Voltammetry and Polarography Lecture 6 1 Cyclic Voltammetry
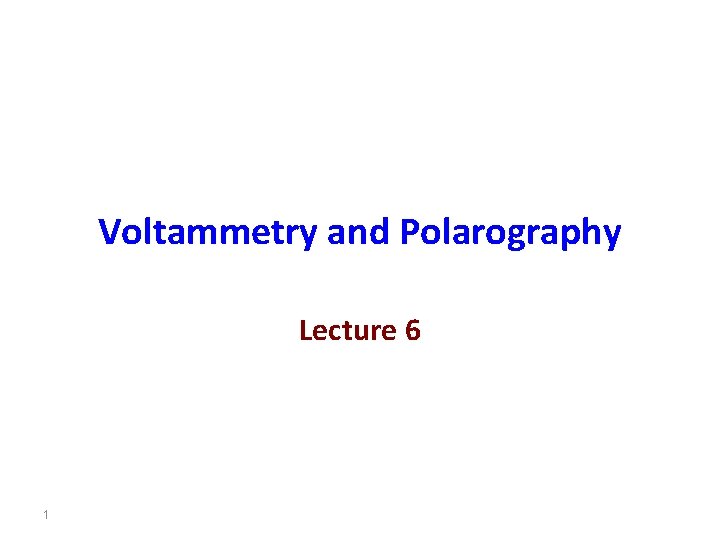
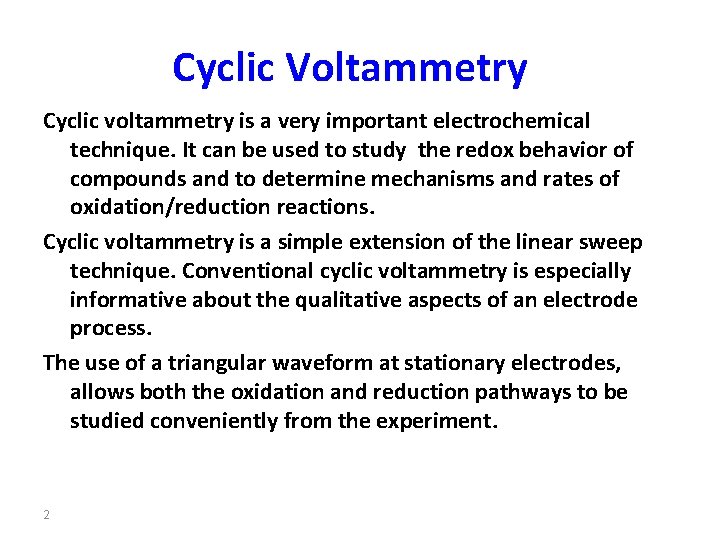
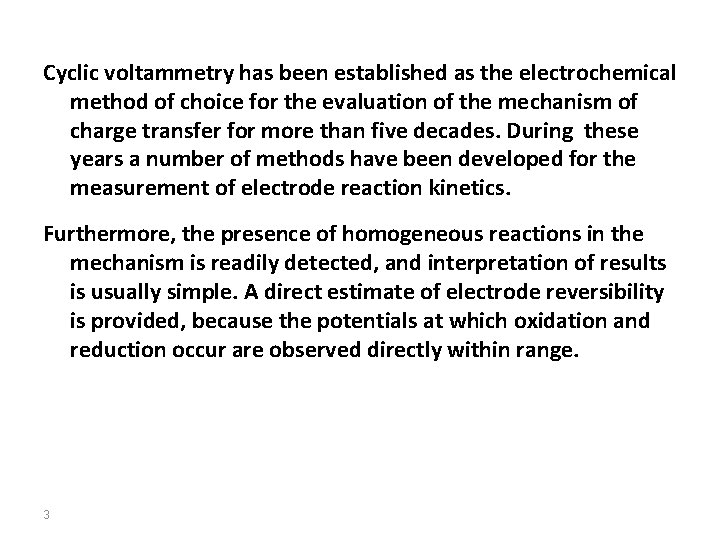
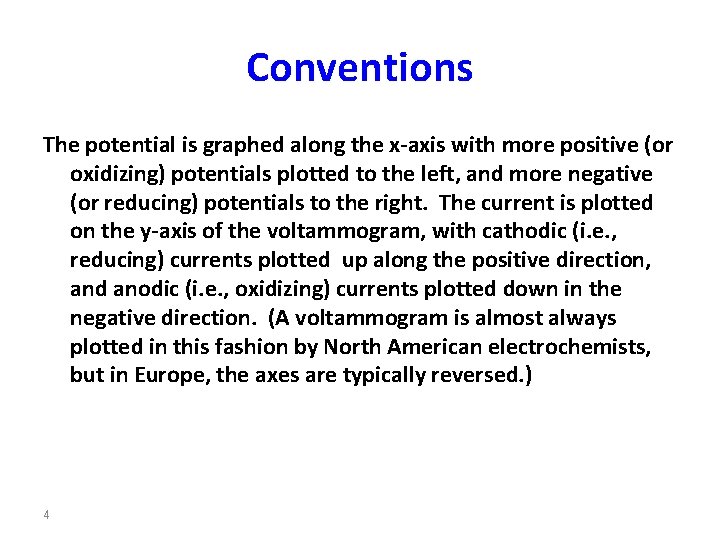
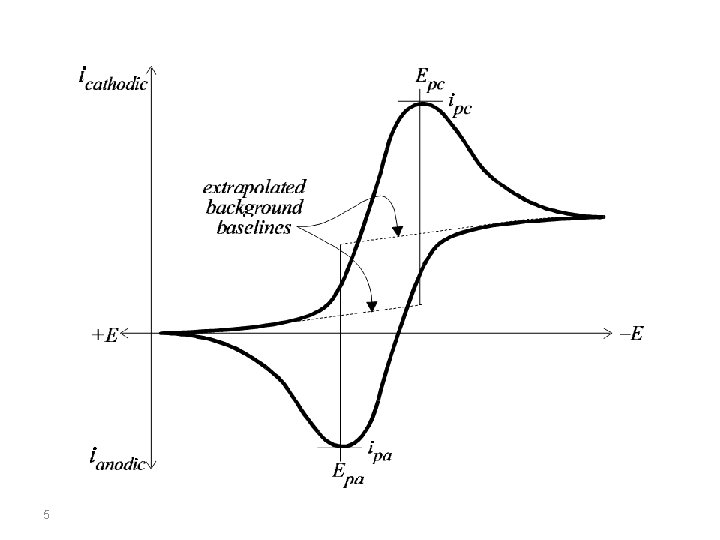
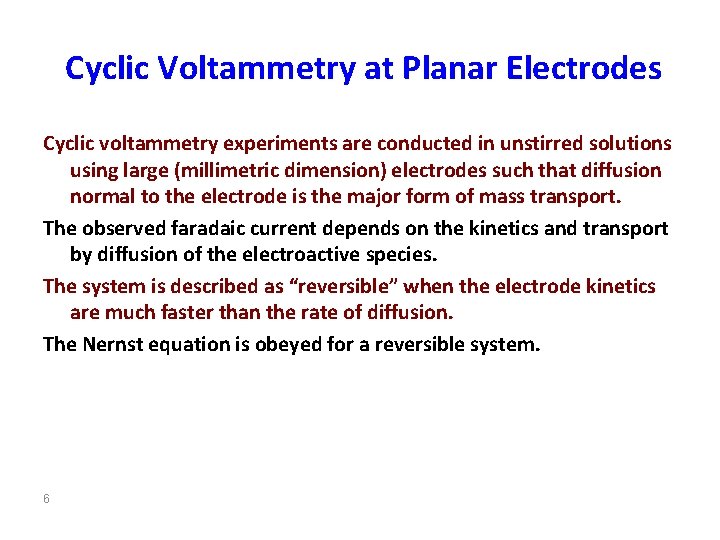
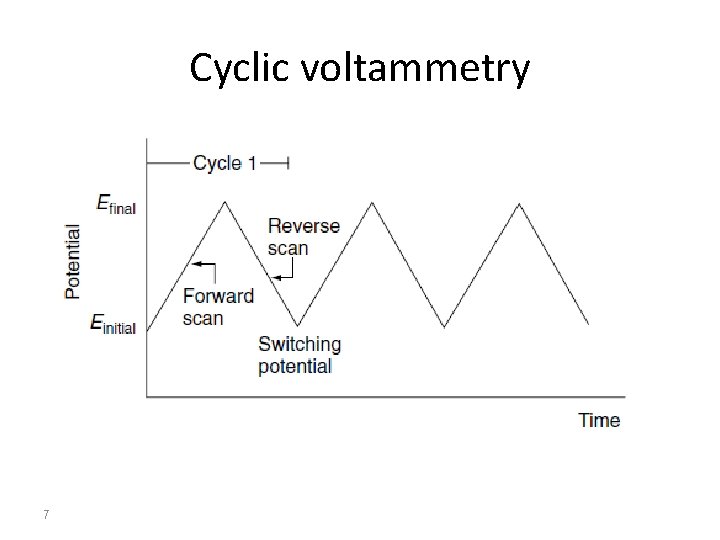
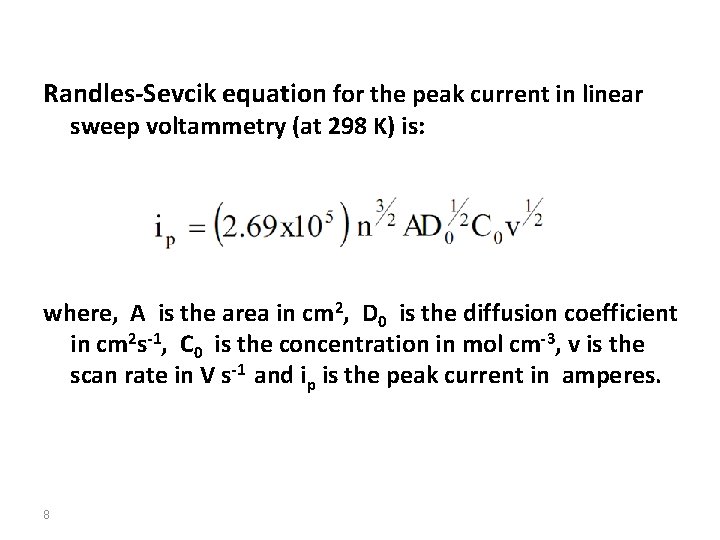
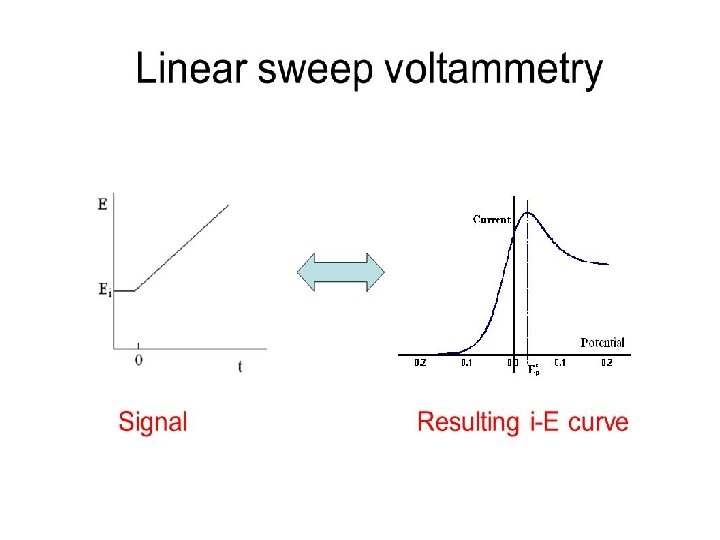
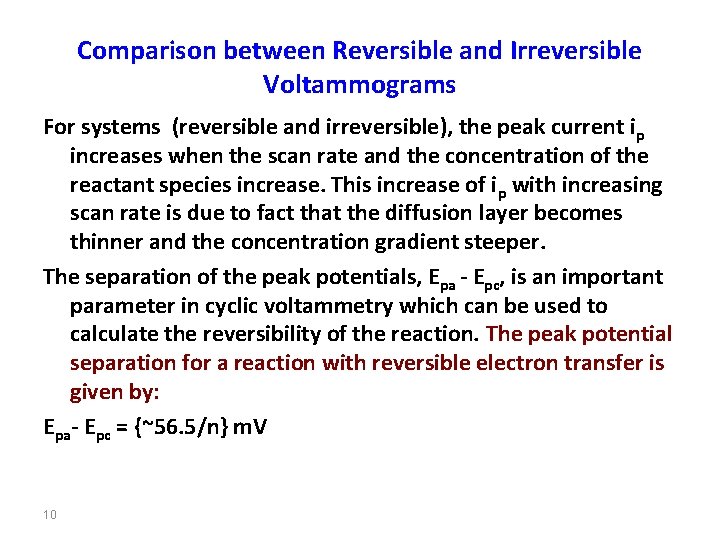
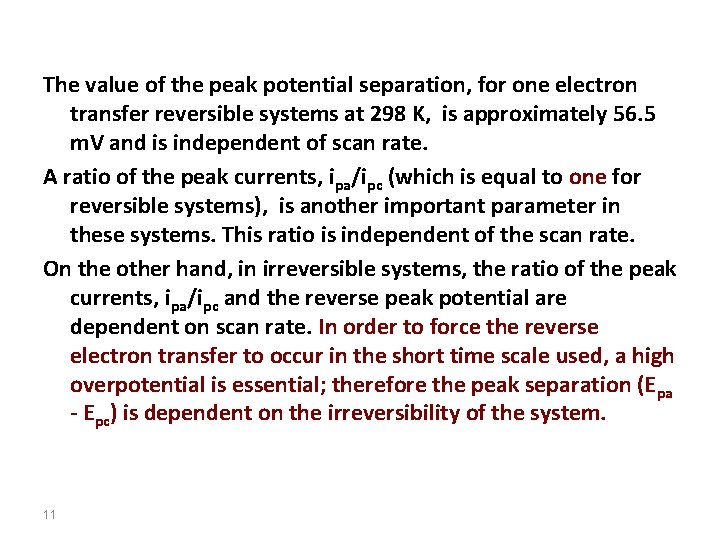
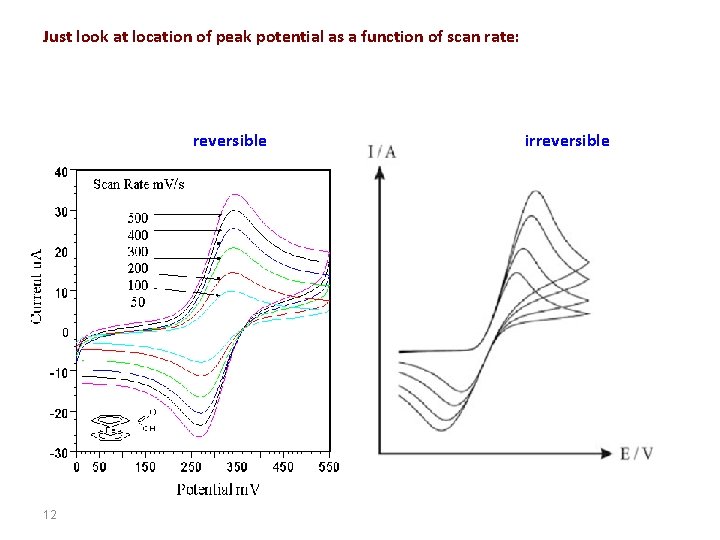
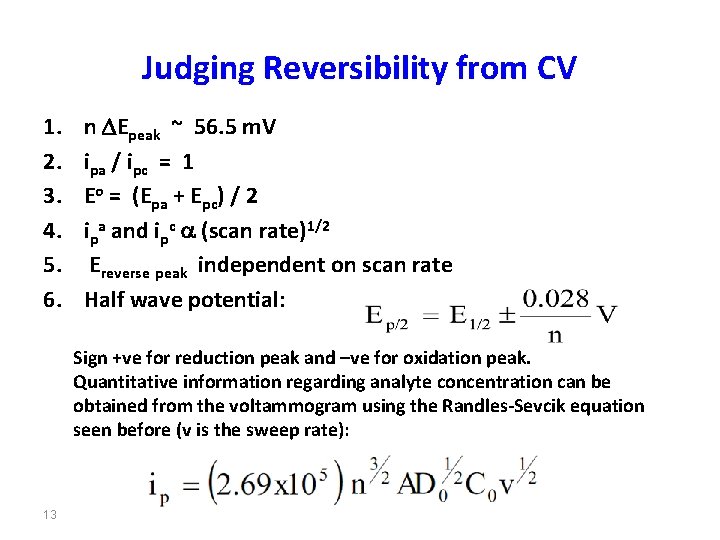
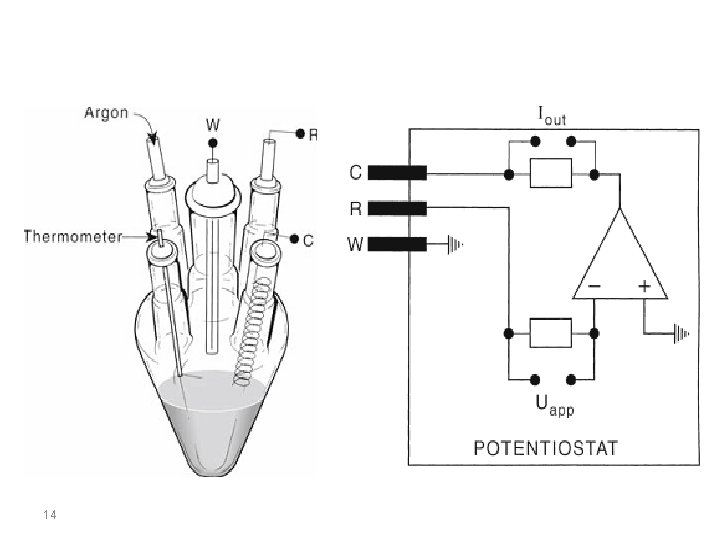
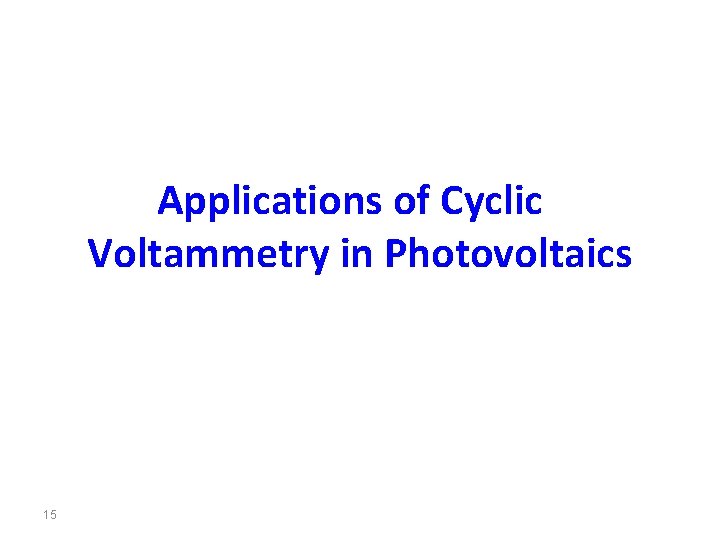
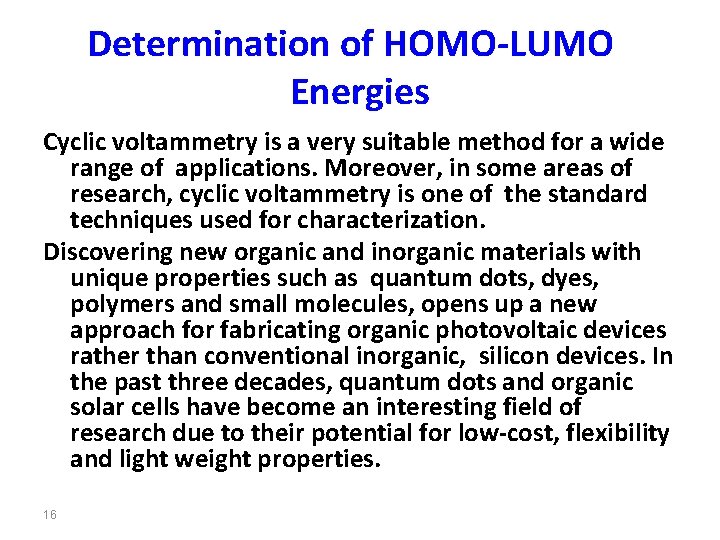
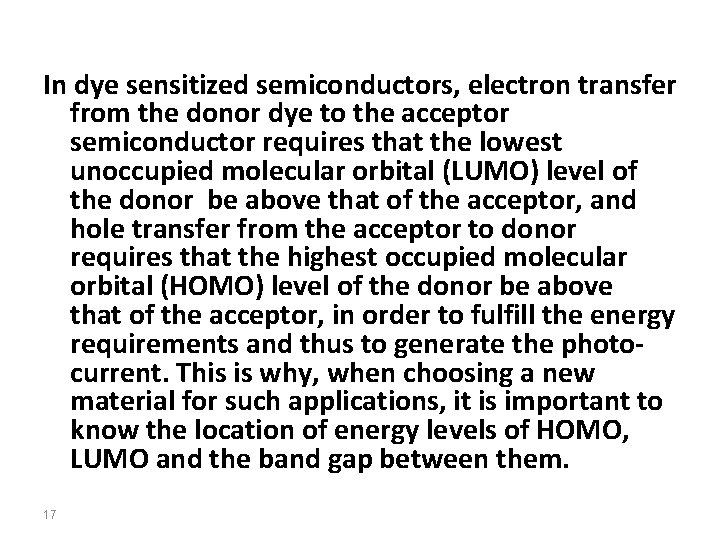
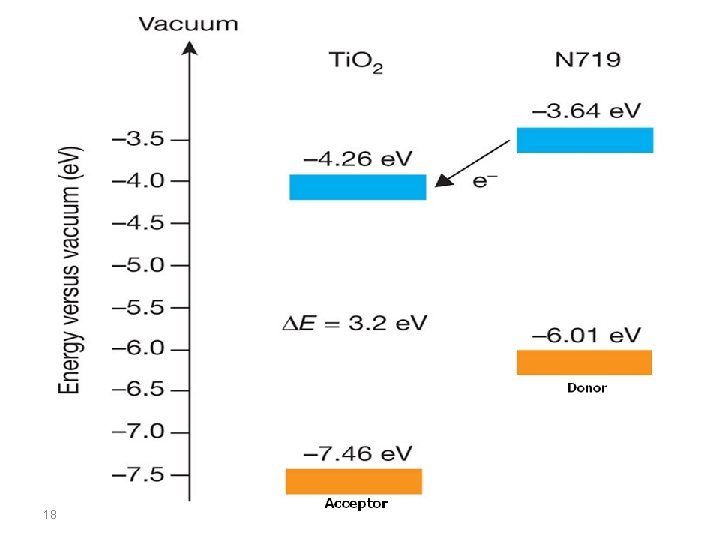
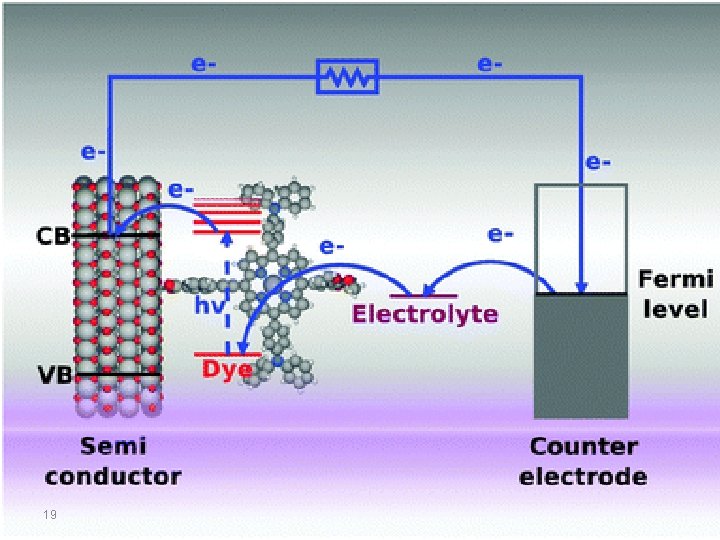
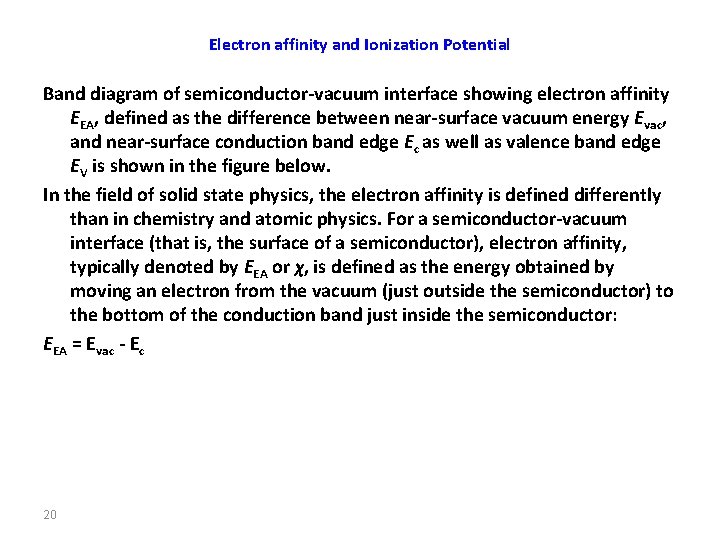
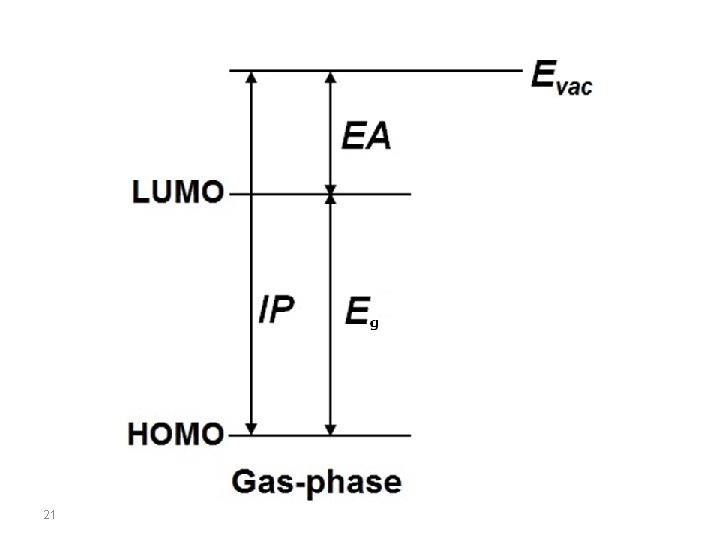
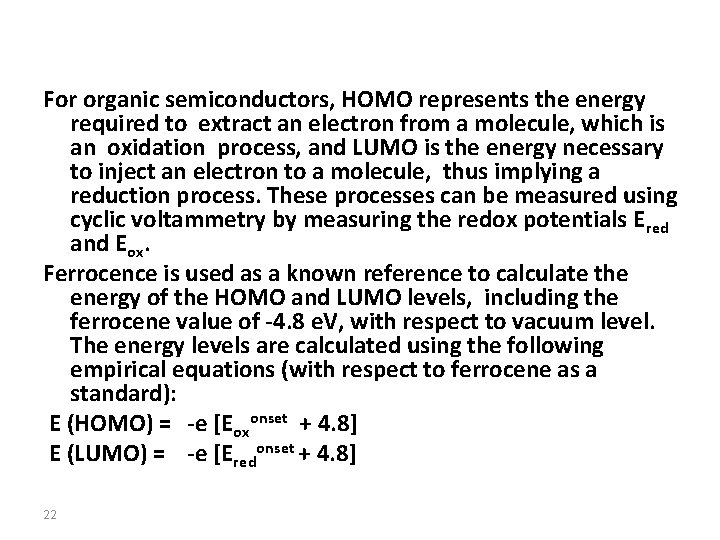
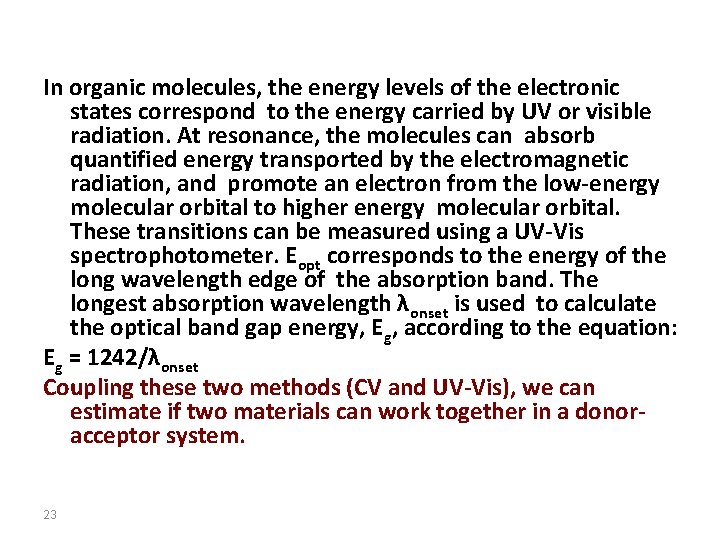
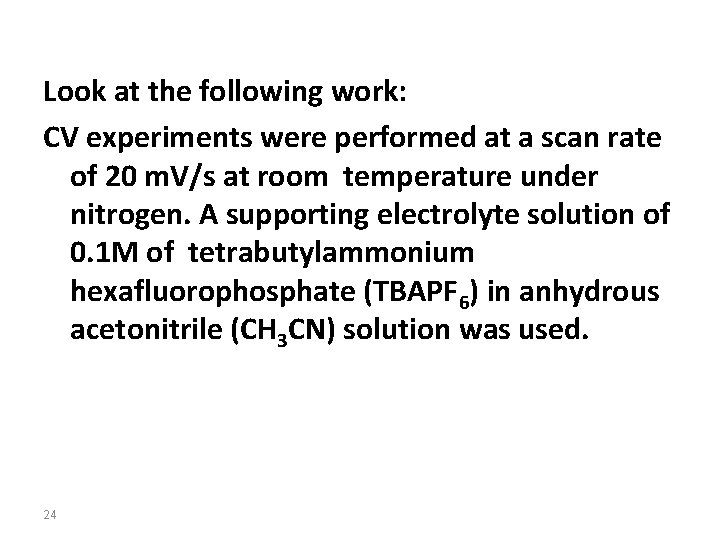
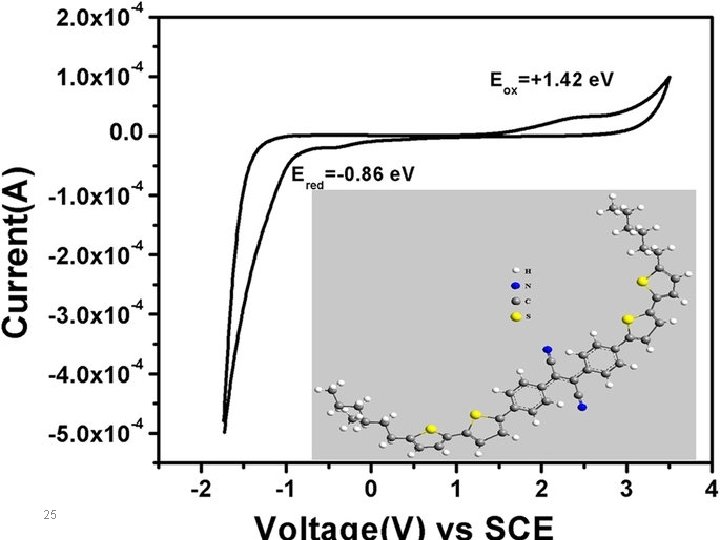
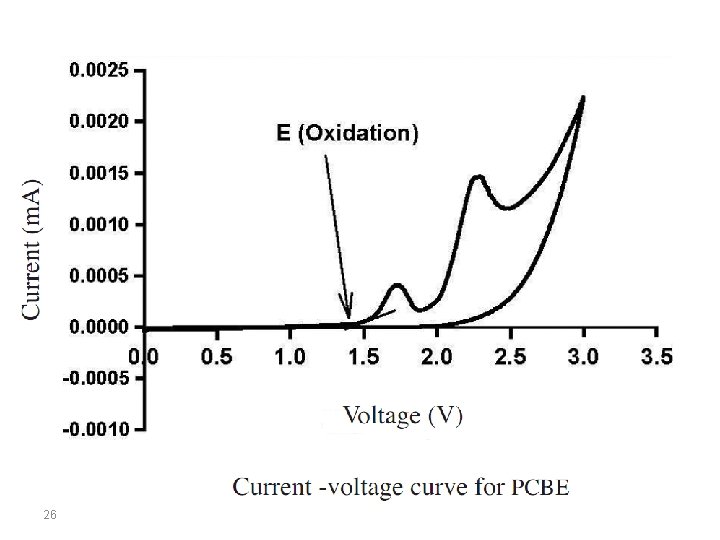
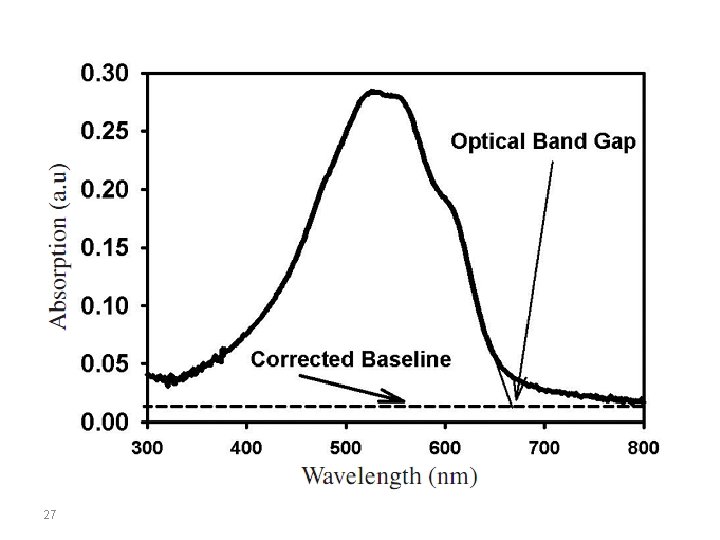
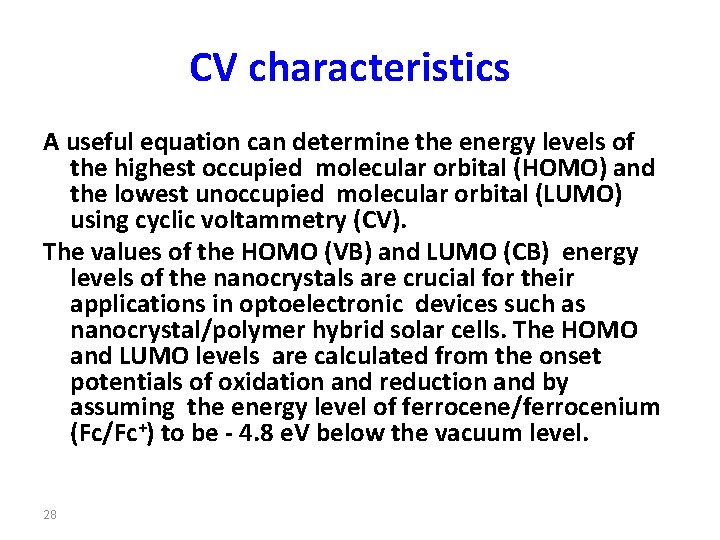
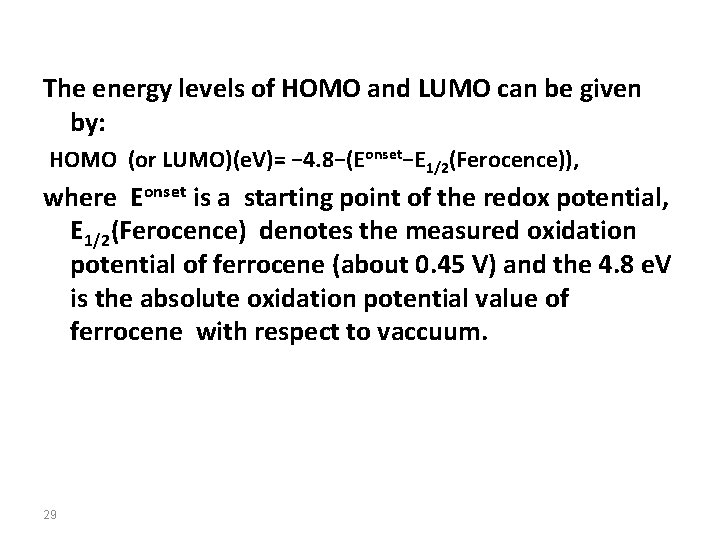
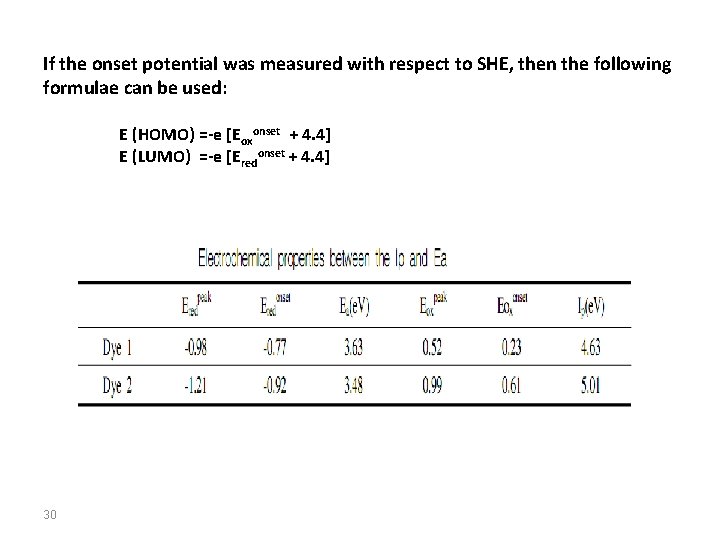
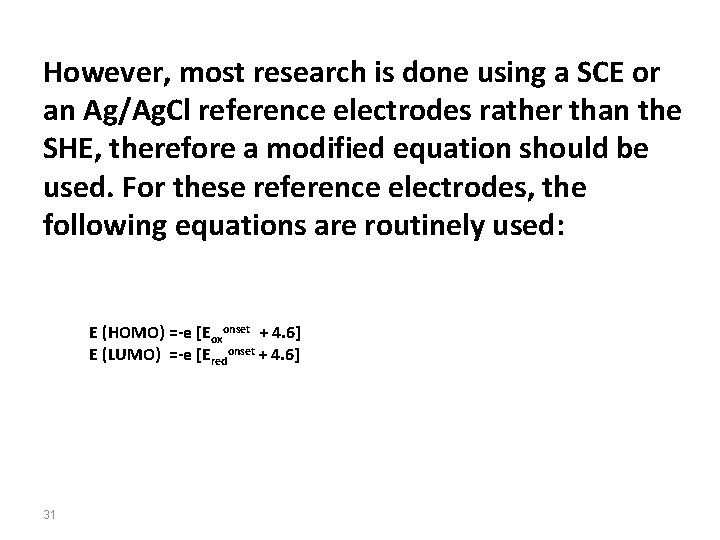
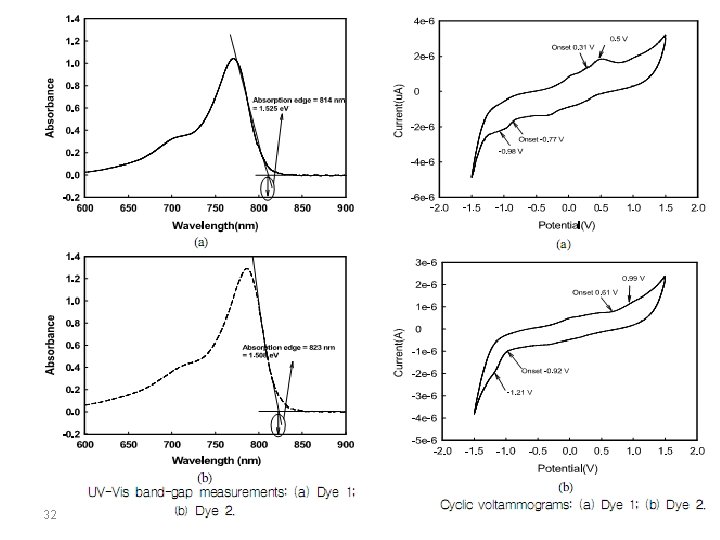
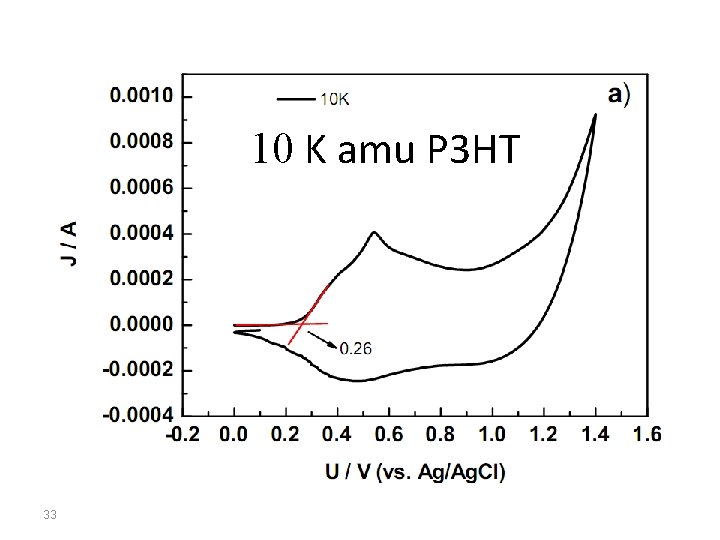
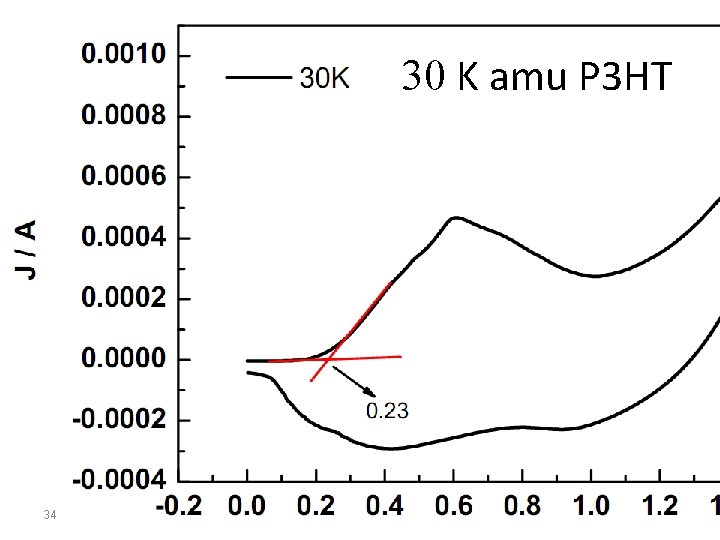
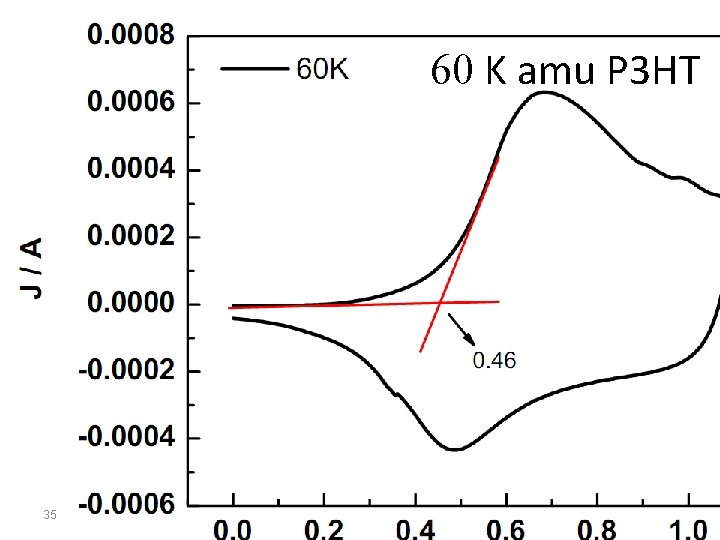
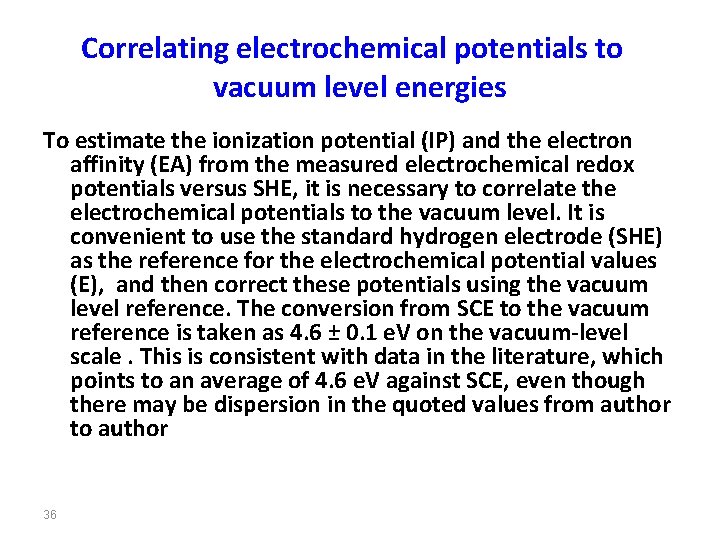
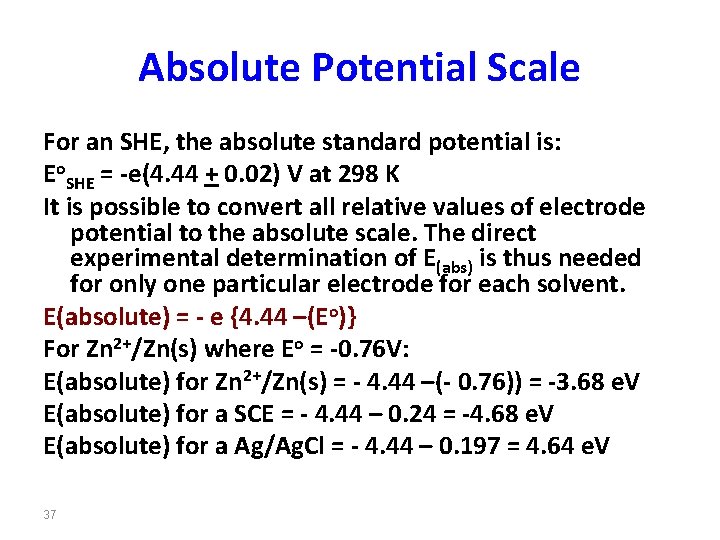
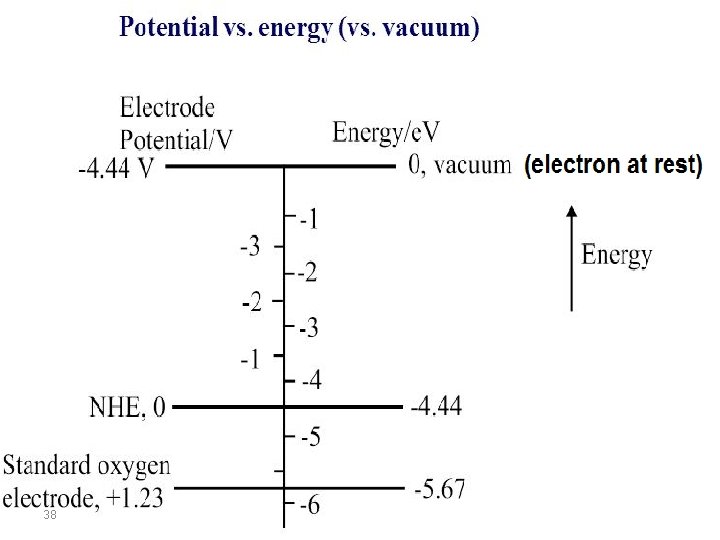
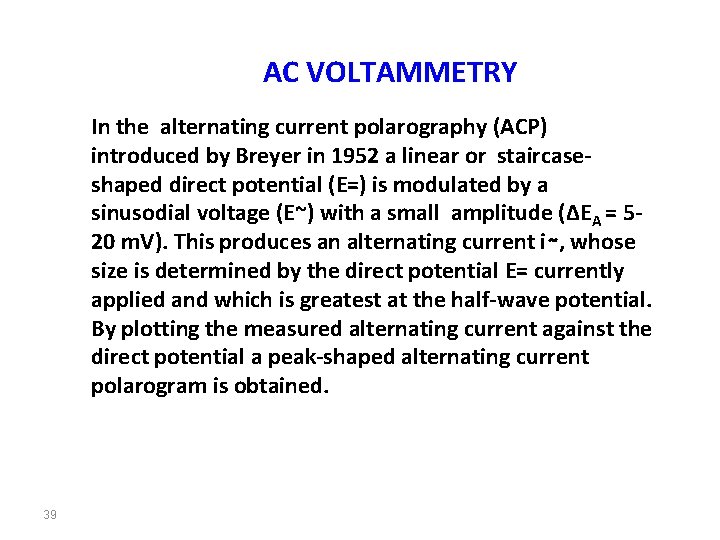
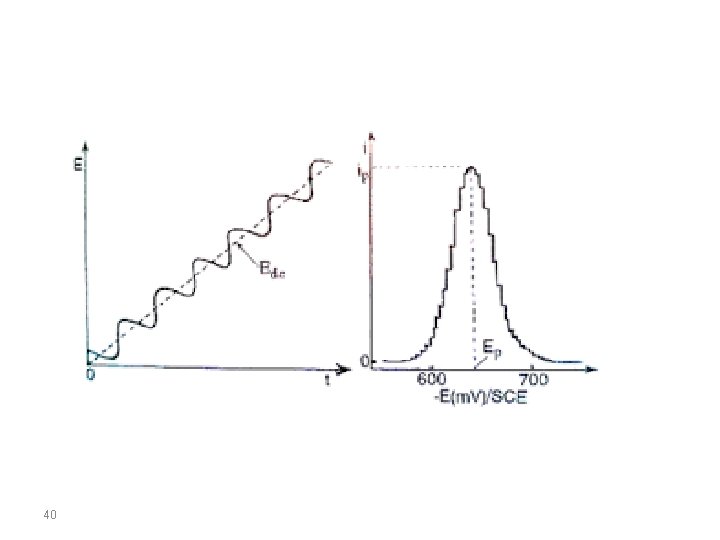
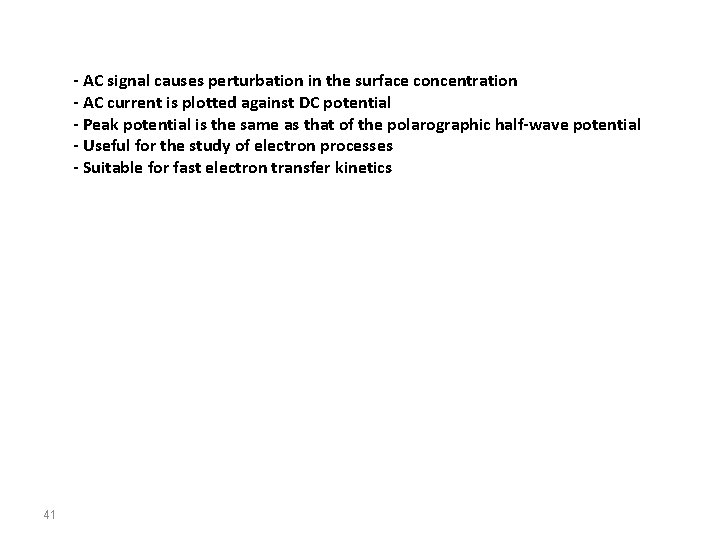
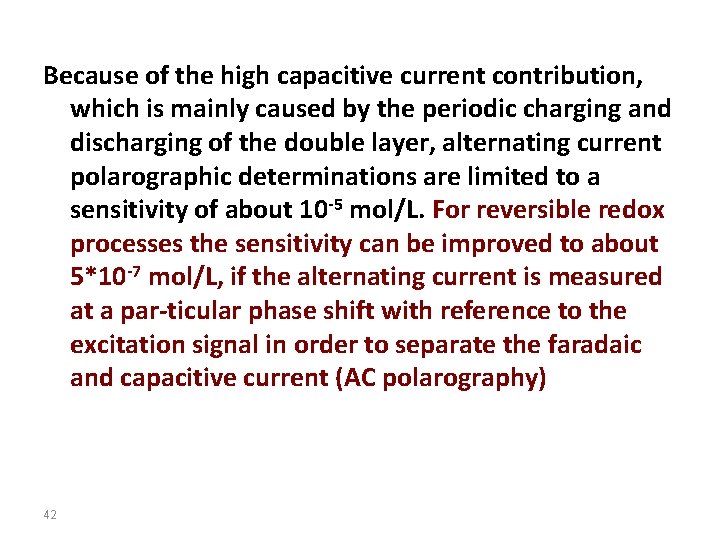
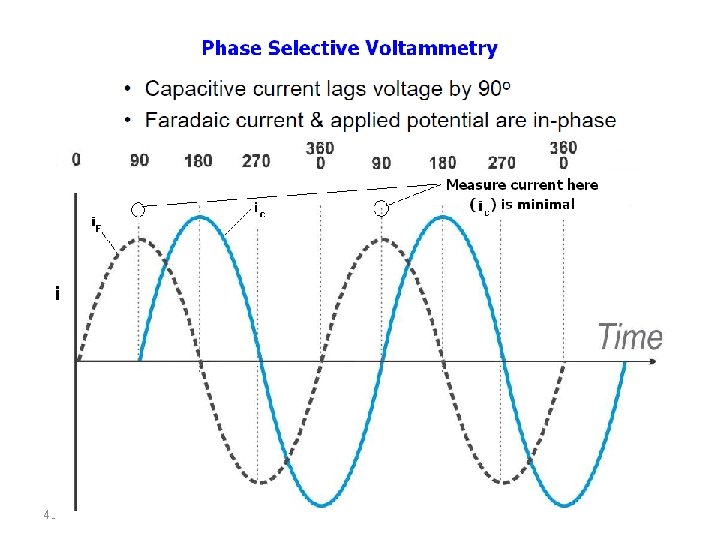
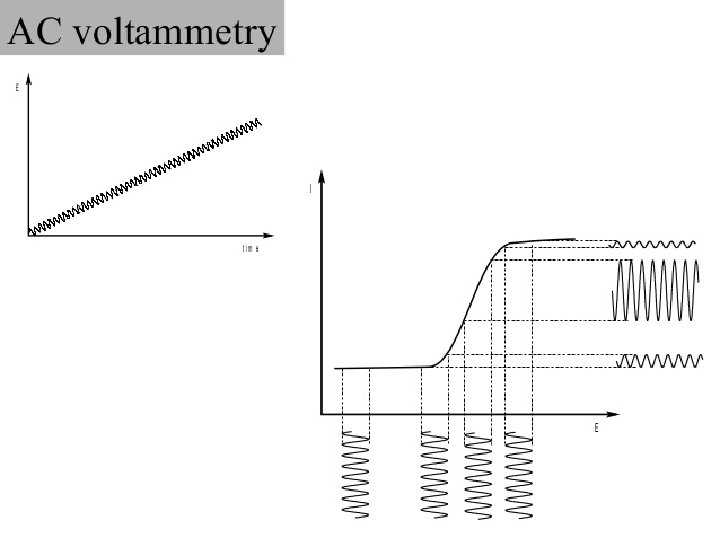
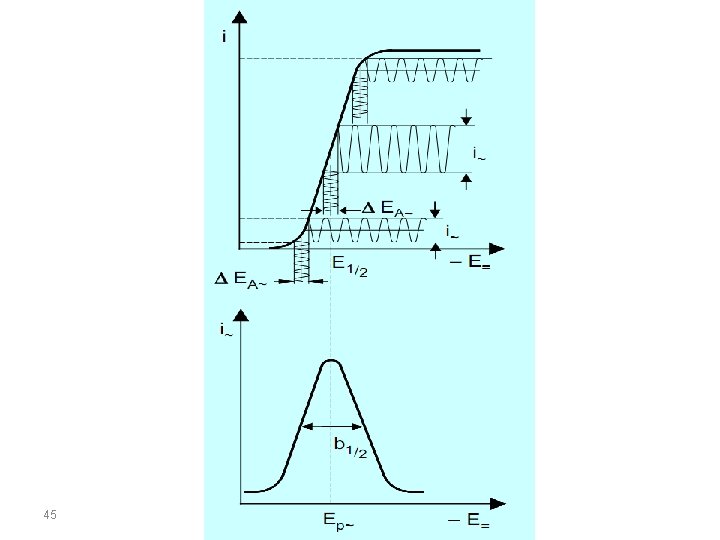
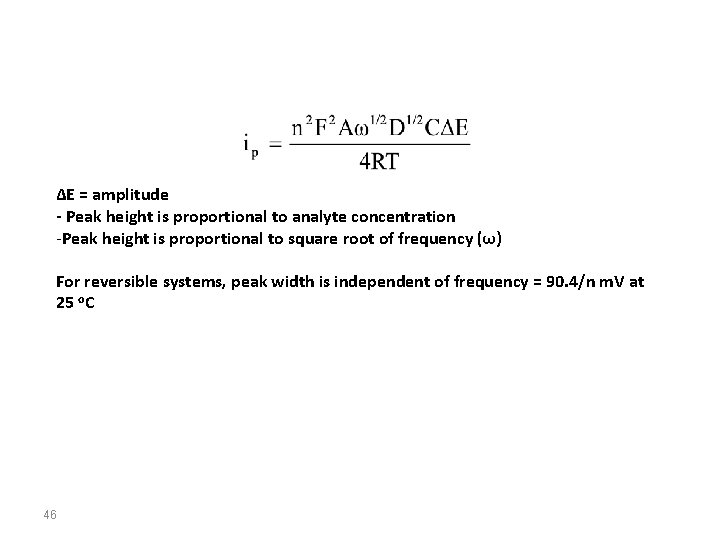
- Slides: 46
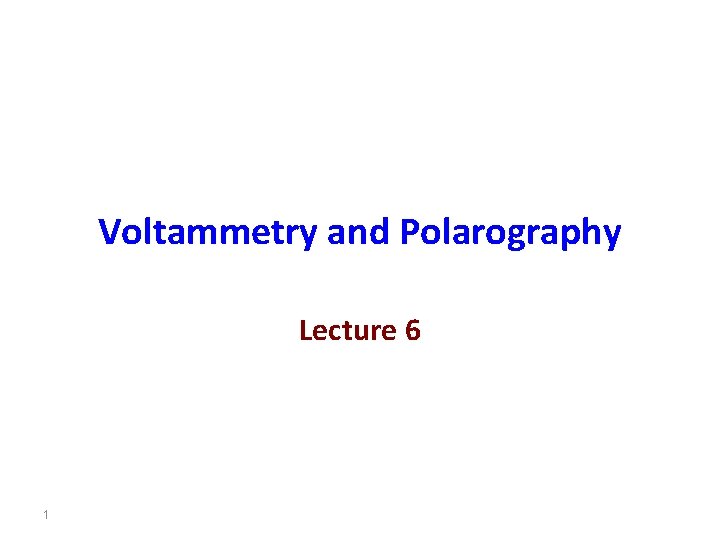
Voltammetry and Polarography Lecture 6 1
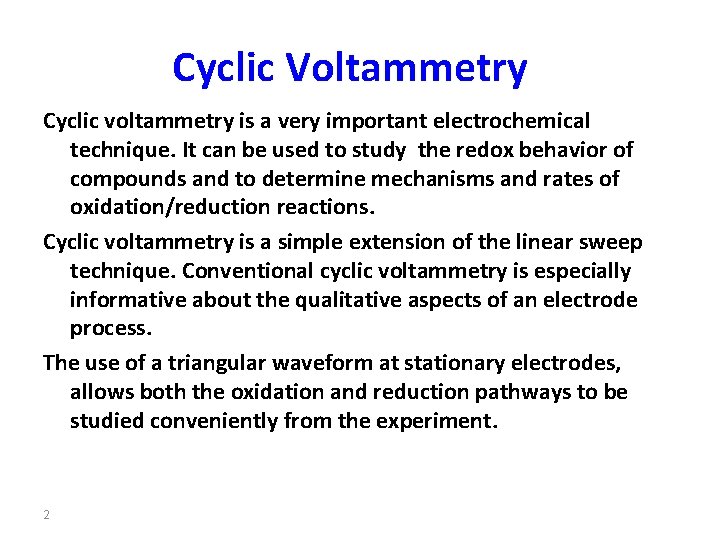
Cyclic Voltammetry Cyclic voltammetry is a very important electrochemical technique. It can be used to study the redox behavior of compounds and to determine mechanisms and rates of oxidation/reduction reactions. Cyclic voltammetry is a simple extension of the linear sweep technique. Conventional cyclic voltammetry is especially informative about the qualitative aspects of an electrode process. The use of a triangular waveform at stationary electrodes, allows both the oxidation and reduction pathways to be studied conveniently from the experiment. 2
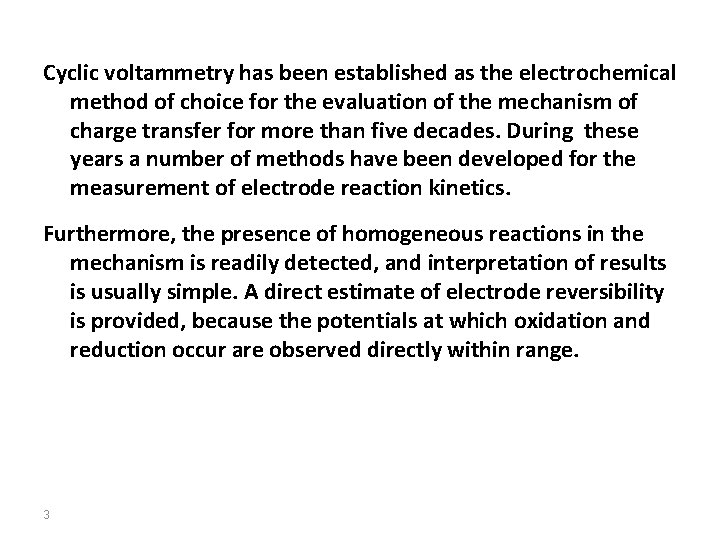
Cyclic voltammetry has been established as the electrochemical method of choice for the evaluation of the mechanism of charge transfer for more than five decades. During these years a number of methods have been developed for the measurement of electrode reaction kinetics. Furthermore, the presence of homogeneous reactions in the mechanism is readily detected, and interpretation of results is usually simple. A direct estimate of electrode reversibility is provided, because the potentials at which oxidation and reduction occur are observed directly within range. 3
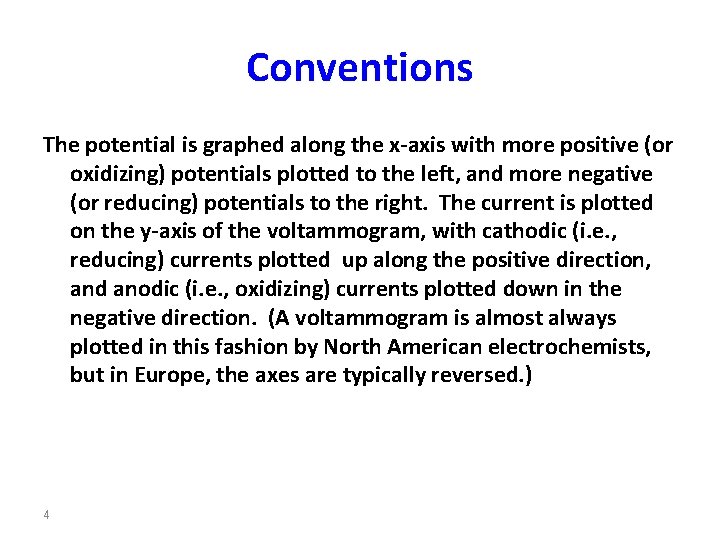
Conventions The potential is graphed along the x-axis with more positive (or oxidizing) potentials plotted to the left, and more negative (or reducing) potentials to the right. The current is plotted on the y-axis of the voltammogram, with cathodic (i. e. , reducing) currents plotted up along the positive direction, and anodic (i. e. , oxidizing) currents plotted down in the negative direction. (A voltammogram is almost always plotted in this fashion by North American electrochemists, but in Europe, the axes are typically reversed. ) 4
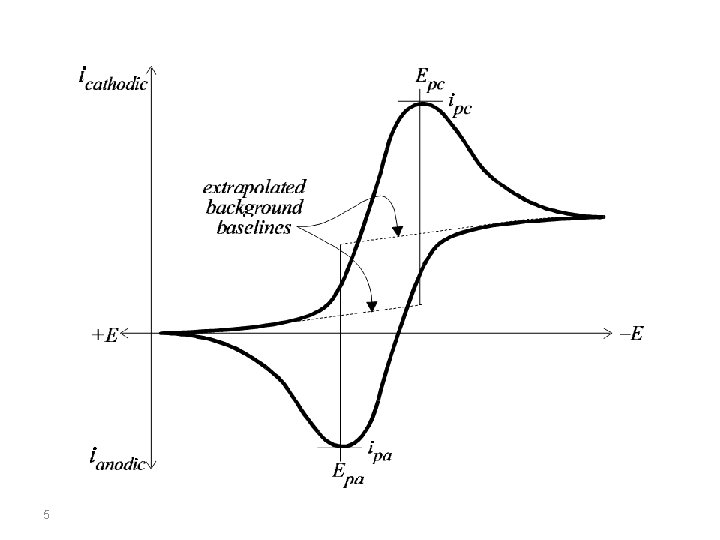
5
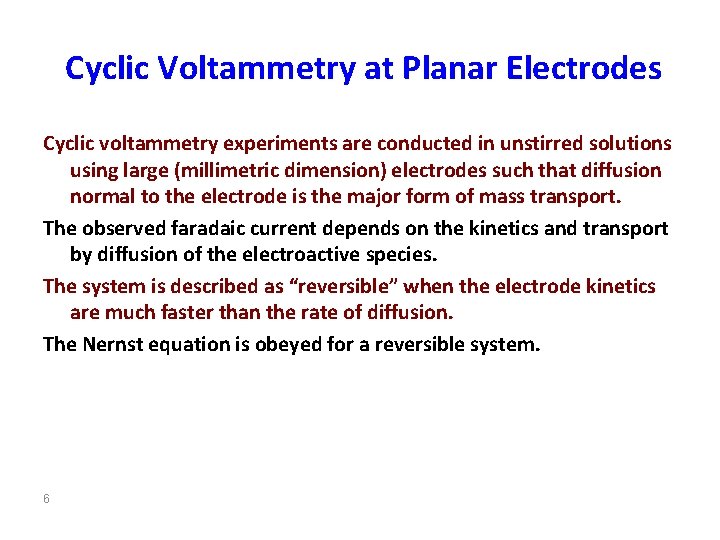
Cyclic Voltammetry at Planar Electrodes Cyclic voltammetry experiments are conducted in unstirred solutions using large (millimetric dimension) electrodes such that diffusion normal to the electrode is the major form of mass transport. The observed faradaic current depends on the kinetics and transport by diffusion of the electroactive species. The system is described as “reversible” when the electrode kinetics are much faster than the rate of diffusion. The Nernst equation is obeyed for a reversible system. 6
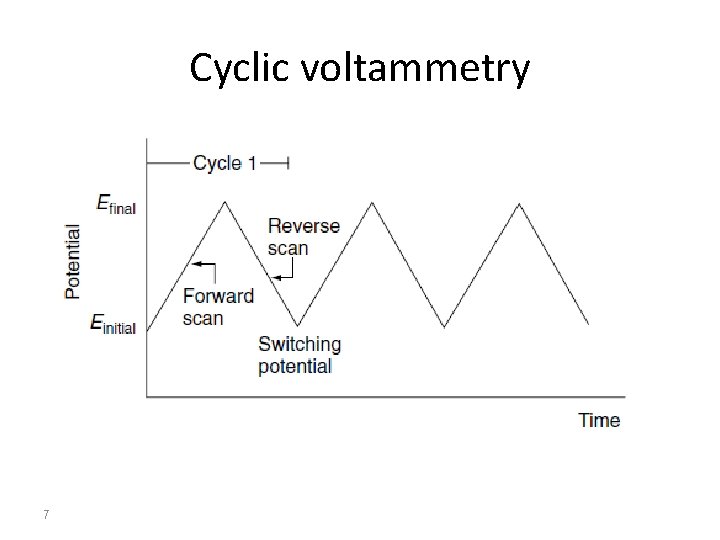
Cyclic voltammetry 7
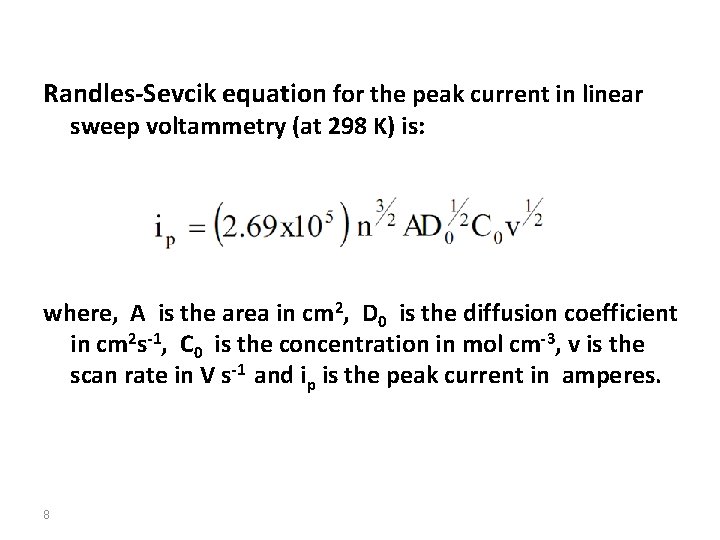
Randles-Sevcik equation for the peak current in linear sweep voltammetry (at 298 K) is: where, A is the area in cm 2, D 0 is the diffusion coefficient in cm 2 s-1, C 0 is the concentration in mol cm-3, v is the scan rate in V s-1 and ip is the peak current in amperes. 8
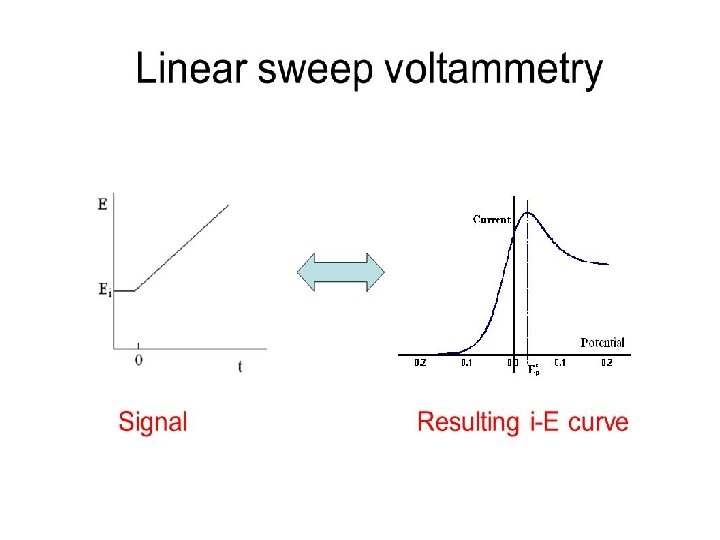
9
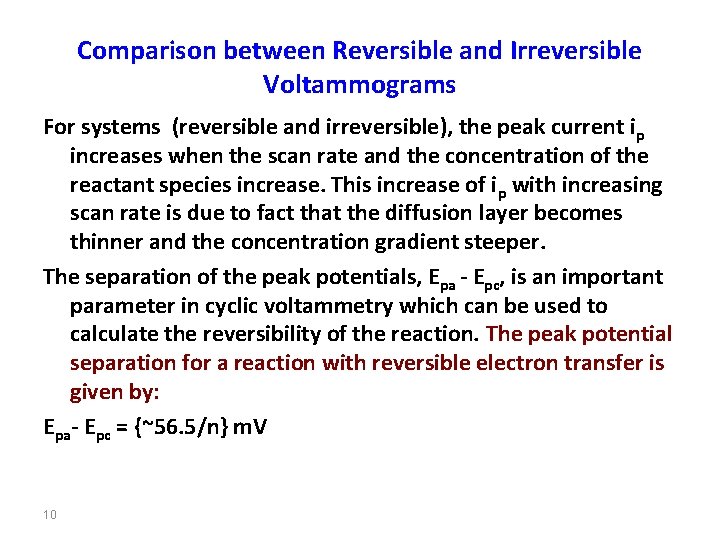
Comparison between Reversible and Irreversible Voltammograms For systems (reversible and irreversible), the peak current i p increases when the scan rate and the concentration of the reactant species increase. This increase of ip with increasing scan rate is due to fact that the diffusion layer becomes thinner and the concentration gradient steeper. The separation of the peak potentials, Epa - Epc, is an important parameter in cyclic voltammetry which can be used to calculate the reversibility of the reaction. The peak potential separation for a reaction with reversible electron transfer is given by: Epa- Epc = {~56. 5/n} m. V 10
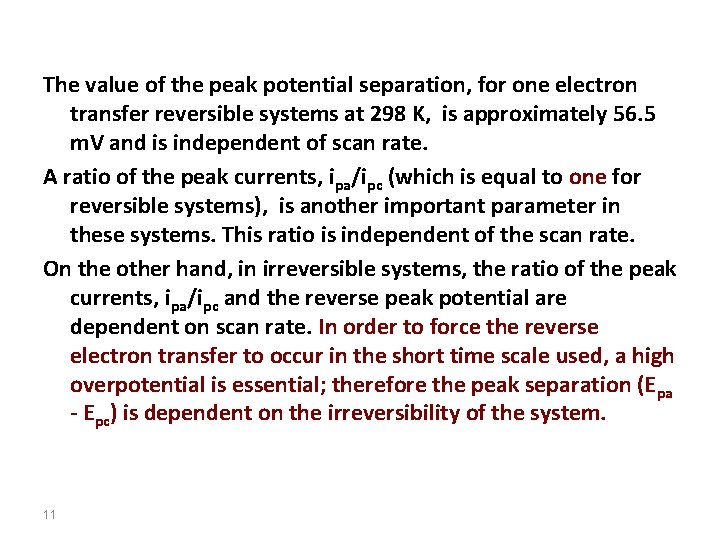
The value of the peak potential separation, for one electron transfer reversible systems at 298 K, is approximately 56. 5 m. V and is independent of scan rate. A ratio of the peak currents, ipa/ipc (which is equal to one for reversible systems), is another important parameter in these systems. This ratio is independent of the scan rate. On the other hand, in irreversible systems, the ratio of the peak currents, ipa/ipc and the reverse peak potential are dependent on scan rate. In order to force the reverse electron transfer to occur in the short time scale used, a high overpotential is essential; therefore the peak separation (E pa - Epc) is dependent on the irreversibility of the system. 11
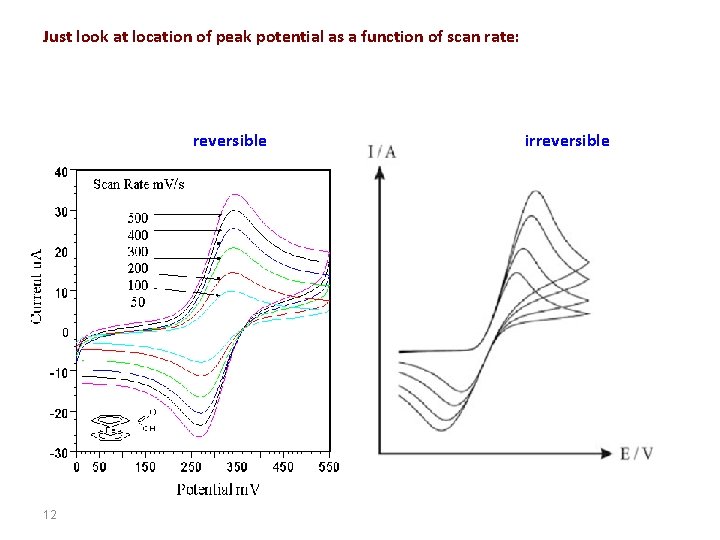
Just look at location of peak potential as a function of scan rate: reversible 12 irreversible
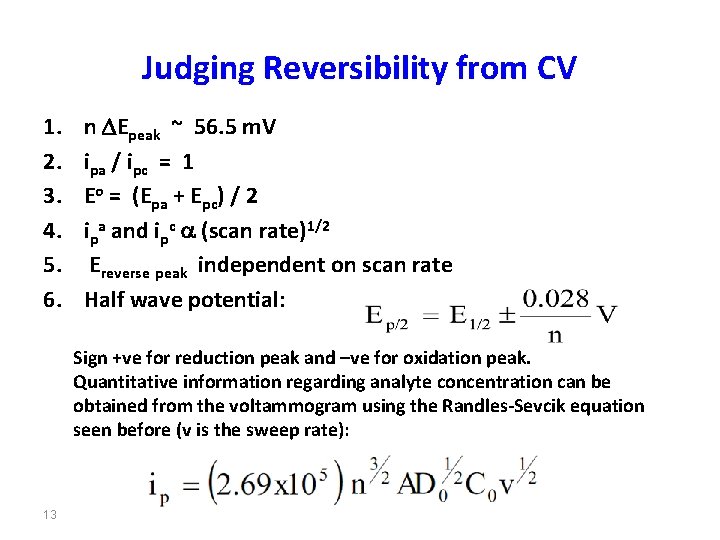
Judging Reversibility from CV 1. 2. 3. 4. 5. 6. n DEpeak ~ 56. 5 m. V ipa / ipc = 1 Eo = (Epa + Epc) / 2 ipa and ipc a (scan rate)1/2 Ereverse peak independent on scan rate Half wave potential: Sign +ve for reduction peak and –ve for oxidation peak. Quantitative information regarding analyte concentration can be obtained from the voltammogram using the Randles-Sevcik equation seen before (v is the sweep rate): 13
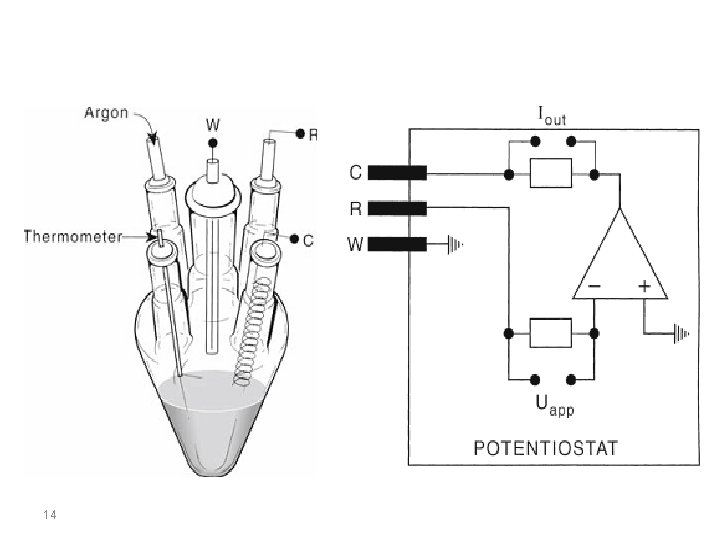
14
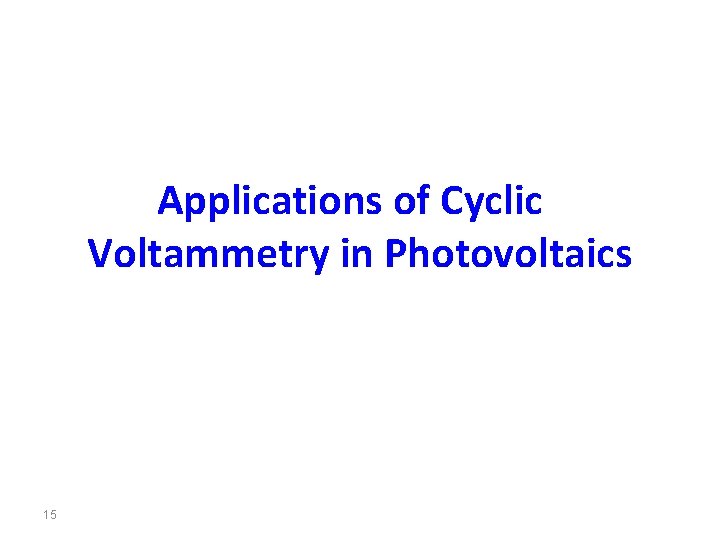
Applications of Cyclic Voltammetry in Photovoltaics 15
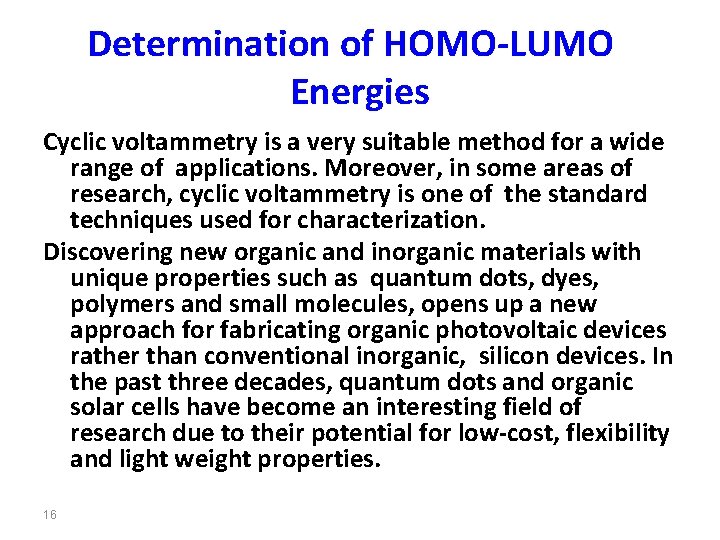
Determination of HOMO-LUMO Energies Cyclic voltammetry is a very suitable method for a wide range of applications. Moreover, in some areas of research, cyclic voltammetry is one of the standard techniques used for characterization. Discovering new organic and inorganic materials with unique properties such as quantum dots, dyes, polymers and small molecules, opens up a new approach for fabricating organic photovoltaic devices rather than conventional inorganic, silicon devices. In the past three decades, quantum dots and organic solar cells have become an interesting field of research due to their potential for low-cost, flexibility and light weight properties. 16
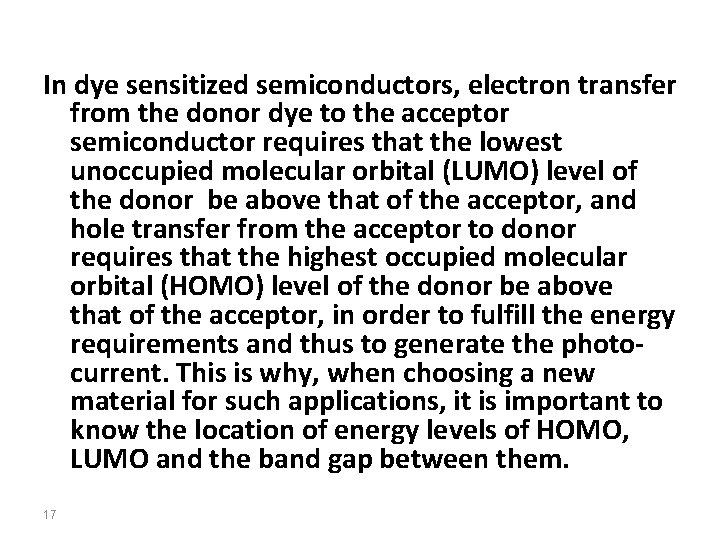
In dye sensitized semiconductors, electron transfer from the donor dye to the acceptor semiconductor requires that the lowest unoccupied molecular orbital (LUMO) level of the donor be above that of the acceptor, and hole transfer from the acceptor to donor requires that the highest occupied molecular orbital (HOMO) level of the donor be above that of the acceptor, in order to fulfill the energy requirements and thus to generate the photocurrent. This is why, when choosing a new material for such applications, it is important to know the location of energy levels of HOMO, LUMO and the band gap between them. 17
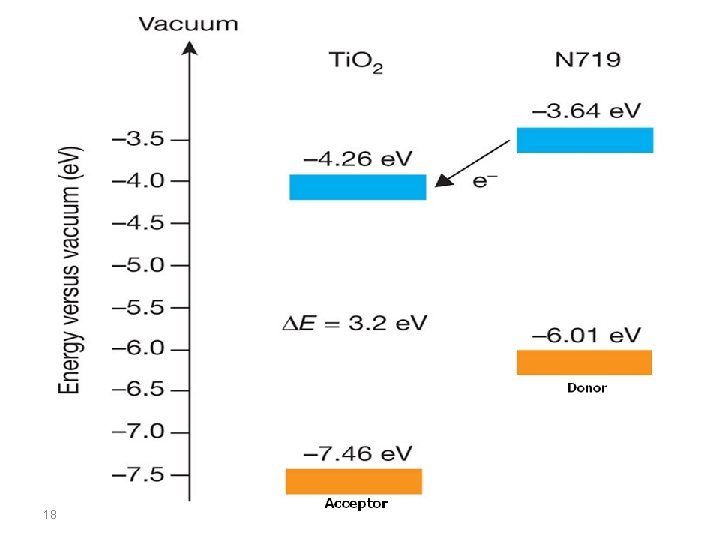
18
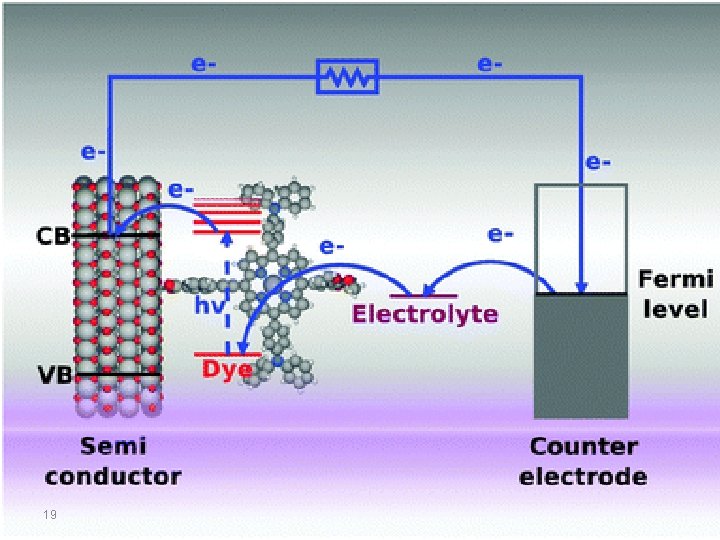
19
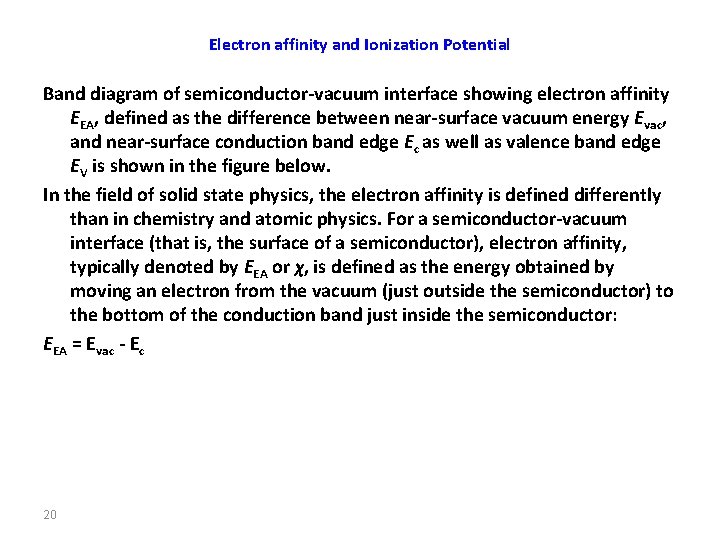
Electron affinity and Ionization Potential Band diagram of semiconductor-vacuum interface showing electron affinity EEA, defined as the difference between near-surface vacuum energy Evac, and near-surface conduction band edge Ec as well as valence band edge EV is shown in the figure below. In the field of solid state physics, the electron affinity is defined differently than in chemistry and atomic physics. For a semiconductor-vacuum interface (that is, the surface of a semiconductor), electron affinity, typically denoted by EEA or χ, is defined as the energy obtained by moving an electron from the vacuum (just outside the semiconductor) to the bottom of the conduction band just inside the semiconductor: EEA = Evac - Ec 20
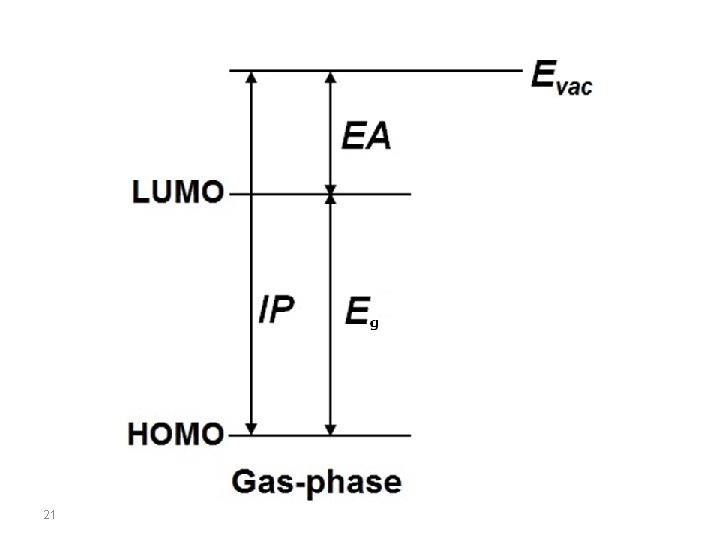
21
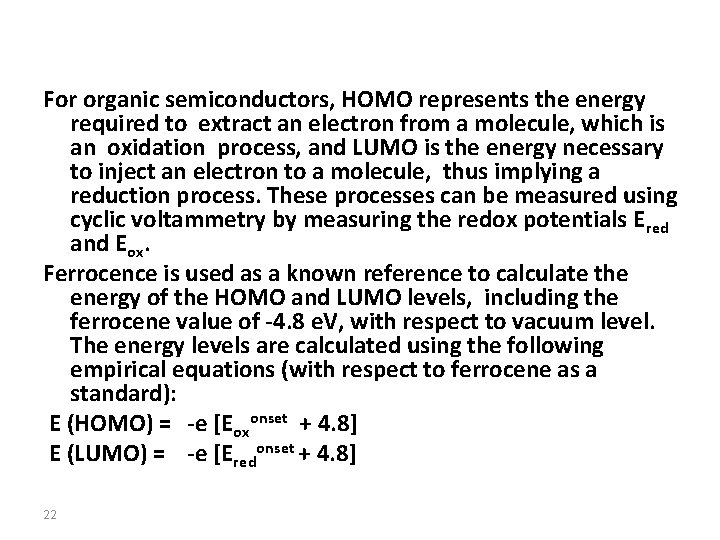
For organic semiconductors, HOMO represents the energy required to extract an electron from a molecule, which is an oxidation process, and LUMO is the energy necessary to inject an electron to a molecule, thus implying a reduction process. These processes can be measured using cyclic voltammetry by measuring the redox potentials Ered and Eox. Ferrocence is used as a known reference to calculate the energy of the HOMO and LUMO levels, including the ferrocene value of -4. 8 e. V, with respect to vacuum level. The energy levels are calculated using the following empirical equations (with respect to ferrocene as a standard): E (HOMO) = -e [Eoxonset + 4. 8] E (LUMO) = -e [Eredonset + 4. 8] 22
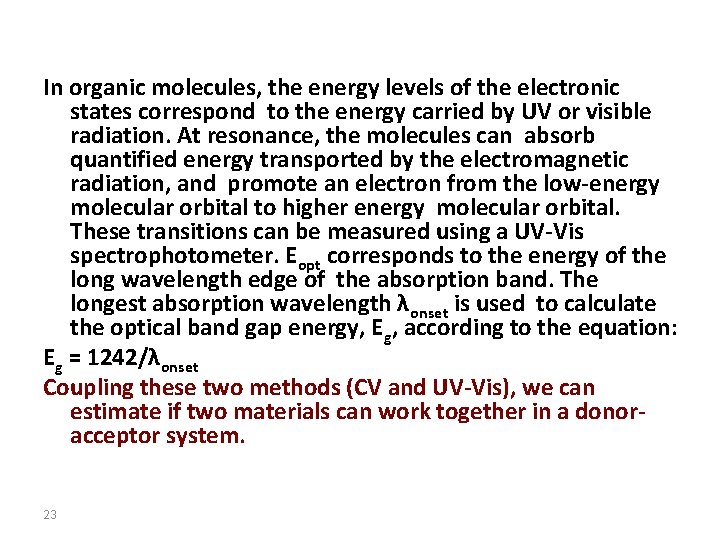
In organic molecules, the energy levels of the electronic states correspond to the energy carried by UV or visible radiation. At resonance, the molecules can absorb quantified energy transported by the electromagnetic radiation, and promote an electron from the low-energy molecular orbital to higher energy molecular orbital. These transitions can be measured using a UV-Vis spectrophotometer. Eopt corresponds to the energy of the long wavelength edge of the absorption band. The longest absorption wavelength λonset is used to calculate the optical band gap energy, Eg, according to the equation: Eg = 1242/λonset Coupling these two methods (CV and UV-Vis), we can estimate if two materials can work together in a donoracceptor system. 23
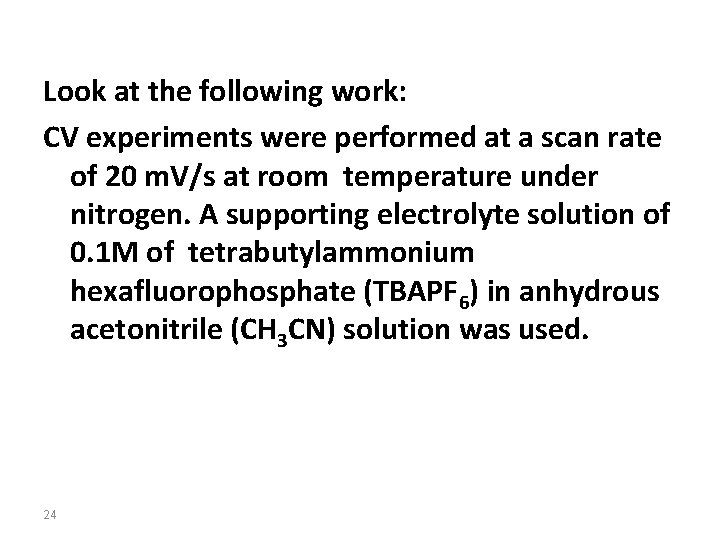
Look at the following work: CV experiments were performed at a scan rate of 20 m. V/s at room temperature under nitrogen. A supporting electrolyte solution of 0. 1 M of tetrabutylammonium hexafluorophosphate (TBAPF 6) in anhydrous acetonitrile (CH 3 CN) solution was used. 24
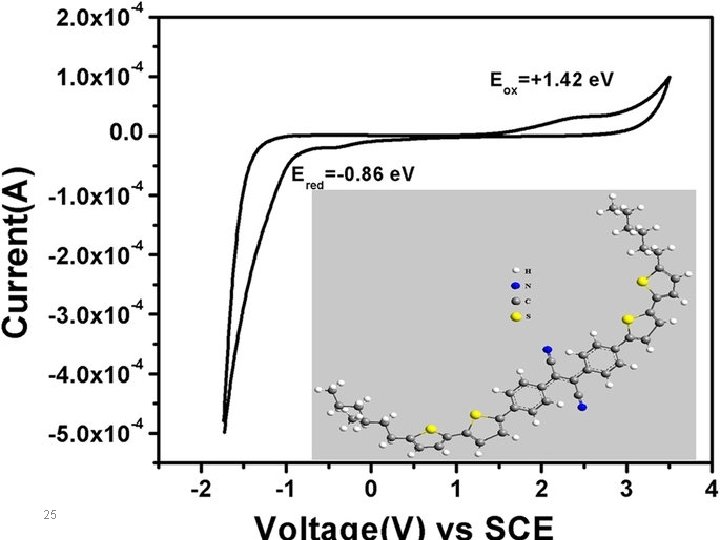
25
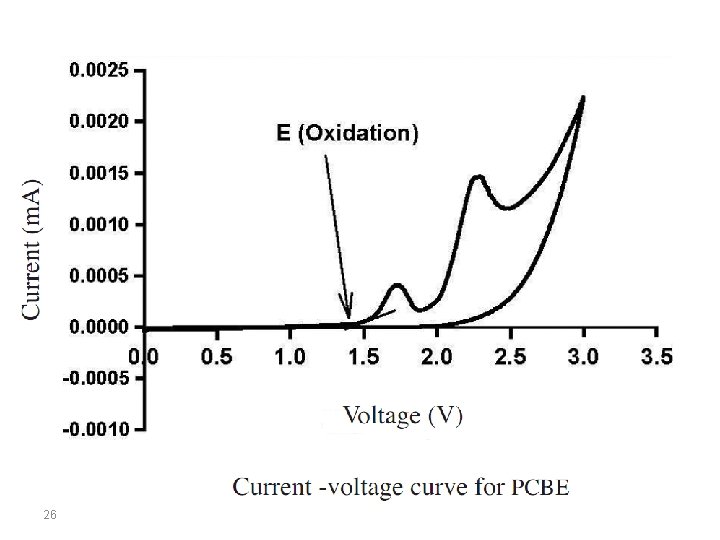
26
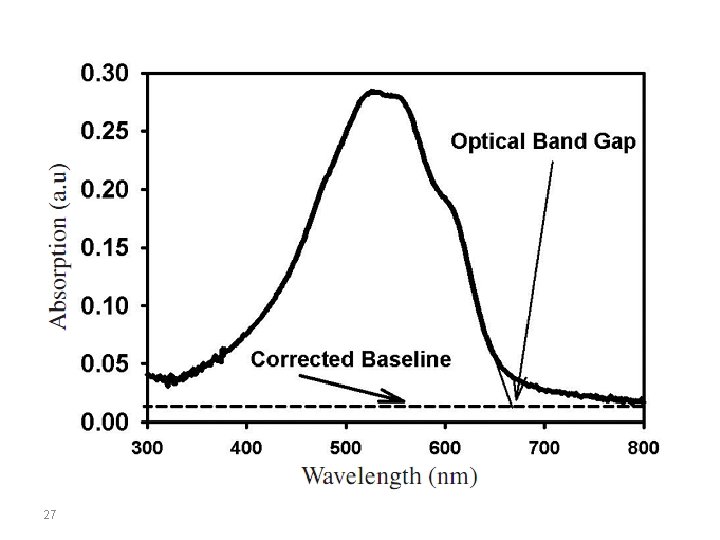
27
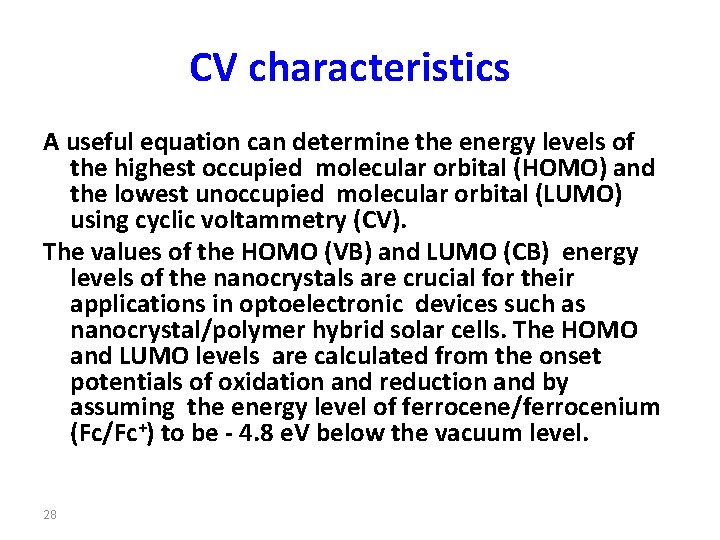
CV characteristics A useful equation can determine the energy levels of the highest occupied molecular orbital (HOMO) and the lowest unoccupied molecular orbital (LUMO) using cyclic voltammetry (CV). The values of the HOMO (VB) and LUMO (CB) energy levels of the nanocrystals are crucial for their applications in optoelectronic devices such as nanocrystal/polymer hybrid solar cells. The HOMO and LUMO levels are calculated from the onset potentials of oxidation and reduction and by assuming the energy level of ferrocene/ferrocenium (Fc/Fc+) to be - 4. 8 e. V below the vacuum level. 28
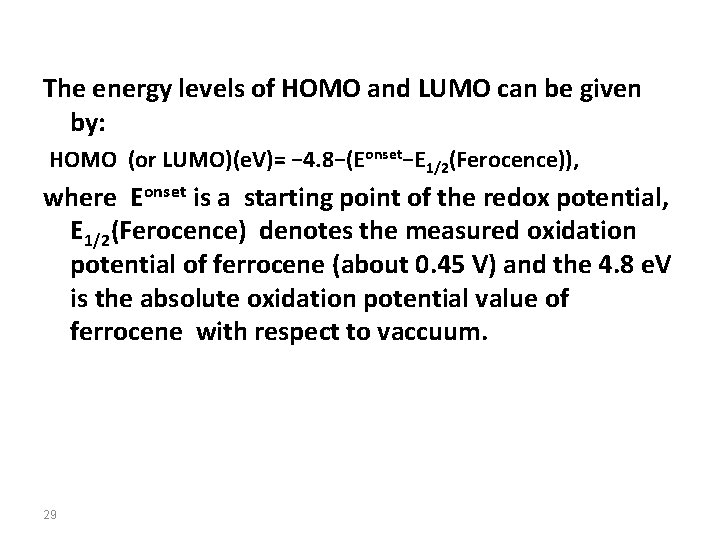
The energy levels of HOMO and LUMO can be given by: HOMO (or LUMO)(e. V)= − 4. 8−(Eonset−E 1/2(Ferocence)), where Eonset is a starting point of the redox potential, E 1/2(Ferocence) denotes the measured oxidation potential of ferrocene (about 0. 45 V) and the 4. 8 e. V is the absolute oxidation potential value of ferrocene with respect to vaccuum. 29
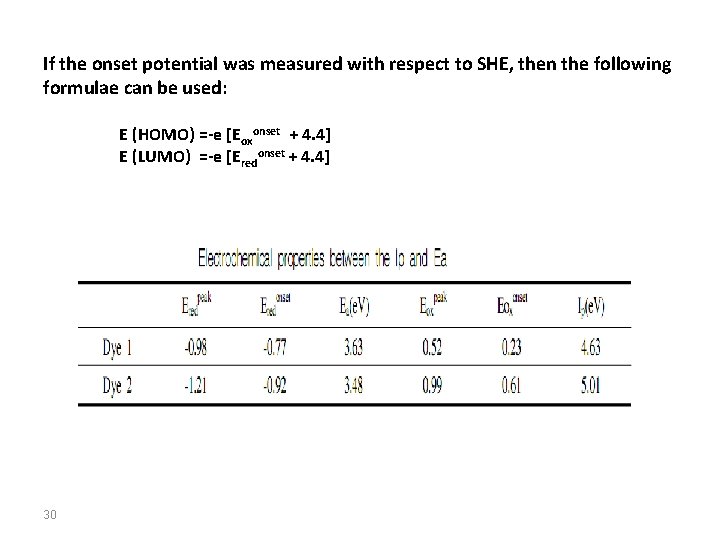
If the onset potential was measured with respect to SHE, then the following formulae can be used: E (HOMO) =-e [Eoxonset + 4. 4] E (LUMO) =-e [Eredonset + 4. 4] 30
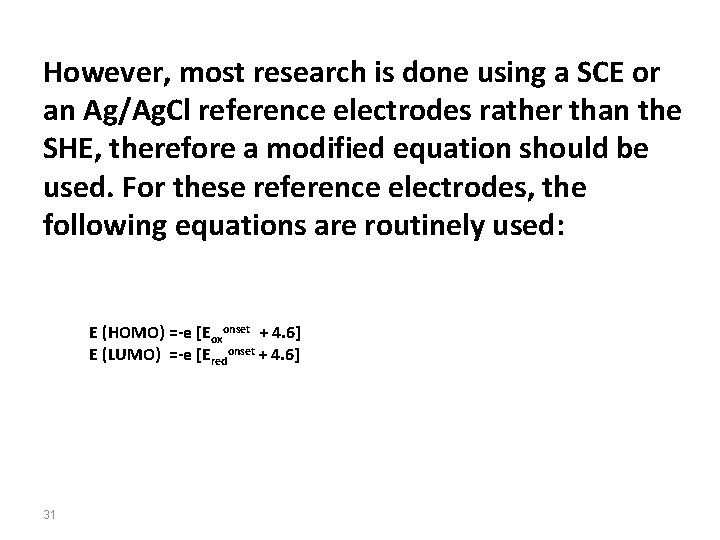
However, most research is done using a SCE or an Ag/Ag. Cl reference electrodes rather than the SHE, therefore a modified equation should be used. For these reference electrodes, the following equations are routinely used: E (HOMO) =-e [Eoxonset + 4. 6] E (LUMO) =-e [Eredonset + 4. 6] 31
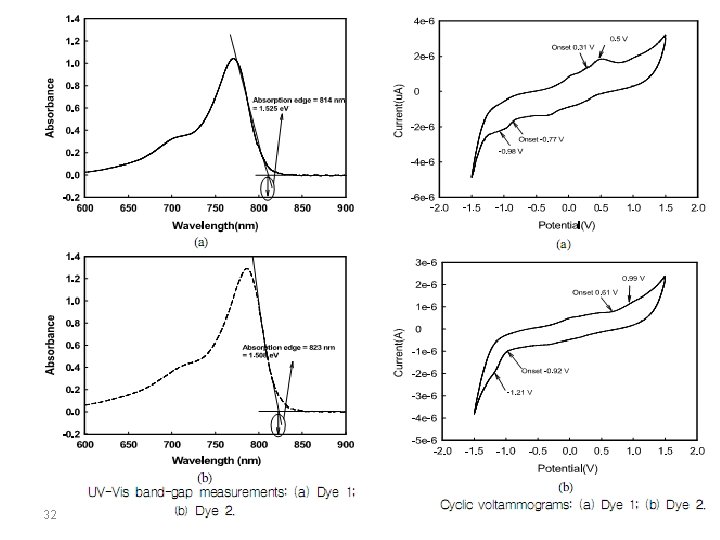
32
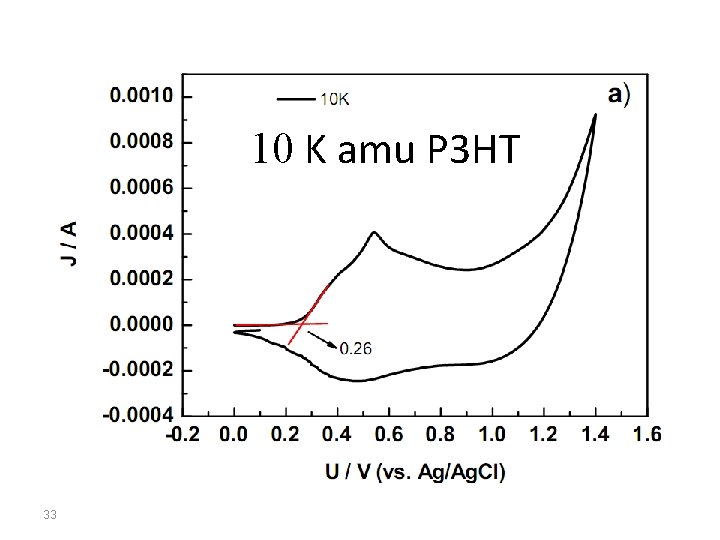
10 K amu P 3 HT 33
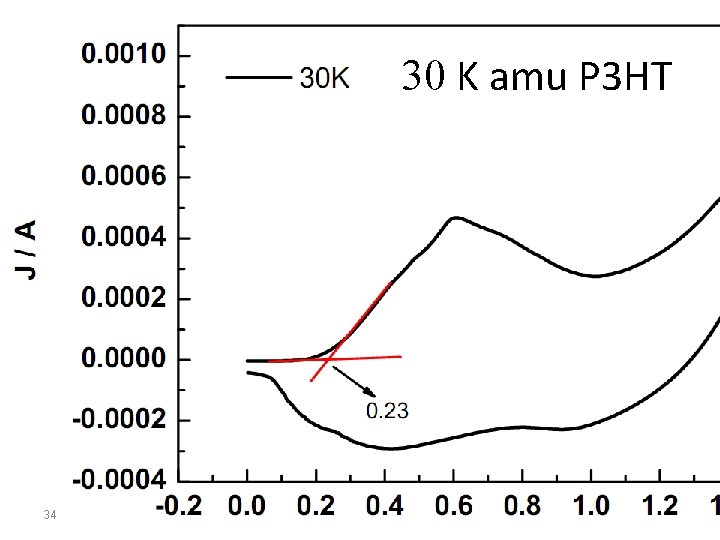
30 K amu P 3 HT 34
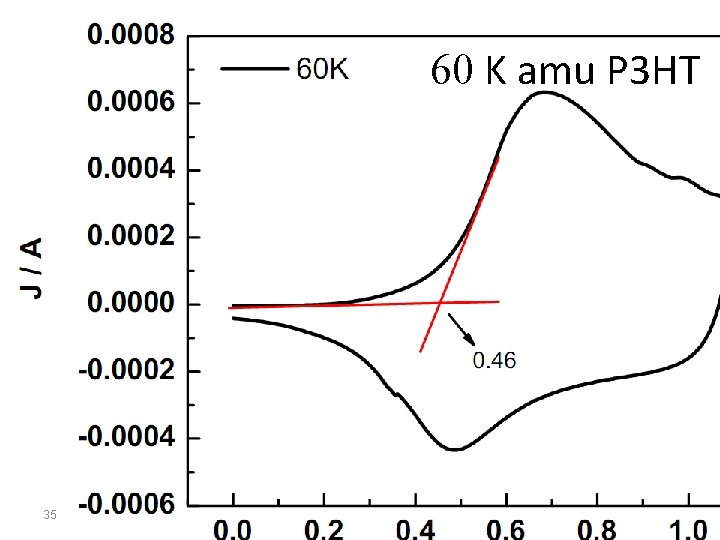
60 K amu P 3 HT 35
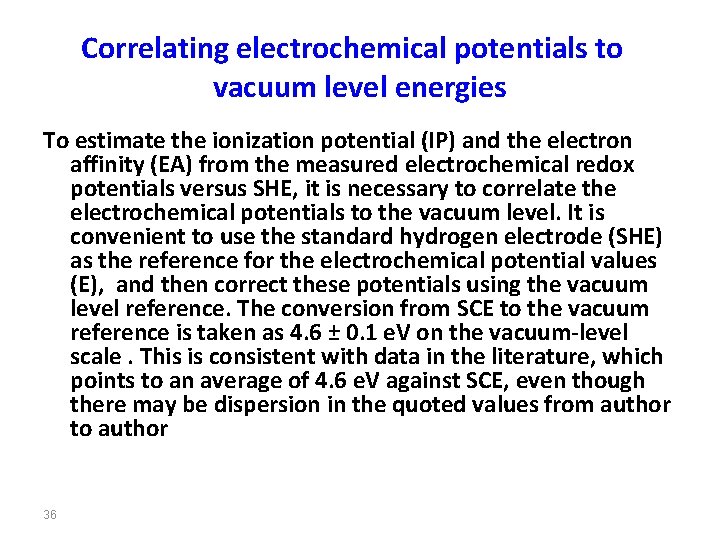
Correlating electrochemical potentials to vacuum level energies To estimate the ionization potential (IP) and the electron affinity (EA) from the measured electrochemical redox potentials versus SHE, it is necessary to correlate the electrochemical potentials to the vacuum level. It is convenient to use the standard hydrogen electrode (SHE) as the reference for the electrochemical potential values (E), and then correct these potentials using the vacuum level reference. The conversion from SCE to the vacuum reference is taken as 4. 6 ± 0. 1 e. V on the vacuum-level scale. This is consistent with data in the literature, which points to an average of 4. 6 e. V against SCE, even though there may be dispersion in the quoted values from author to author 36
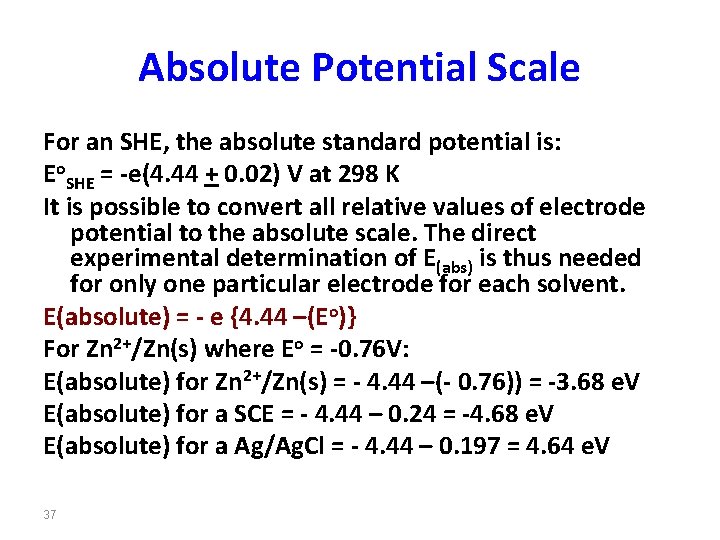
Absolute Potential Scale For an SHE, the absolute standard potential is: Eo. SHE = -e(4. 44 + 0. 02) V at 298 K It is possible to convert all relative values of electrode potential to the absolute scale. The direct experimental determination of E(abs) is thus needed for only one particular electrode for each solvent. E(absolute) = - e {4. 44 –(Eo)} For Zn 2+/Zn(s) where Eo = -0. 76 V: E(absolute) for Zn 2+/Zn(s) = - 4. 44 –(- 0. 76)) = -3. 68 e. V E(absolute) for a SCE = - 4. 44 – 0. 24 = -4. 68 e. V E(absolute) for a Ag/Ag. Cl = - 4. 44 – 0. 197 = 4. 64 e. V 37
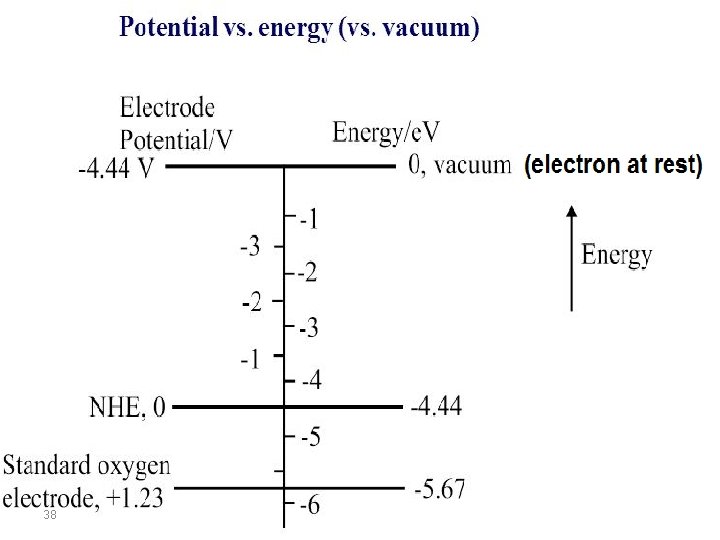
38
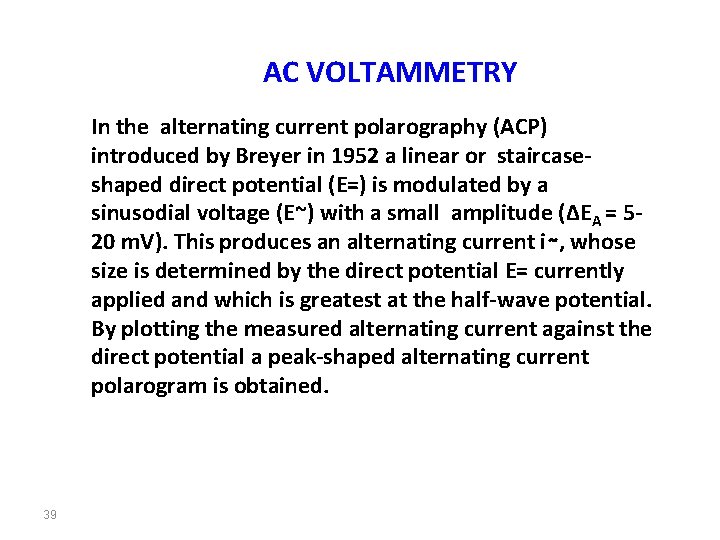
AC VOLTAMMETRY In the alternating current polarography (ACP) introduced by Breyer in 1952 a linear or staircaseshaped direct potential (E=) is modulated by a sinusodial voltage (E~) with a small amplitude (∆EA = 520 m. V). This produces an alternating current i∼, whose size is determined by the direct potential E= currently applied and which is greatest at the half-wave potential. By plotting the measured alternating current against the direct potential a peak-shaped alternating current polarogram is obtained. 39
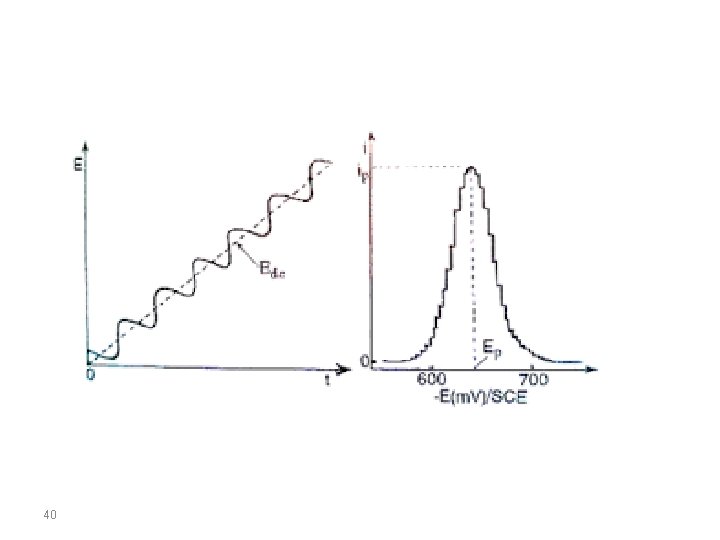
40
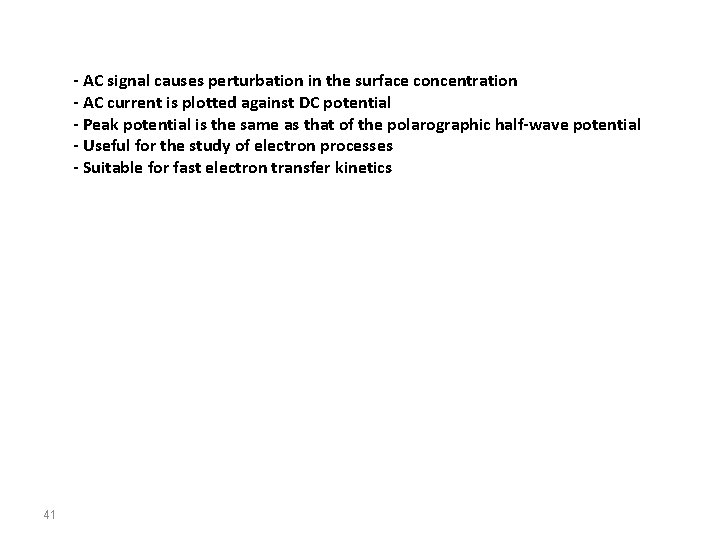
- AC signal causes perturbation in the surface concentration - AC current is plotted against DC potential - Peak potential is the same as that of the polarographic half-wave potential - Useful for the study of electron processes - Suitable for fast electron transfer kinetics 41
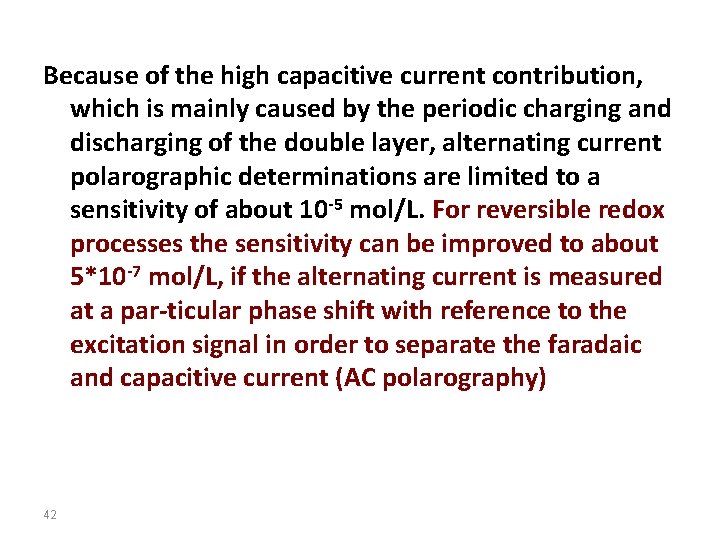
Because of the high capacitive current contribution, which is mainly caused by the periodic charging and discharging of the double layer, alternating current polarographic determinations are limited to a sensitivity of about 10 -5 mol/L. For reversible redox processes the sensitivity can be improved to about 5*10 -7 mol/L, if the alternating current is measured at a par-ticular phase shift with reference to the excitation signal in order to separate the faradaic and capacitive current (AC polarography) 42
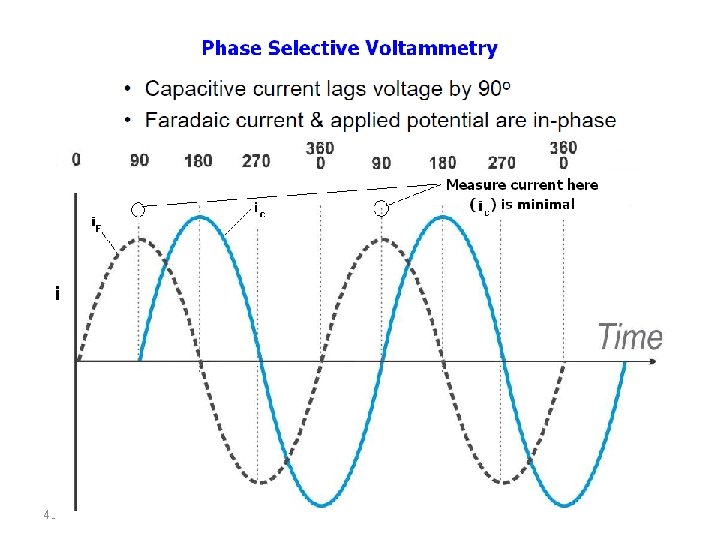
43
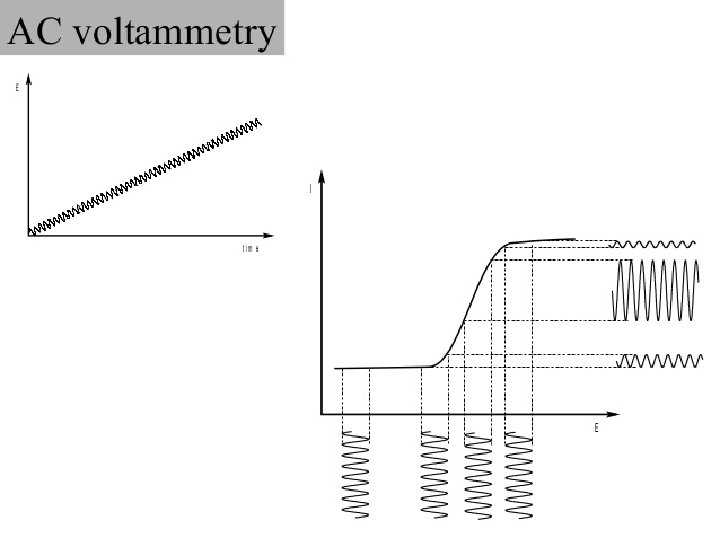
44
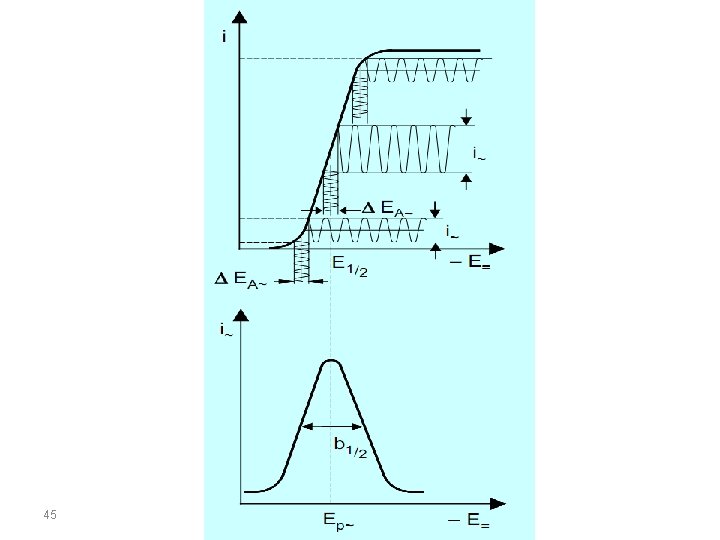
45
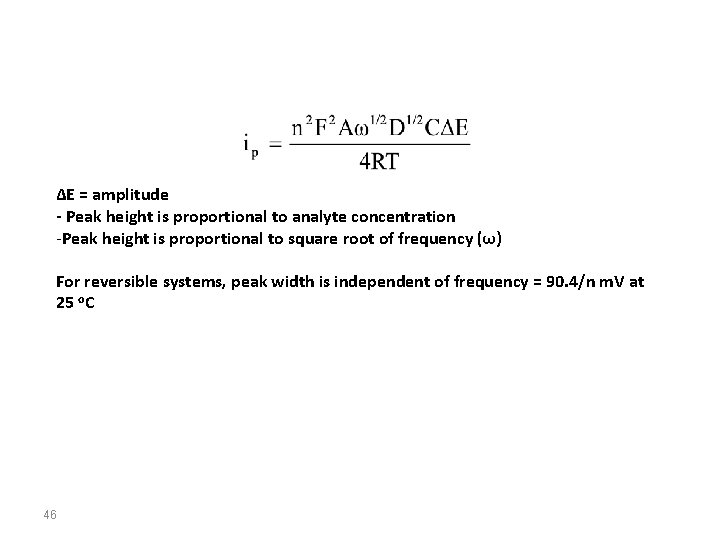
∆E = amplitude - Peak height is proportional to analyte concentration -Peak height is proportional to square root of frequency (ω) For reversible systems, peak width is independent of frequency = 90. 4/n m. V at 25 o. C 46