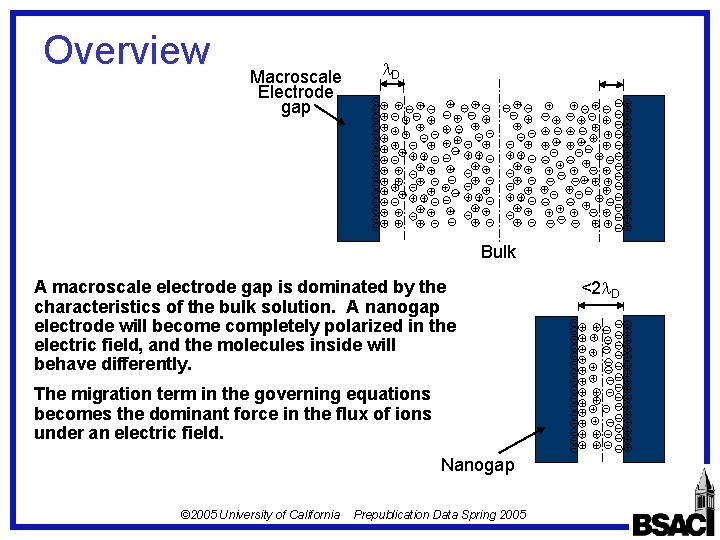
Overview Macroscale Electrode gap D Bulk A macroscale electrode gap is dominated by the characteristics of the bulk solution. A nanogap electrode will become completely polarized in the electric field, and the molecules inside will behave differently. The migration term in the governing equations becomes the dominant force in the flux of ions under an electric field. Nanogap © 2005 University of California Prepublication Data Spring 2005 <2 D
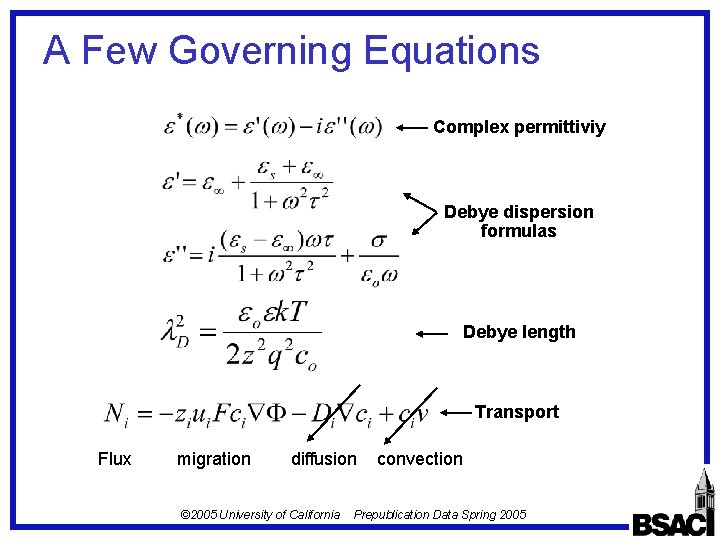
A Few Governing Equations Complex permittiviy Debye dispersion formulas Debye length Transport Flux migration diffusion © 2005 University of California convection Prepublication Data Spring 2005
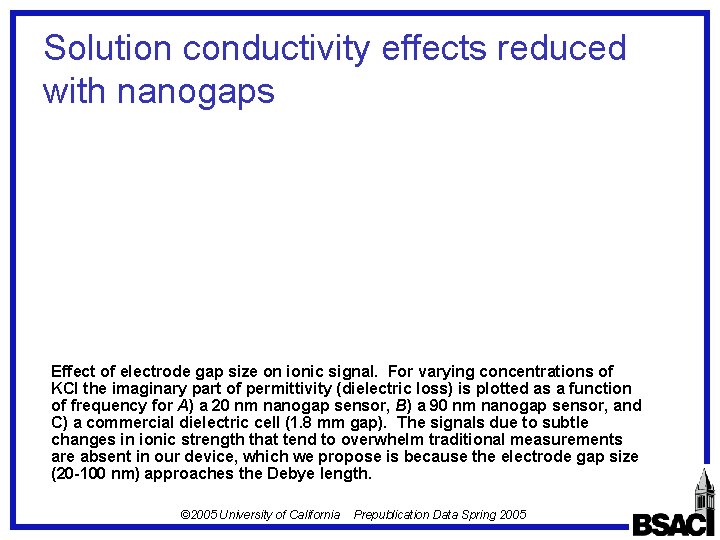
Solution conductivity effects reduced with nanogaps Effect of electrode gap size on ionic signal. For varying concentrations of KCl the imaginary part of permittivity (dielectric loss) is plotted as a function of frequency for A) a 20 nm nanogap sensor, B) a 90 nm nanogap sensor, and C) a commercial dielectric cell (1. 8 mm gap). The signals due to subtle changes in ionic strength that tend to overwhelm traditional measurements are absent in our device, which we propose is because the electrode gap size (20 -100 nm) approaches the Debye length. © 2005 University of California Prepublication Data Spring 2005
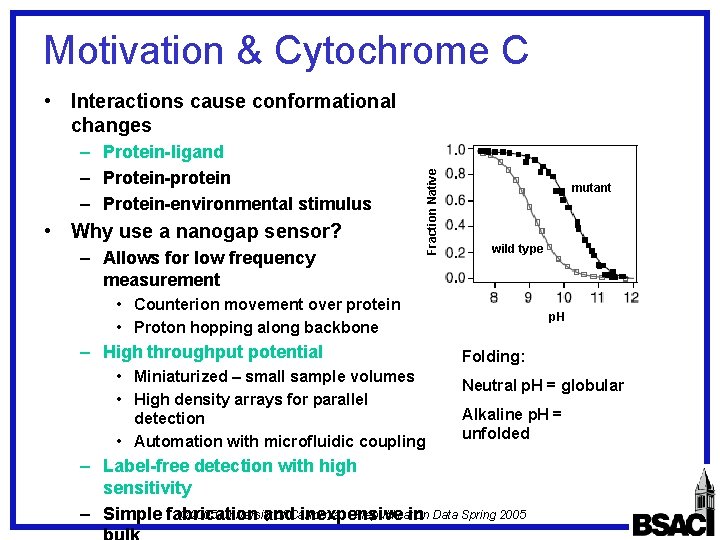
Motivation & Cytochrome C – Protein-ligand – Protein-protein – Protein-environmental stimulus • Why use a nanogap sensor? – Allows for low frequency measurement Fraction Native • Interactions cause conformational changes mutant wild type • Counterion movement over protein • Proton hopping along backbone – High throughput potential • Miniaturized – small sample volumes • High density arrays for parallel detection • Automation with microfluidic coupling p. H Folding: Neutral p. H = globular Alkaline p. H = unfolded – Label-free detection with high sensitivity © 2005 University of California Prepublication – Simple fabrication and inexpensive in Data Spring 2005
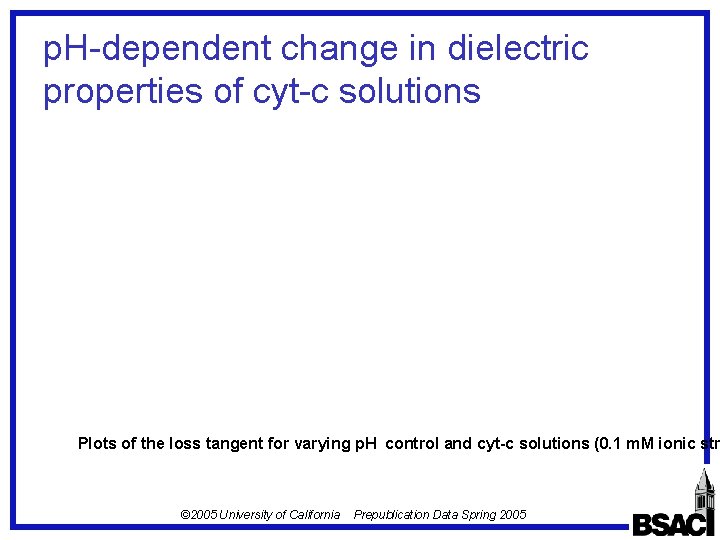
p. H-dependent change in dielectric properties of cyt-c solutions Plots of the loss tangent for varying p. H control and cyt-c solutions (0. 1 m. M ionic str © 2005 University of California Prepublication Data Spring 2005
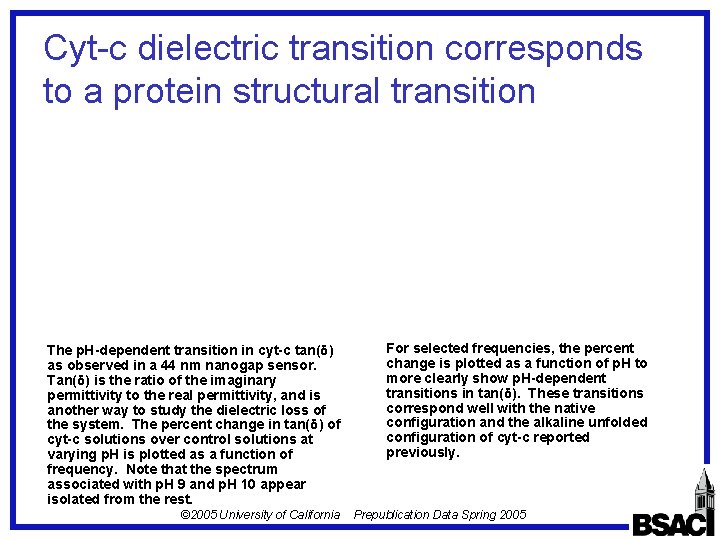
Cyt-c dielectric transition corresponds to a protein structural transition The p. H-dependent transition in cyt-c tan(δ) as observed in a 44 nm nanogap sensor. Tan(δ) is the ratio of the imaginary permittivity to the real permittivity, and is another way to study the dielectric loss of the system. The percent change in tan(δ) of cyt-c solutions over control solutions at varying p. H is plotted as a function of frequency. Note that the spectrum associated with p. H 9 and p. H 10 appear isolated from the rest. © 2005 University of California For selected frequencies, the percent change is plotted as a function of p. H to more clearly show p. H-dependent transitions in tan(δ). These transitions correspond well with the native configuration and the alkaline unfolded configuration of cyt-c reported previously. Prepublication Data Spring 2005
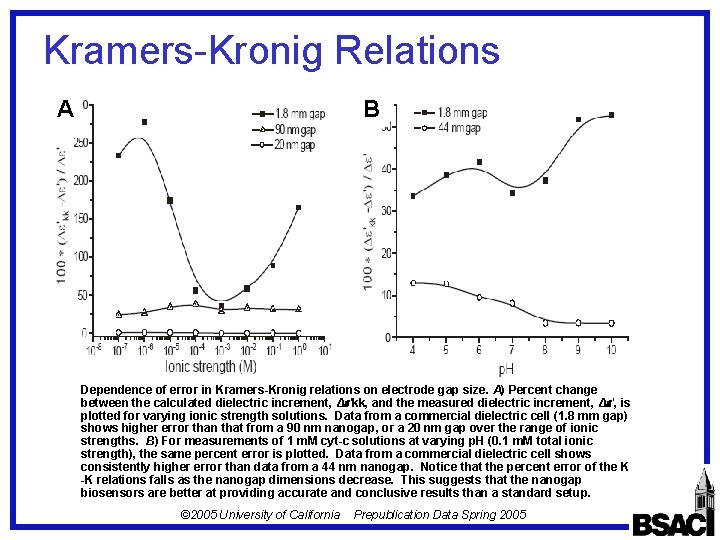
Kramers-Kronig Relations B A Dependence of error in Kramers-Kronig relations on electrode gap size. A) Percent change between the calculated dielectric increment, Δ 'kk, and the measured dielectric increment, Δ ', is plotted for varying ionic strength solutions. Data from a commercial dielectric cell (1. 8 mm gap) shows higher error than that from a 90 nm nanogap, or a 20 nm gap over the range of ionic strengths. B) For measurements of 1 m. M cyt-c solutions at varying p. H (0. 1 m. M total ionic strength), the same percent error is plotted. Data from a commercial dielectric cell shows consistently higher error than data from a 44 nm nanogap. Notice that the percent error of the K -K relations falls as the nanogap dimensions decrease. This suggests that the nanogap biosensors are better at providing accurate and conclusive results than a standard setup. © 2005 University of California Prepublication Data Spring 2005