WELCOME to our science fair project On ASTRONOMY
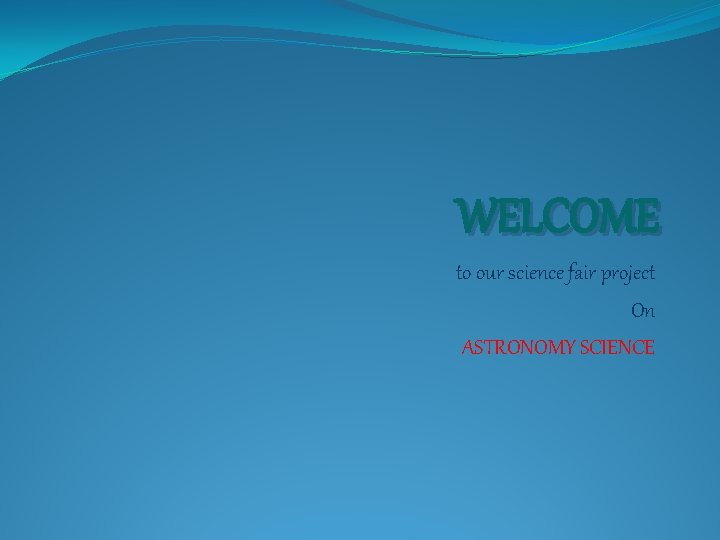
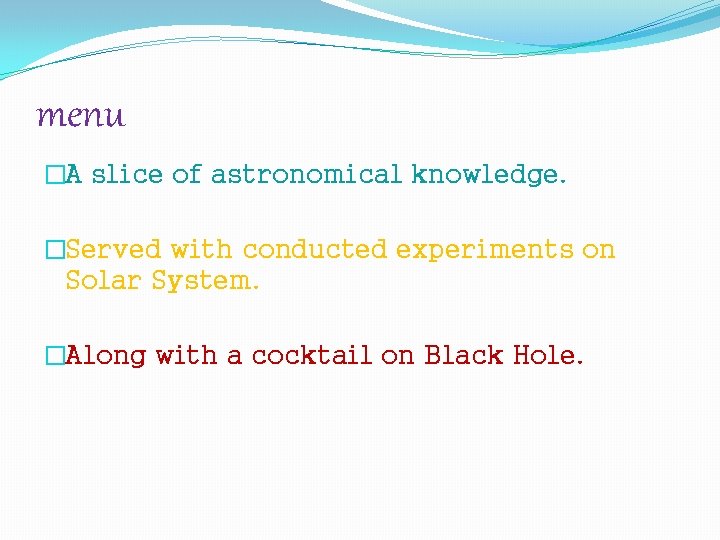
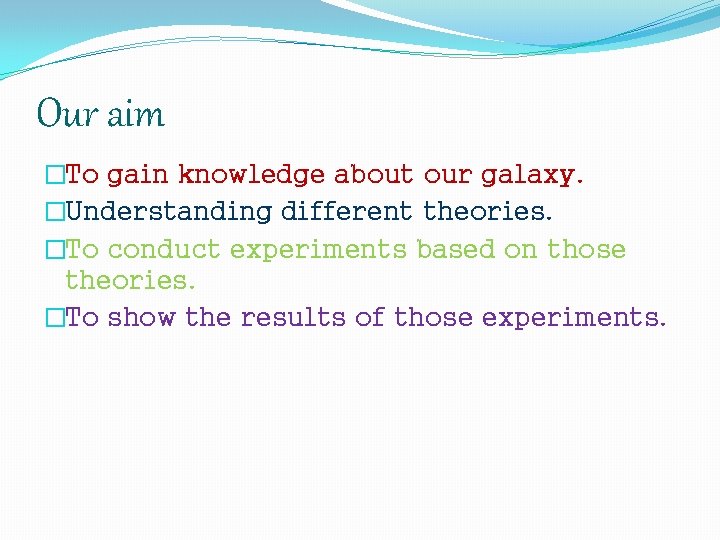
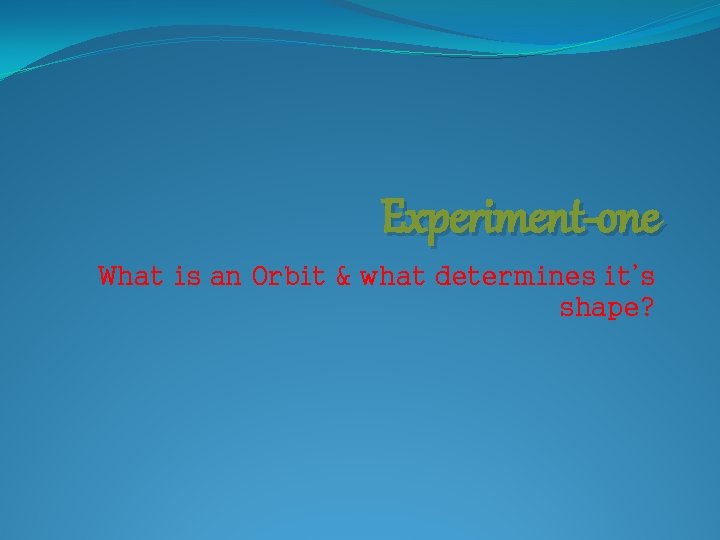
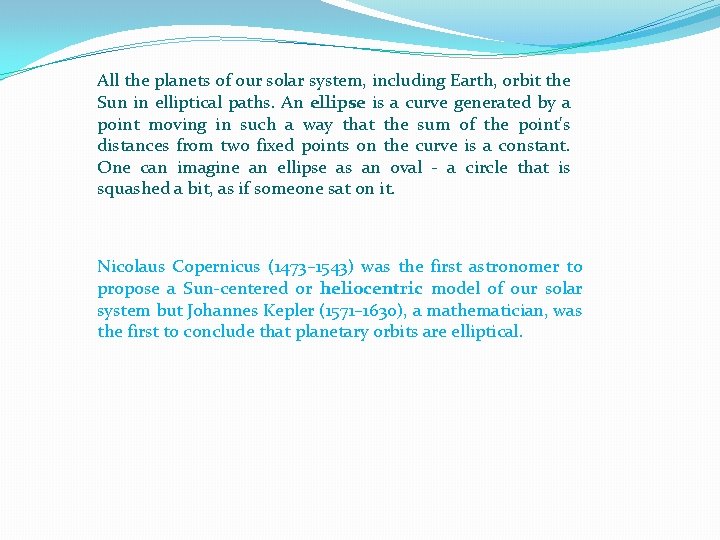
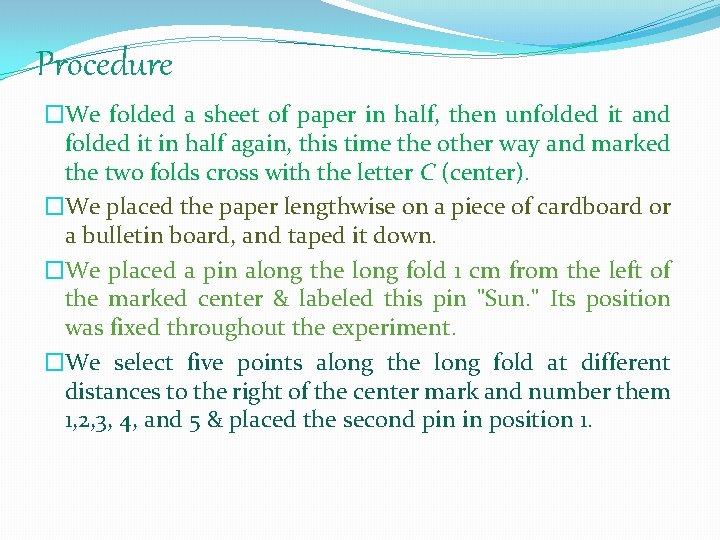
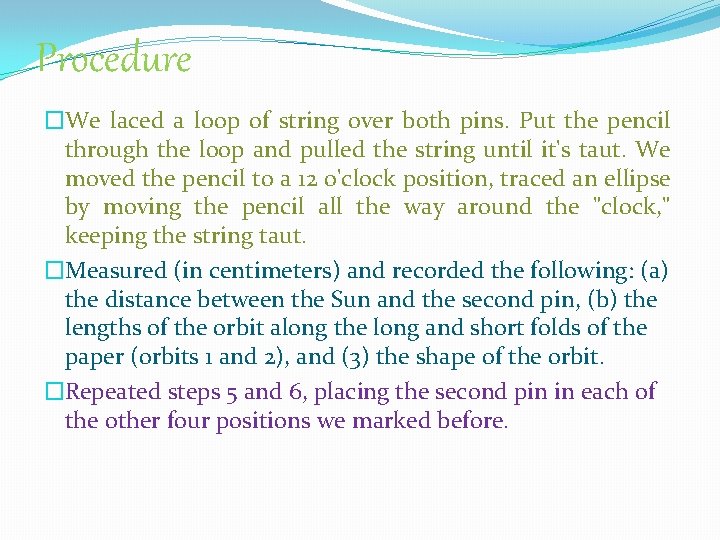
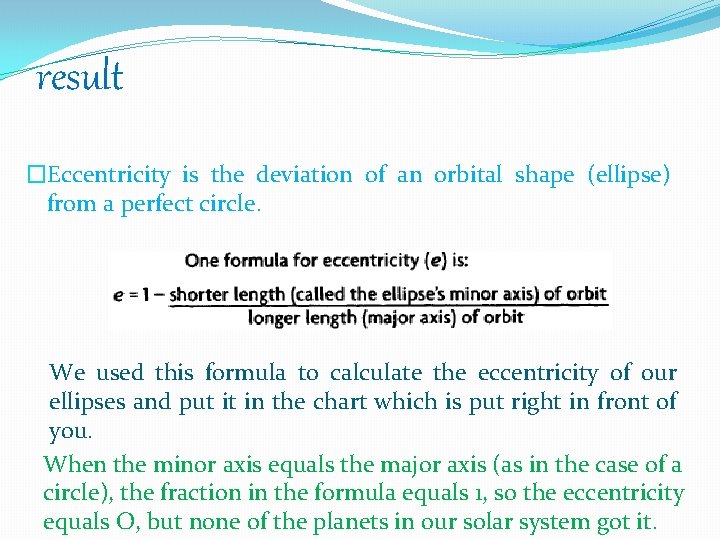
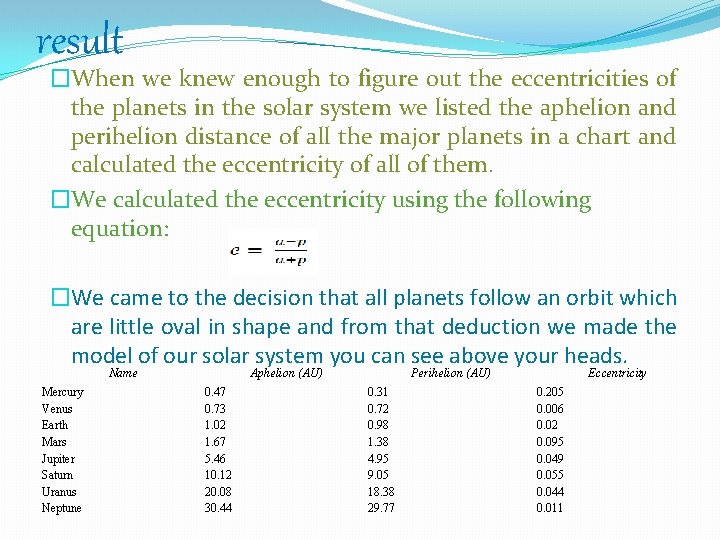
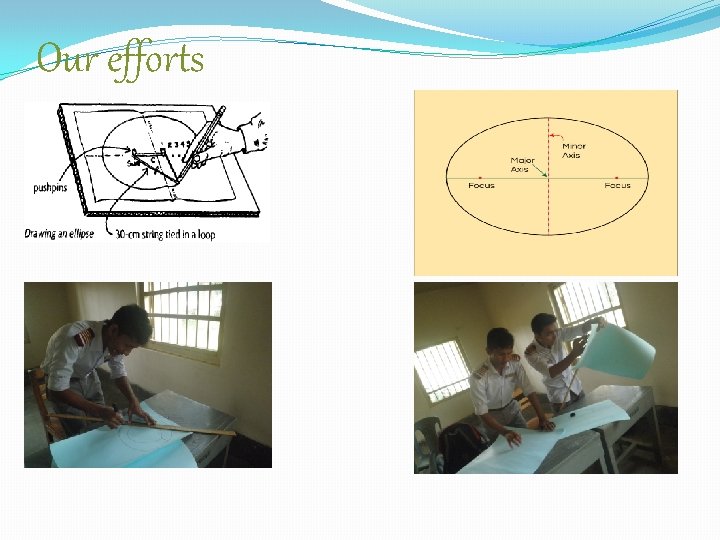
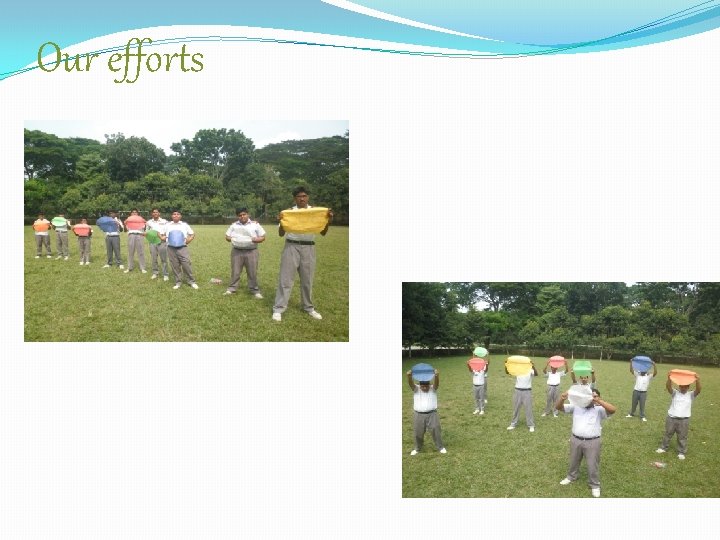
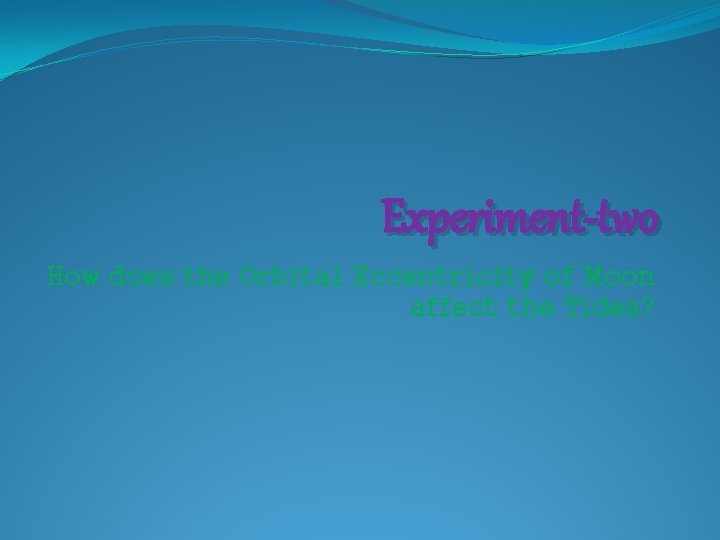
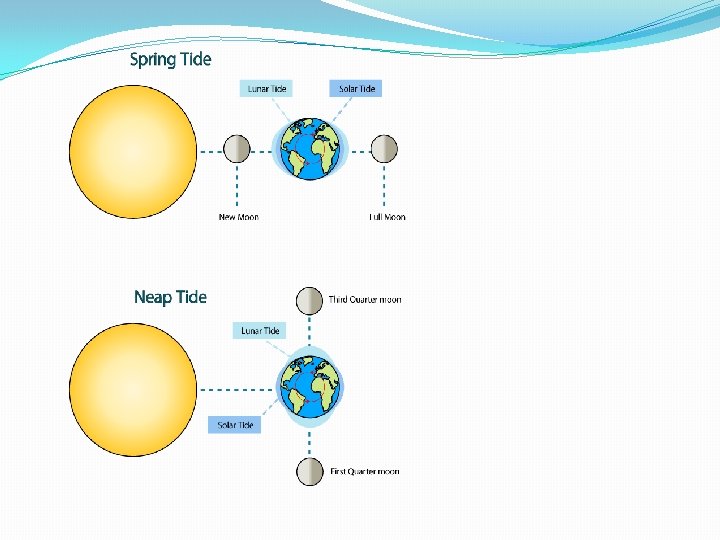
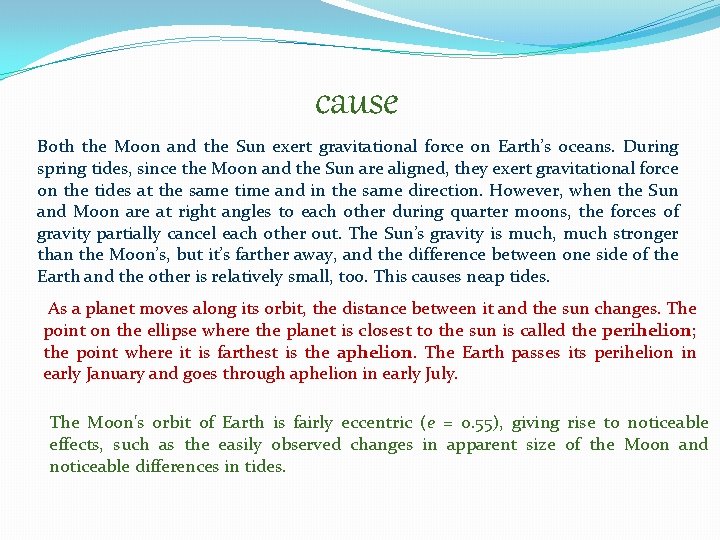
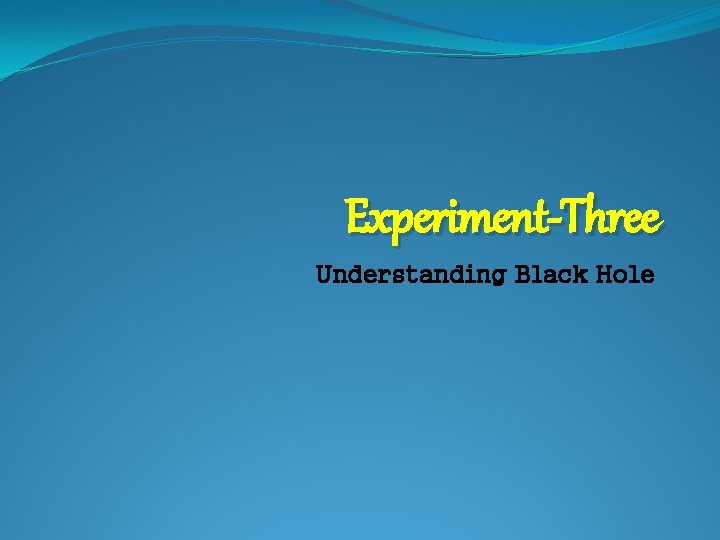
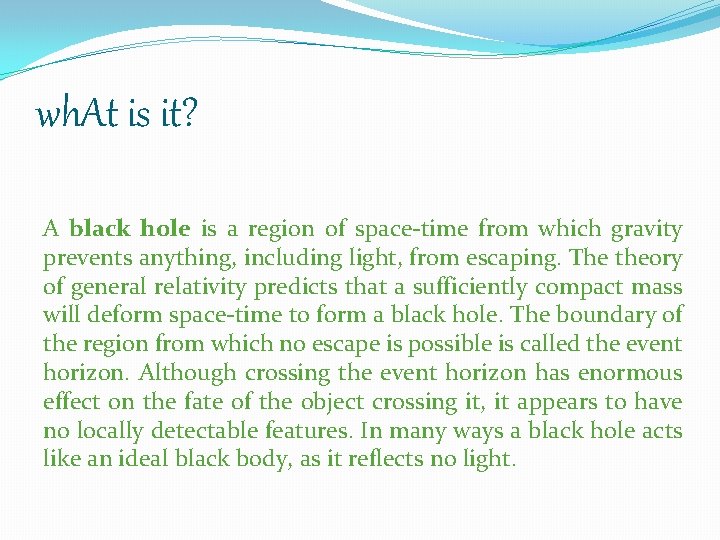
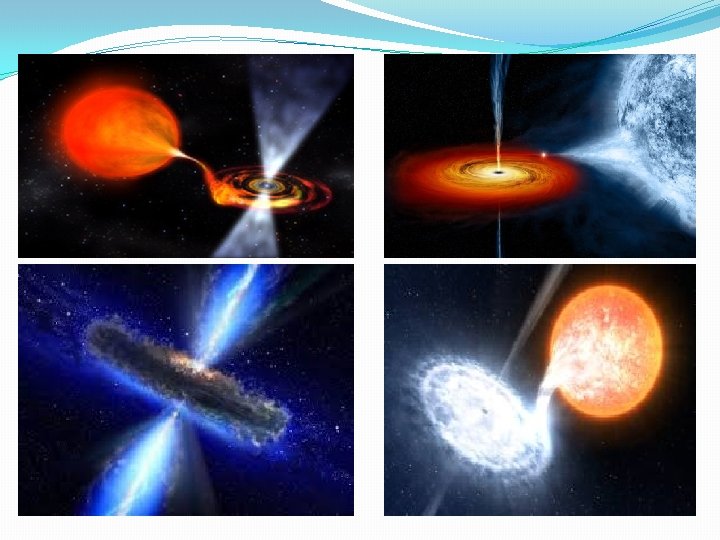
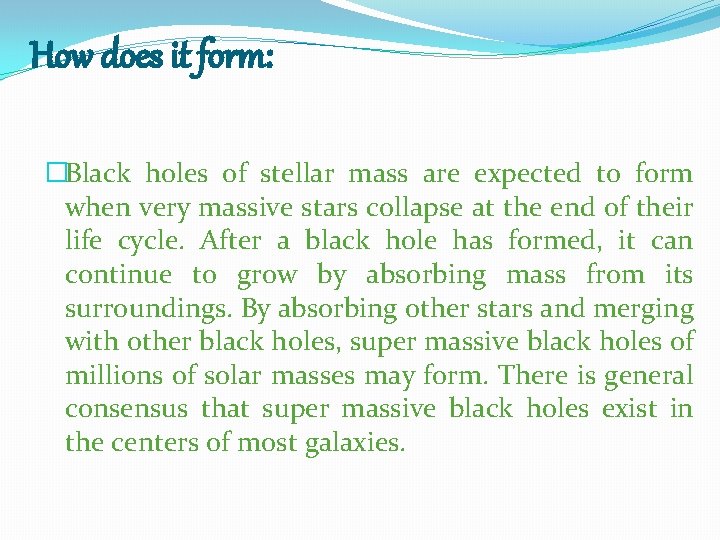
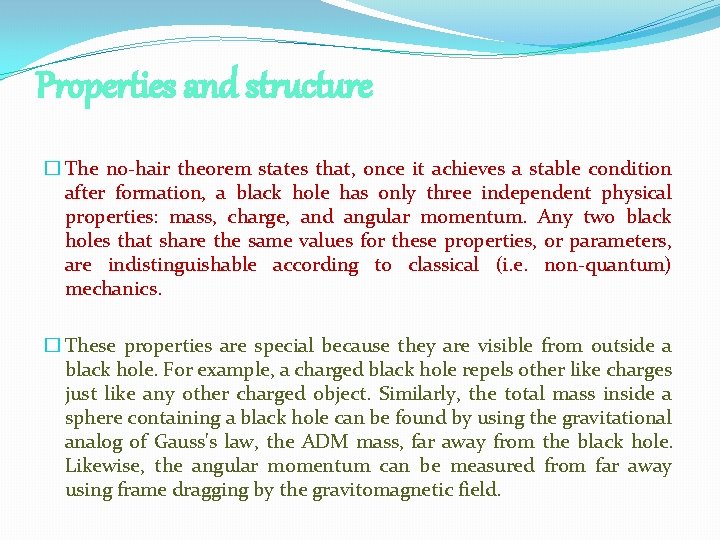
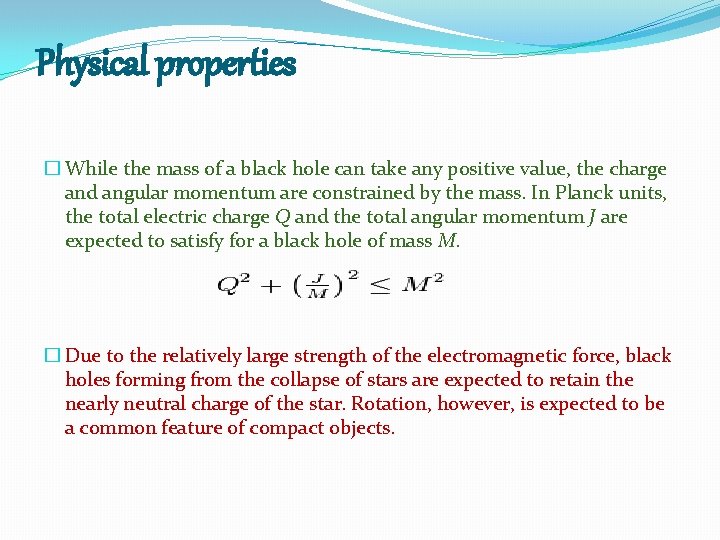
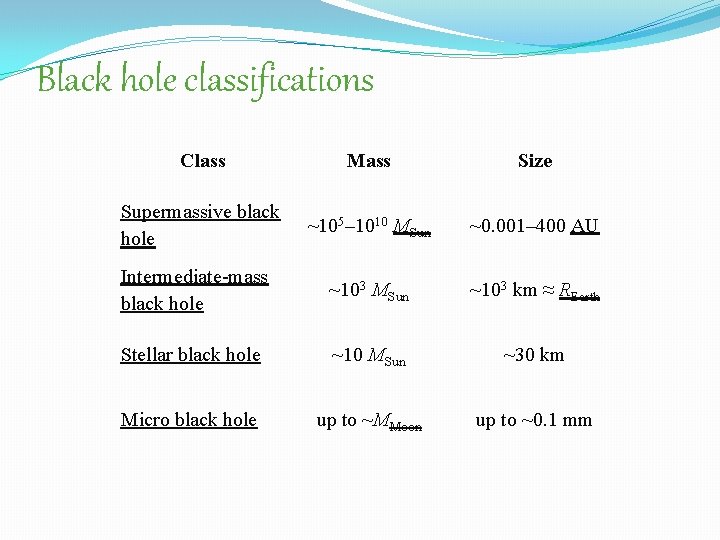
- Slides: 21
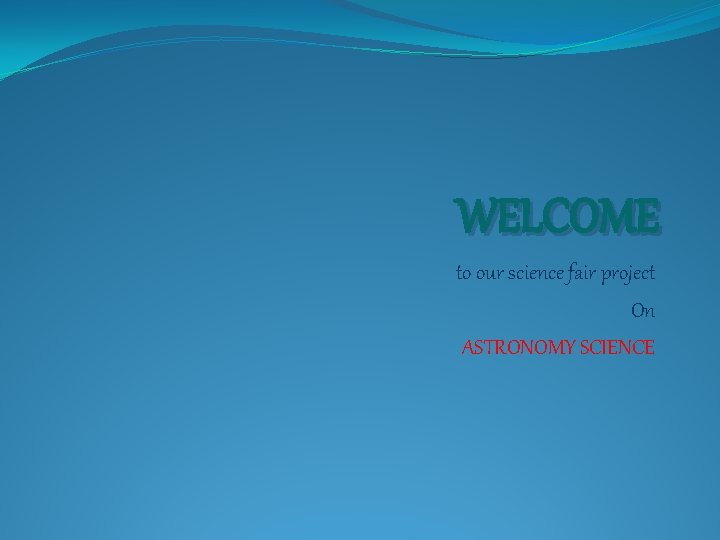
WELCOME to our science fair project On ASTRONOMY SCIENCE
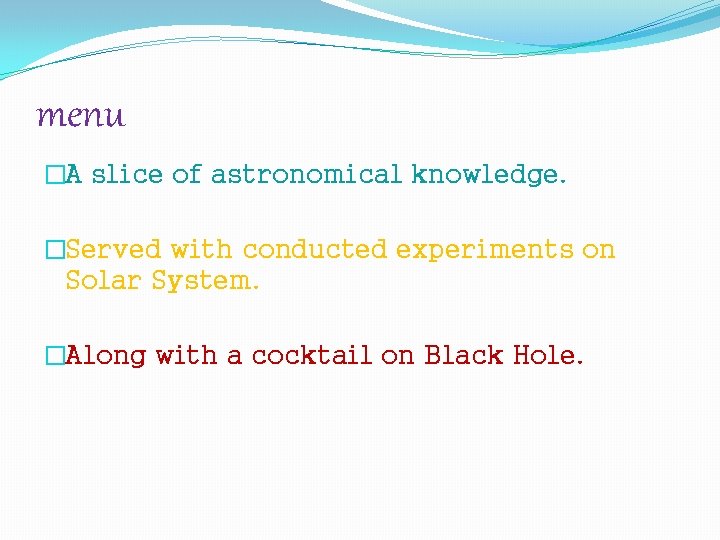
menu �A slice of astronomical knowledge. �Served with conducted experiments on Solar System. �Along with a cocktail on Black Hole.
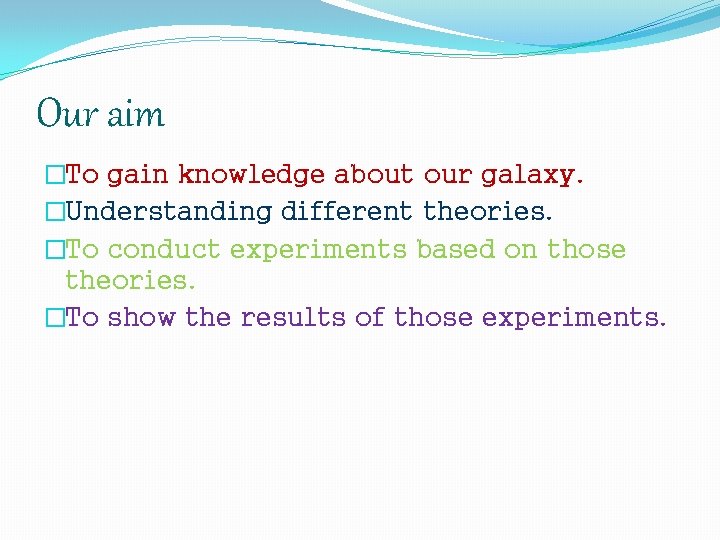
Our aim �To gain knowledge about our galaxy. �Understanding different theories. �To conduct experiments based on those theories. �To show the results of those experiments.
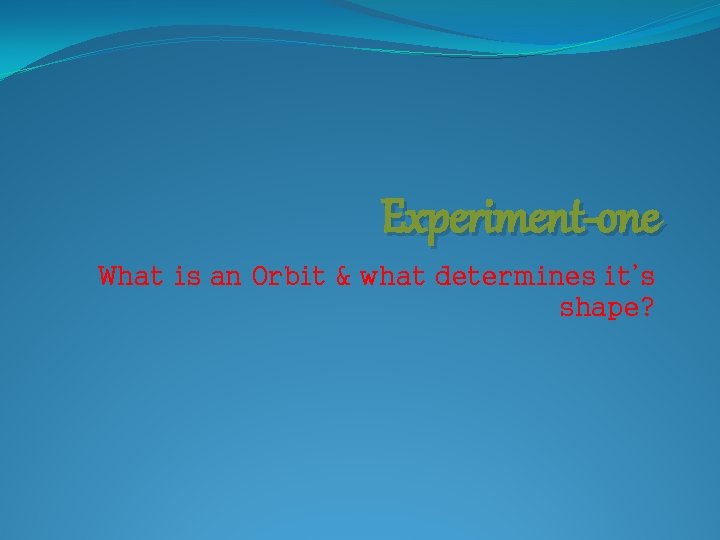
Experiment-one What is an Orbit & what determines it’s shape?
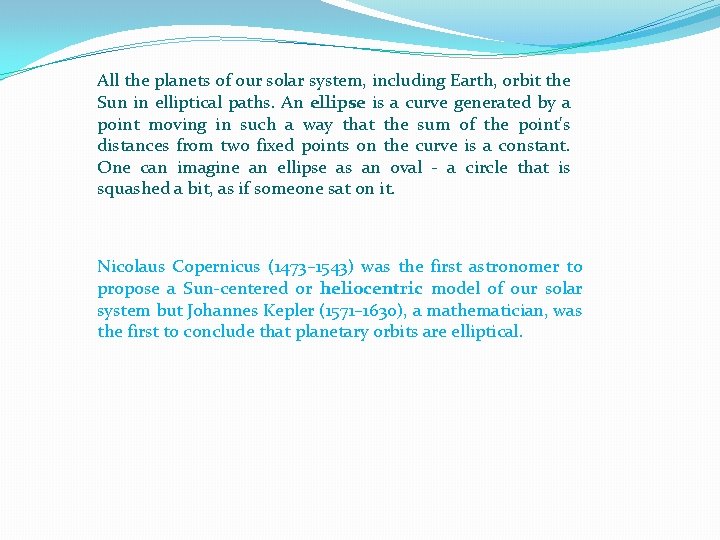
All the planets of our solar system, including Earth, orbit the Sun in elliptical paths. An ellipse is a curve generated by a point moving in such a way that the sum of the point's distances from two fixed points on the curve is a constant. One can imagine an ellipse as an oval - a circle that is squashed a bit, as if someone sat on it. Nicolaus Copernicus (1473– 1543) was the first astronomer to propose a Sun-centered or heliocentric model of our solar system but Johannes Kepler (1571– 1630), a mathematician, was the first to conclude that planetary orbits are elliptical.
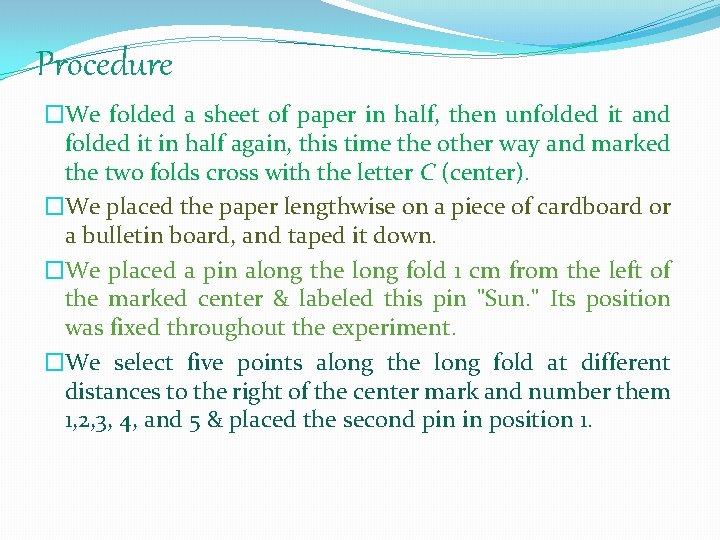
Procedure �We folded a sheet of paper in half, then unfolded it and folded it in half again, this time the other way and marked the two folds cross with the letter C (center). �We placed the paper lengthwise on a piece of cardboard or a bulletin board, and taped it down. �We placed a pin along the long fold 1 cm from the left of the marked center & labeled this pin "Sun. " Its position was fixed throughout the experiment. �We select five points along the long fold at different distances to the right of the center mark and number them 1, 2, 3, 4, and 5 & placed the second pin in position 1.
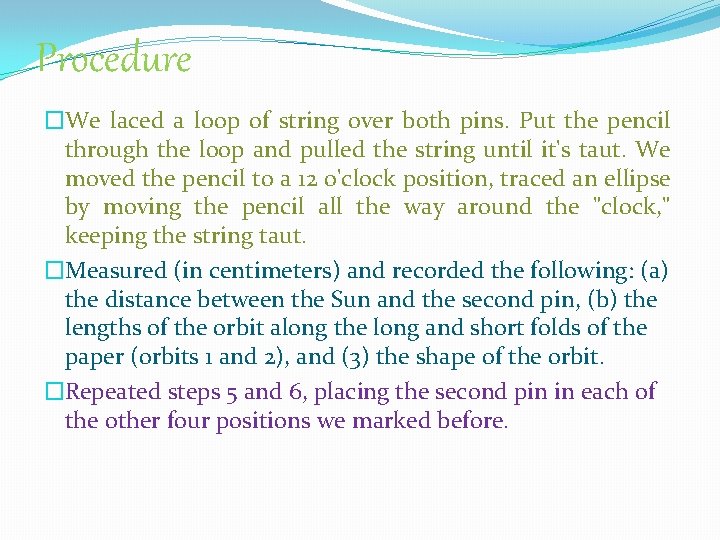
Procedure �We laced a loop of string over both pins. Put the pencil through the loop and pulled the string until it's taut. We moved the pencil to a 12 o'clock position, traced an ellipse by moving the pencil all the way around the "clock, " keeping the string taut. �Measured (in centimeters) and recorded the following: (a) the distance between the Sun and the second pin, (b) the lengths of the orbit along the long and short folds of the paper (orbits 1 and 2), and (3) the shape of the orbit. �Repeated steps 5 and 6, placing the second pin in each of the other four positions we marked before.
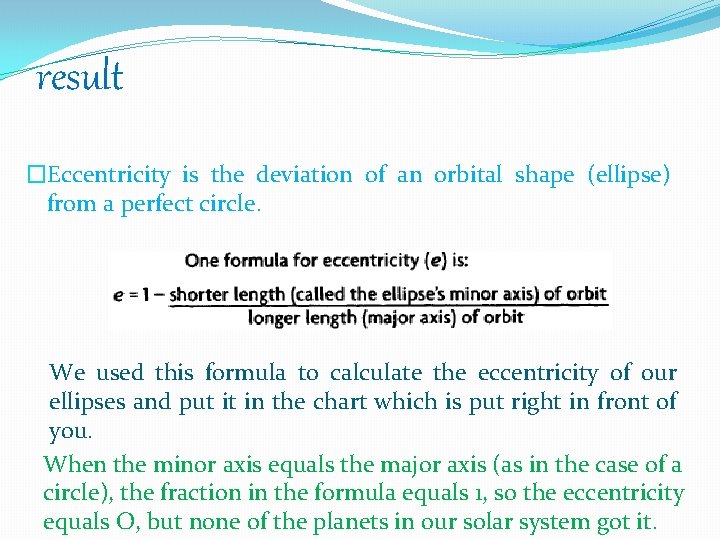
result �Eccentricity is the deviation of an orbital shape (ellipse) from a perfect circle. We used this formula to calculate the eccentricity of our ellipses and put it in the chart which is put right in front of you. When the minor axis equals the major axis (as in the case of a circle), the fraction in the formula equals 1, so the eccentricity equals O, but none of the planets in our solar system got it.
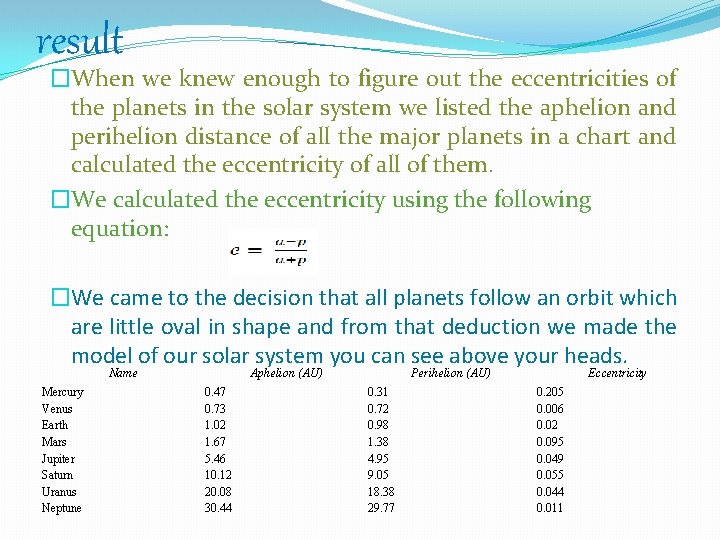
result �When we knew enough to figure out the eccentricities of the planets in the solar system we listed the aphelion and perihelion distance of all the major planets in a chart and calculated the eccentricity of all of them. �We calculated the eccentricity using the following equation: �We came to the decision that all planets follow an orbit which are little oval in shape and from that deduction we made the model of our solar system you can see above your heads. Name Mercury Venus Earth Mars Jupiter Saturn Uranus Neptune Aphelion (AU) 0. 47 0. 73 1. 02 1. 67 5. 46 10. 12 20. 08 30. 44 Perihelion (AU) 0. 31 0. 72 0. 98 1. 38 4. 95 9. 05 18. 38 29. 77 Eccentricity 0. 205 0. 006 0. 02 0. 095 0. 049 0. 055 0. 044 0. 011
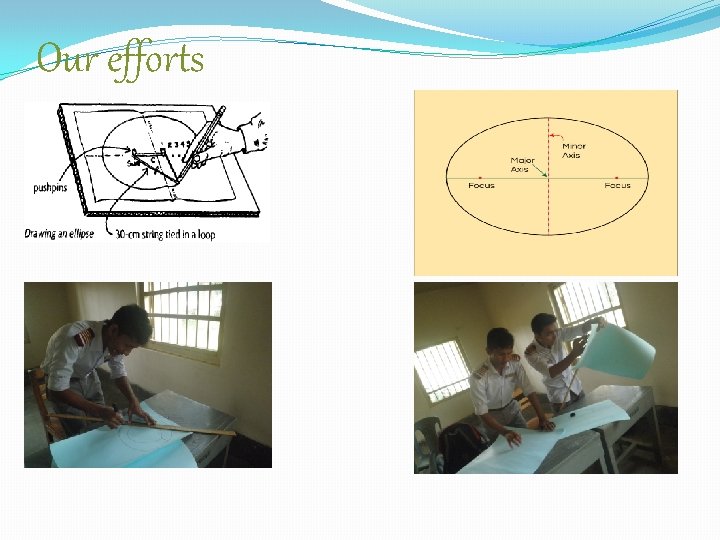
Our efforts
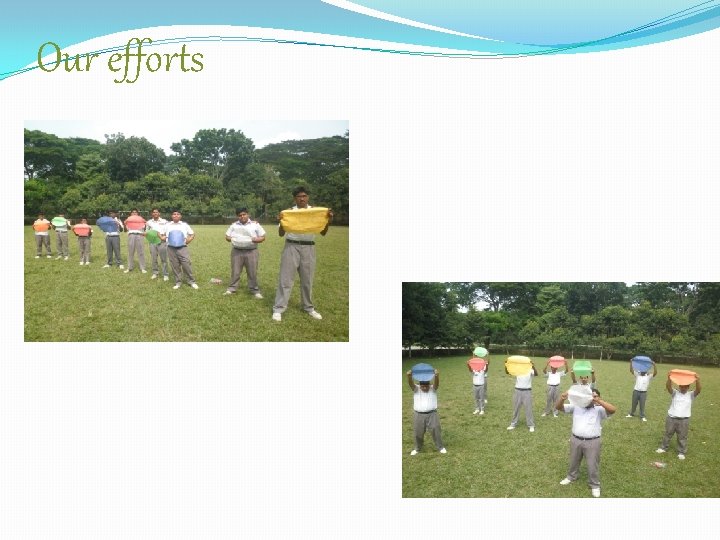
Our efforts
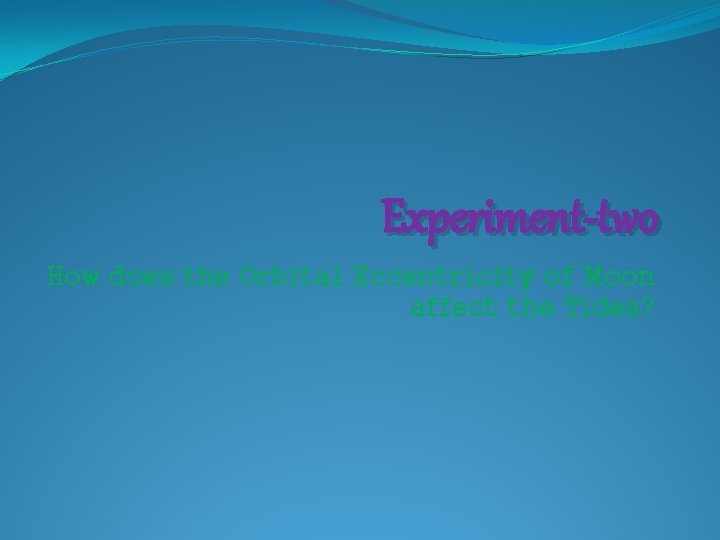
Experiment-two How does the Orbital Eccentricity of Moon affect the Tides?
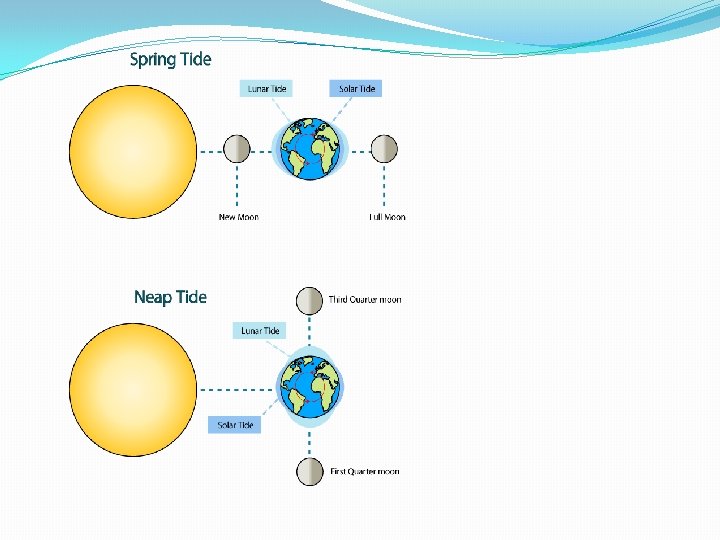
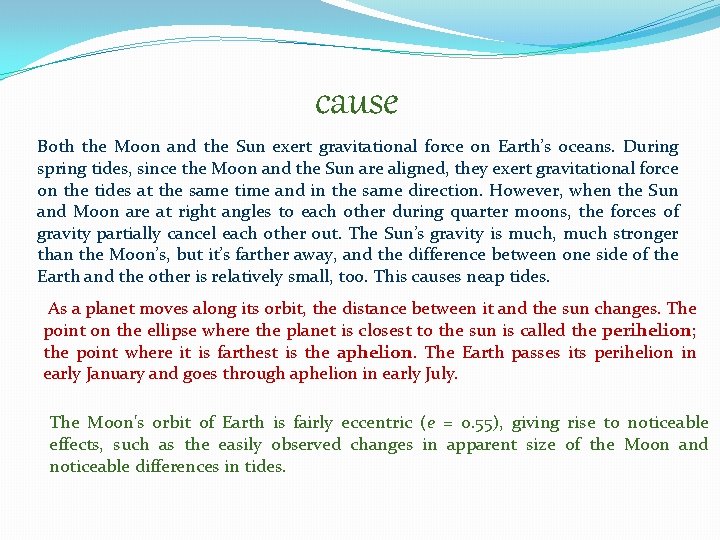
cause Both the Moon and the Sun exert gravitational force on Earth’s oceans. During spring tides, since the Moon and the Sun are aligned, they exert gravitational force on the tides at the same time and in the same direction. However, when the Sun and Moon are at right angles to each other during quarter moons, the forces of gravity partially cancel each other out. The Sun’s gravity is much, much stronger than the Moon’s, but it’s farther away, and the difference between one side of the Earth and the other is relatively small, too. This causes neap tides. As a planet moves along its orbit, the distance between it and the sun changes. The point on the ellipse where the planet is closest to the sun is called the perihelion; the point where it is farthest is the aphelion. The Earth passes its perihelion in early January and goes through aphelion in early July. The Moon's orbit of Earth is fairly eccentric (e = 0. 55), giving rise to noticeable effects, such as the easily observed changes in apparent size of the Moon and noticeable differences in tides.
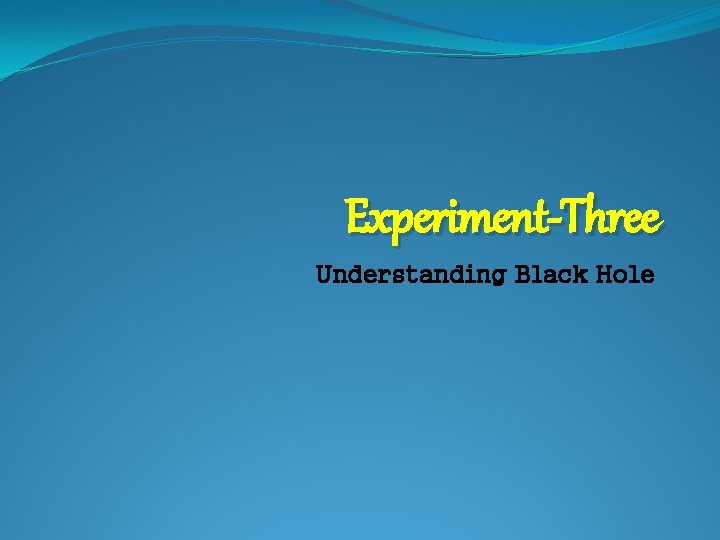
Experiment-Three Understanding Black Hole
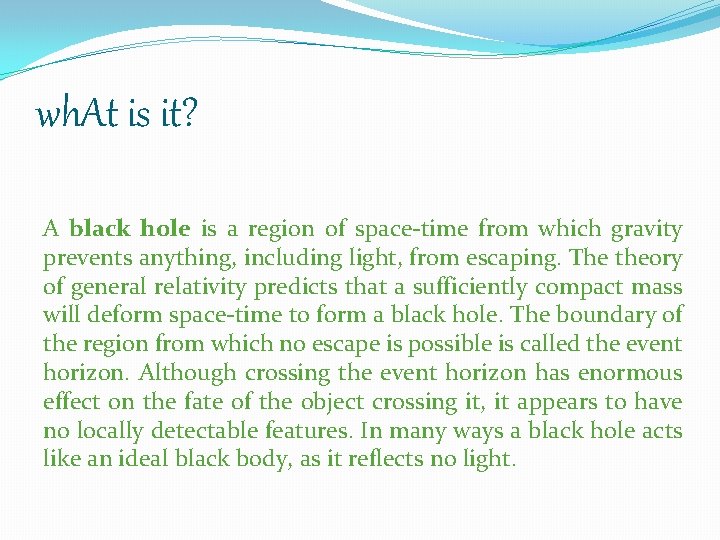
wh. At is it? A black hole is a region of space-time from which gravity prevents anything, including light, from escaping. The theory of general relativity predicts that a sufficiently compact mass will deform space-time to form a black hole. The boundary of the region from which no escape is possible is called the event horizon. Although crossing the event horizon has enormous effect on the fate of the object crossing it, it appears to have no locally detectable features. In many ways a black hole acts like an ideal black body, as it reflects no light.
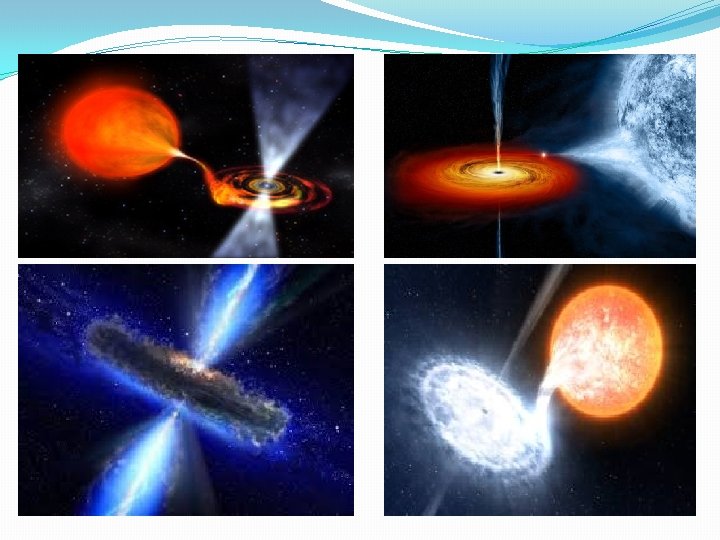
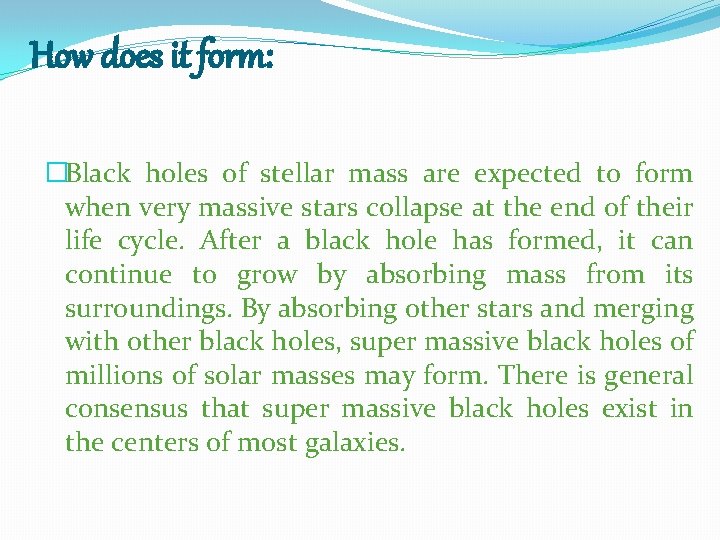
How does it form: �Black holes of stellar mass are expected to form when very massive stars collapse at the end of their life cycle. After a black hole has formed, it can continue to grow by absorbing mass from its surroundings. By absorbing other stars and merging with other black holes, super massive black holes of millions of solar masses may form. There is general consensus that super massive black holes exist in the centers of most galaxies.
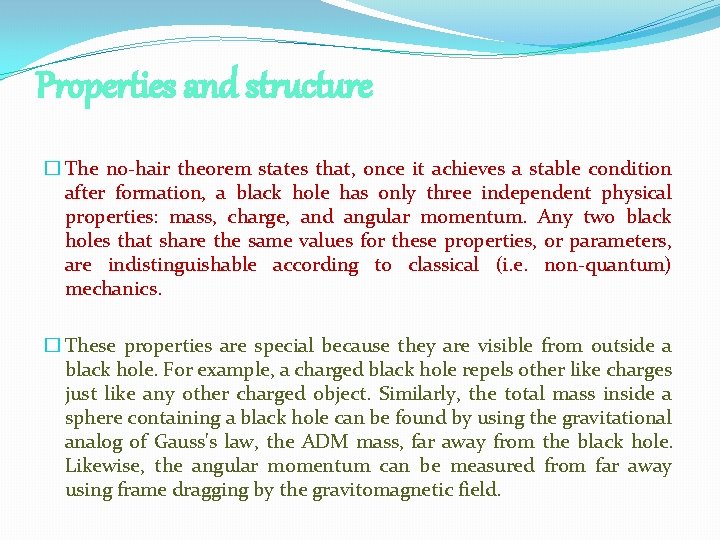
Properties and structure � The no-hair theorem states that, once it achieves a stable condition after formation, a black hole has only three independent physical properties: mass, charge, and angular momentum. Any two black holes that share the same values for these properties, or parameters, are indistinguishable according to classical (i. e. non-quantum) mechanics. � These properties are special because they are visible from outside a black hole. For example, a charged black hole repels other like charges just like any other charged object. Similarly, the total mass inside a sphere containing a black hole can be found by using the gravitational analog of Gauss's law, the ADM mass, far away from the black hole. Likewise, the angular momentum can be measured from far away using frame dragging by the gravitomagnetic field.
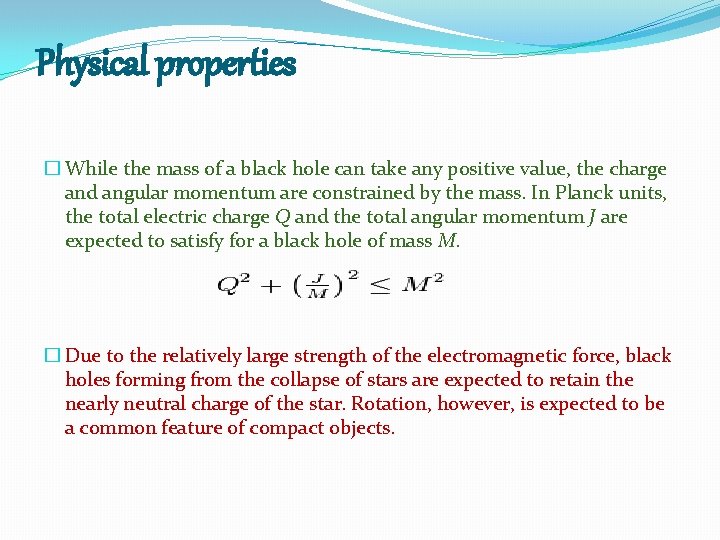
Physical properties � While the mass of a black hole can take any positive value, the charge and angular momentum are constrained by the mass. In Planck units, the total electric charge Q and the total angular momentum J are expected to satisfy for a black hole of mass M. � Due to the relatively large strength of the electromagnetic force, black holes forming from the collapse of stars are expected to retain the nearly neutral charge of the star. Rotation, however, is expected to be a common feature of compact objects.
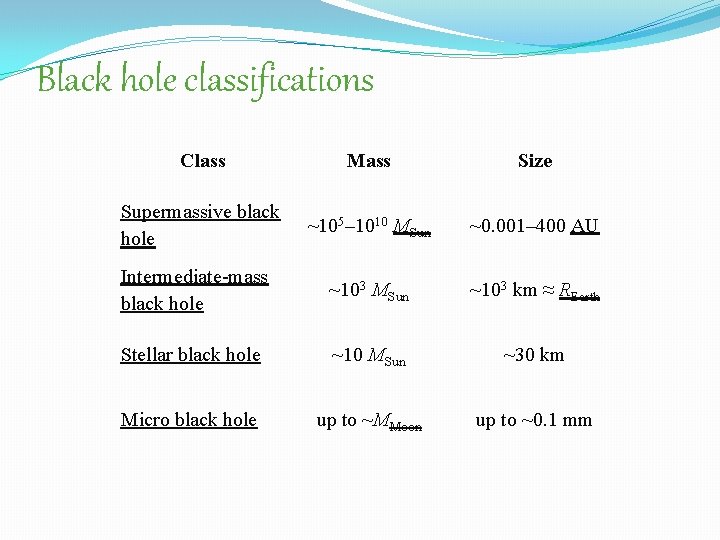
Black hole classifications Class Mass Size Supermassive black hole ~105– 1010 MSun ~0. 001– 400 AU Intermediate-mass black hole ~103 MSun ~103 km ≈ REarth Stellar black hole ~10 MSun ~30 km Micro black hole up to ~MMoon up to ~0. 1 mm