Microfluidic Applications of InducedCharge Electroosmosis Jeremy Levitan Mechanical
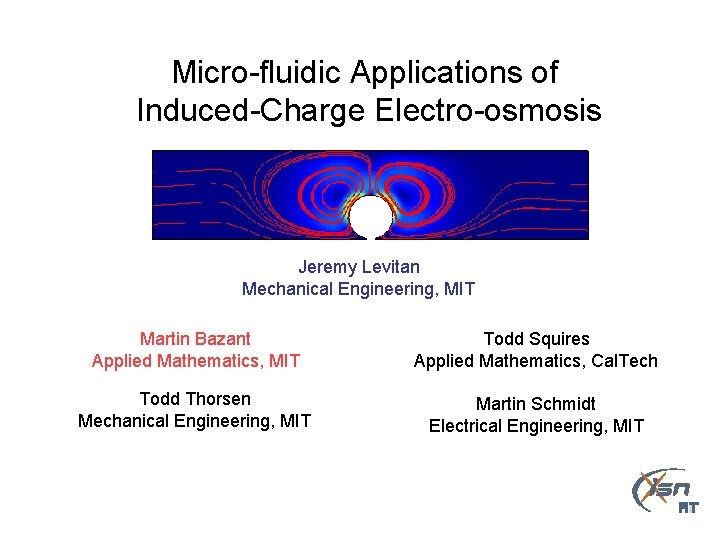
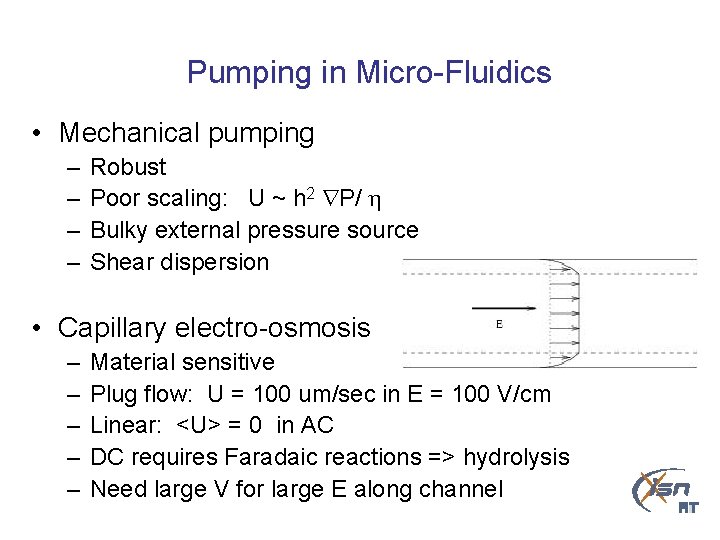
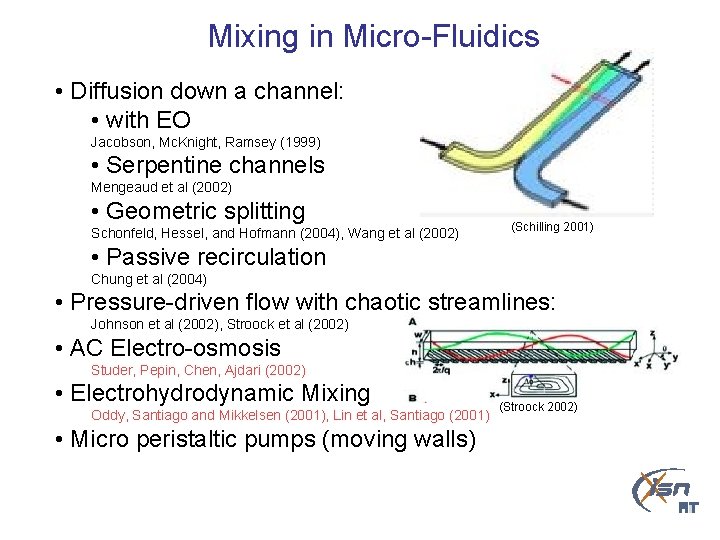
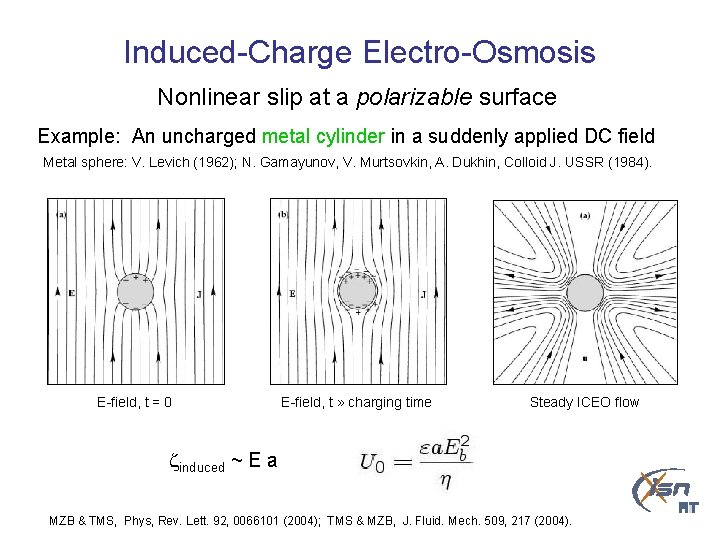
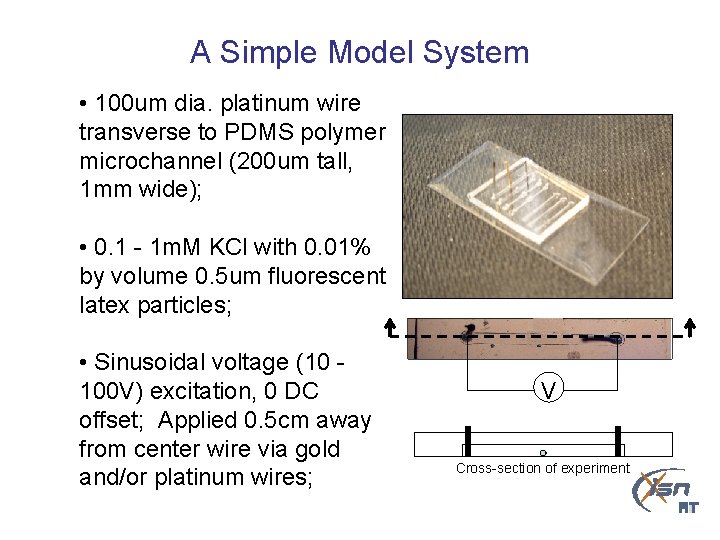
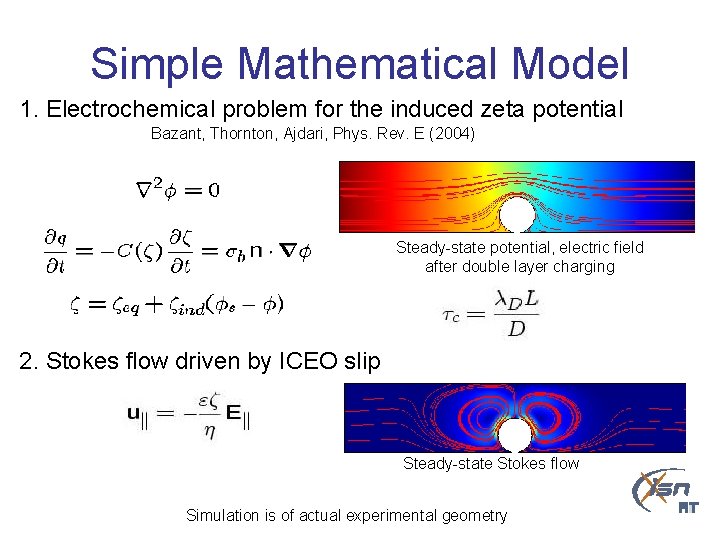
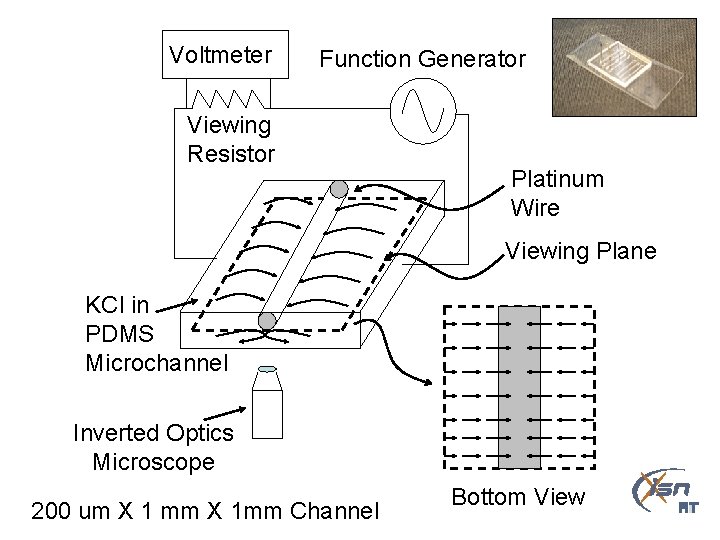
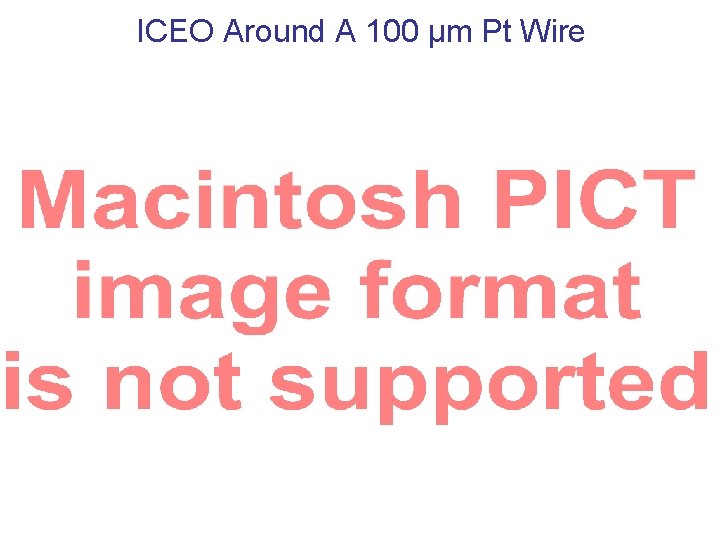
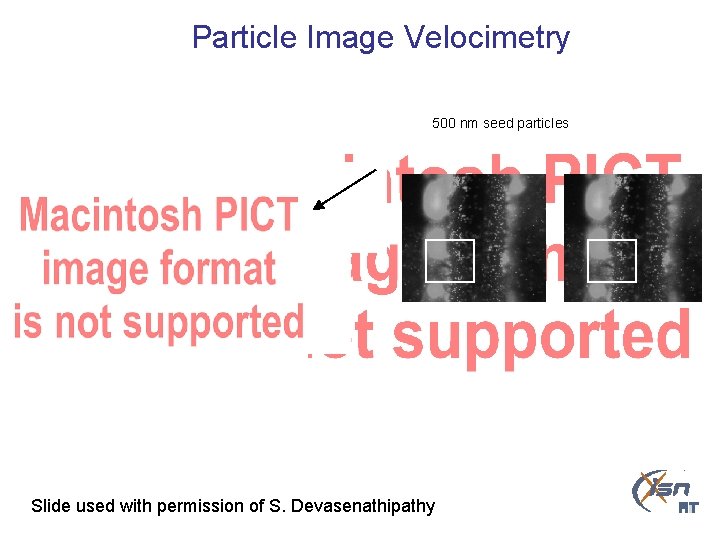
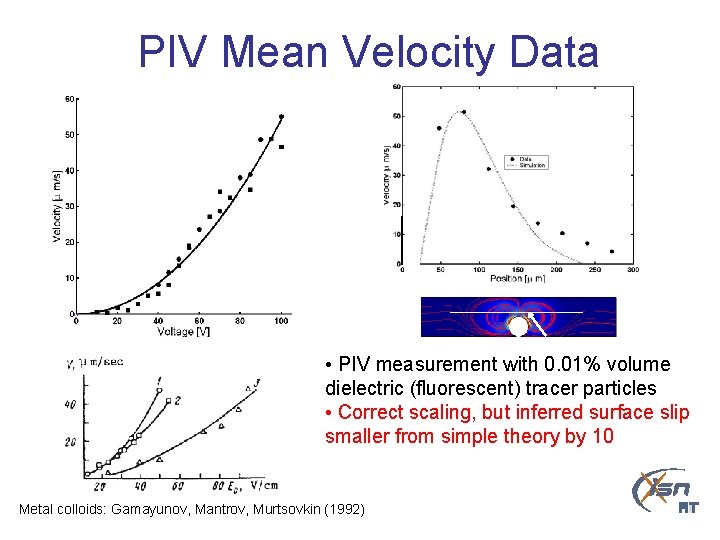
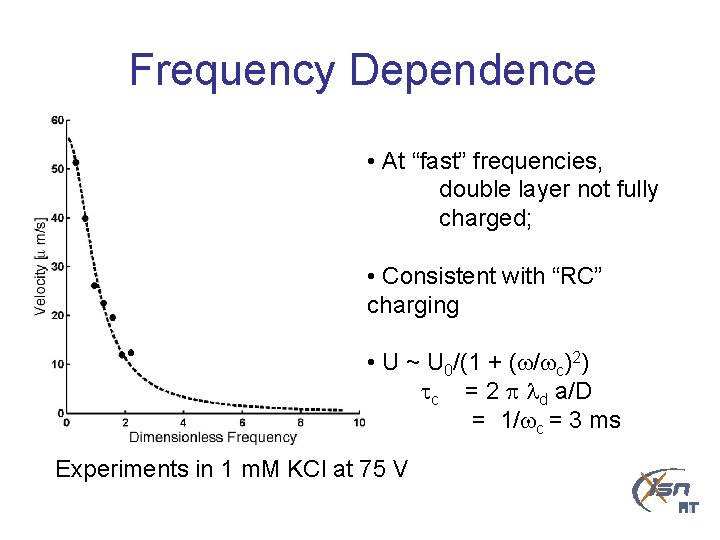
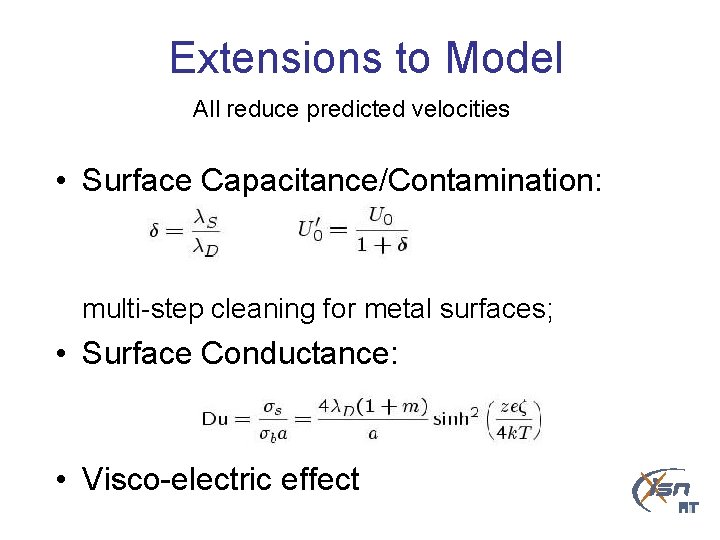
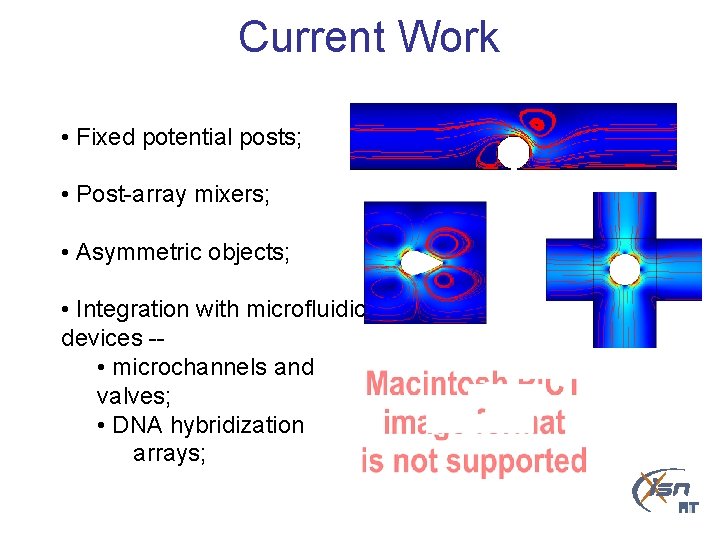
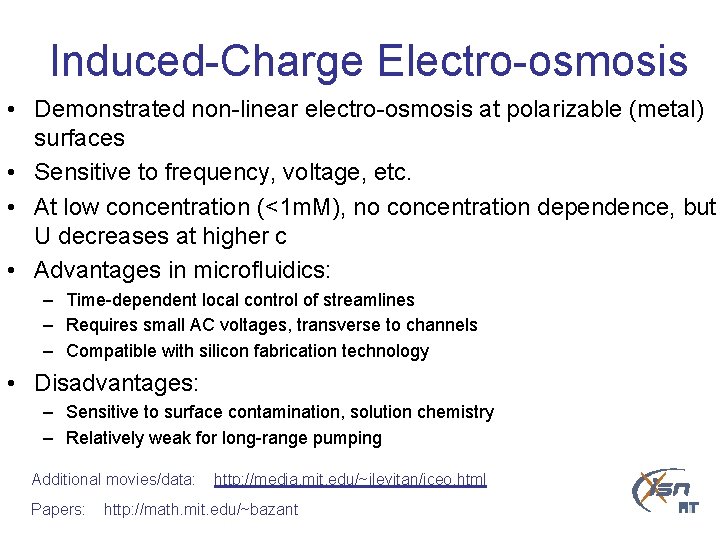
- Slides: 14
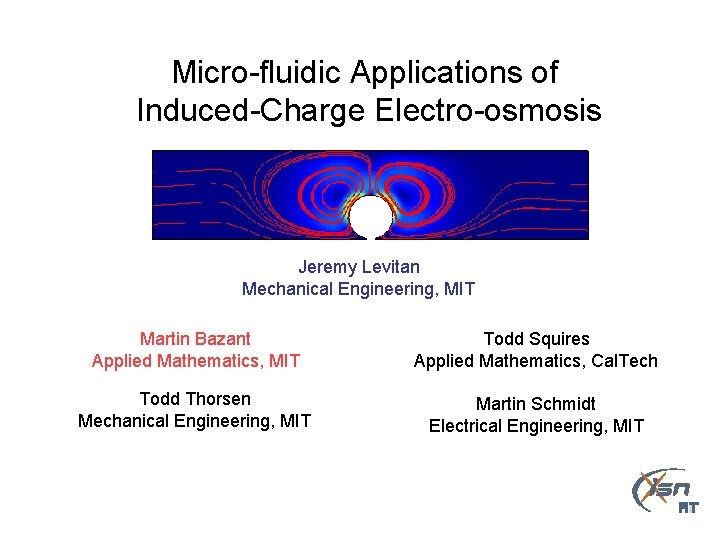
Micro-fluidic Applications of Induced-Charge Electro-osmosis Jeremy Levitan Mechanical Engineering, MIT Martin Bazant Applied Mathematics, MIT Todd Squires Applied Mathematics, Cal. Tech Todd Thorsen Mechanical Engineering, MIT Martin Schmidt Electrical Engineering, MIT
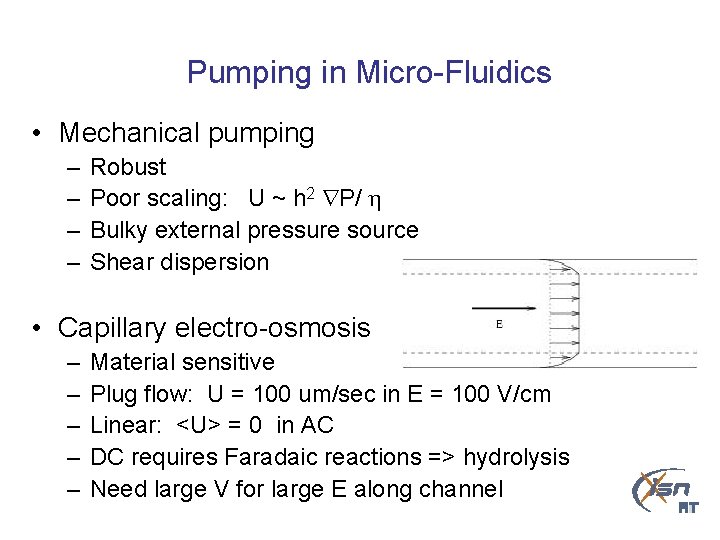
Pumping in Micro-Fluidics • Mechanical pumping – – Robust Poor scaling: U ~ h 2 P/ Bulky external pressure source Shear dispersion • Capillary electro-osmosis – – – Material sensitive Plug flow: U = 100 um/sec in E = 100 V/cm Linear: <U> = 0 in AC DC requires Faradaic reactions => hydrolysis Need large V for large E along channel
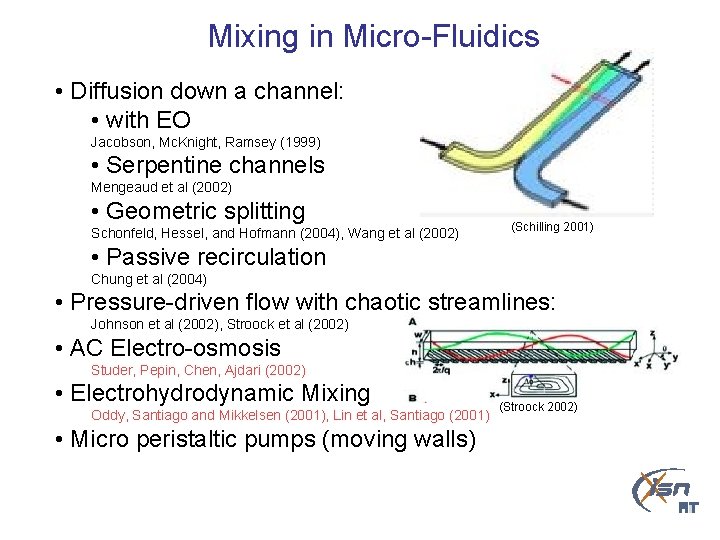
Mixing in Micro-Fluidics • Diffusion down a channel: • with EO Jacobson, Mc. Knight, Ramsey (1999) • Serpentine channels Mengeaud et al (2002) • Geometric splitting Schonfeld, Hessel, and Hofmann (2004), Wang et al (2002) (Schilling 2001) • Passive recirculation Chung et al (2004) • Pressure-driven flow with chaotic streamlines: Johnson et al (2002), Stroock et al (2002) • AC Electro-osmosis Studer, Pepin, Chen, Ajdari (2002) • Electrohydrodynamic Mixing Oddy, Santiago and Mikkelsen (2001), Lin et al, Santiago (2001) • Micro peristaltic pumps (moving walls) (Stroock 2002)
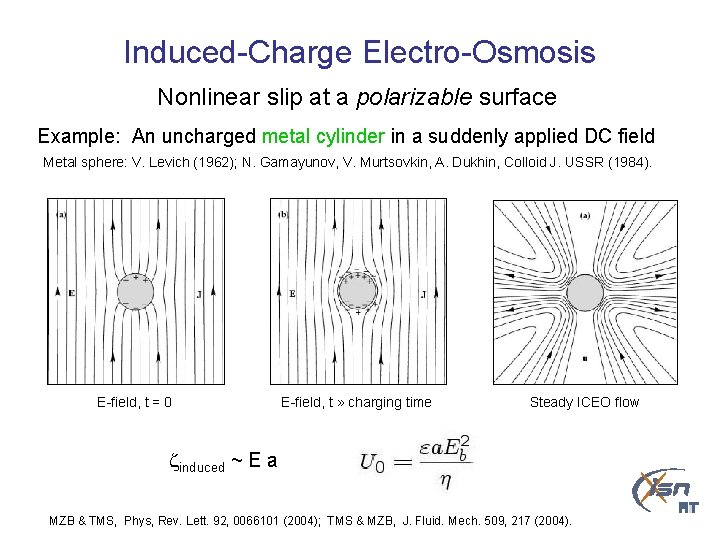
Induced-Charge Electro-Osmosis Nonlinear slip at a polarizable surface Example: An uncharged metal cylinder in a suddenly applied DC field Metal sphere: V. Levich (1962); N. Gamayunov, V. Murtsovkin, A. Dukhin, Colloid J. USSR (1984). E-field, t = 0 E-field, t » charging time Steady ICEO flow induced ~ E a MZB & TMS, Phys, Rev. Lett. 92, 0066101 (2004); TMS & MZB, J. Fluid. Mech. 509, 217 (2004).
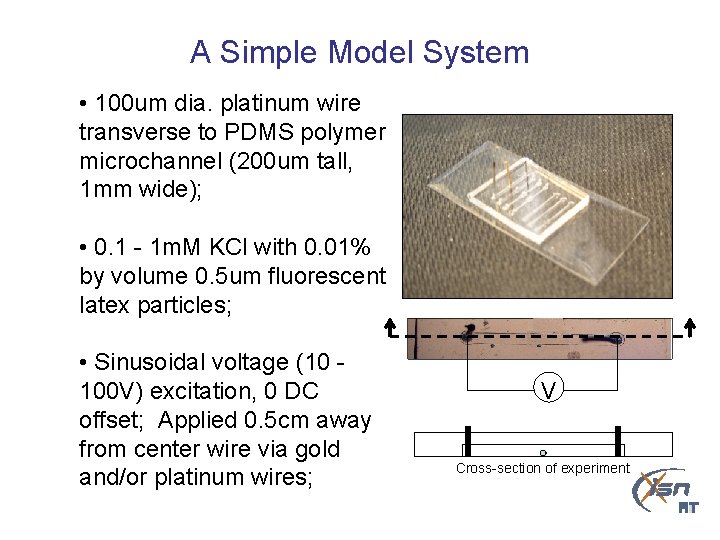
A Simple Model System • 100 um dia. platinum wire transverse to PDMS polymer microchannel (200 um tall, 1 mm wide); • 0. 1 - 1 m. M KCl with 0. 01% by volume 0. 5 um fluorescent latex particles; • Sinusoidal voltage (10 100 V) excitation, 0 DC offset; Applied 0. 5 cm away from center wire via gold and/or platinum wires; V Cross-section of experiment
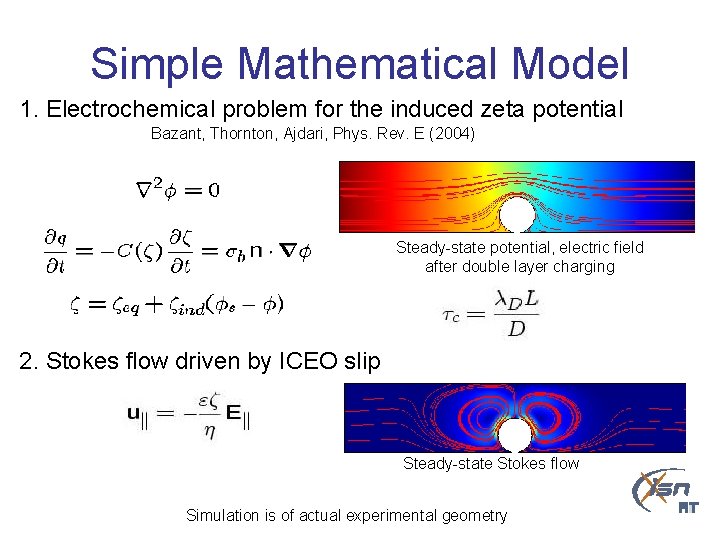
Simple Mathematical Model 1. Electrochemical problem for the induced zeta potential Bazant, Thornton, Ajdari, Phys. Rev. E (2004) Steady-state potential, electric field after double layer charging 2. Stokes flow driven by ICEO slip Steady-state Stokes flow Simulation is of actual experimental geometry
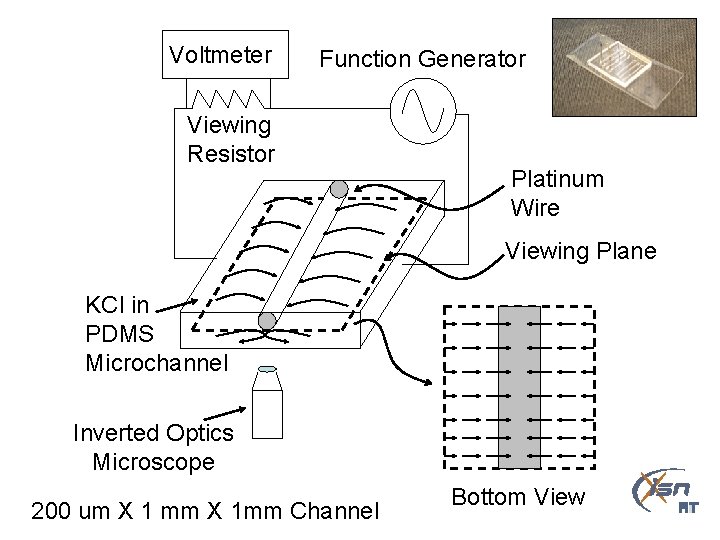
Voltmeter Function Generator Viewing Resistor Platinum Wire Viewing Plane KCl in PDMS Microchannel Inverted Optics Microscope 200 um X 1 mm X 1 mm Channel Bottom View
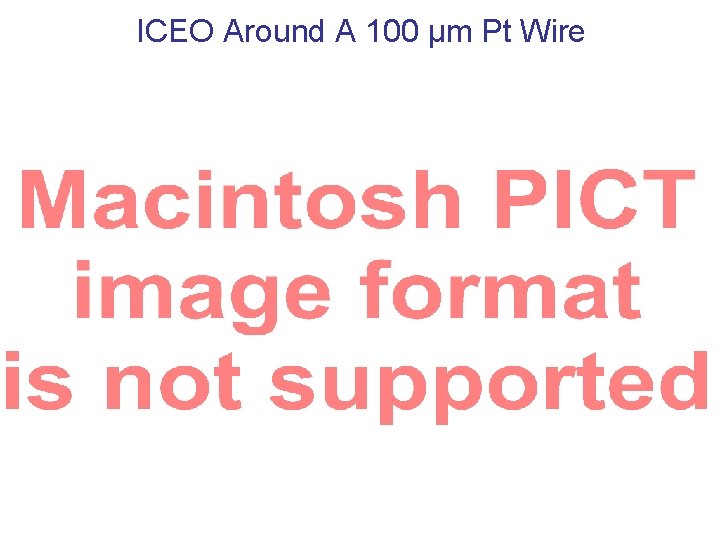
ICEO Around A 100 µm Pt Wire
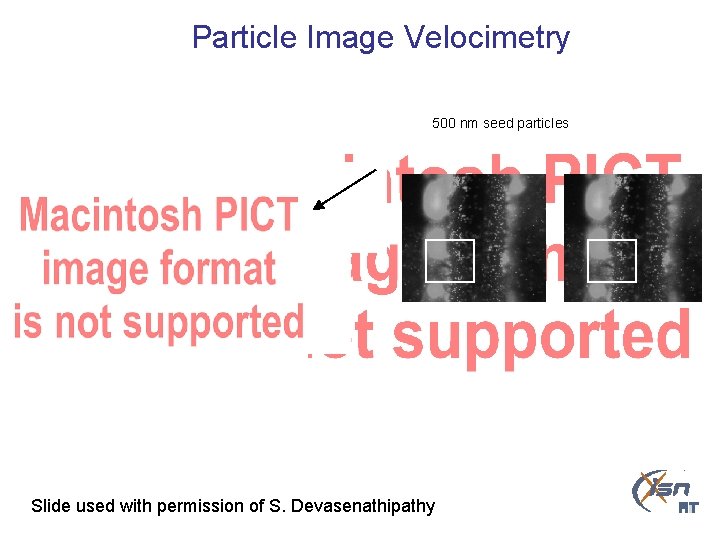
Particle Image Velocimetry 500 nm seed particles Slide used with permission of S. Devasenathipathy
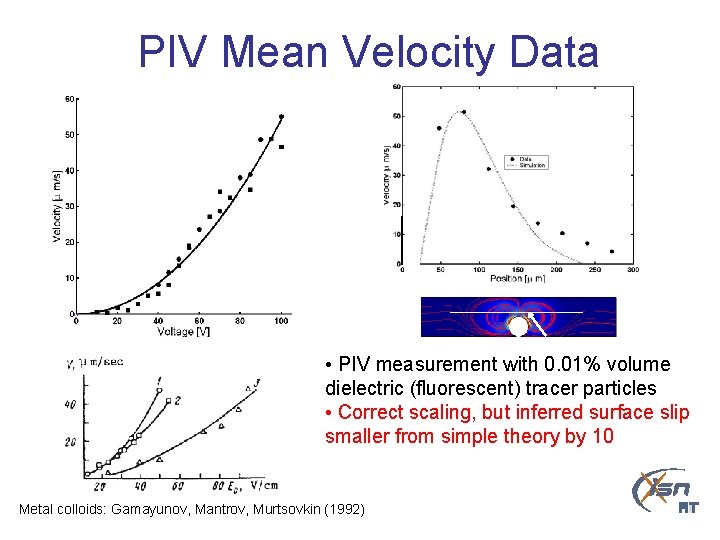
PIV Mean Velocity Data • PIV measurement with 0. 01% volume dielectric (fluorescent) tracer particles • Correct scaling, but inferred surface slip smaller from simple theory by 10 Metal colloids: Gamayunov, Mantrov, Murtsovkin (1992)
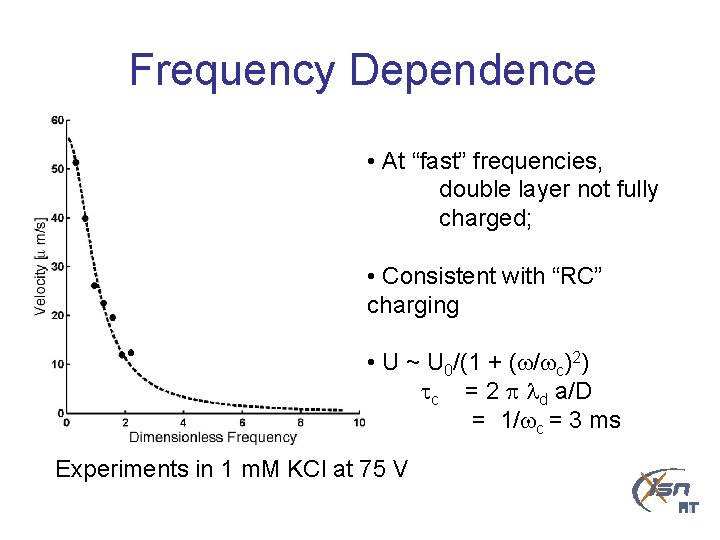
Frequency Dependence • At “fast” frequencies, double layer not fully charged; • Consistent with “RC” charging • U ~ U 0/(1 + ( / c)2) c = 2 d a/D = 1/ c = 3 ms Experiments in 1 m. M KCl at 75 V
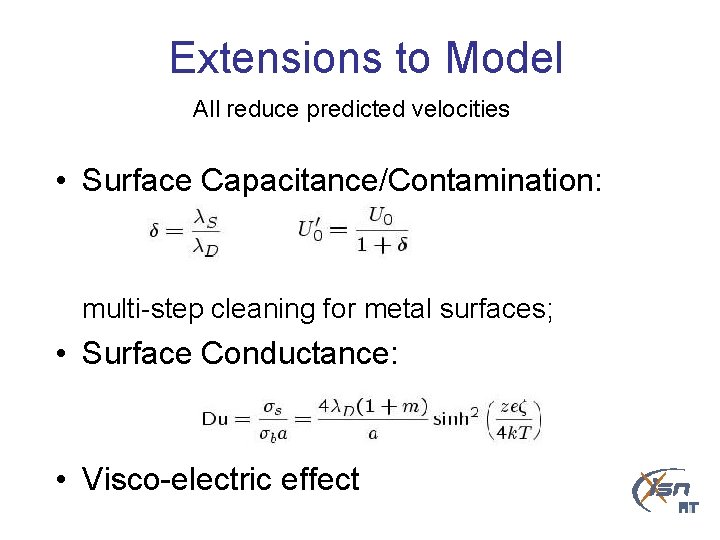
Extensions to Model All reduce predicted velocities • Surface Capacitance/Contamination: multi-step cleaning for metal surfaces; • Surface Conductance: • Visco-electric effect
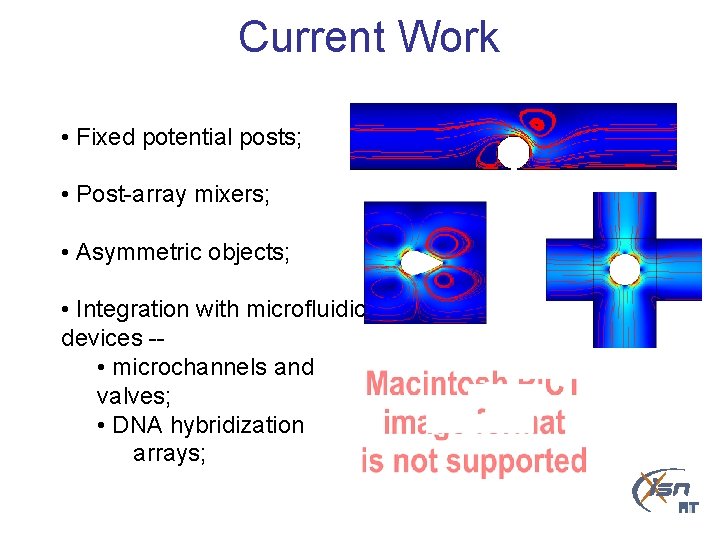
Current Work • Fixed potential posts; • Post-array mixers; • Asymmetric objects; • Integration with microfluidic devices - • microchannels and valves; • DNA hybridization arrays;
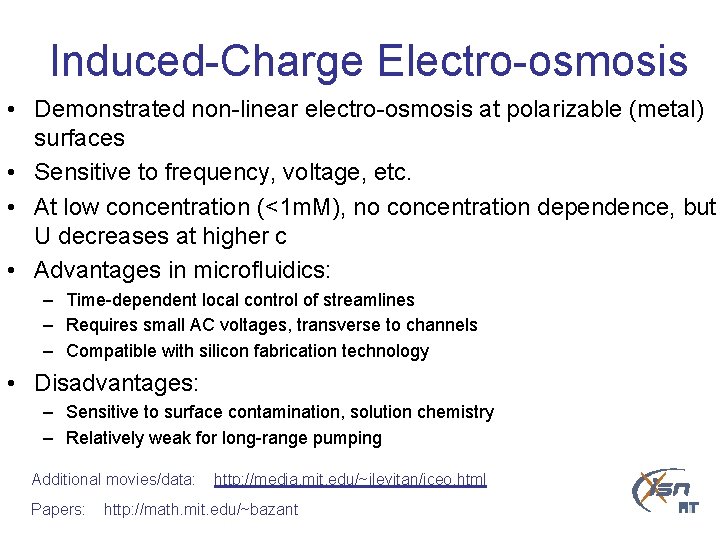
Induced-Charge Electro-osmosis • Demonstrated non-linear electro-osmosis at polarizable (metal) surfaces • Sensitive to frequency, voltage, etc. • At low concentration (<1 m. M), no concentration dependence, but U decreases at higher c • Advantages in microfluidics: – Time-dependent local control of streamlines – Requires small AC voltages, transverse to channels – Compatible with silicon fabrication technology • Disadvantages: – Sensitive to surface contamination, solution chemistry – Relatively weak for long-range pumping Additional movies/data: Papers: http: //media. mit. edu/~jlevitan/iceo. html http: //math. mit. edu/~bazant