Large Eddy Simulations of Compositional Density Currents Flowing
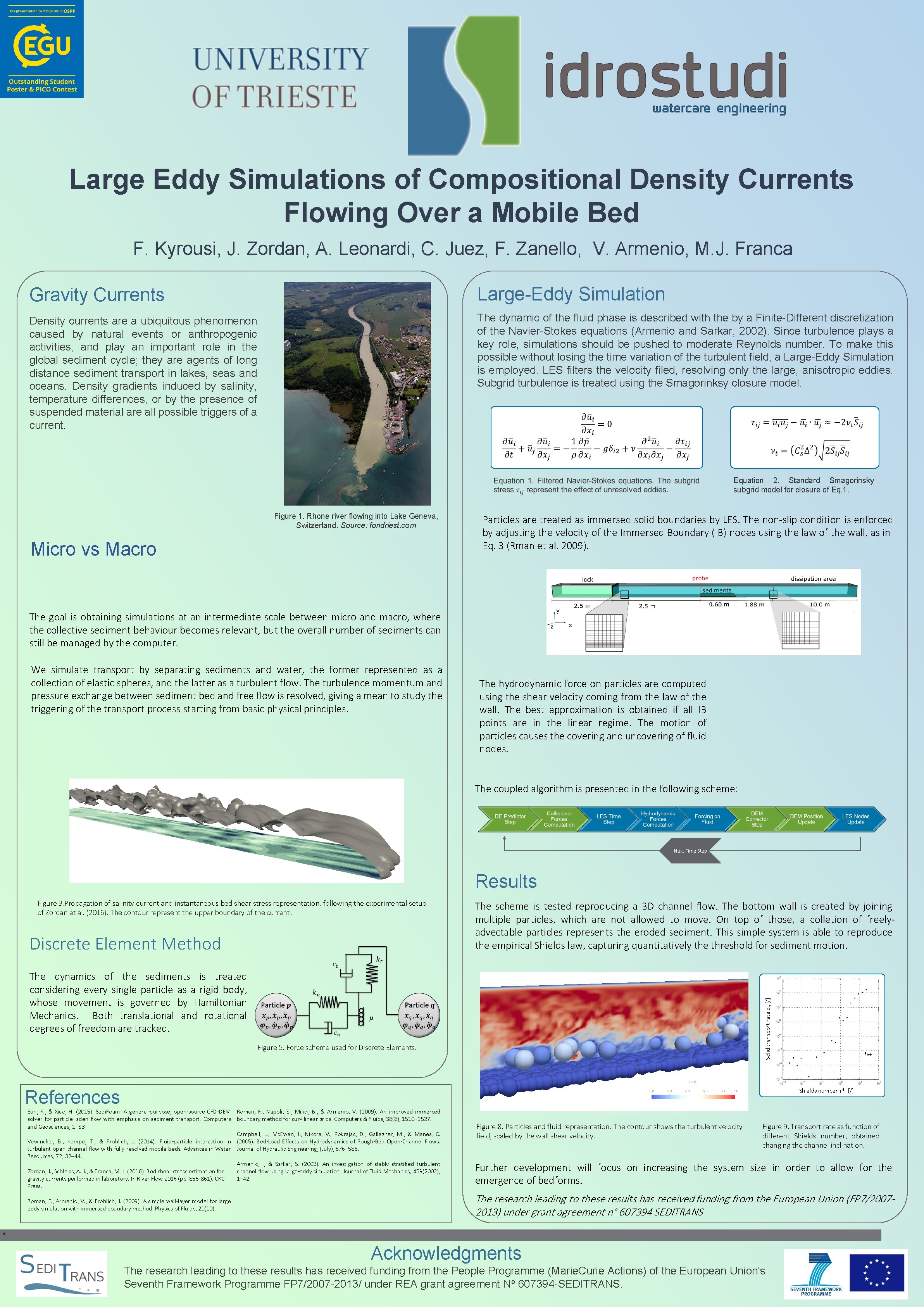
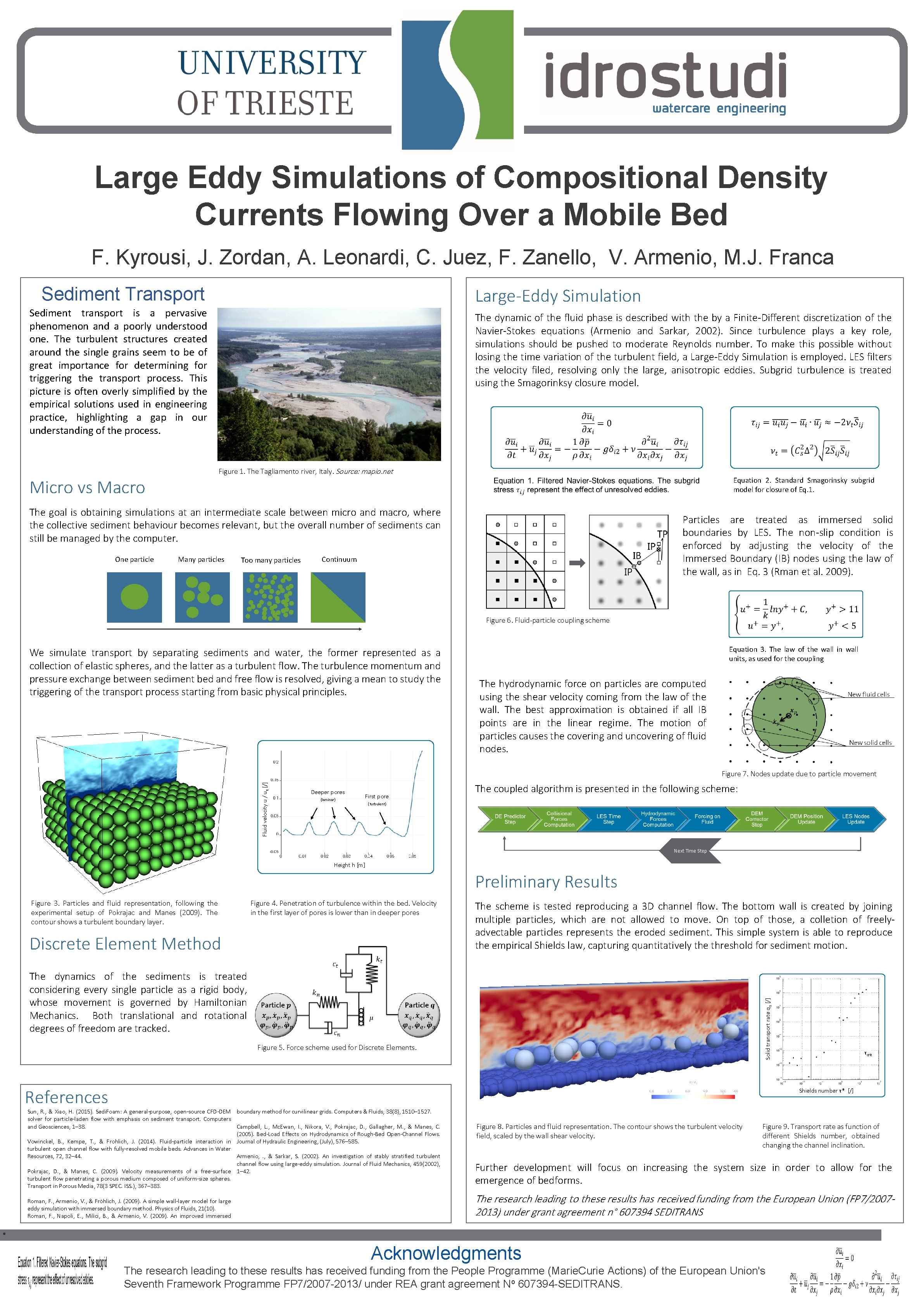
- Slides: 2
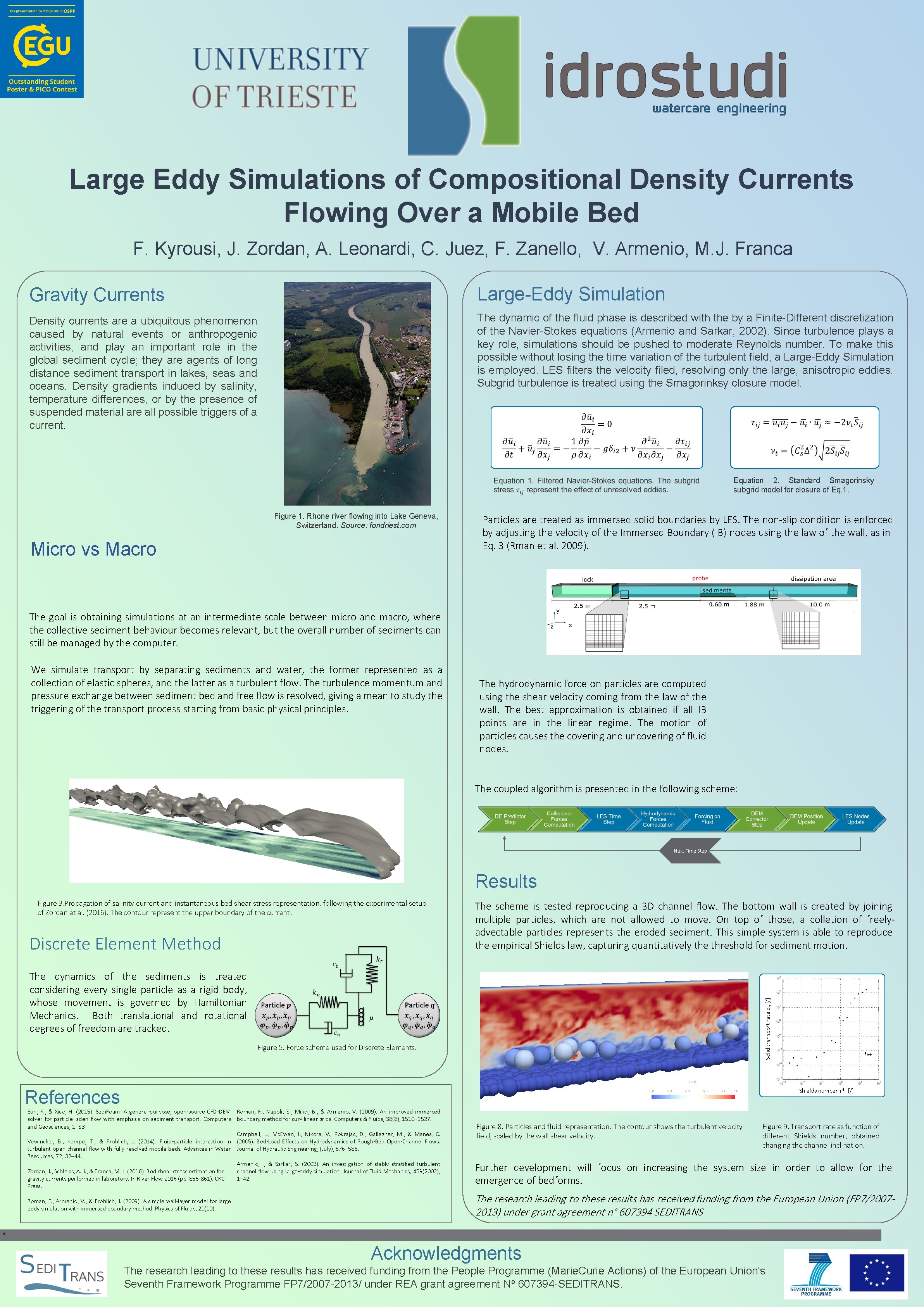
Large Eddy Simulations of Compositional Density Currents Flowing Over a Mobile Bed F. Kyrousi, J. Zordan, A. Leonardi, C. Juez, F. Zanello, V. Armenio, M. J. Franca Gravity Currents Large-Eddy Simulation Density currents are a ubiquitous phenomenon caused by natural events or anthropogenic activities, and play an important role in the global sediment cycle; they are agents of long distance sediment transport in lakes, seas and oceans. Density gradients induced by salinity, temperature differences, or by the presence of suspended material are all possible triggers of a current. The dynamic of the fluid phase is described with the by a Finite-Different discretization of the Navier-Stokes equations (Armenio and Sarkar, 2002). Since turbulence plays a key role, simulations should be pushed to moderate Reynolds number. To make this possible without losing the time variation of the turbulent field, a Large-Eddy Simulation is employed. LES filters the velocity filed, resolving only the large, anisotropic eddies. Subgrid turbulence is treated using the Smagorinksy closure model. Equation 2. Standard Smagorinsky subgrid model for closure of Eq. 1. Figure 1. Rhone river flowing into Lake Geneva, Switzerland. Source: fondriest. com Micro vs Macro Particles are treated as immersed solid boundaries by LES. The non-slip condition is enforced by adjusting the velocity of the Immersed Boundary (IB) nodes using the law of the wall, as in Eq. 3 (Rman et al. 2009). The goal is obtaining simulations at an intermediate scale between micro and macro, where the collective sediment behaviour becomes relevant, but the overall number of sediments can still be managed by the computer. We simulate transport by separating sediments and water, the former represented as a collection of elastic spheres, and the latter as a turbulent flow. The turbulence momentum and pressure exchange between sediment bed and free flow is resolved, giving a mean to study the triggering of the transport process starting from basic physical principles. The hydrodynamic force on particles are computed using the shear velocity coming from the law of the wall. The best approximation is obtained if all IB points are in the linear regime. The motion of particles causes the covering and uncovering of fluid nodes. The coupled algorithm is presented in the following scheme: Next Time Step Results Figure 3. Propagation of salinity current and instantaneous bed shear stress representation, following the experimental setup of Zordan et al. (2016). The contour represent the upper boundary of the current. Discrete Element Method The scheme is tested reproducing a 3 D channel flow. The bottom wall is created by joining multiple particles, which are not allowed to move. On top of those, a colletion of freelyadvectable particles represents the eroded sediment. This simple system is able to reproduce the empirical Shields law, capturing quantitatively the threshold for sediment motion. Solid transport rate qs [/] The dynamics of the sediments is treated considering every single particle as a rigid body, whose movement is governed by Hamiltonian Mechanics. Both translational and rotational degrees of freedom are tracked. Figure 5. Force scheme used for Discrete Elements. τcrit Shields number τ* [/] References Sun, R. , & Xiao, H. (2015). Sedi. Foam: A general-purpose, open-source CFD-DEM Roman, F. , Napoli, E. , Milici, B. , & Armenio, V. (2009). An improved immersed solver for particle-laden flow with emphasis on sediment transport. Computers boundary method for curvilinear grids. Computers & Fluids, 38(8), 1510– 1527. and Geosciences, 1– 38. Campbell, L. , Mc. Ewan, I. , Nikora, V. , Pokrajac, D. , Gallagher, M. , & Manes, C. Vowinckel, B. , Kempe, T. , & Frohlich, J. (2014). Fluid-particle interaction in (2005). Bed-Load Effects on Hydrodynamics of Rough-Bed Open-Channel Flows. turbulent open channel flow with fully-resolved mobile beds. Advances in Water Journal of Hydraulic Engineering, (July), 576– 585. Resources, 72, 32– 44. Armenio, . , & Sarkar, S. (2002). An investigation of stably stratified turbulent Zordan, J. , Schleiss, A. J. , & Franca, M. J. (2016). Bed shear stress estimation for channel flow using large-eddy simulation. Journal of Fluid Mechanics, 459(2002), gravity currents performed in laboratory. In River Flow 2016 (pp. 855 -861). CRC 1– 42. Press. Roman, F. , Armenio, V. , & Fröhlich, J. (2009). A simple wall-layer model for large eddy simulation with immersed boundary method. Physics of Fluids, 21(10). Figure 8. Particles and fluid representation. The contour shows the turbulent velocity field, scaled by the wall shear velocity. Figure 9. Transport rate as function of different Shields number, obtained changing the channel inclination. Further development will focus on increasing the system size in order to allow for the emergence of bedforms. The research leading to these results has received funding from the European Union (FP 7/20072013) under grant agreement n° 607394 SEDITRANS • Acknowledgments The research leading to these results has received funding from the People Programme (Marie. Curie Actions) of the European Union's Seventh Framework Programme FP 7/2007 -2013/ under REA grant agreement No 607394 -SEDITRANS.
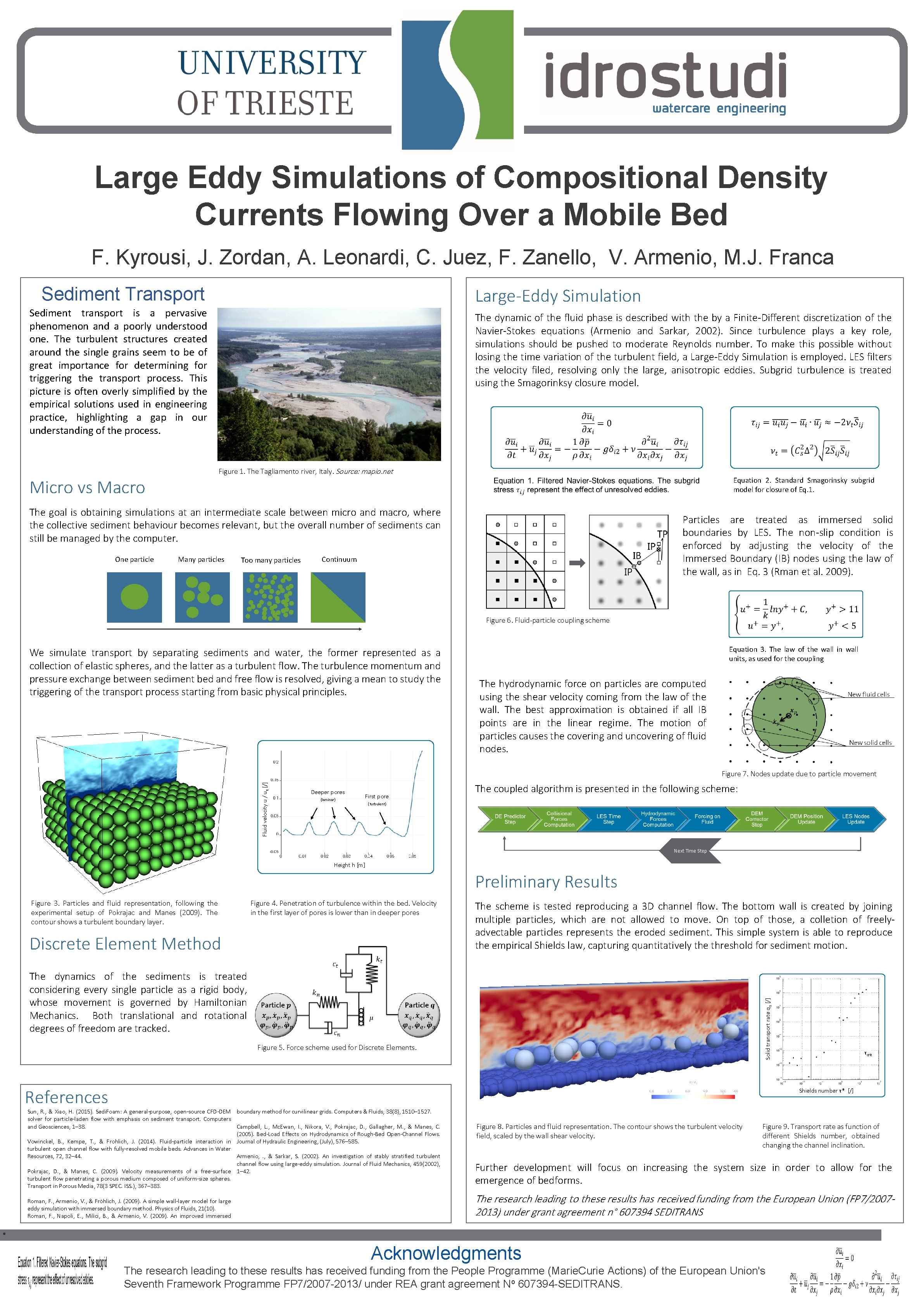
Large Eddy Simulations of Compositional Density Currents Flowing Over a Mobile Bed F. Kyrousi, J. Zordan, A. Leonardi, C. Juez, F. Zanello, V. Armenio, M. J. Franca Large-Eddy Simulation Sediment Transport Sediment transport is a pervasive phenomenon and a poorly understood one. The turbulent structures created around the single grains seem to be of great importance for determining for triggering the transport process. This picture is often overly simplified by the empirical solutions used in engineering practice, highlighting a gap in our understanding of the process. The dynamic of the fluid phase is described with the by a Finite-Different discretization of the Navier-Stokes equations (Armenio and Sarkar, 2002). Since turbulence plays a key role, simulations should be pushed to moderate Reynolds number. To make this possible without losing the time variation of the turbulent field, a Large-Eddy Simulation is employed. LES filters the velocity filed, resolving only the large, anisotropic eddies. Subgrid turbulence is treated using the Smagorinksy closure model. Figure 1. The Tagliamento river, Italy. Source: mapio. net Micro vs Macro Equation 2. Standard Smagorinsky subgrid model for closure of Eq. 1. The goal is obtaining simulations at an intermediate scale between micro and macro, where the collective sediment behaviour becomes relevant, but the overall number of sediments can still be managed by the computer. One particle Many particles Too many particles Particles are treated as immersed solid boundaries by LES. The non-slip condition is enforced by adjusting the velocity of the Immersed Boundary (IB) nodes using the law of the wall, as in Eq. 3 (Rman et al. 2009). Continuum Figure 6. Fluid-particle coupling scheme We simulate transport by separating sediments and water, the former represented as a collection of elastic spheres, and the latter as a turbulent flow. The turbulence momentum and pressure exchange between sediment bed and free flow is resolved, giving a mean to study the triggering of the transport process starting from basic physical principles. Equation 3. The law of the wall in wall units, as used for the coupling The hydrodynamic force on particles are computed using the shear velocity coming from the law of the wall. The best approximation is obtained if all IB points are in the linear regime. The motion of particles causes the covering and uncovering of fluid nodes. Fluid velocity u / uτ [/] Figure 7. Nodes update due to particle movement Deeper pores (laminar) First pore The coupled algorithm is presented in the following scheme: (turbulent) Next Time Step Height h [m] Preliminary Results Figure 3. Particles and fluid representation, following the experimental setup of Pokrajac and Manes (2009). The contour shows a turbulent boundary layer. Figure 4. Penetration of turbulence within the bed. Velocity in the first layer of pores is lower than in deeper pores Discrete Element Method The scheme is tested reproducing a 3 D channel flow. The bottom wall is created by joining multiple particles, which are not allowed to move. On top of those, a colletion of freelyadvectable particles represents the eroded sediment. This simple system is able to reproduce the empirical Shields law, capturing quantitatively the threshold for sediment motion. Solid transport rate qs [/] The dynamics of the sediments is treated considering every single particle as a rigid body, whose movement is governed by Hamiltonian Mechanics. Both translational and rotational degrees of freedom are tracked. Figure 5. Force scheme used for Discrete Elements. References τcrit Shields number τ* [/] Sun, R. , & Xiao, H. (2015). Sedi. Foam: A general-purpose, open-source CFD-DEM boundary method for curvilinear grids. Computers & Fluids, 38(8), 1510– 1527. solver for particle-laden flow with emphasis on sediment transport. Computers and Geosciences, 1– 38. Campbell, L. , Mc. Ewan, I. , Nikora, V. , Pokrajac, D. , Gallagher, M. , & Manes, C. (2005). Bed-Load Effects on Hydrodynamics of Rough-Bed Open-Channel Flows. Vowinckel, B. , Kempe, T. , & Frohlich, J. (2014). Fluid-particle interaction in Journal of Hydraulic Engineering, (July), 576– 585. turbulent open channel flow with fully-resolved mobile beds. Advances in Water Resources, 72, 32– 44. Armenio, . , & Sarkar, S. (2002). An investigation of stably stratified turbulent channel flow using large-eddy simulation. Journal of Fluid Mechanics, 459(2002), Pokrajac, D. , & Manes, C. (2009). Velocity measurements of a free-surface 1– 42. turbulent flow penetrating a porous medium composed of uniform-size spheres. Transport in Porous Media, 78(3 SPEC. ISS. ), 367– 383. Further development will focus on increasing the system size in order to allow for the emergence of bedforms. Roman, F. , Armenio, V. , & Fröhlich, J. (2009). A simple wall-layer model for large eddy simulation with immersed boundary method. Physics of Fluids, 21(10). Roman, F. , Napoli, E. , Milici, B. , & Armenio, V. (2009). An improved immersed The research leading to these results has received funding from the European Union (FP 7/20072013) under grant agreement n° 607394 SEDITRANS Figure 8. Particles and fluid representation. The contour shows the turbulent velocity field, scaled by the wall shear velocity. Figure 9. Transport rate as function of different Shields number, obtained changing the channel inclination. • Acknowledgments The research leading to these results has received funding from the People Programme (Marie. Curie Actions) of the European Union's Seventh Framework Programme FP 7/2007 -2013/ under REA grant agreement No 607394 -SEDITRANS.