Nuclear symmetry energy effects on neutron star cooling
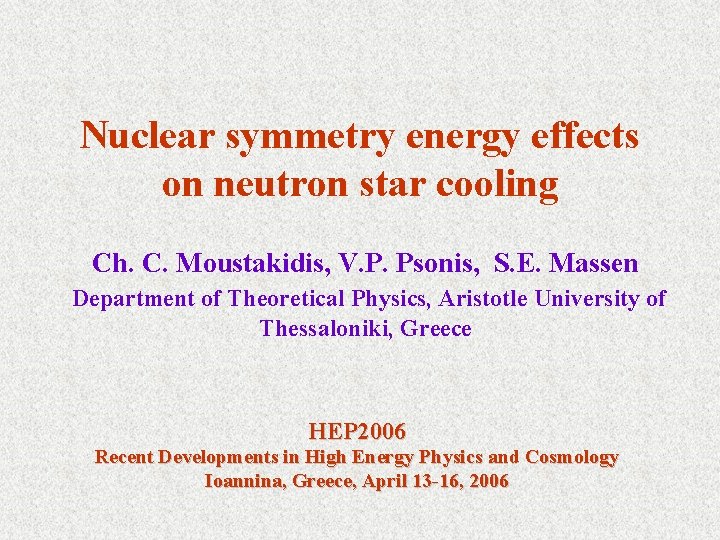
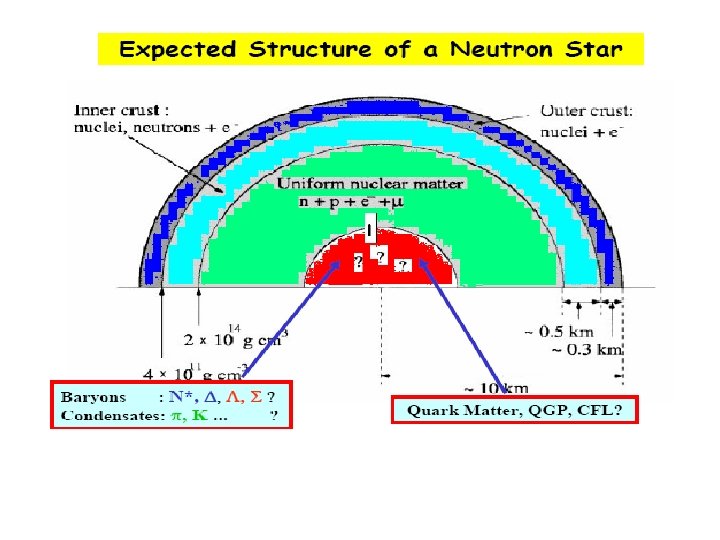
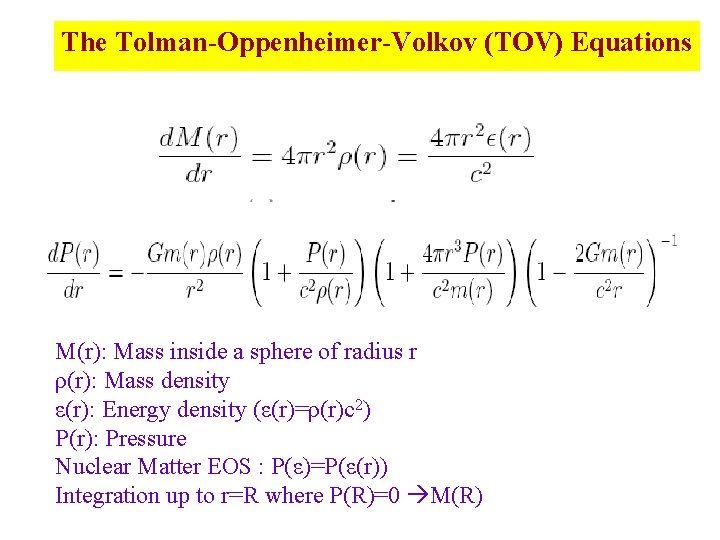
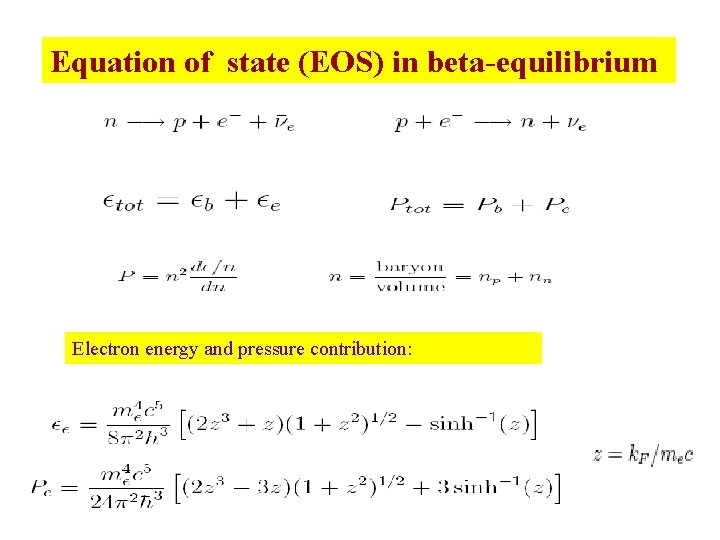
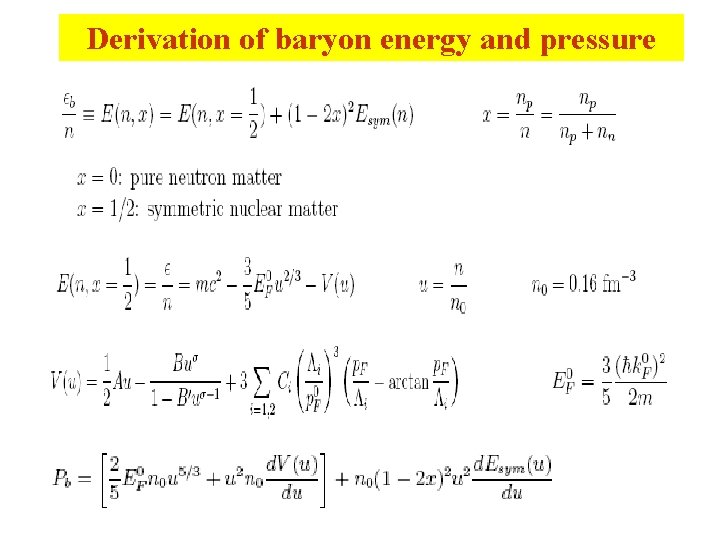
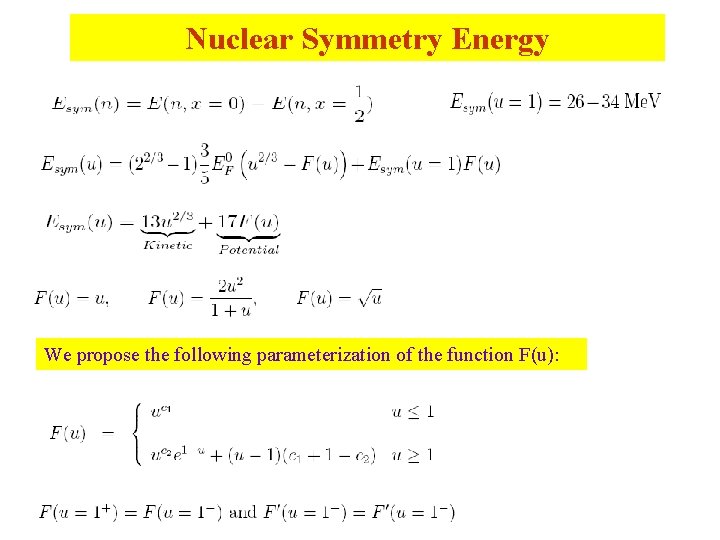
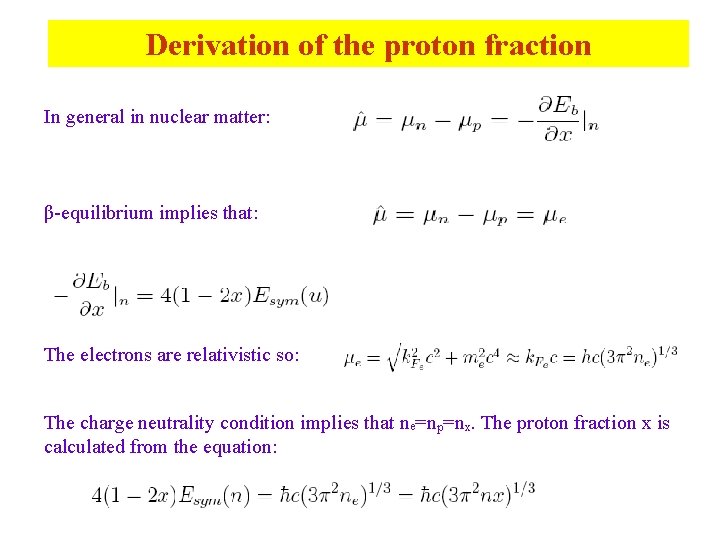
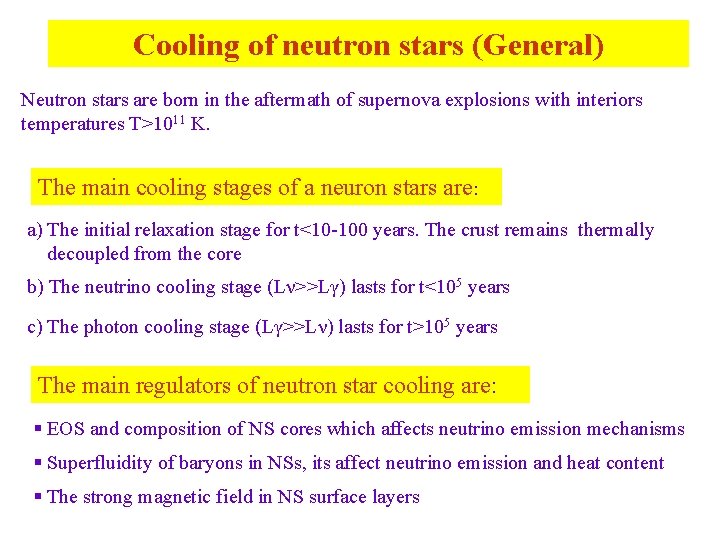
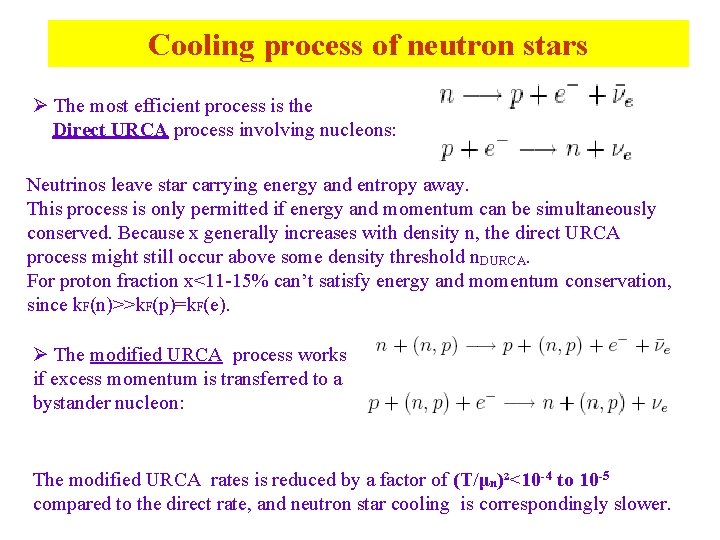
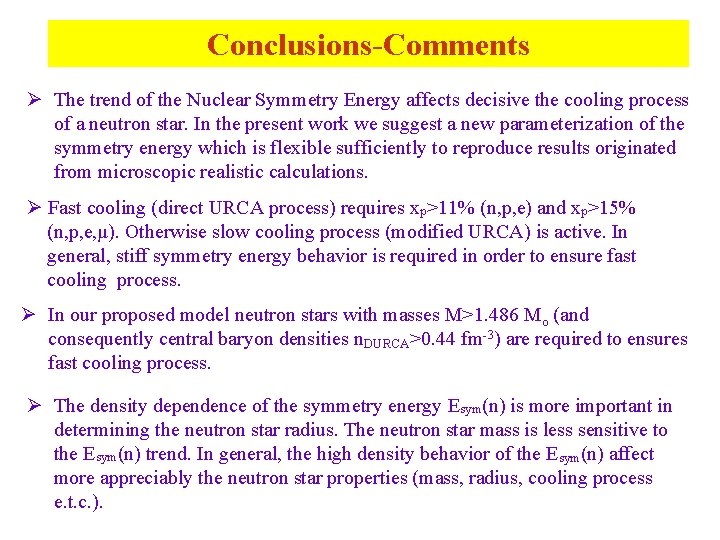
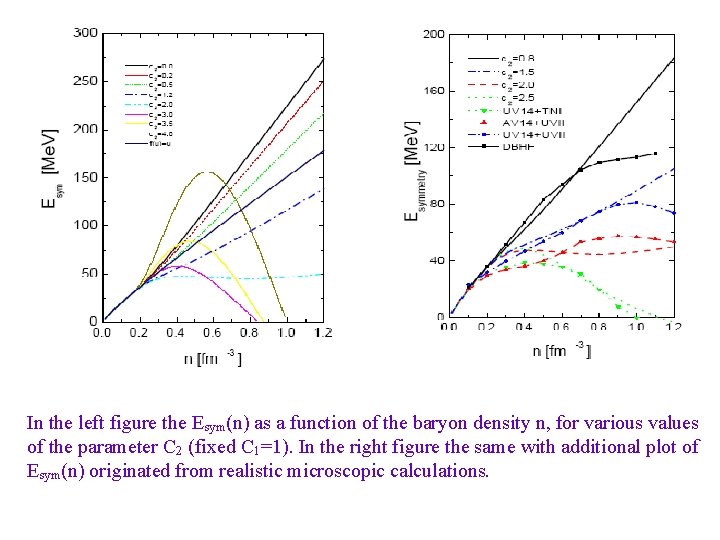
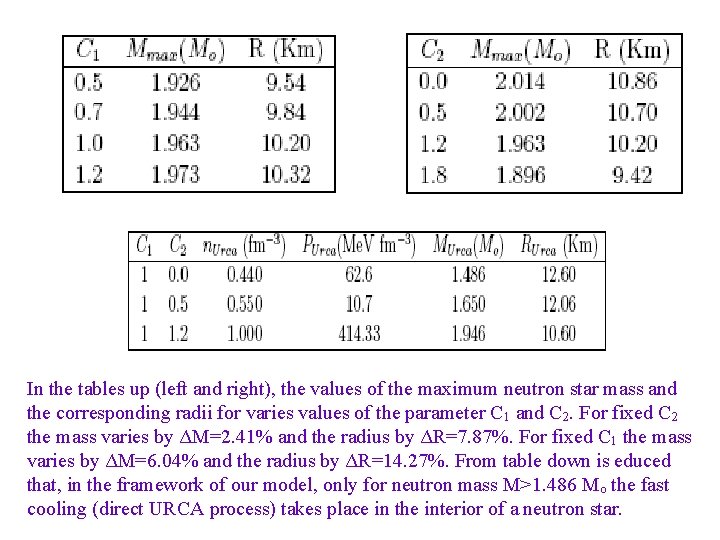
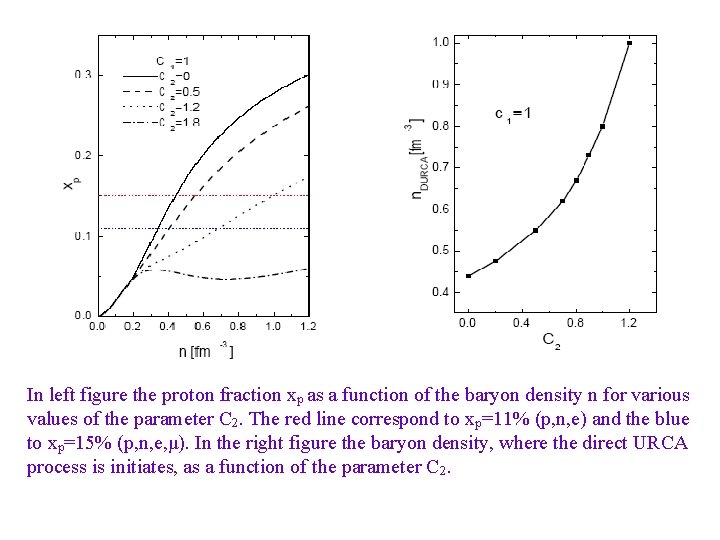
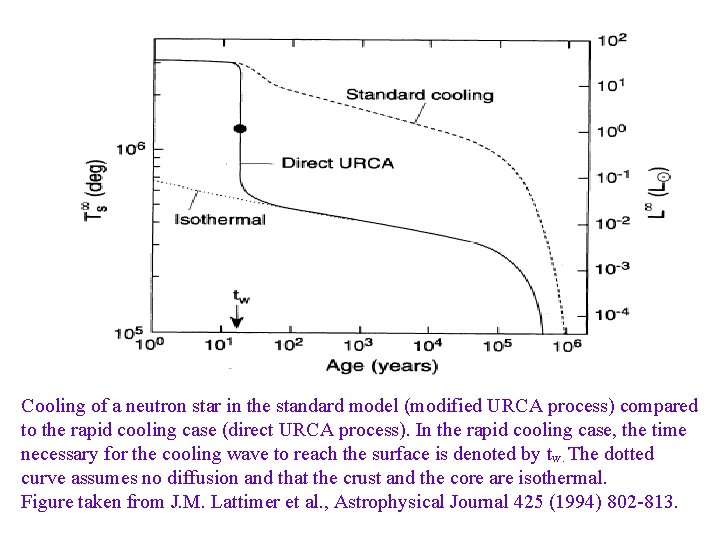
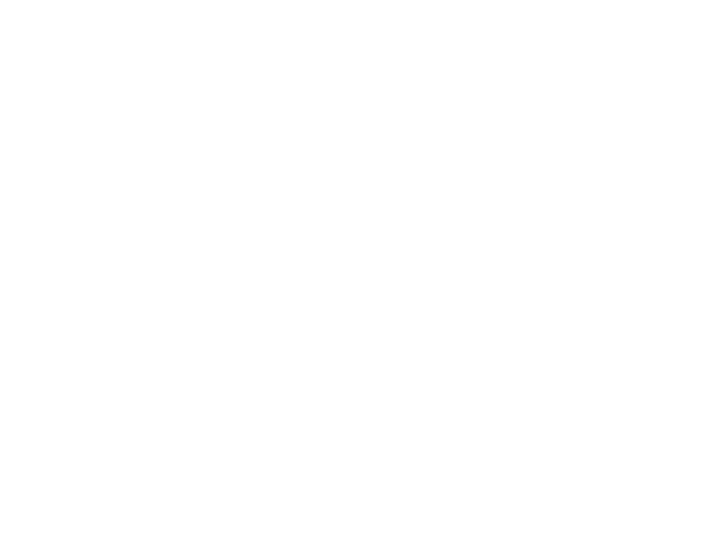
- Slides: 15
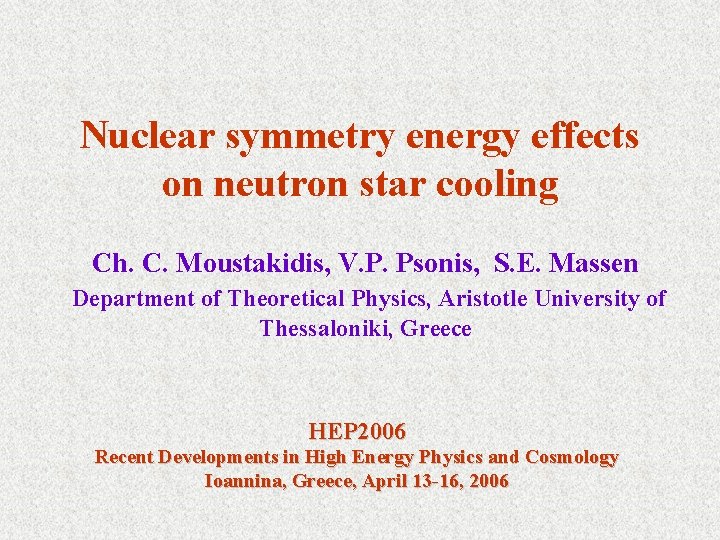
Nuclear symmetry energy effects on neutron star cooling Ch. C. Moustakidis, V. P. Psonis, S. E. Massen Department of Theoretical Physics, Aristotle University of Thessaloniki, Greece HEP 2006 Recent Developments in High Energy Physics and Cosmology Ioannina, Greece, April 13 -16, 2006
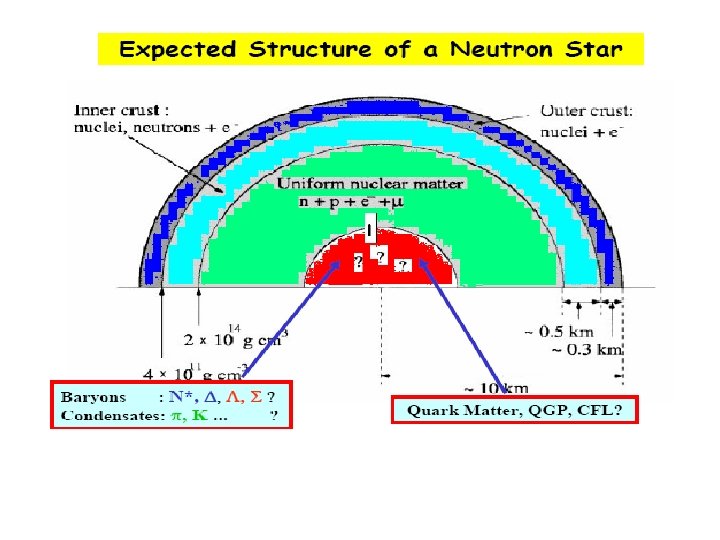
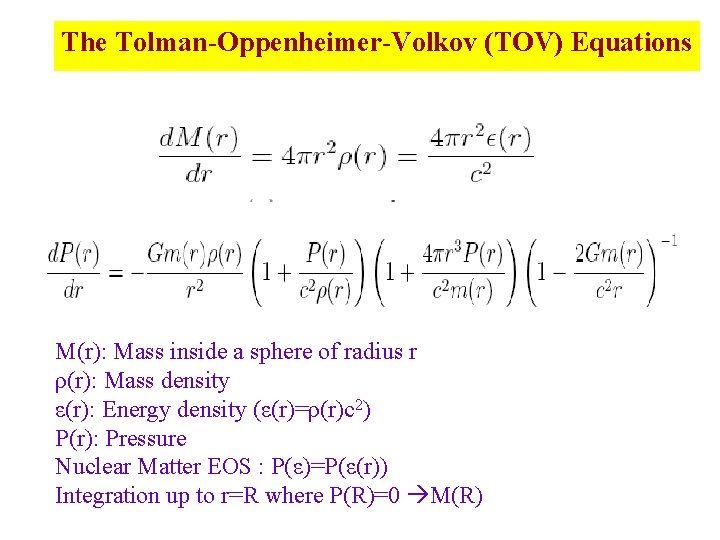
The Tolman-Oppenheimer-Volkov (TOV) Equations M(r): Mass inside a sphere of radius r ρ(r): Mass density ε(r): Energy density (ε(r)=ρ(r)c 2) P(r): Pressure Nuclear Matter EOS : P(ε)=P(ε(r)) Integration up to r=R where P(R)=0 M(R)
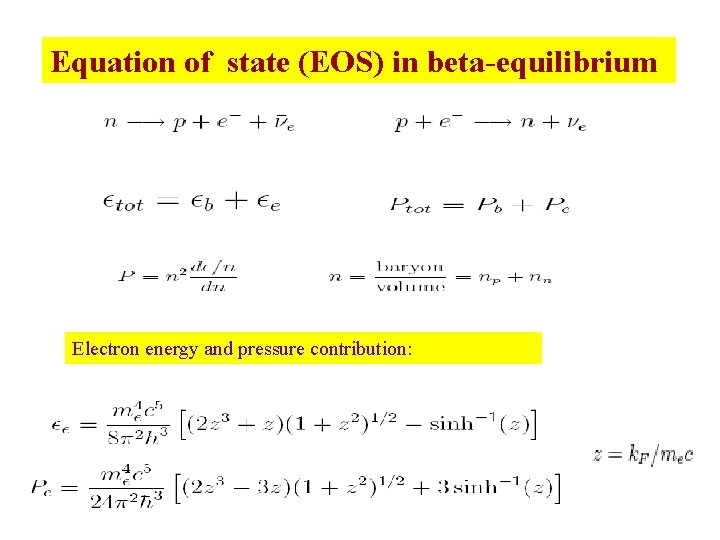
Equation of state (EOS) in beta-equilibrium Electron energy and pressure contribution:
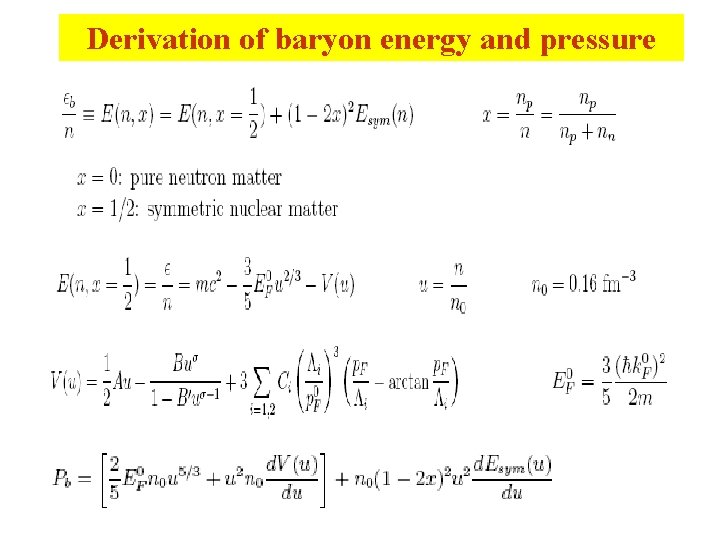
Derivation of baryon energy and pressure
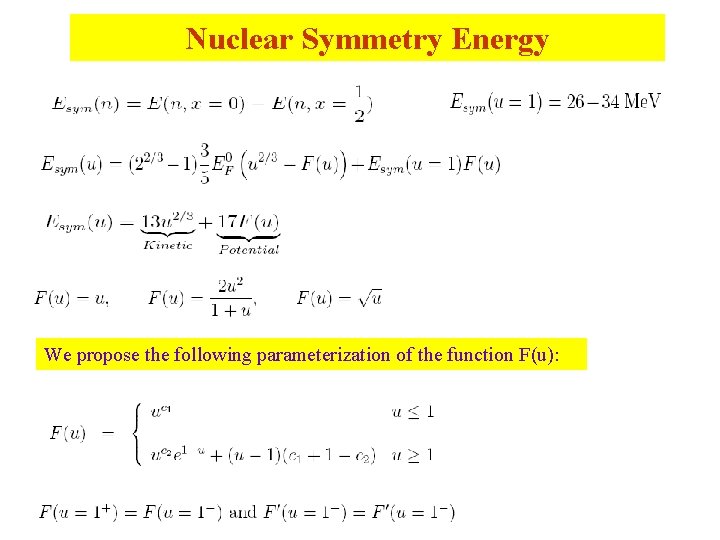
Nuclear Symmetry Energy We propose the following parameterization of the function F(u):
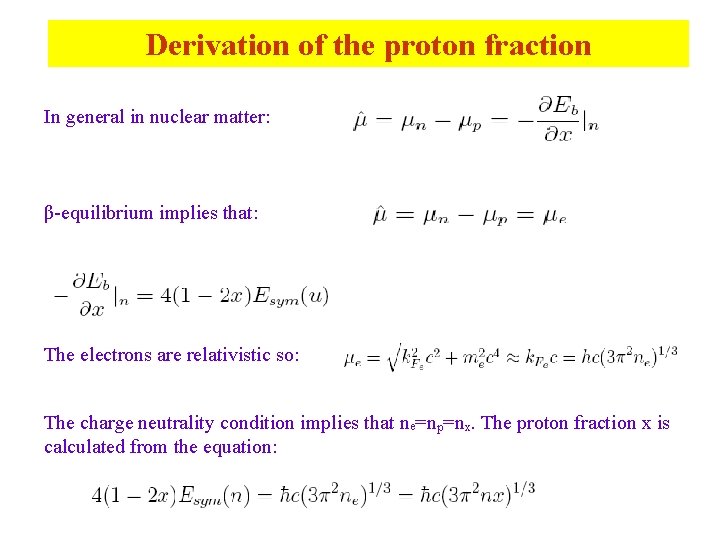
Derivation of the proton fraction In general in nuclear matter: β-equilibrium implies that: The electrons are relativistic so: The charge neutrality condition implies that ne=np=nx. The proton fraction x is calculated from the equation:
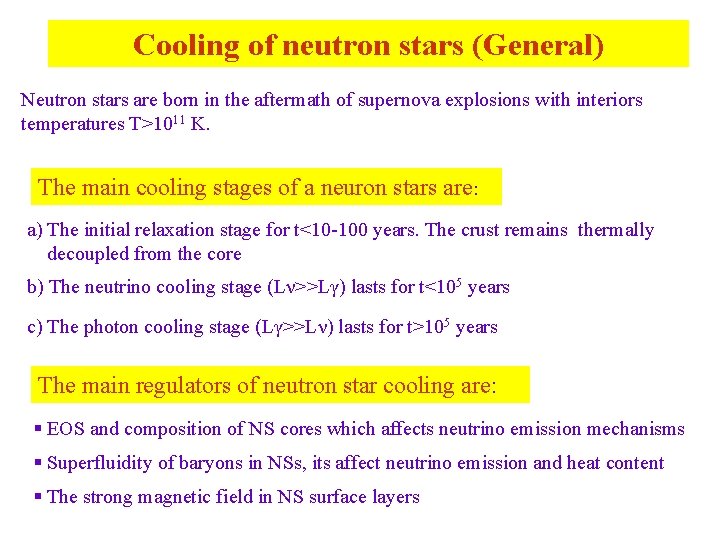
Cooling of neutron stars (General) Neutron stars are born in the aftermath of supernova explosions with interiors temperatures T>1011 K. The main cooling stages of a neuron stars are: a) The initial relaxation stage for t<10 -100 years. The crust remains thermally decoupled from the core b) The neutrino cooling stage (Lν>>Lγ) lasts for t<105 years c) The photon cooling stage (Lγ>>Lν) lasts for t>105 years The main regulators of neutron star cooling are: § EOS and composition of NS cores which affects neutrino emission mechanisms § Superfluidity of baryons in NSs, its affect neutrino emission and heat content § The strong magnetic field in NS surface layers
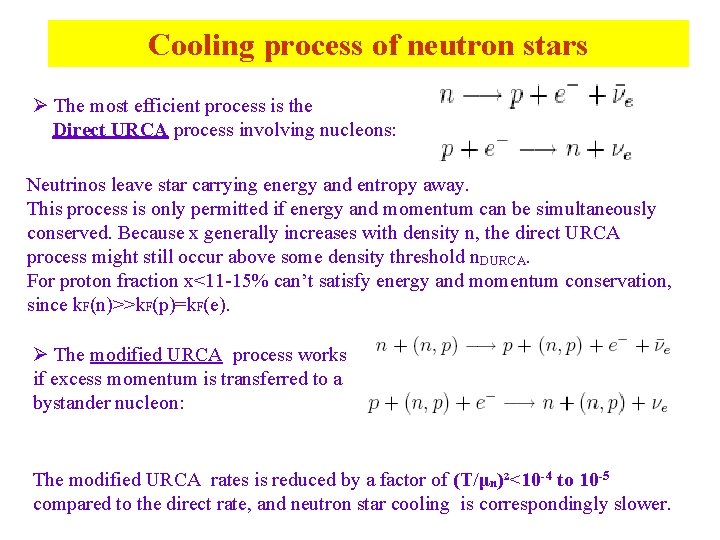
Cooling process of neutron stars Ø The most efficient process is the Direct URCA process involving nucleons: Neutrinos leave star carrying energy and entropy away. This process is only permitted if energy and momentum can be simultaneously conserved. Because x generally increases with density n, the direct URCA process might still occur above some density threshold n. DURCA. For proton fraction x<11 -15% can’t satisfy energy and momentum conservation, since k. F(n)>>k. F(p)=k. F(e). Ø The modified URCA process works if excess momentum is transferred to a bystander nucleon: The modified URCA rates is reduced by a factor of (T/μn)²<10 -4 to 10 -5 compared to the direct rate, and neutron star cooling is correspondingly slower.
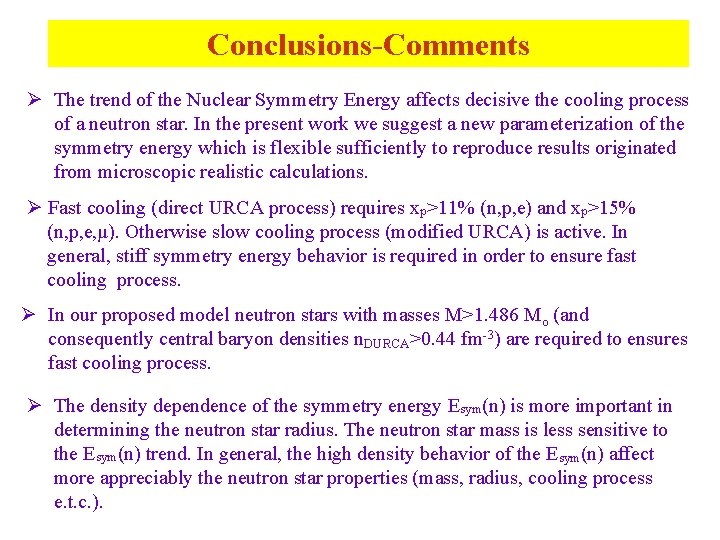
Conclusions-Comments Ø The trend of the Nuclear Symmetry Energy affects decisive the cooling process of a neutron star. In the present work we suggest a new parameterization of the symmetry energy which is flexible sufficiently to reproduce results originated from microscopic realistic calculations. Ø Fast cooling (direct URCA process) requires xp>11% (n, p, e) and xp>15% (n, p, e, μ). Otherwise slow cooling process (modified URCA) is active. In general, stiff symmetry energy behavior is required in order to ensure fast cooling process. Ø In our proposed model neutron stars with masses M>1. 486 Mo (and consequently central baryon densities n. DURCA>0. 44 fm-3) are required to ensures fast cooling process. Ø The density dependence of the symmetry energy Esym(n) is more important in determining the neutron star radius. The neutron star mass is less sensitive to the Esym(n) trend. In general, the high density behavior of the Esym(n) affect more appreciably the neutron star properties (mass, radius, cooling process e. t. c. ).
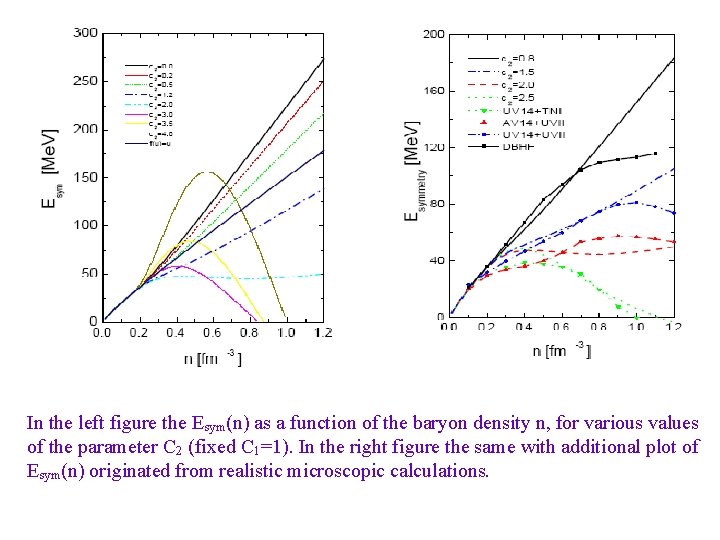
In the left figure the Esym(n) as a function of the baryon density n, for various values of the parameter C 2 (fixed C 1=1). In the right figure the same with additional plot of Esym(n) originated from realistic microscopic calculations.
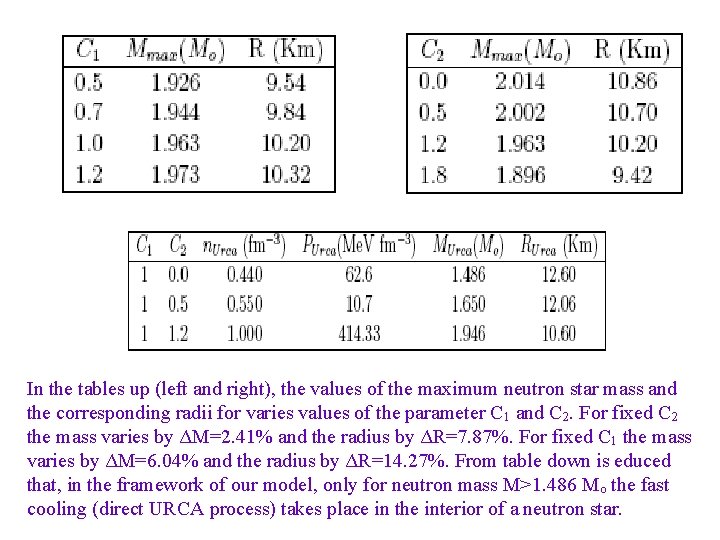
In the tables up (left and right), the values of the maximum neutron star mass and the corresponding radii for varies values of the parameter C 1 and C 2. For fixed C 2 the mass varies by ΔΜ=2. 41% and the radius by ΔR=7. 87%. For fixed C 1 the mass varies by ΔΜ=6. 04% and the radius by ΔR=14. 27%. From table down is educed that, in the framework of our model, only for neutron mass M>1. 486 Mo the fast cooling (direct URCA process) takes place in the interior of a neutron star.
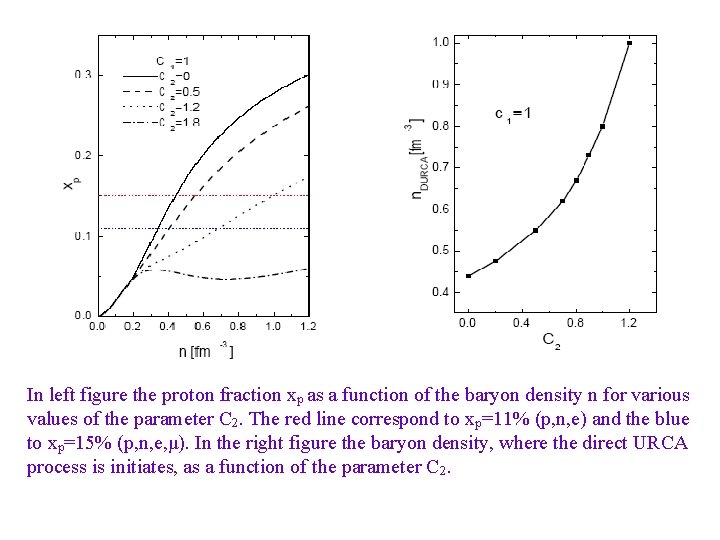
In left figure the proton fraction xp as a function of the baryon density n for various values of the parameter C 2. The red line correspond to xp=11% (p, n, e) and the blue to xp=15% (p, n, e, μ). In the right figure the baryon density, where the direct URCA process is initiates, as a function of the parameter C 2.
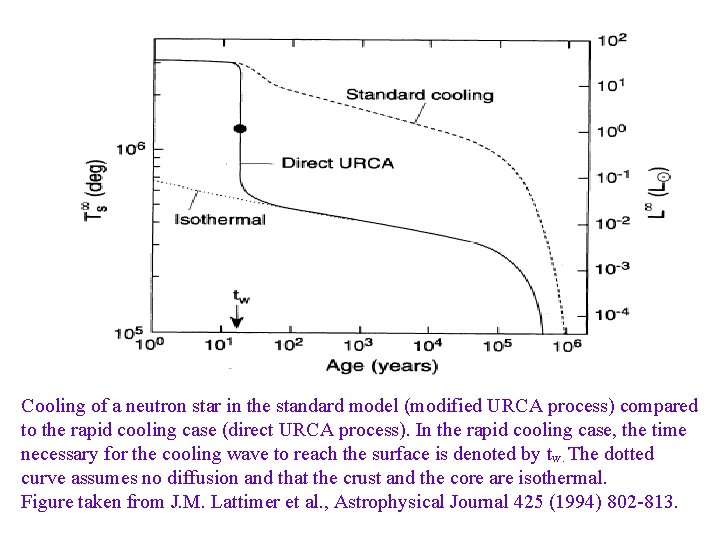
Cooling of a neutron star in the standard model (modified URCA process) compared to the rapid cooling case (direct URCA process). In the rapid cooling case, the time necessary for the cooling wave to reach the surface is denoted by tw. The dotted curve assumes no diffusion and that the crust and the core are isothermal. Figure taken from J. M. Lattimer et al. , Astrophysical Journal 425 (1994) 802 -813.
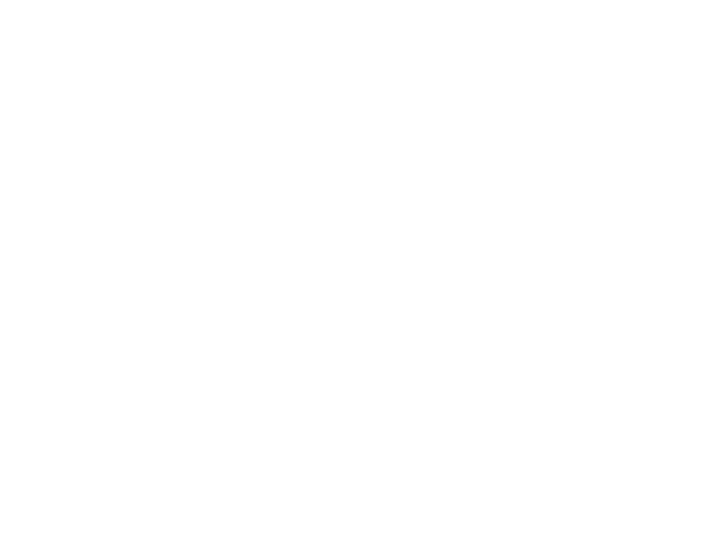
Star of wonder star of night star of royal beauty bright
Anatomy of a star
Neutron star
Neutron star
Neutron degeneracy pressure
Escape velocity of neutron star
Neutron star
Neutron star
Neutron star
Neutron star
Neutron star
Neutron star
Star objective
Binary neutron star
Neutron star router
Lesson 15 nuclear quest nuclear reactions