A PyroElectric Crystal Particle Accelerator Chelsea L Harris
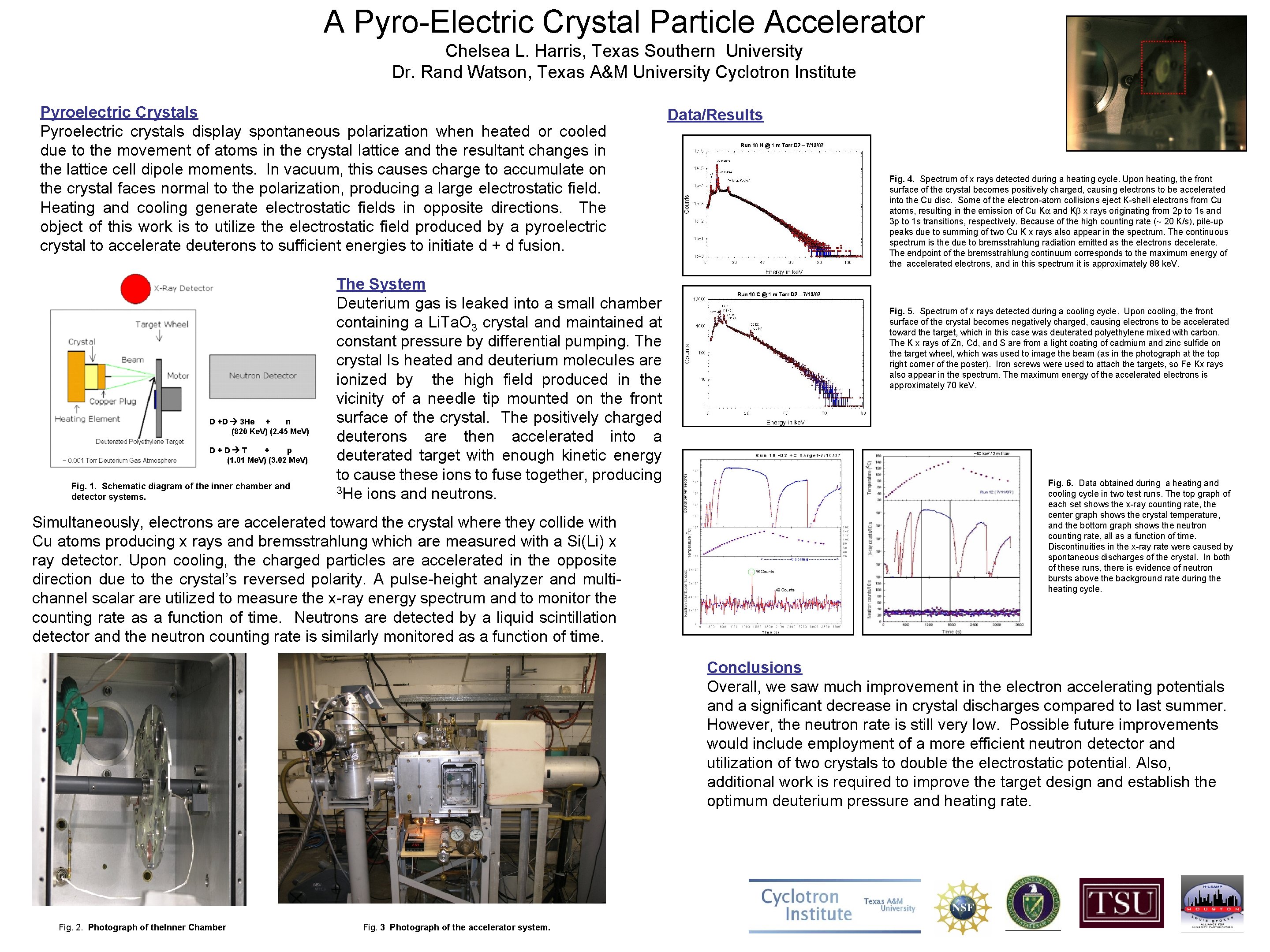
- Slides: 1
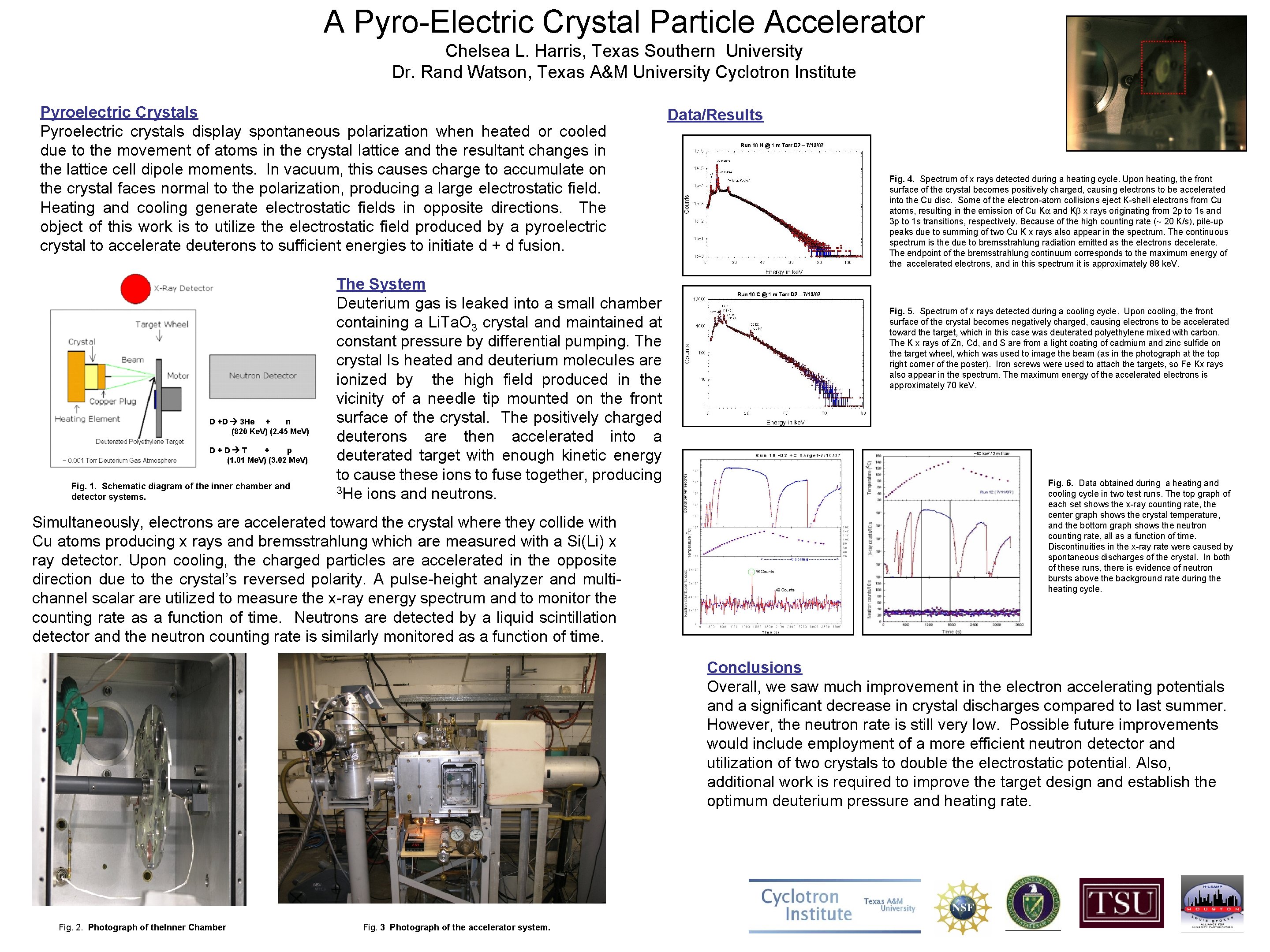
A Pyro-Electric Crystal Particle Accelerator Chelsea L. Harris, Texas Southern University Dr. Rand Watson, Texas A&M University Cyclotron Institute Pyroelectric Crystals Pyroelectric crystals display spontaneous polarization when heated or cooled due to the movement of atoms in the crystal lattice and the resultant changes in the lattice cell dipole moments. In vacuum, this causes charge to accumulate on the crystal faces normal to the polarization, producing a large electrostatic field. Heating and cooling generate electrostatic fields in opposite directions. The object of this work is to utilize the electrostatic field produced by a pyroelectric crystal to accelerate deuterons to sufficient energies to initiate d + d fusion. D +D 3 He + n (820 Ke. V) (2. 45 Me. V) D+D T + p (1. 01 Me. V) (3. 02 Me. V) Fig. 1. Schematic diagram of the inner chamber and detector systems. The System Deuterium gas is leaked into a small chamber containing a Li. Ta. O 3 crystal and maintained at constant pressure by differential pumping. The crystal Is heated and deuterium molecules are ionized by the high field produced in the vicinity of a needle tip mounted on the front surface of the crystal. The positively charged deuterons are then accelerated into a deuterated target with enough kinetic energy to cause these ions to fuse together, producing 3 He ions and neutrons. Simultaneously, electrons are accelerated toward the crystal where they collide with Cu atoms producing x rays and bremsstrahlung which are measured with a Si(Li) x ray detector. Upon cooling, the charged particles are accelerated in the opposite direction due to the crystal’s reversed polarity. A pulse-height analyzer and multichannel scalar are utilized to measure the x-ray energy spectrum and to monitor the counting rate as a function of time. Neutrons are detected by a liquid scintillation detector and the neutron counting rate is similarly monitored as a function of time. Data/Results Fig. 4. Spectrum of x rays detected during a heating cycle. Upon heating, the front surface of the crystal becomes positively charged, causing electrons to be accelerated into the Cu disc. Some of the electron-atom collisions eject K-shell electrons from Cu atoms, resulting in the emission of Cu K and K x rays originating from 2 p to 1 s and 3 p to 1 s transitions, respectively. Because of the high counting rate ( 20 K/s), pile-up peaks due to summing of two Cu K x rays also appear in the spectrum. The continuous spectrum is the due to bremsstrahlung radiation emitted as the electrons decelerate. The endpoint of the bremsstrahlung continuum corresponds to the maximum energy of the accelerated electrons, and in this spectrum it is approximately 88 ke. V. Fig. 5. Spectrum of x rays detected during a cooling cycle. Upon cooling, the front surface of the crystal becomes negatively charged, causing electrons to be accelerated toward the target, which in this case was deuterated polyethylene mixed with carbon. The K x rays of Zn, Cd, and S are from a light coating of cadmium and zinc sulfide on the target wheel, which was used to image the beam (as in the photograph at the top right corner of the poster). Iron screws were used to attach the targets, so Fe Kx rays also appear in the spectrum. The maximum energy of the accelerated electrons is approximately 70 ke. V. Fig. 6. Data obtained during a heating and cooling cycle in two test runs. The top graph of each set shows the x-ray counting rate, the center graph shows the crystal temperature, and the bottom graph shows the neutron counting rate, all as a function of time. Discontinuities in the x-ray rate were caused by spontaneous discharges of the crystal. In both of these runs, there is evidence of neutron bursts above the background rate during the heating cycle. Conclusions Overall, we saw much improvement in the electron accelerating potentials and a significant decrease in crystal discharges compared to last summer. However, the neutron rate is still very low. Possible future improvements would include employment of a more efficient neutron detector and utilization of two crystals to double the electrostatic potential. Also, additional work is required to improve the target design and establish the optimum deuterium pressure and heating rate. Fig. 2. Photograph of the. Inner Chamber Fig. 3 Photograph of the accelerator system.