Electronic Structure Theory Contents Jack Simons Henry Eyring
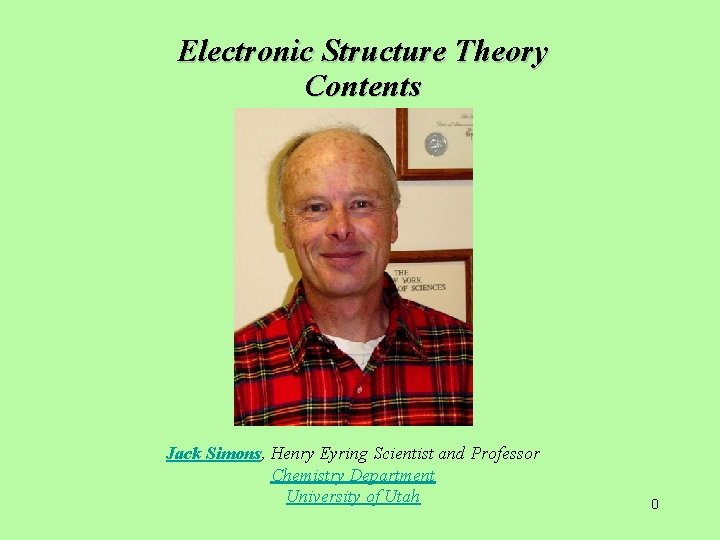
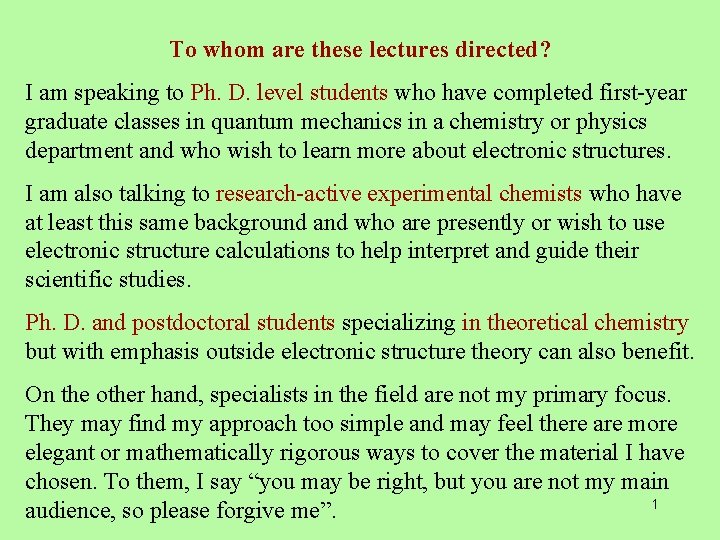
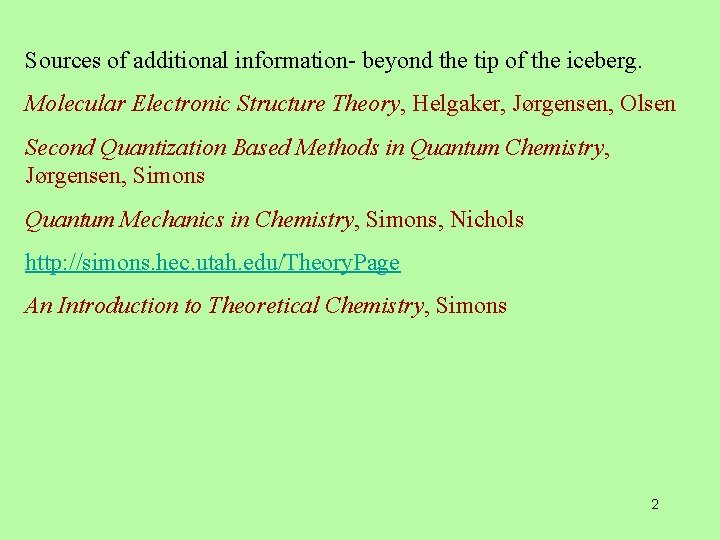
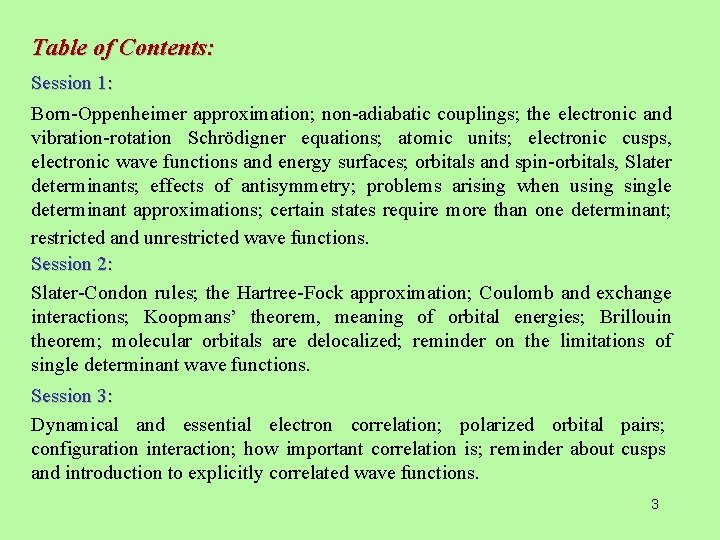
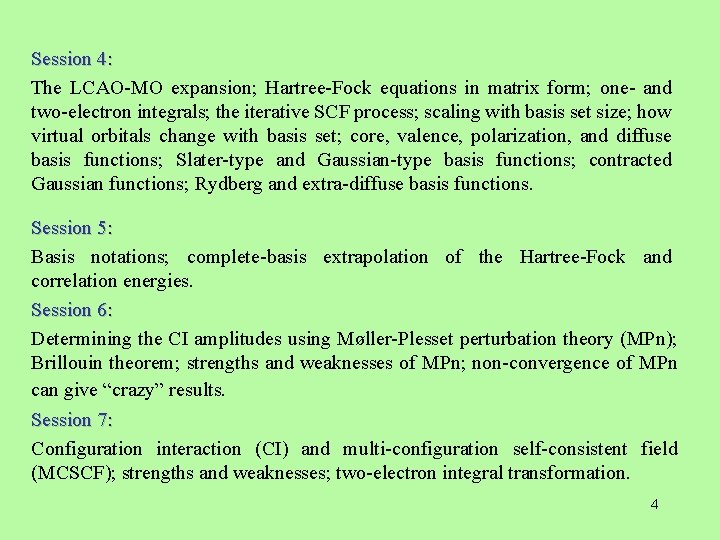
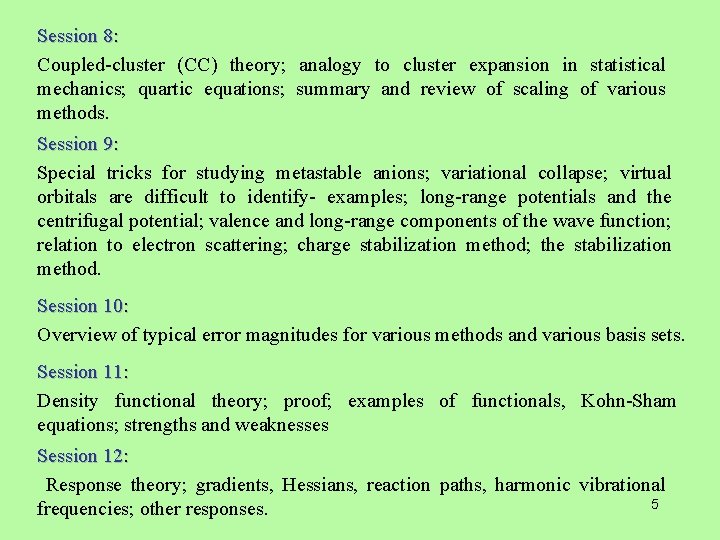
- Slides: 6
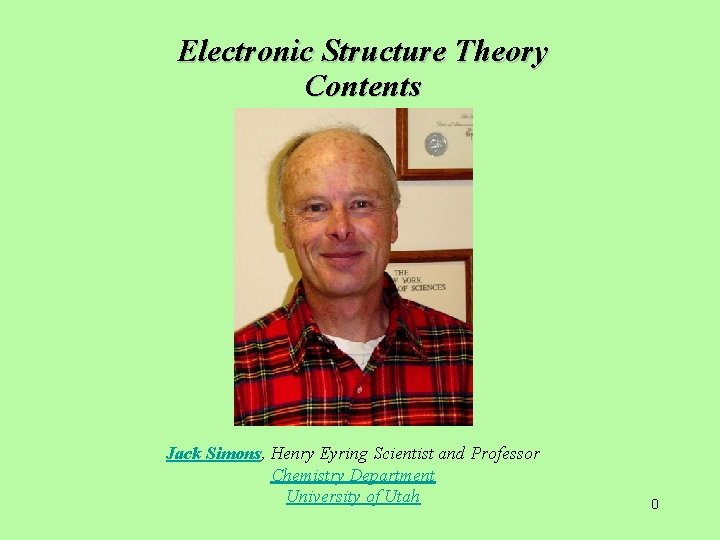
Electronic Structure Theory Contents Jack Simons, Henry Eyring Scientist and Professor Chemistry Department University of Utah 0
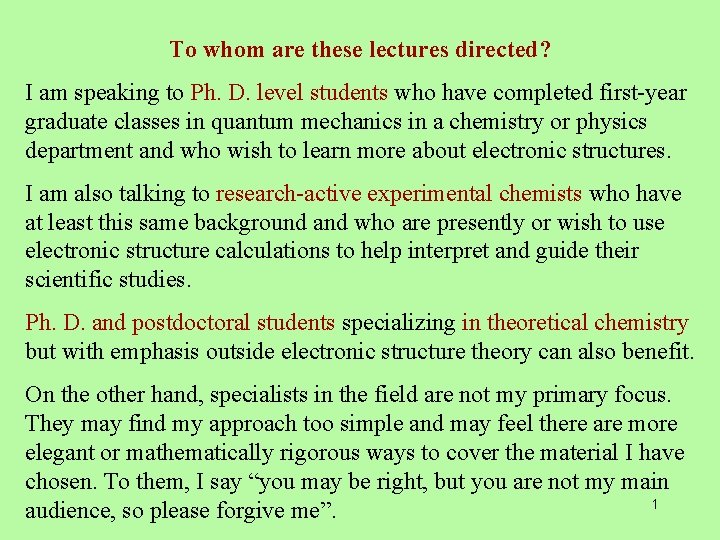
To whom are these lectures directed? I am speaking to Ph. D. level students who have completed first-year graduate classes in quantum mechanics in a chemistry or physics department and who wish to learn more about electronic structures. I am also talking to research-active experimental chemists who have at least this same background and who are presently or wish to use electronic structure calculations to help interpret and guide their scientific studies. Ph. D. and postdoctoral students specializing in theoretical chemistry but with emphasis outside electronic structure theory can also benefit. On the other hand, specialists in the field are not my primary focus. They may find my approach too simple and may feel there are more elegant or mathematically rigorous ways to cover the material I have chosen. To them, I say “you may be right, but you are not my main 1 audience, so please forgive me”.
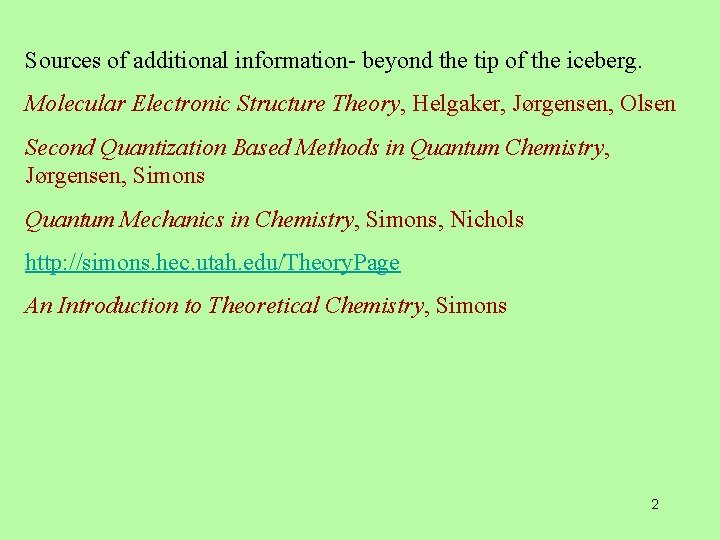
Sources of additional information- beyond the tip of the iceberg. Molecular Electronic Structure Theory, Helgaker, Jørgensen, Olsen Second Quantization Based Methods in Quantum Chemistry, Jørgensen, Simons Quantum Mechanics in Chemistry, Simons, Nichols http: //simons. hec. utah. edu/Theory. Page An Introduction to Theoretical Chemistry, Simons 2
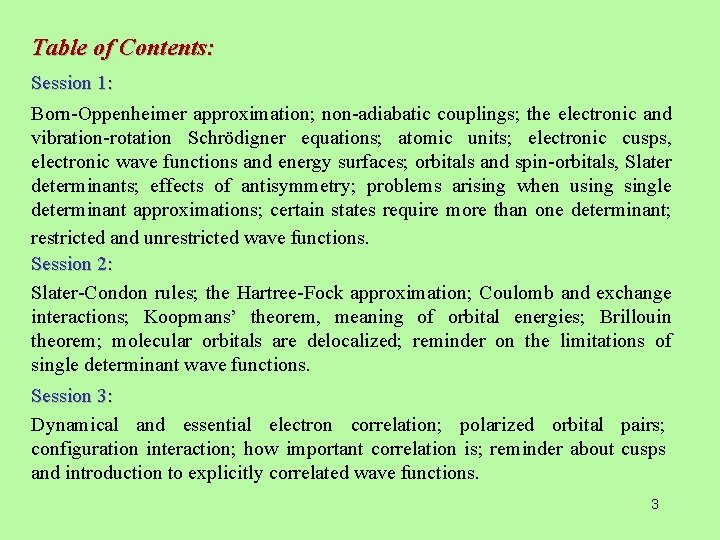
Table of Contents: Session 1: Born-Oppenheimer approximation; non-adiabatic couplings; the electronic and vibration-rotation Schrödigner equations; atomic units; electronic cusps, electronic wave functions and energy surfaces; orbitals and spin-orbitals, Slater determinants; effects of antisymmetry; problems arising when usingle determinant approximations; certain states require more than one determinant; restricted and unrestricted wave functions. Session 2: Slater-Condon rules; the Hartree-Fock approximation; Coulomb and exchange interactions; Koopmans’ theorem, meaning of orbital energies; Brillouin theorem; molecular orbitals are delocalized; reminder on the limitations of single determinant wave functions. Session 3: Dynamical and essential electron correlation; polarized orbital pairs; configuration interaction; how important correlation is; reminder about cusps and introduction to explicitly correlated wave functions. 3
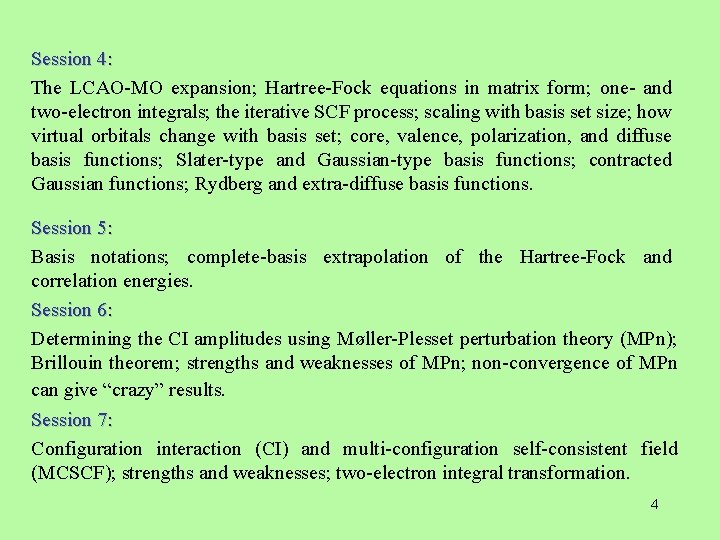
Session 4: The LCAO-MO expansion; Hartree-Fock equations in matrix form; one- and two-electron integrals; the iterative SCF process; scaling with basis set size; how virtual orbitals change with basis set; core, valence, polarization, and diffuse basis functions; Slater-type and Gaussian-type basis functions; contracted Gaussian functions; Rydberg and extra-diffuse basis functions. Session 5: Basis notations; complete-basis extrapolation of the Hartree-Fock and correlation energies. Session 6: Determining the CI amplitudes using Møller-Plesset perturbation theory (MPn); Brillouin theorem; strengths and weaknesses of MPn; non-convergence of MPn can give “crazy” results. Session 7: Configuration interaction (CI) and multi-configuration self-consistent field (MCSCF); strengths and weaknesses; two-electron integral transformation. 4
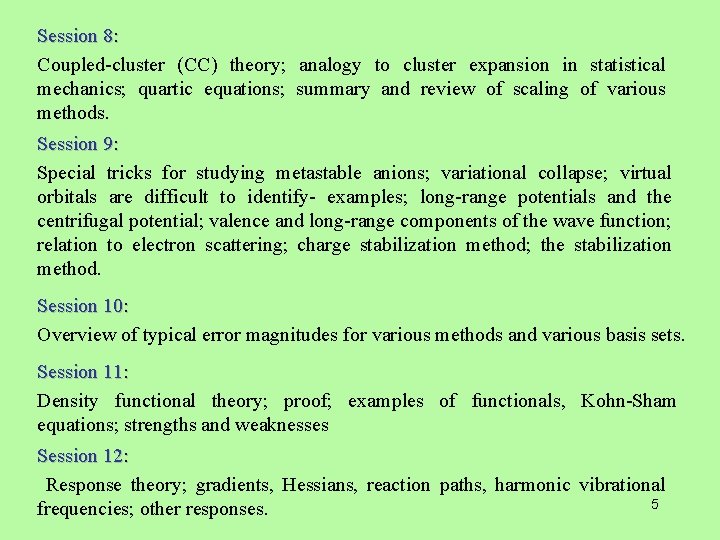
Session 8: Coupled-cluster (CC) theory; analogy to cluster expansion in statistical mechanics; quartic equations; summary and review of scaling of various methods. Session 9: Special tricks for studying metastable anions; variational collapse; virtual orbitals are difficult to identify- examples; long-range potentials and the centrifugal potential; valence and long-range components of the wave function; relation to electron scattering; charge stabilization method; the stabilization method. Session 10: Overview of typical error magnitudes for various methods and various basis sets. Session 11: Density functional theory; proof; examples of functionals, Kohn-Sham equations; strengths and weaknesses Session 12: Response theory; gradients, Hessians, reaction paths, harmonic vibrational 5 frequencies; other responses.