Two types of signal conduction within a single
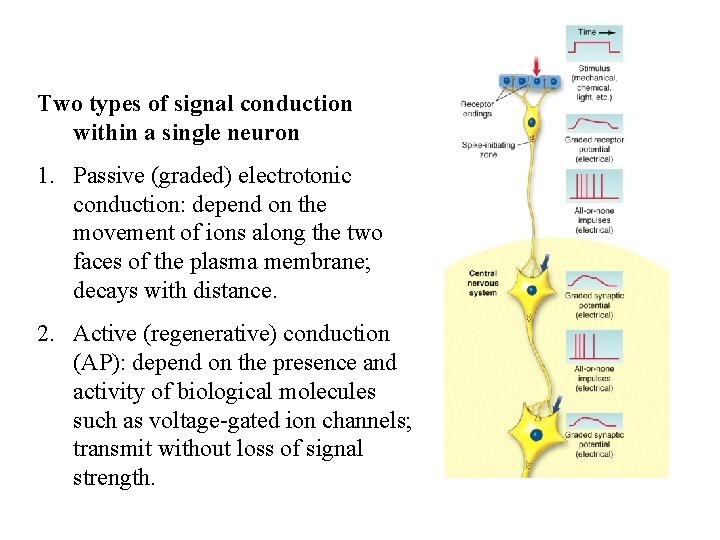
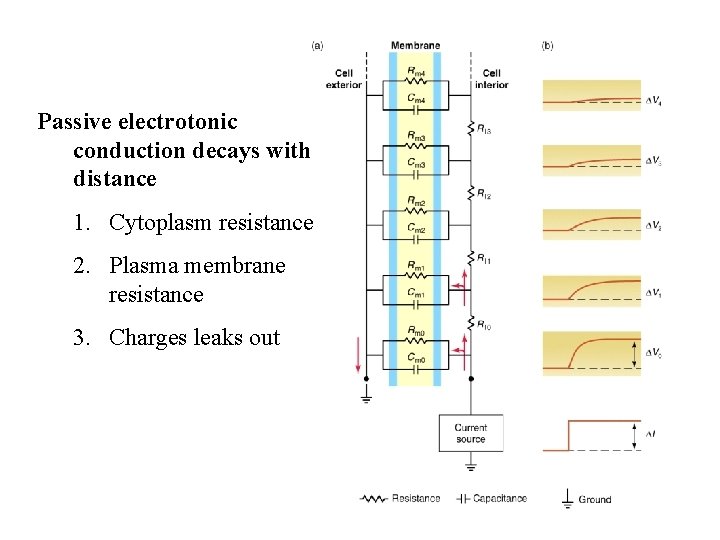
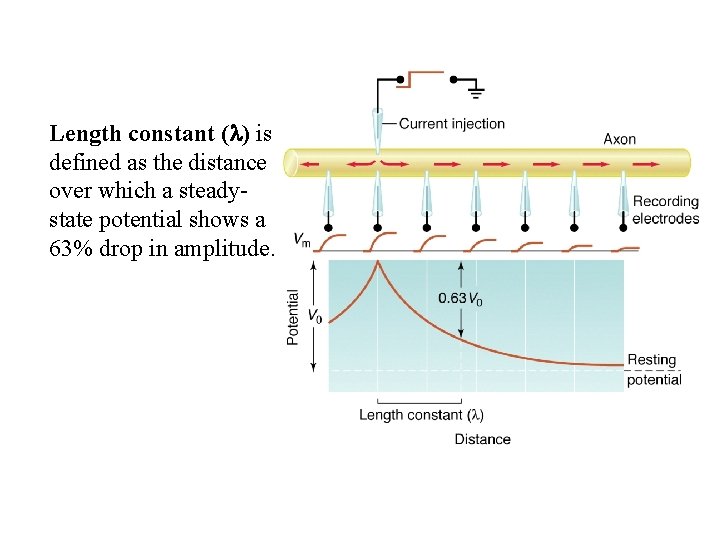
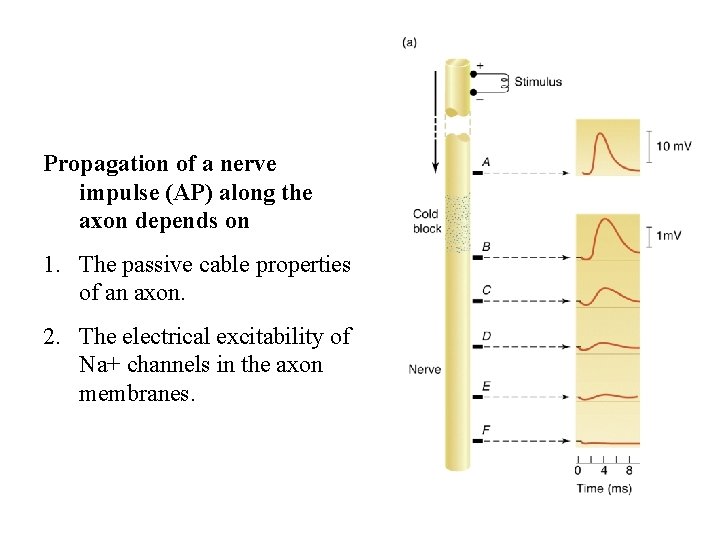
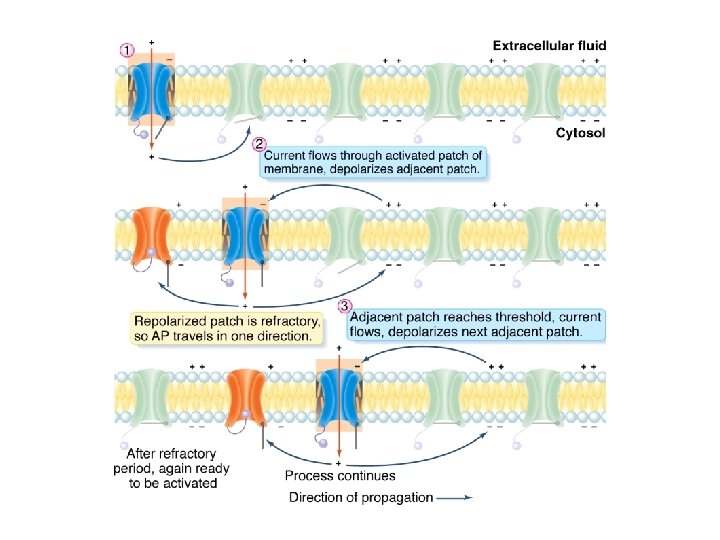
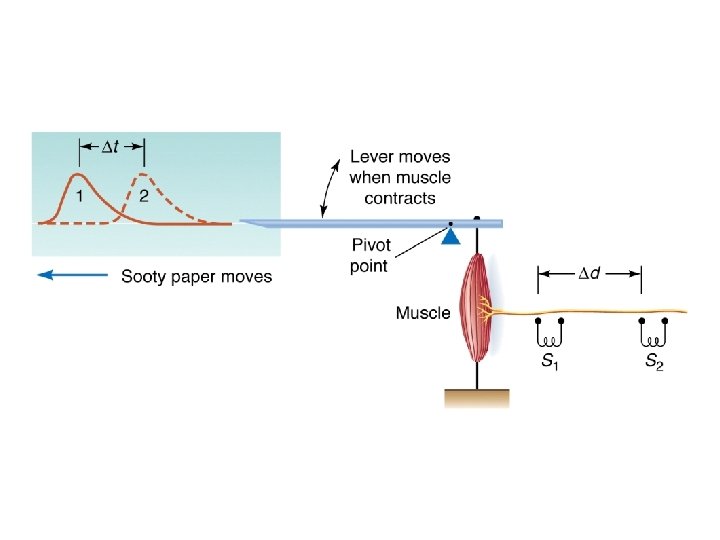
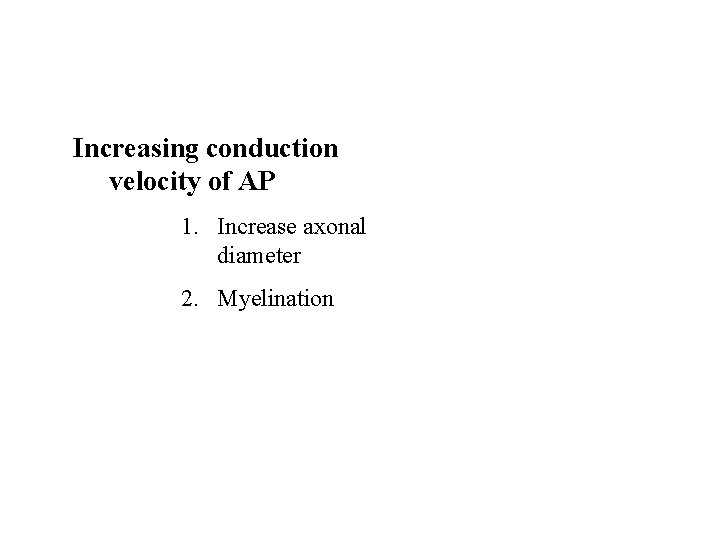
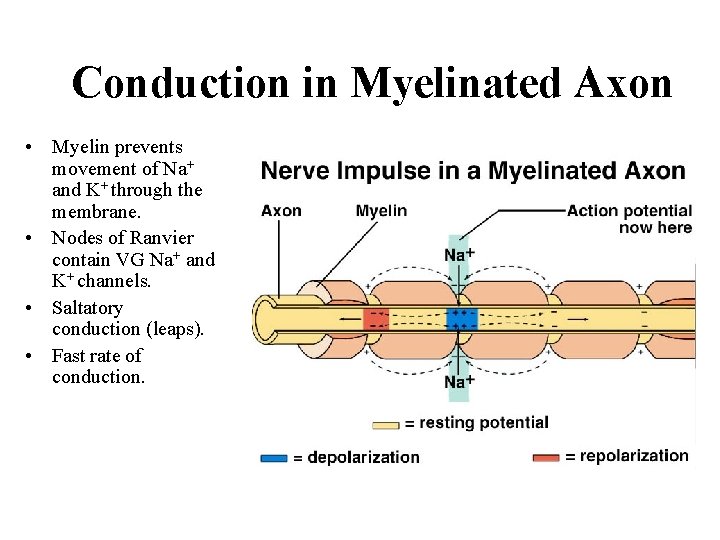
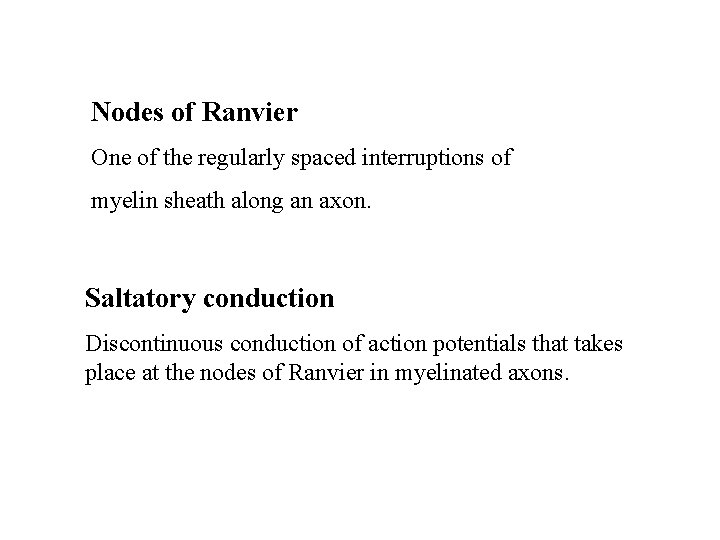
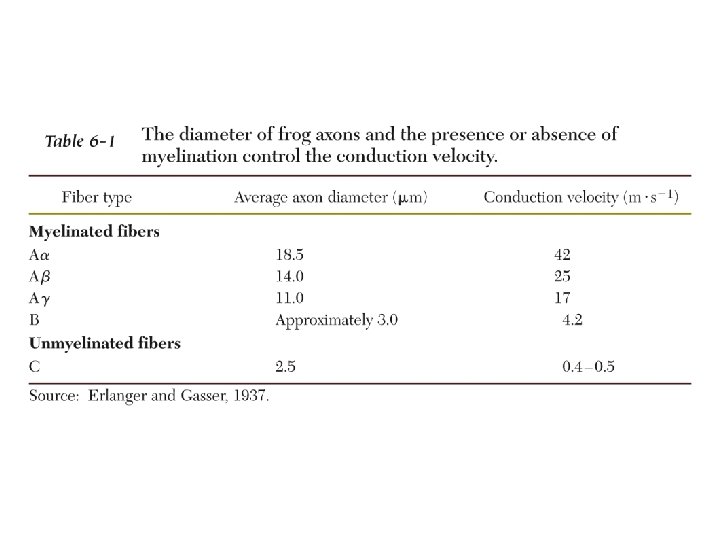
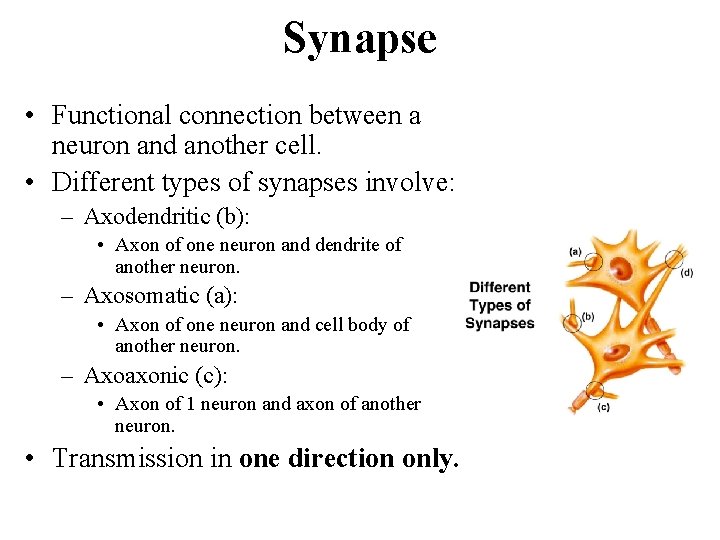
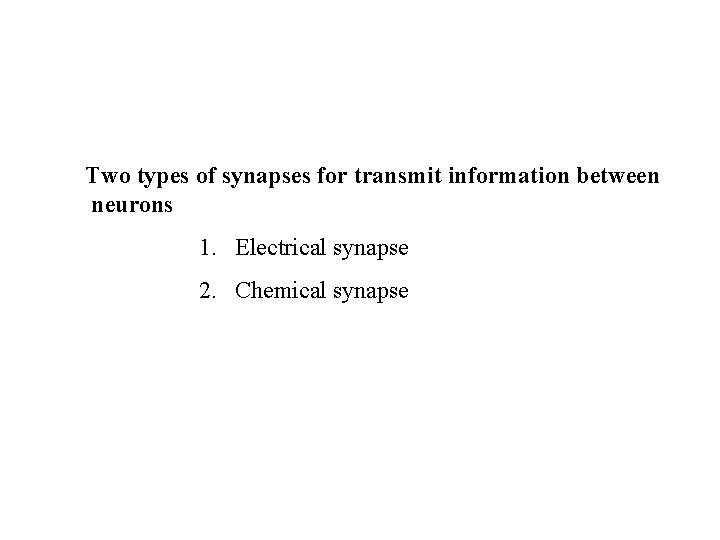
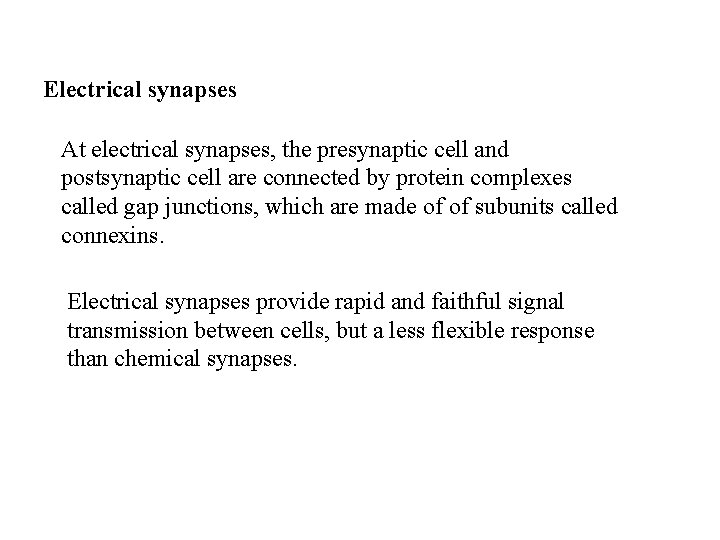
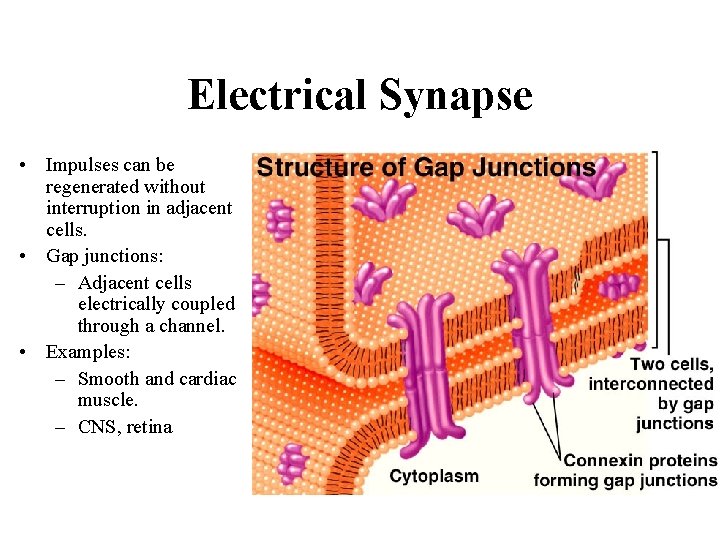
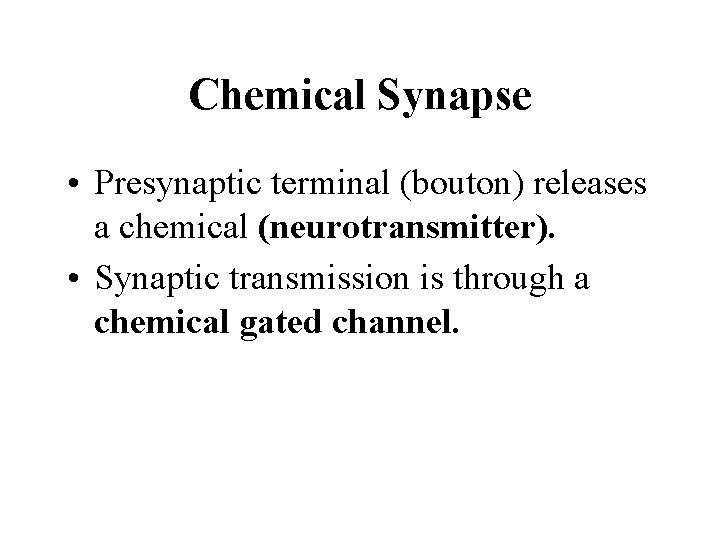
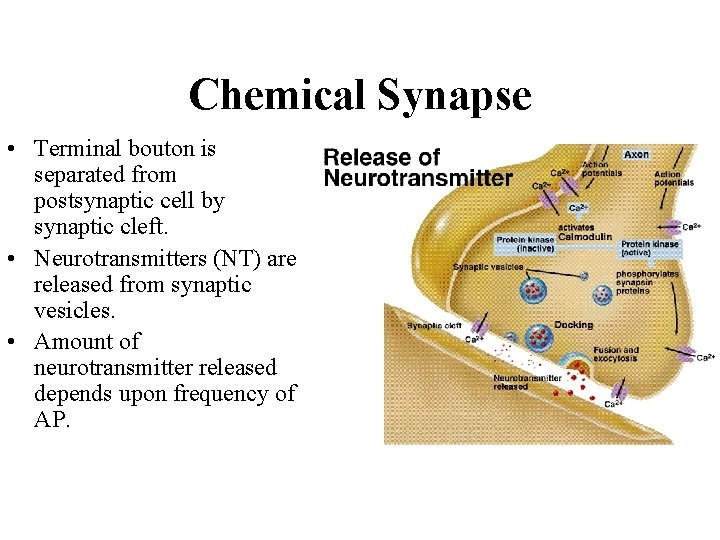
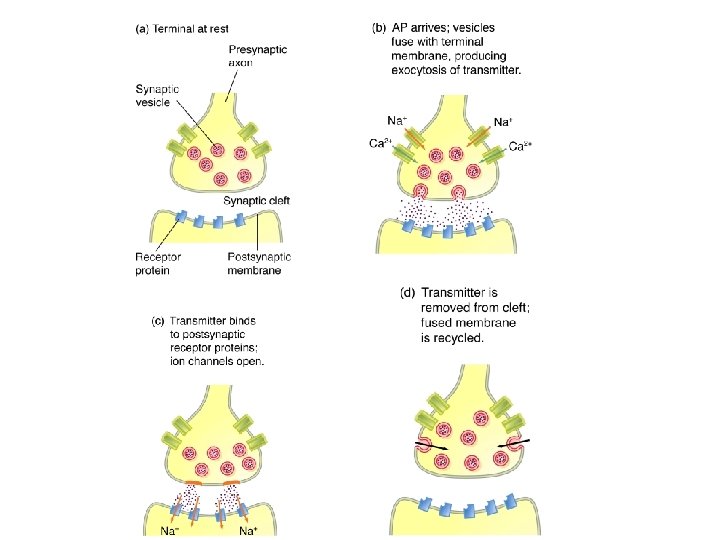
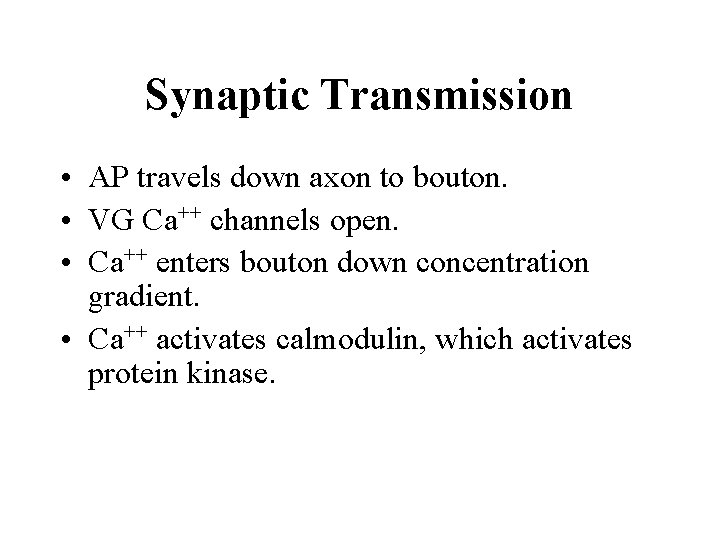
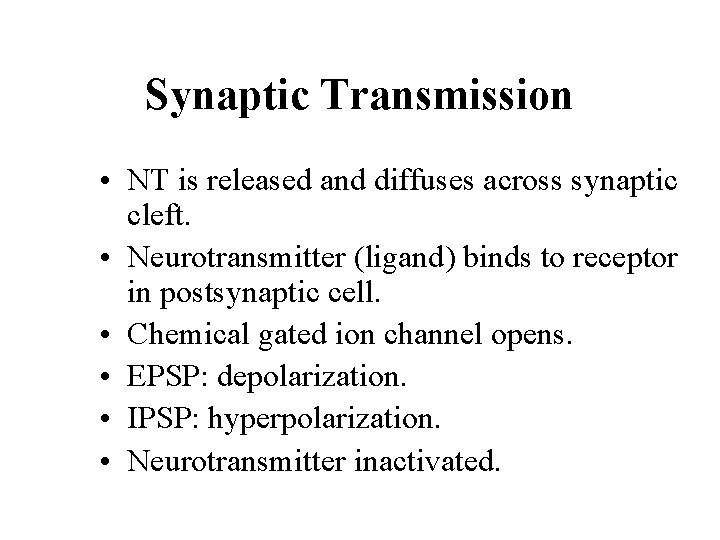
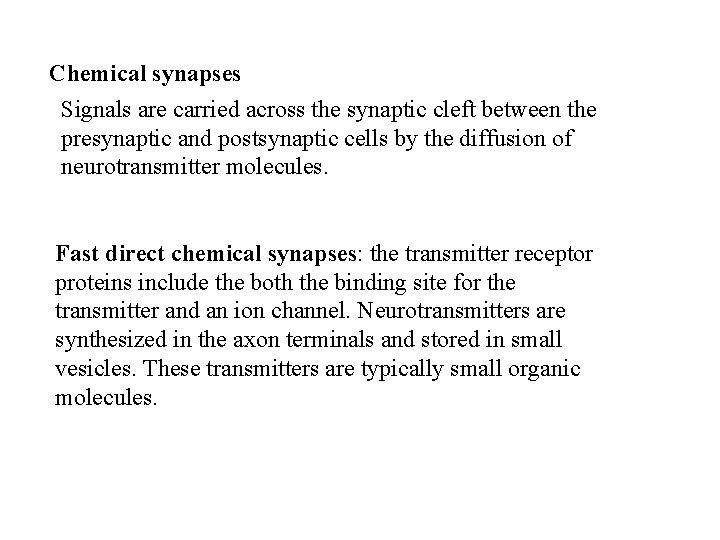
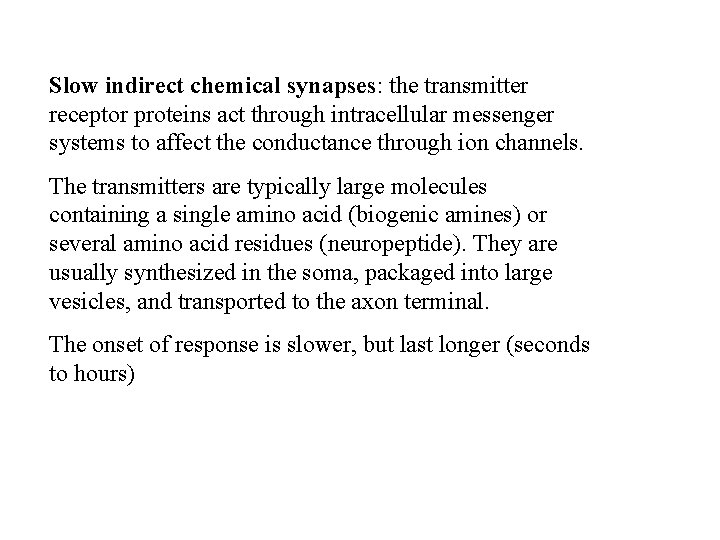
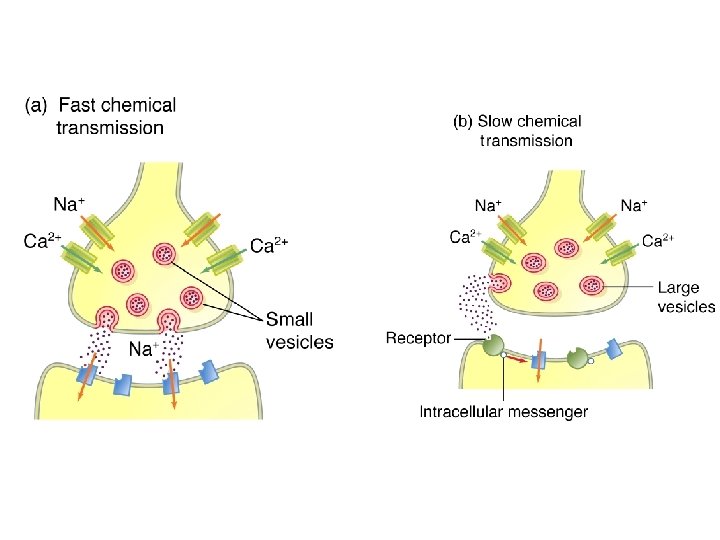
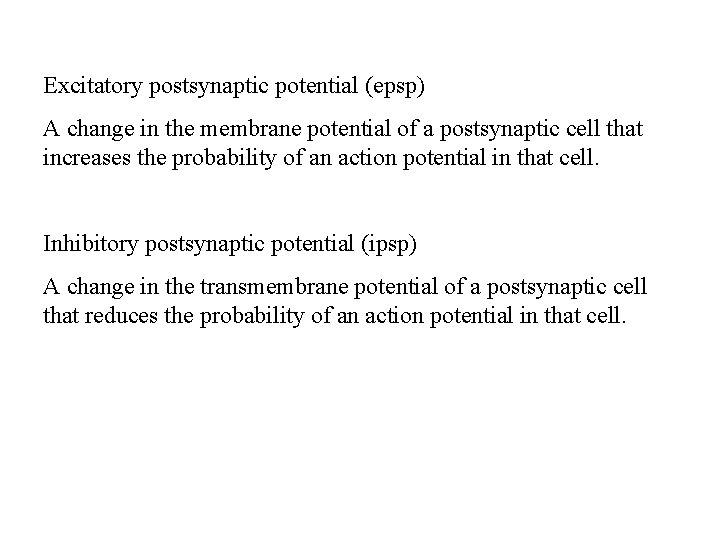
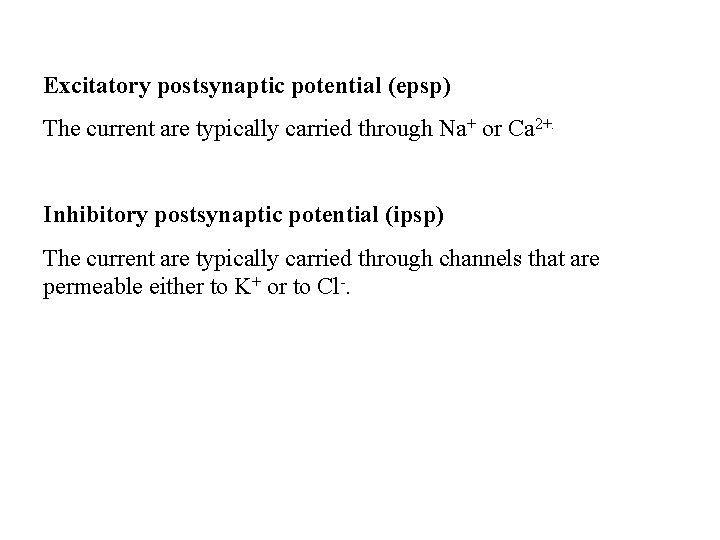
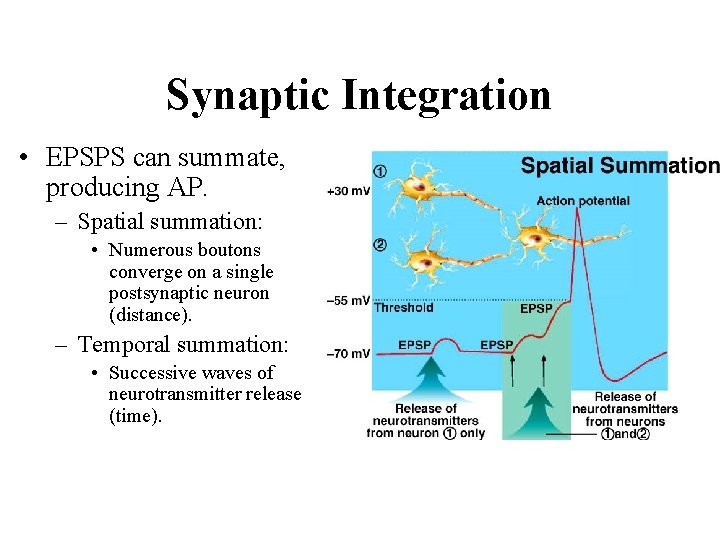
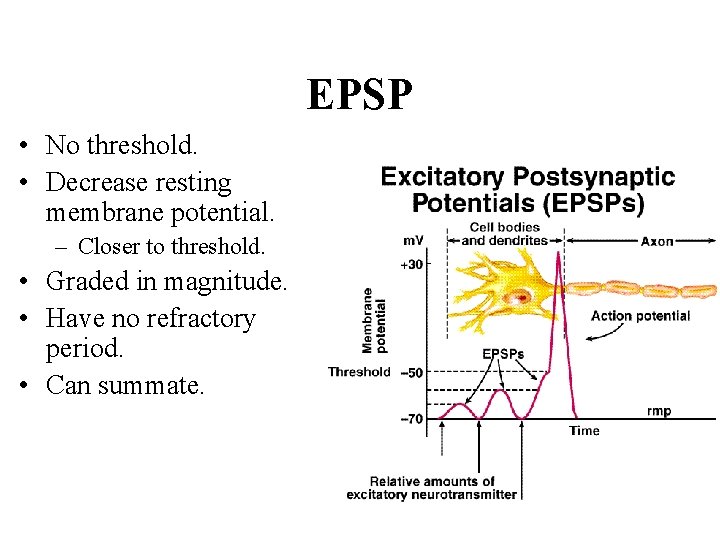
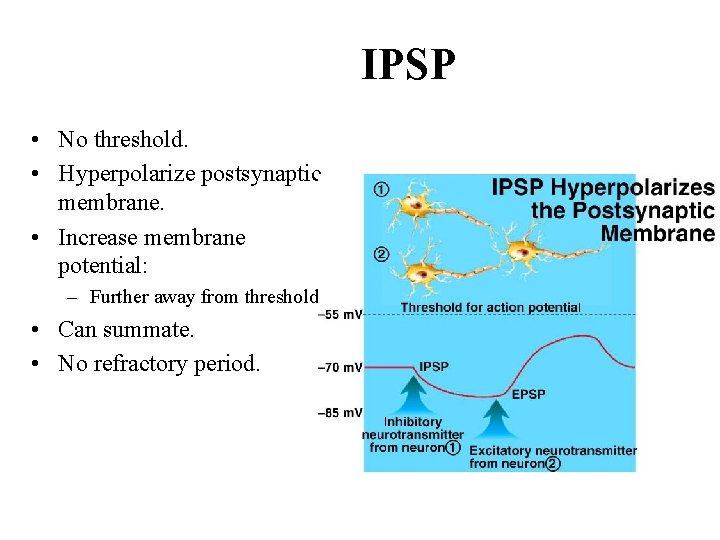
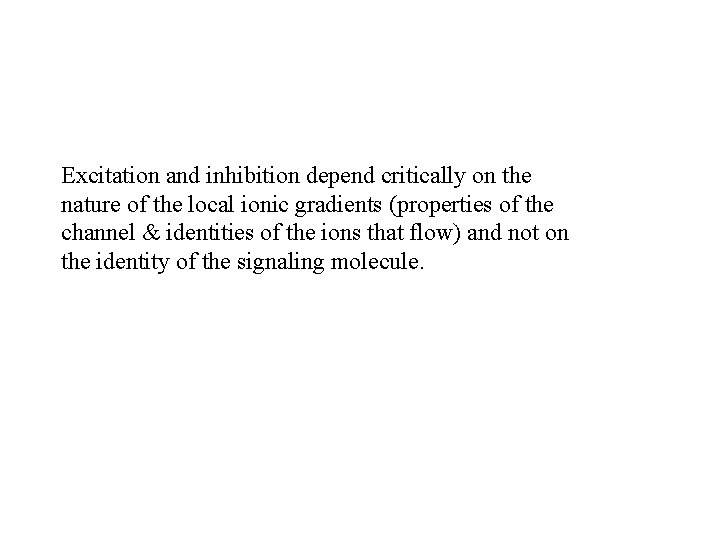
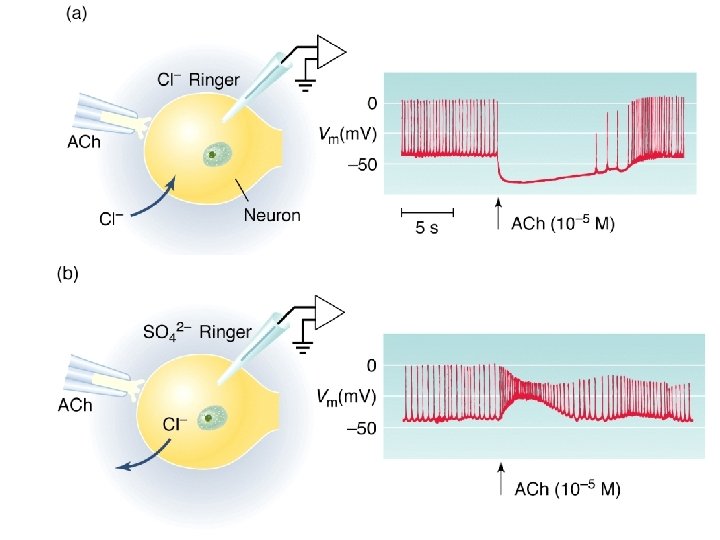
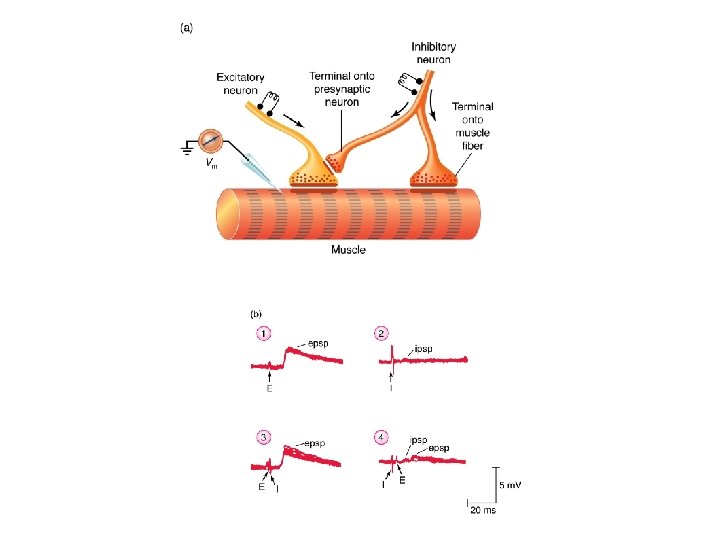
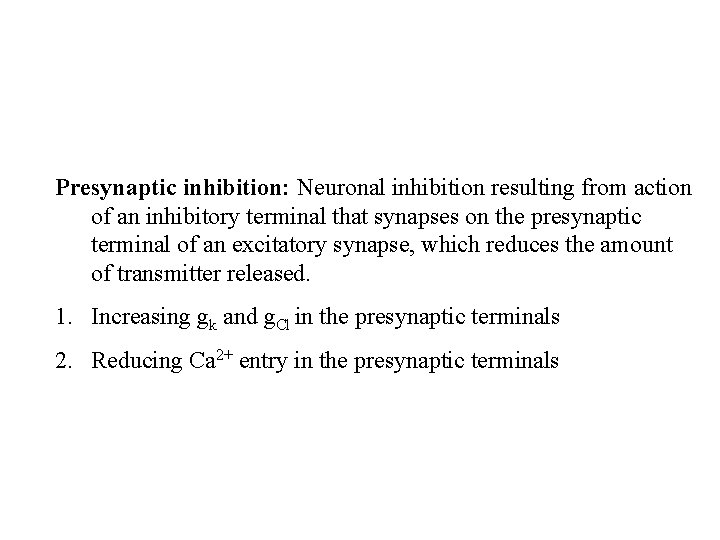
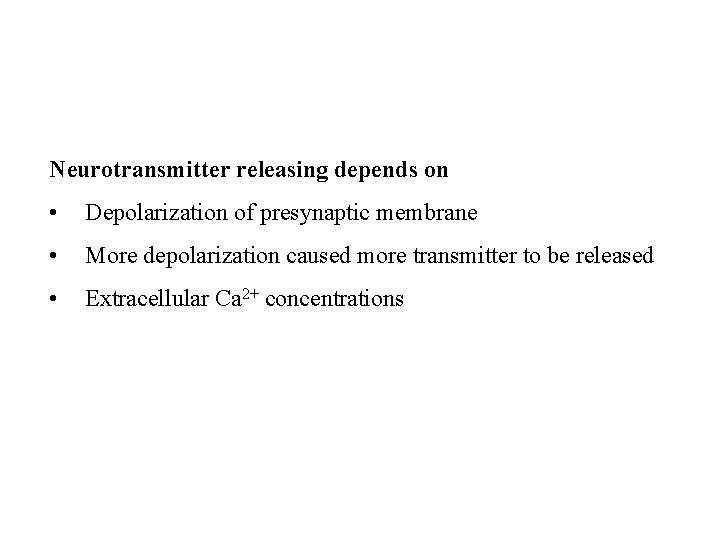
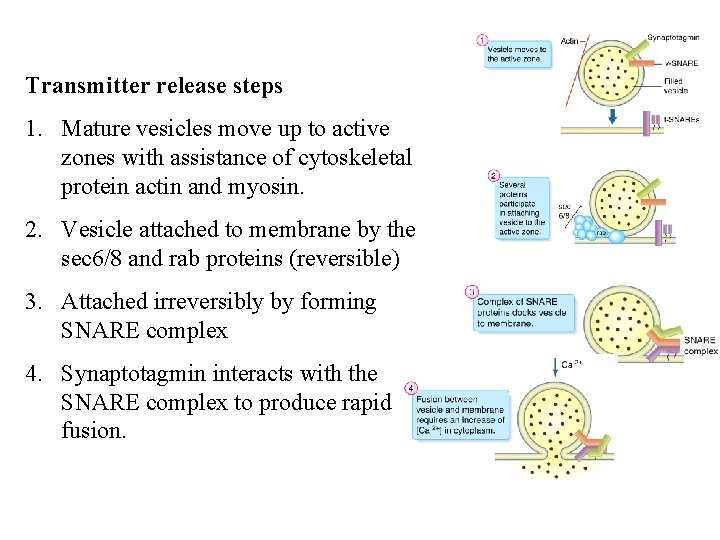
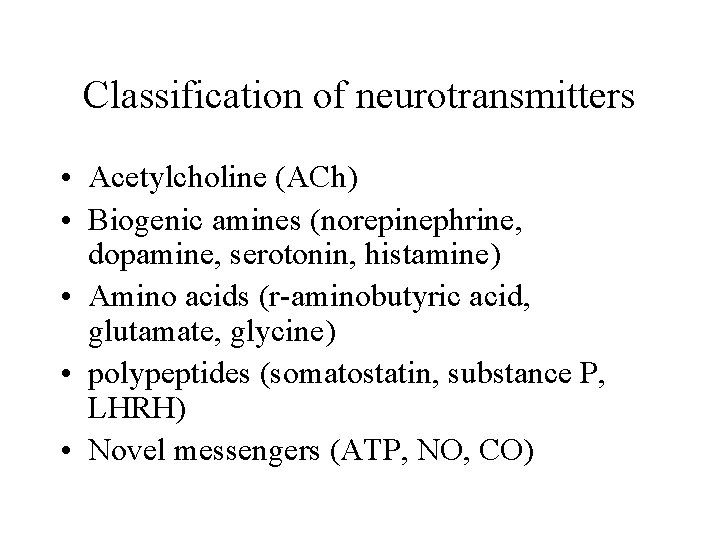
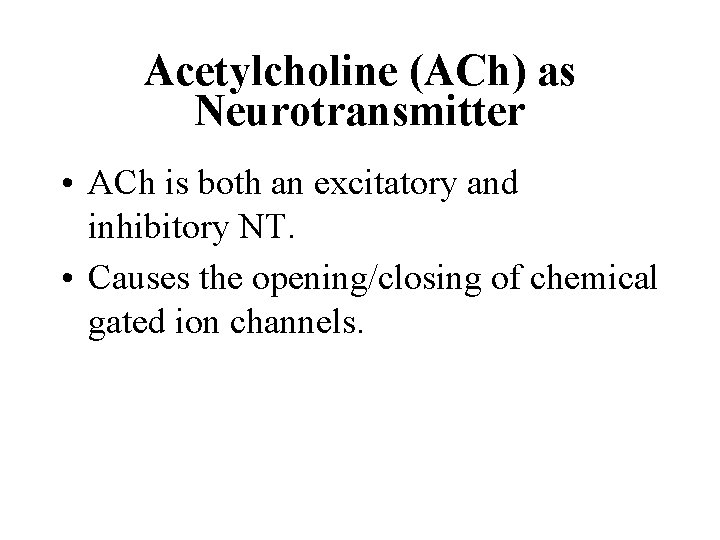
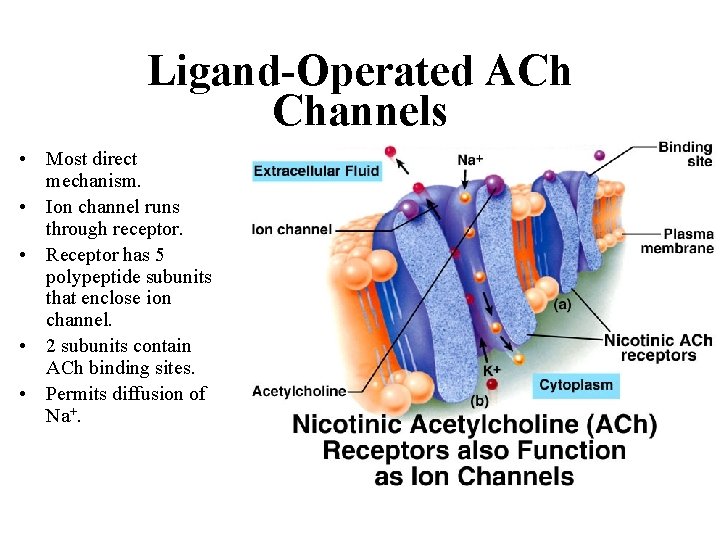
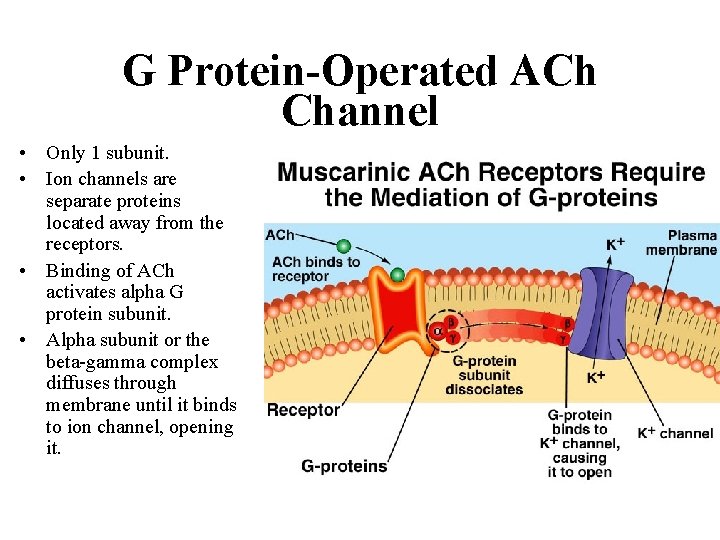
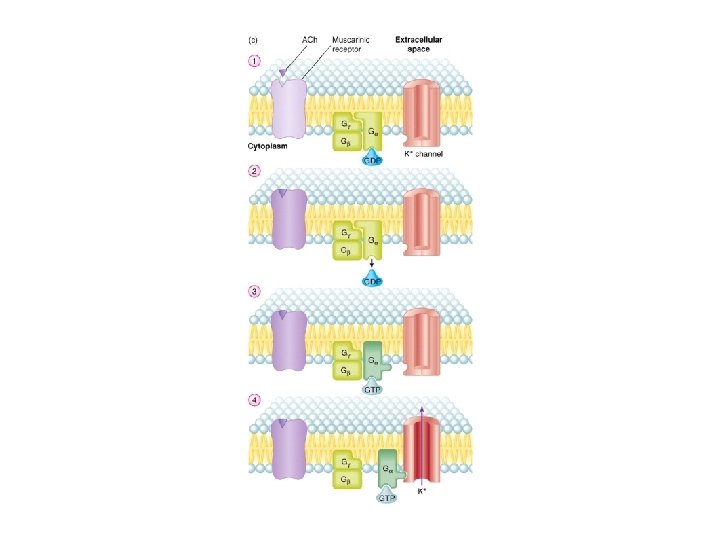
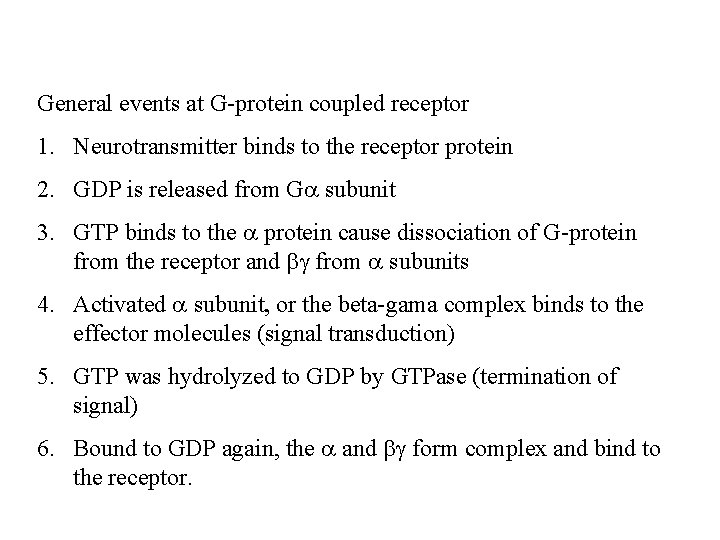
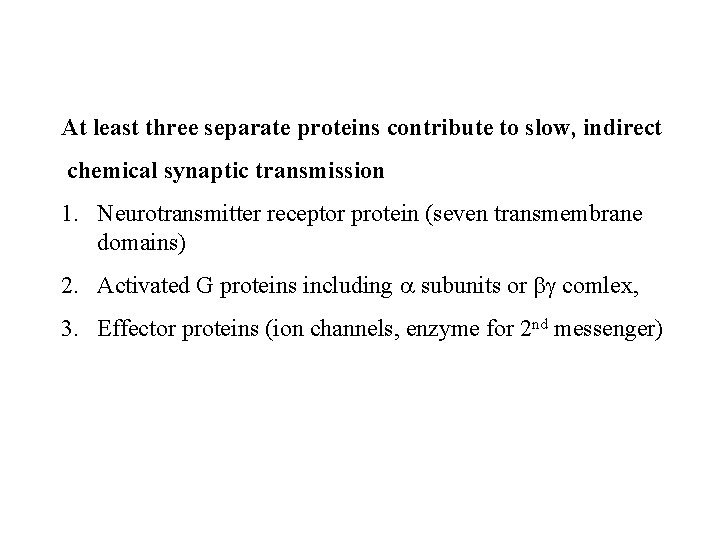
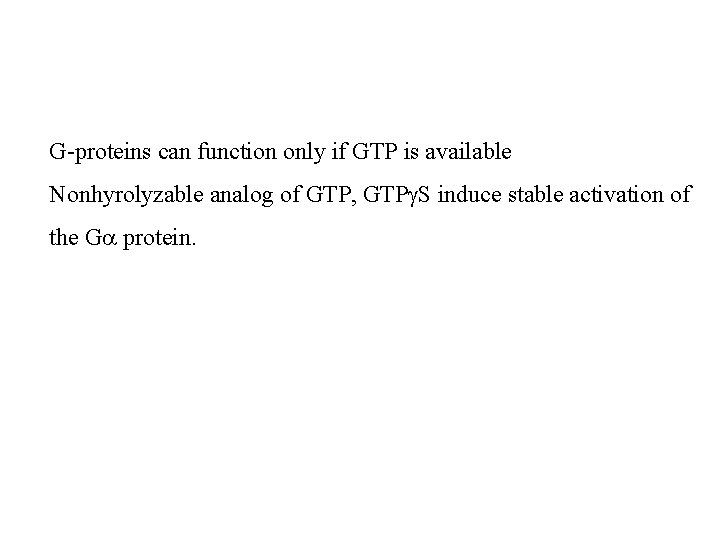
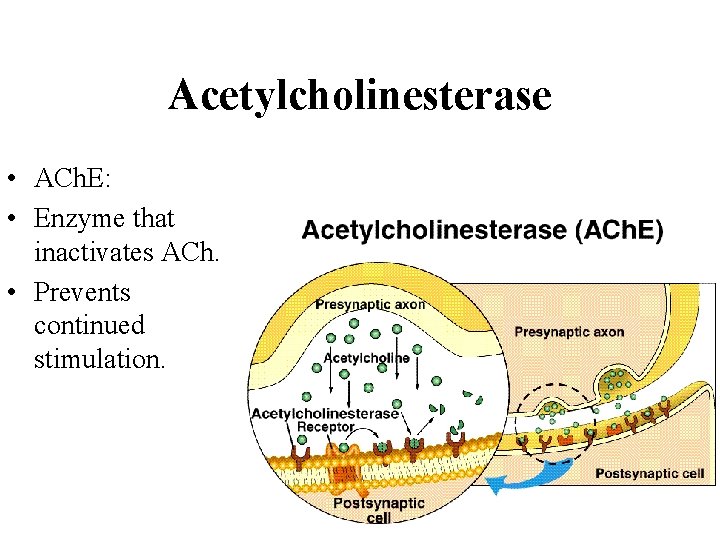
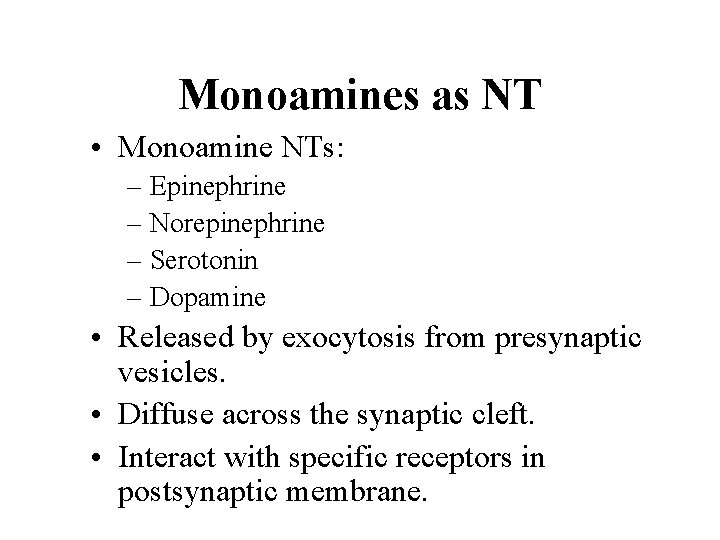
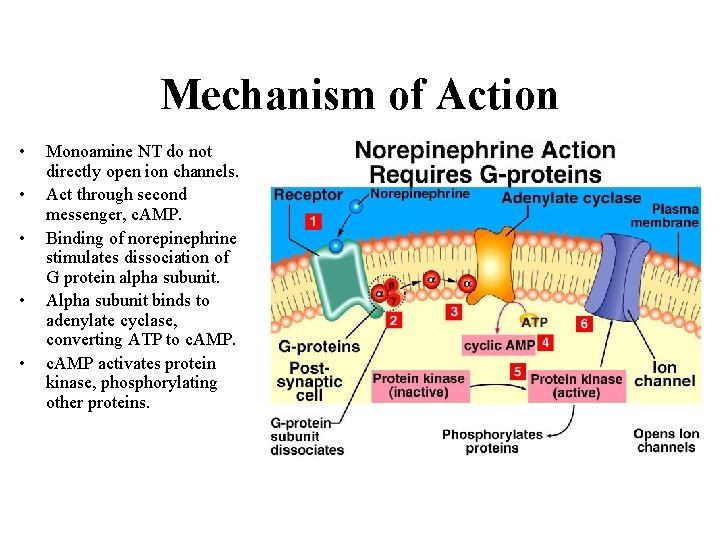
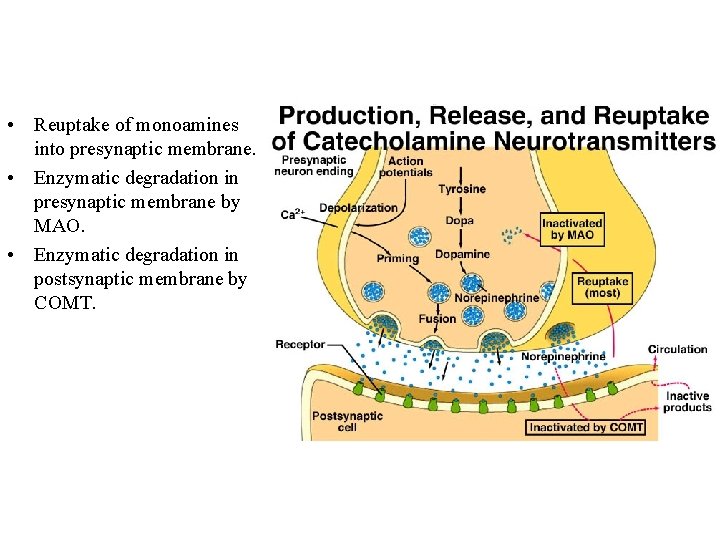
- Slides: 45
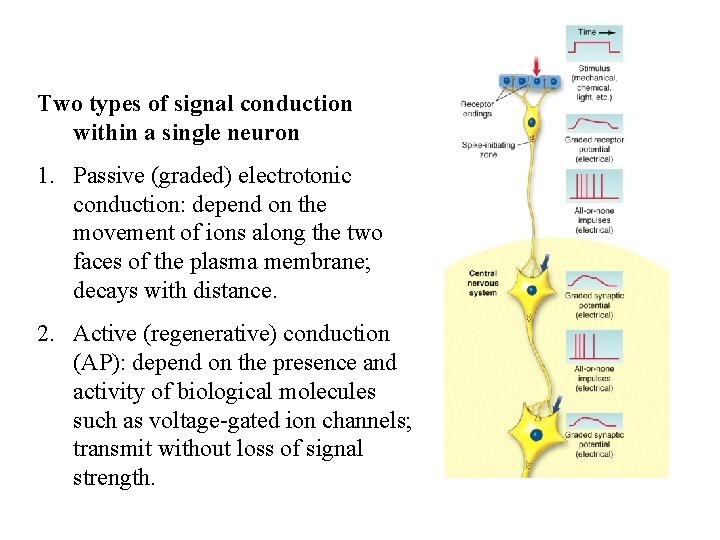
Two types of signal conduction within a single neuron 1. Passive (graded) electrotonic conduction: depend on the movement of ions along the two faces of the plasma membrane; decays with distance. 2. Active (regenerative) conduction (AP): depend on the presence and activity of biological molecules such as voltage-gated ion channels; transmit without loss of signal strength.
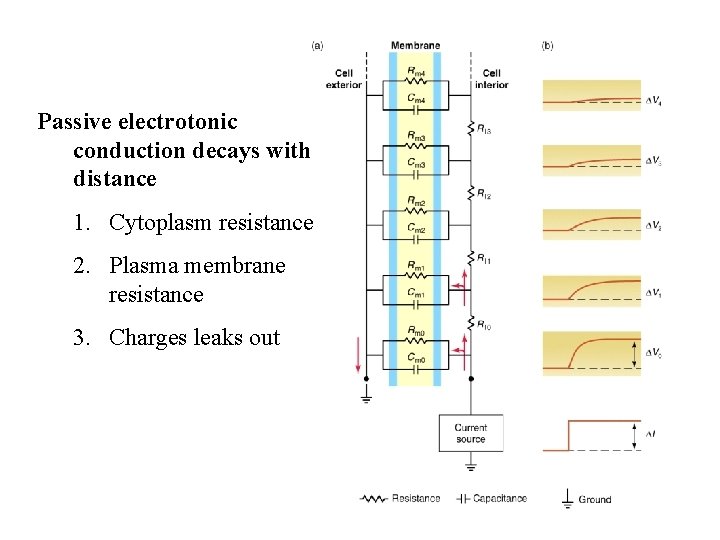
Passive electrotonic conduction decays with distance 1. Cytoplasm resistance 2. Plasma membrane resistance 3. Charges leaks out
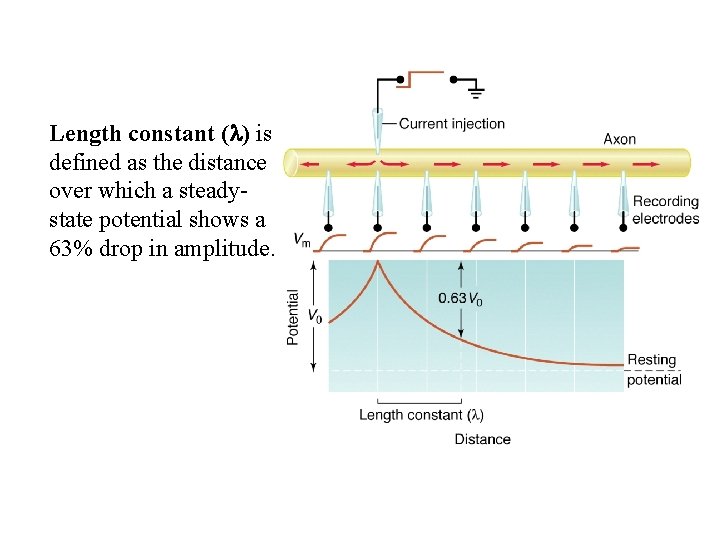
Length constant ( ) is defined as the distance over which a steadystate potential shows a 63% drop in amplitude.
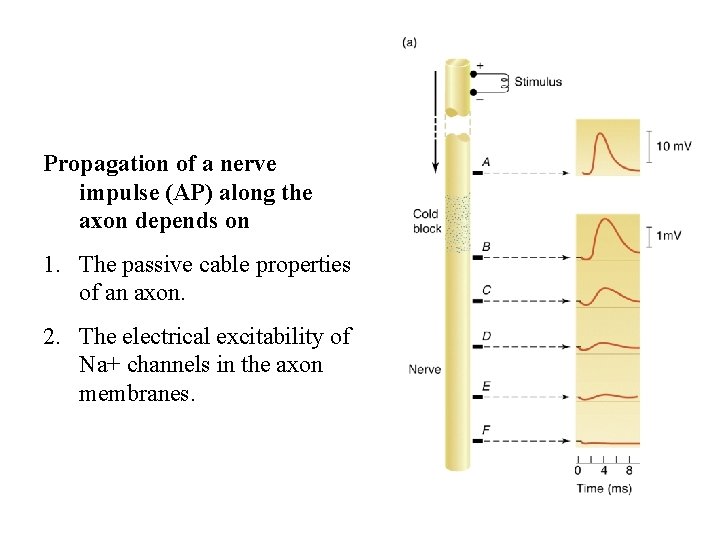
Propagation of a nerve impulse (AP) along the axon depends on 1. The passive cable properties of an axon. 2. The electrical excitability of Na+ channels in the axon membranes.
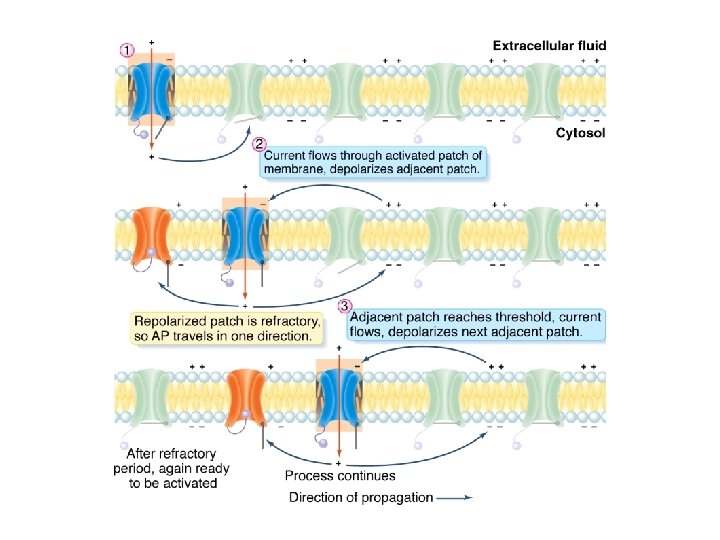
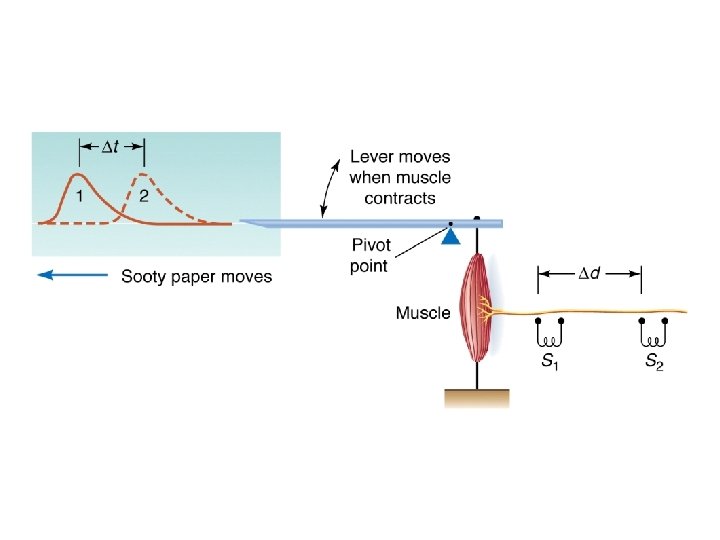
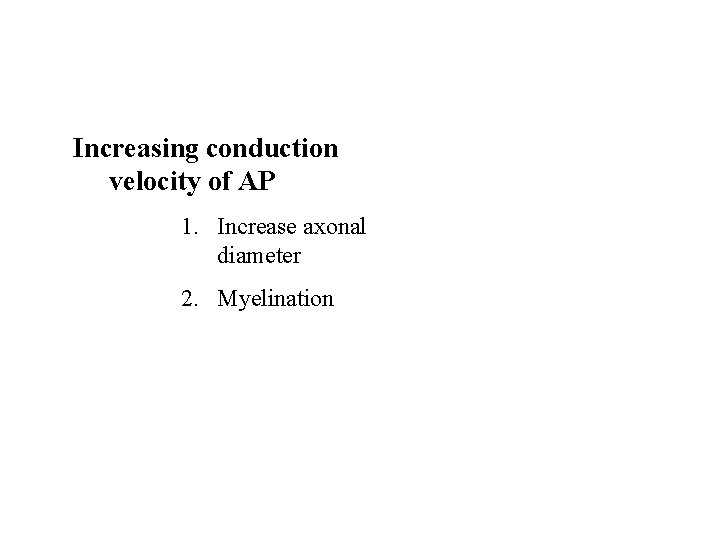
Increasing conduction velocity of AP 1. Increase axonal diameter 2. Myelination
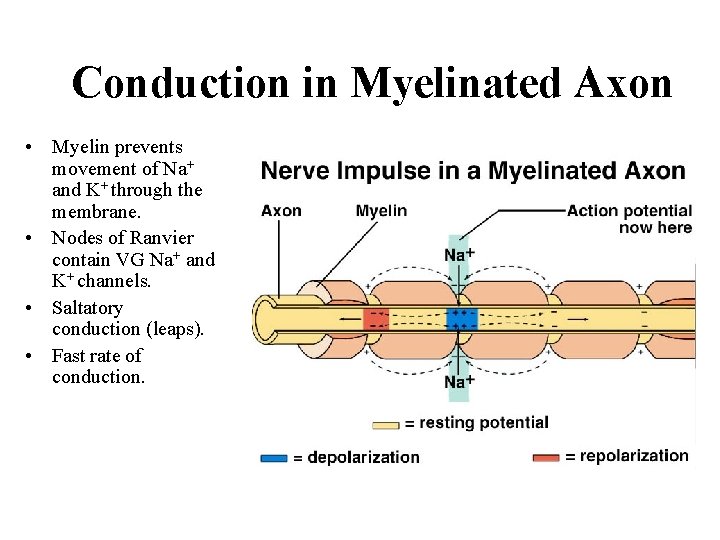
Conduction in Myelinated Axon • Myelin prevents movement of Na+ and K+ through the membrane. • Nodes of Ranvier contain VG Na+ and K+ channels. • Saltatory conduction (leaps). • Fast rate of conduction.
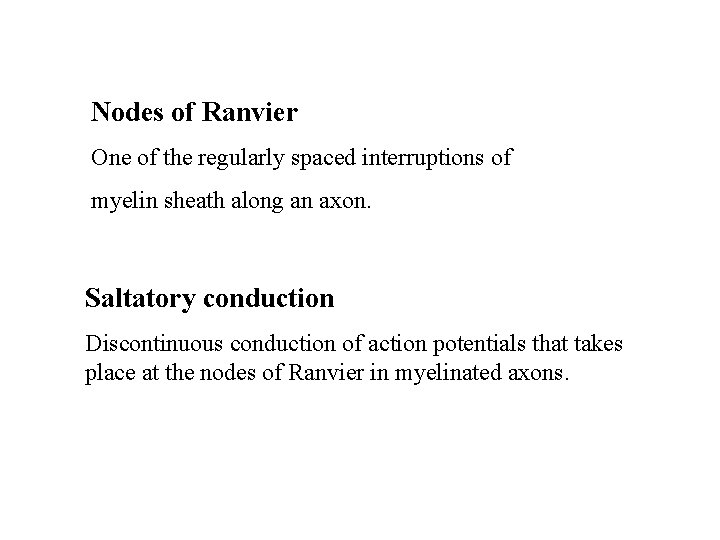
Nodes of Ranvier One of the regularly spaced interruptions of myelin sheath along an axon. Saltatory conduction Discontinuous conduction of action potentials that takes place at the nodes of Ranvier in myelinated axons.
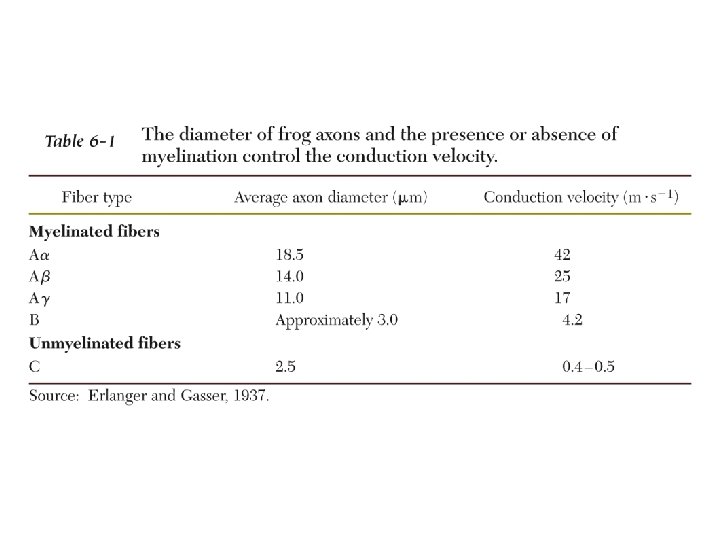
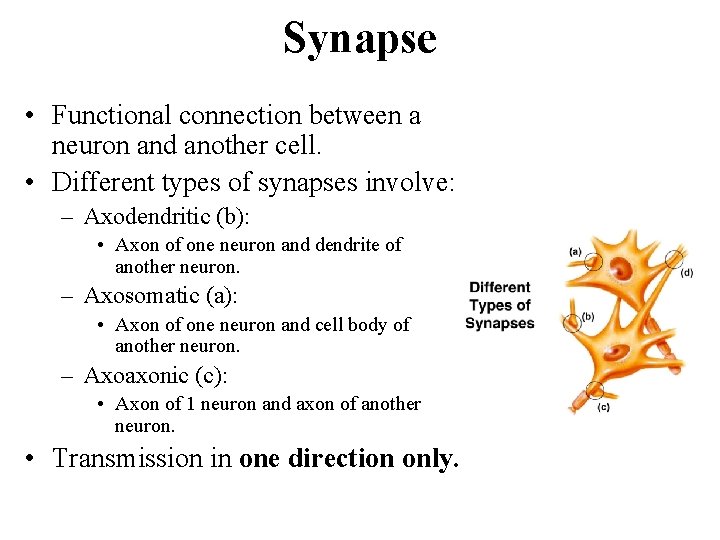
Synapse • Functional connection between a neuron and another cell. • Different types of synapses involve: – Axodendritic (b): • Axon of one neuron and dendrite of another neuron. – Axosomatic (a): • Axon of one neuron and cell body of another neuron. – Axoaxonic (c): • Axon of 1 neuron and axon of another neuron. • Transmission in one direction only.
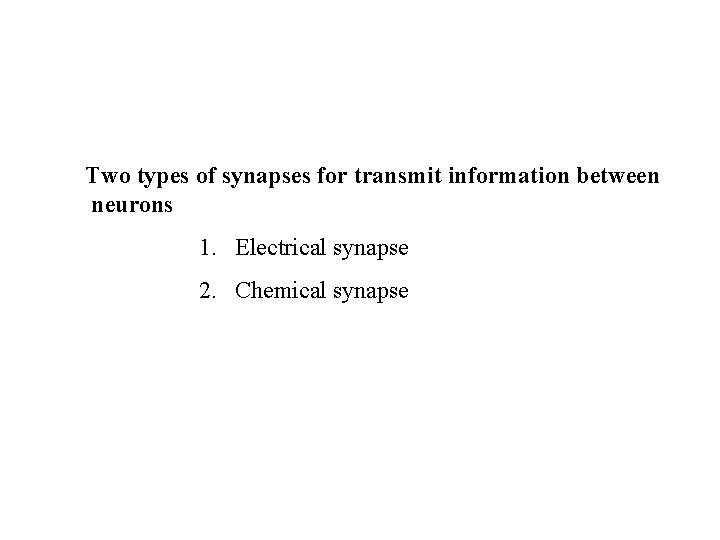
Two types of synapses for transmit information between neurons 1. Electrical synapse 2. Chemical synapse
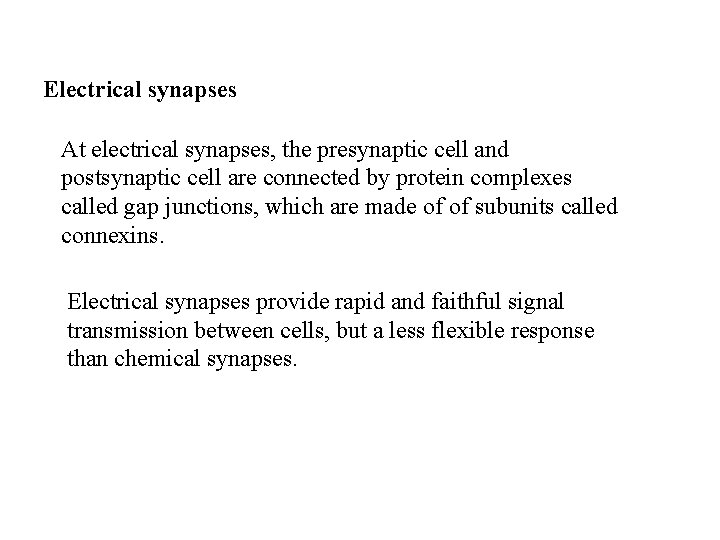
Electrical synapses At electrical synapses, the presynaptic cell and postsynaptic cell are connected by protein complexes called gap junctions, which are made of of subunits called connexins. Electrical synapses provide rapid and faithful signal transmission between cells, but a less flexible response than chemical synapses.
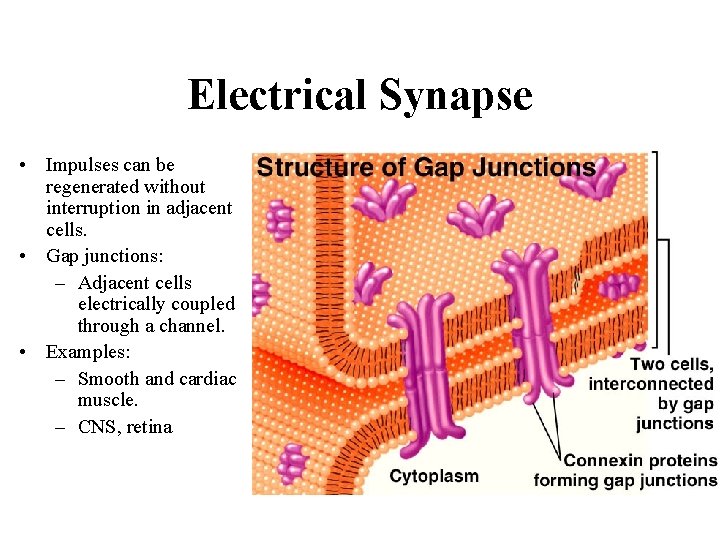
Electrical Synapse • Impulses can be regenerated without interruption in adjacent cells. • Gap junctions: – Adjacent cells electrically coupled through a channel. • Examples: – Smooth and cardiac muscle. – CNS, retina
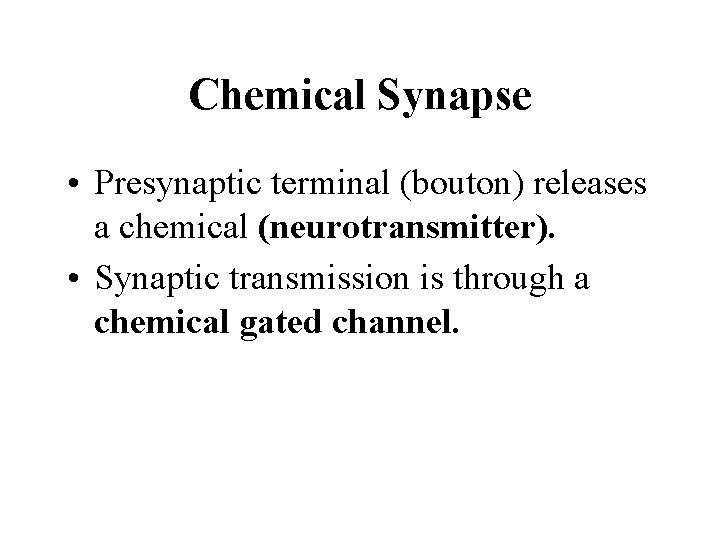
Chemical Synapse • Presynaptic terminal (bouton) releases a chemical (neurotransmitter). • Synaptic transmission is through a chemical gated channel.
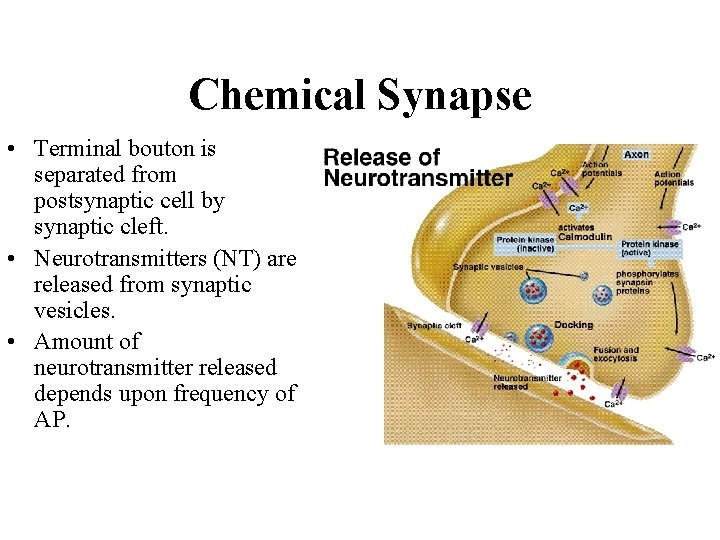
Chemical Synapse • Terminal bouton is separated from postsynaptic cell by synaptic cleft. • Neurotransmitters (NT) are released from synaptic vesicles. • Amount of neurotransmitter released depends upon frequency of AP.
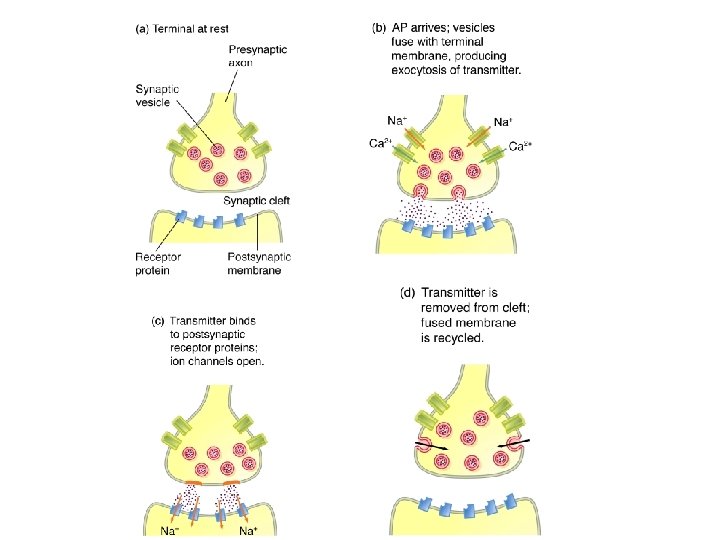
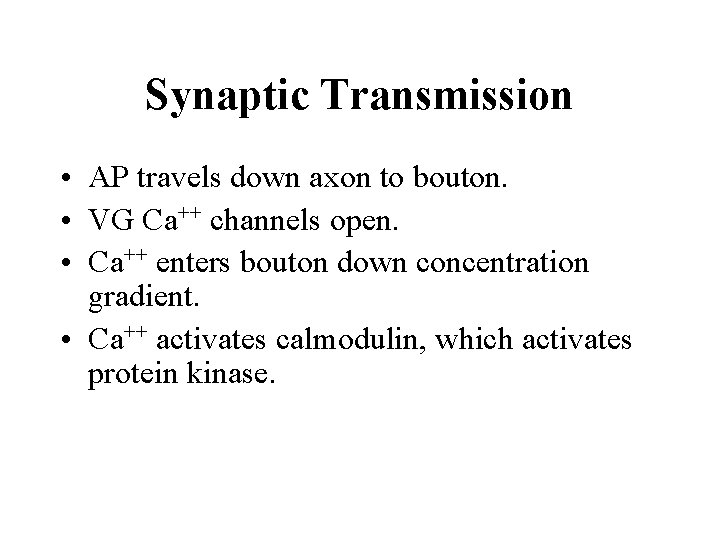
Synaptic Transmission • AP travels down axon to bouton. • VG Ca++ channels open. • Ca++ enters bouton down concentration gradient. • Ca++ activates calmodulin, which activates protein kinase.
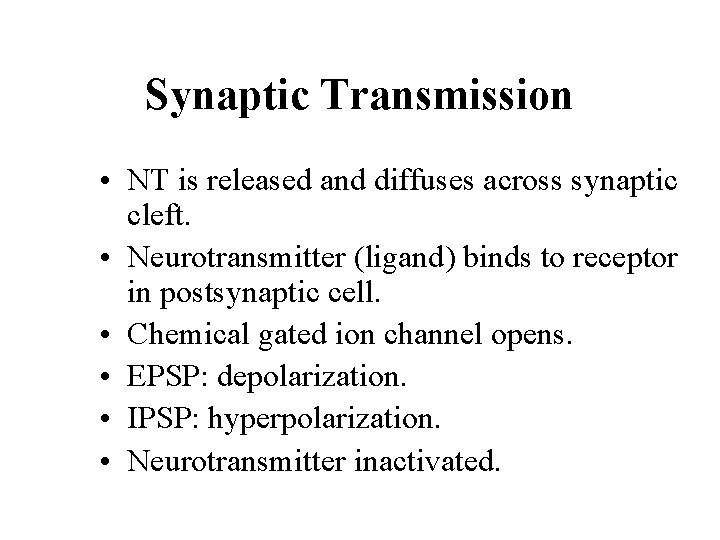
Synaptic Transmission • NT is released and diffuses across synaptic cleft. • Neurotransmitter (ligand) binds to receptor in postsynaptic cell. • Chemical gated ion channel opens. • EPSP: depolarization. • IPSP: hyperpolarization. • Neurotransmitter inactivated.
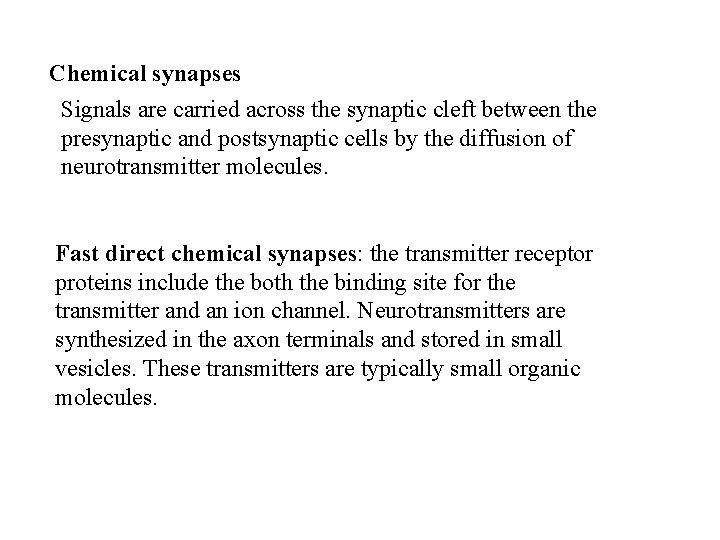
Chemical synapses Signals are carried across the synaptic cleft between the presynaptic and postsynaptic cells by the diffusion of neurotransmitter molecules. Fast direct chemical synapses: the transmitter receptor proteins include the both the binding site for the transmitter and an ion channel. Neurotransmitters are synthesized in the axon terminals and stored in small vesicles. These transmitters are typically small organic molecules.
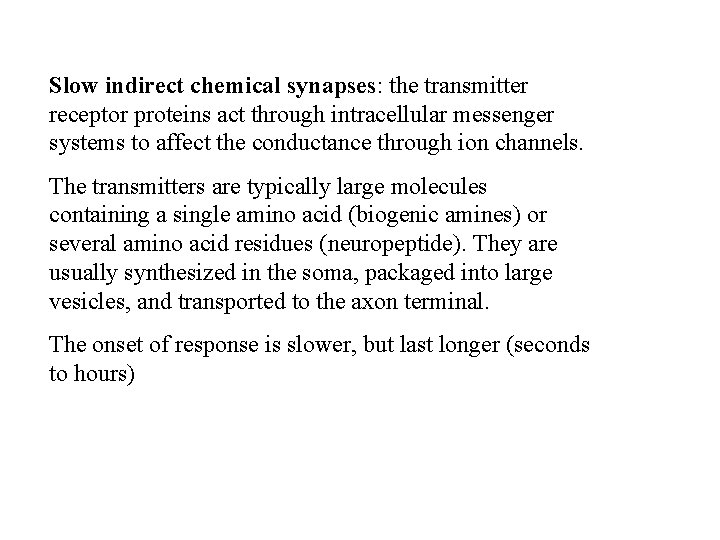
Slow indirect chemical synapses: the transmitter receptor proteins act through intracellular messenger systems to affect the conductance through ion channels. The transmitters are typically large molecules containing a single amino acid (biogenic amines) or several amino acid residues (neuropeptide). They are usually synthesized in the soma, packaged into large vesicles, and transported to the axon terminal. The onset of response is slower, but last longer (seconds to hours)
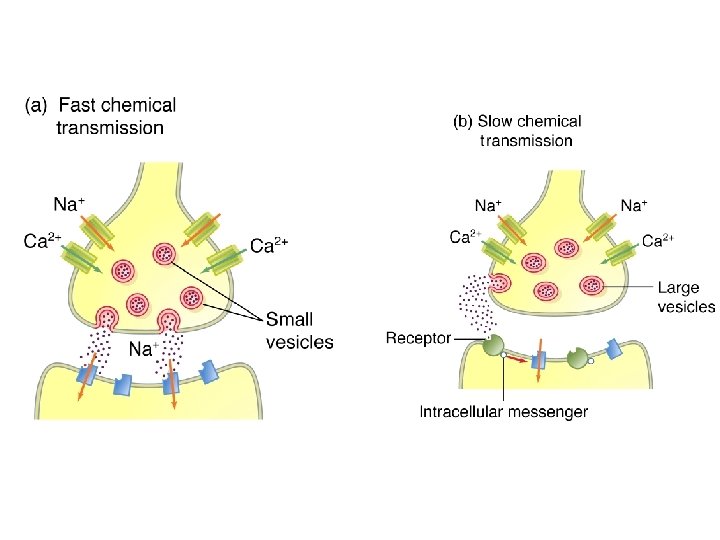
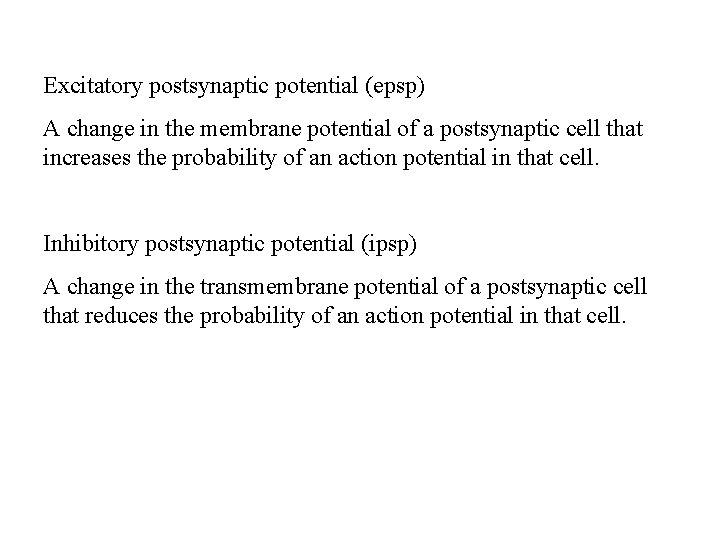
Excitatory postsynaptic potential (epsp) A change in the membrane potential of a postsynaptic cell that increases the probability of an action potential in that cell. Inhibitory postsynaptic potential (ipsp) A change in the transmembrane potential of a postsynaptic cell that reduces the probability of an action potential in that cell.
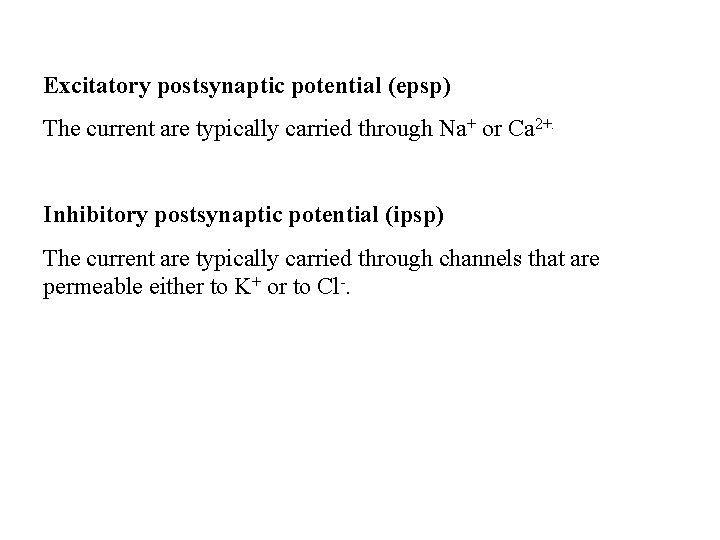
Excitatory postsynaptic potential (epsp) The current are typically carried through Na+ or Ca 2+. Inhibitory postsynaptic potential (ipsp) The current are typically carried through channels that are permeable either to K+ or to Cl-.
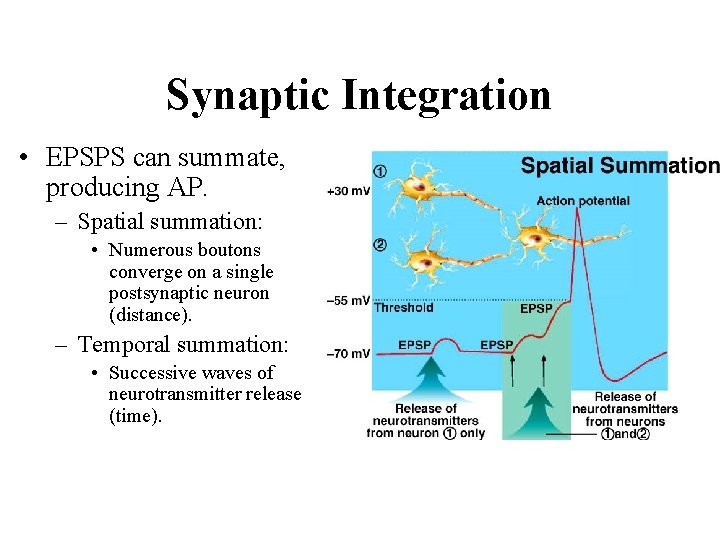
Synaptic Integration • EPSPS can summate, producing AP. – Spatial summation: • Numerous boutons converge on a single postsynaptic neuron (distance). – Temporal summation: • Successive waves of neurotransmitter release (time).
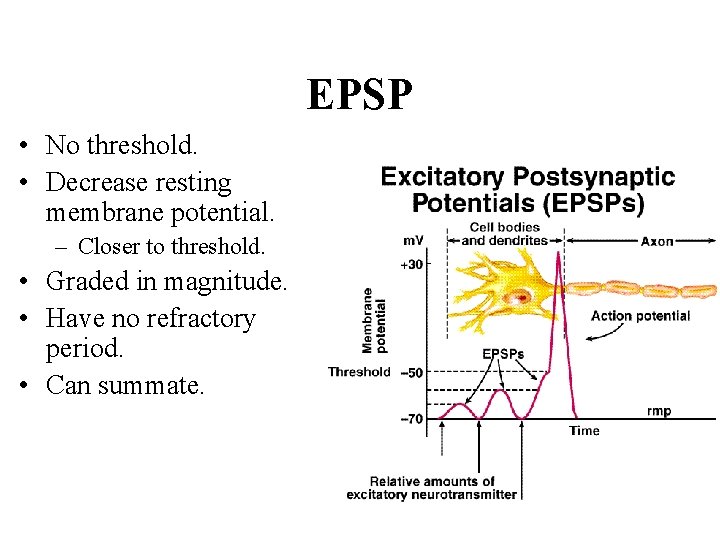
EPSP • No threshold. • Decrease resting membrane potential. – Closer to threshold. • Graded in magnitude. • Have no refractory period. • Can summate.
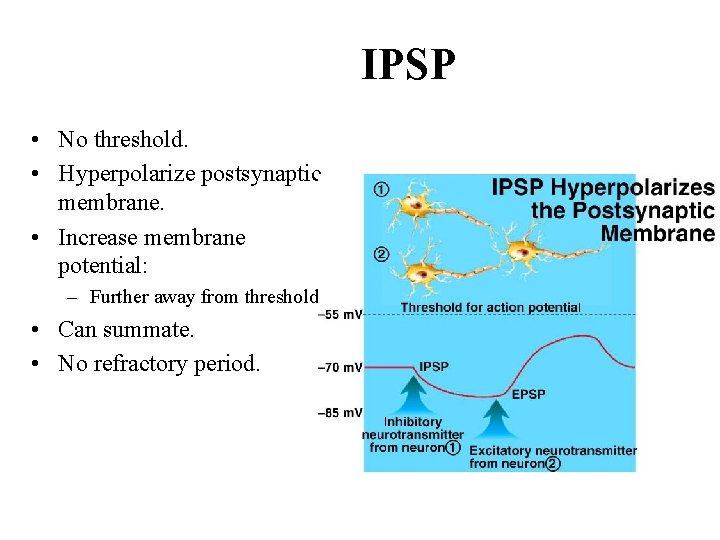
IPSP • No threshold. • Hyperpolarize postsynaptic membrane. • Increase membrane potential: – Further away from threshold. • Can summate. • No refractory period.
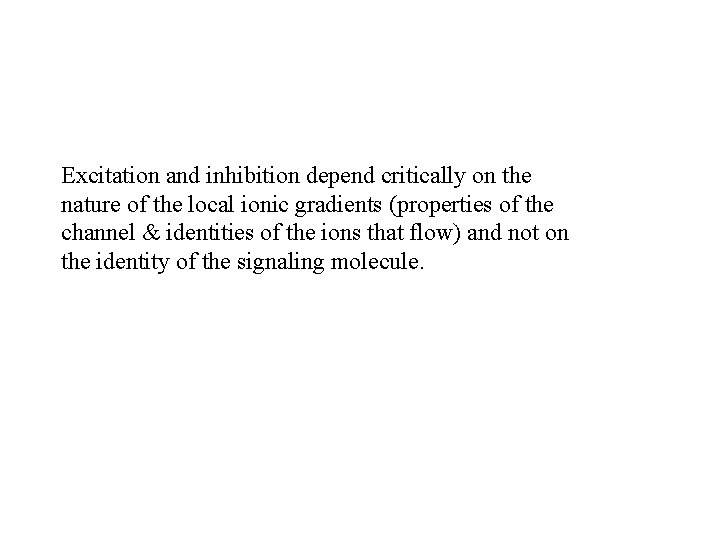
Excitation and inhibition depend critically on the nature of the local ionic gradients (properties of the channel & identities of the ions that flow) and not on the identity of the signaling molecule.
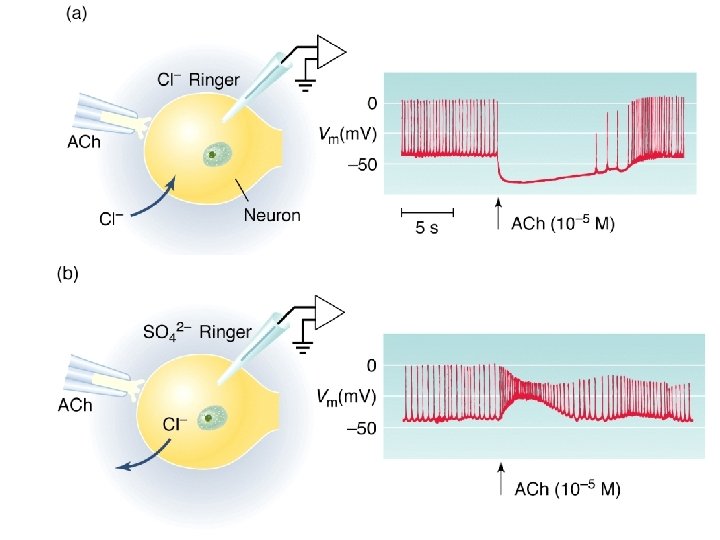
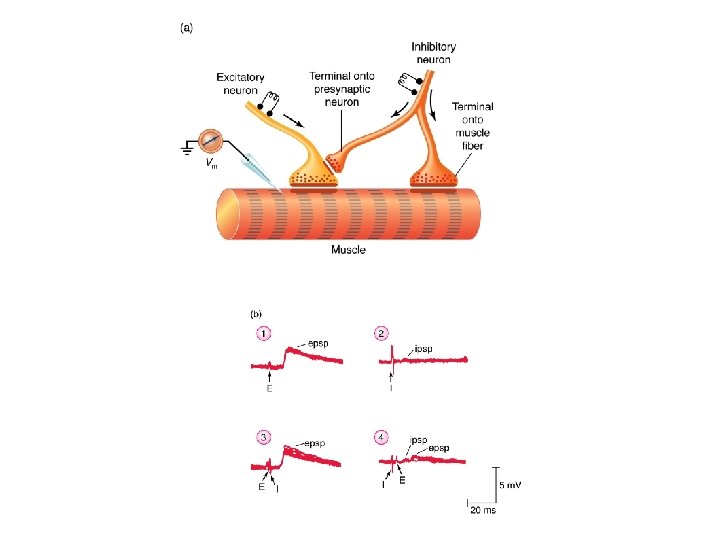
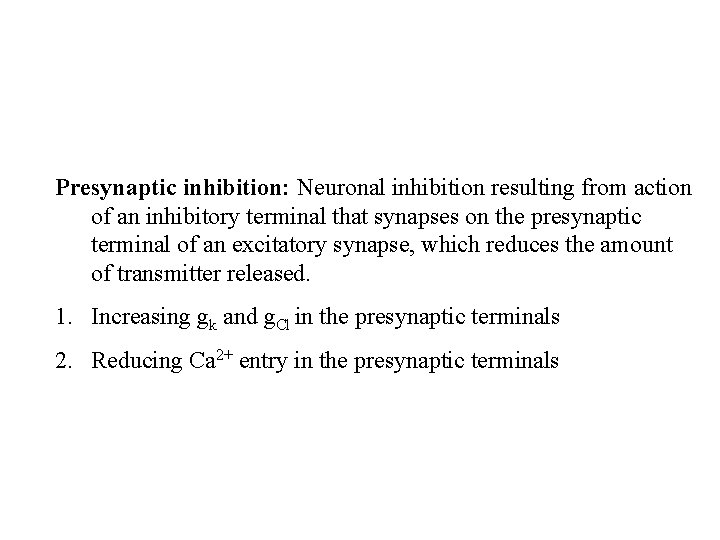
Presynaptic inhibition: Neuronal inhibition resulting from action of an inhibitory terminal that synapses on the presynaptic terminal of an excitatory synapse, which reduces the amount of transmitter released. 1. Increasing gk and g. Cl in the presynaptic terminals 2. Reducing Ca 2+ entry in the presynaptic terminals
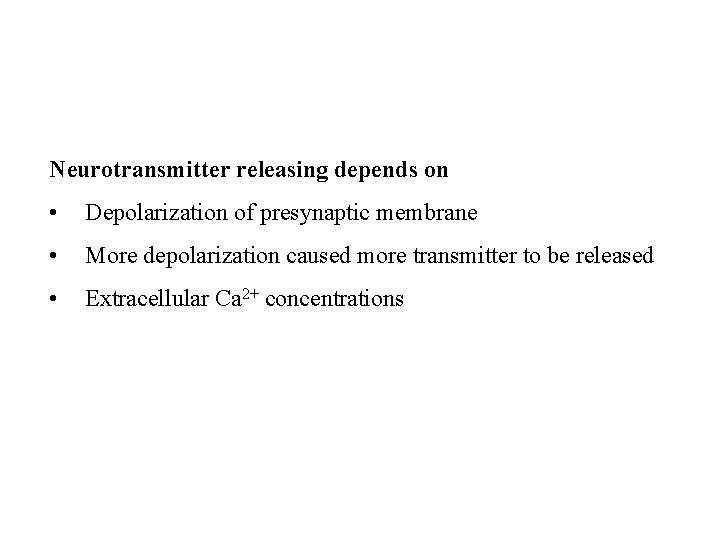
Neurotransmitter releasing depends on • Depolarization of presynaptic membrane • More depolarization caused more transmitter to be released • Extracellular Ca 2+ concentrations
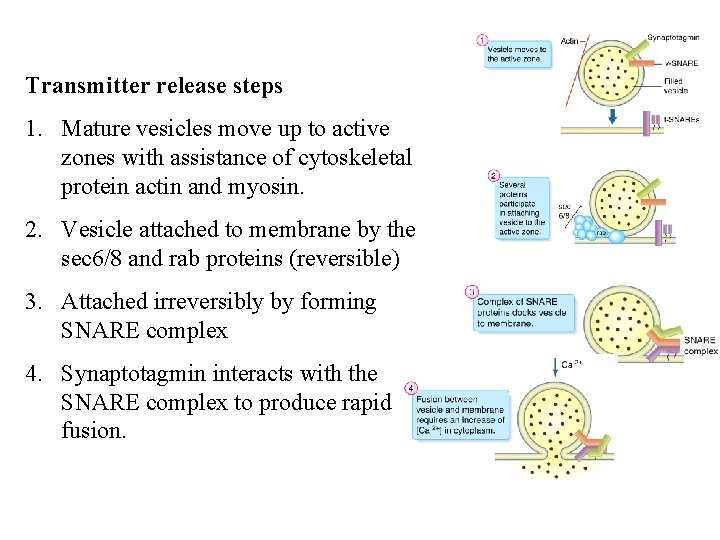
Transmitter release steps 1. Mature vesicles move up to active zones with assistance of cytoskeletal protein actin and myosin. 2. Vesicle attached to membrane by the sec 6/8 and rab proteins (reversible) 3. Attached irreversibly by forming SNARE complex 4. Synaptotagmin interacts with the SNARE complex to produce rapid fusion.
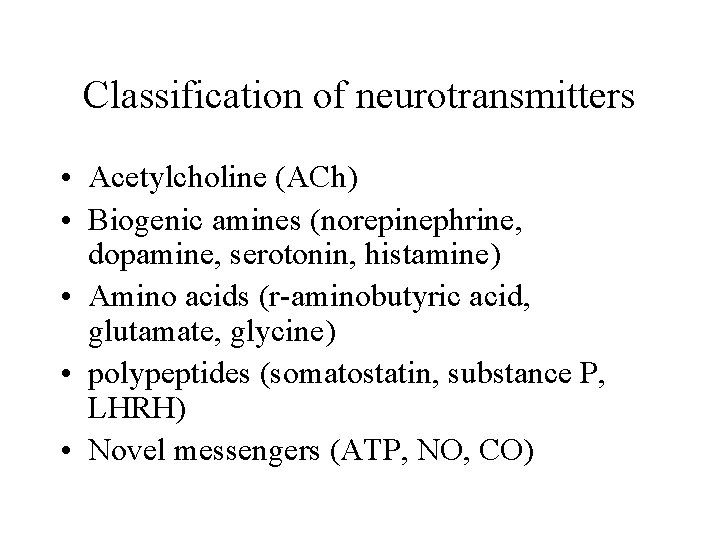
Classification of neurotransmitters • Acetylcholine (ACh) • Biogenic amines (norepinephrine, dopamine, serotonin, histamine) • Amino acids (r-aminobutyric acid, glutamate, glycine) • polypeptides (somatostatin, substance P, LHRH) • Novel messengers (ATP, NO, CO)
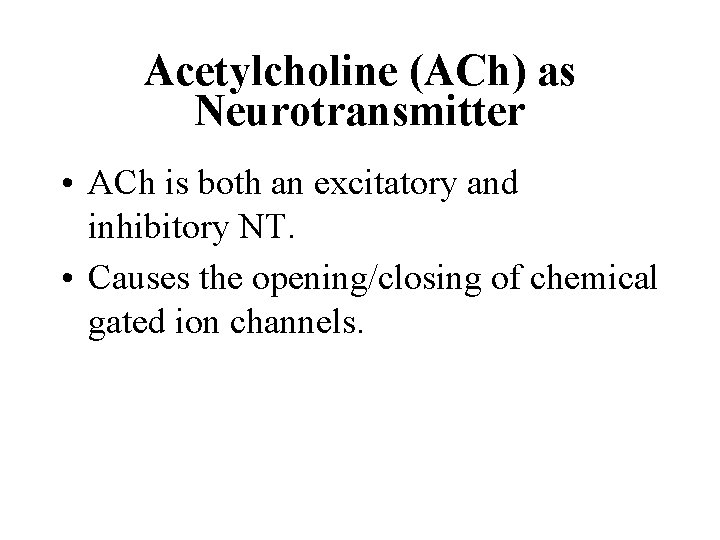
Acetylcholine (ACh) as Neurotransmitter • ACh is both an excitatory and inhibitory NT. • Causes the opening/closing of chemical gated ion channels.
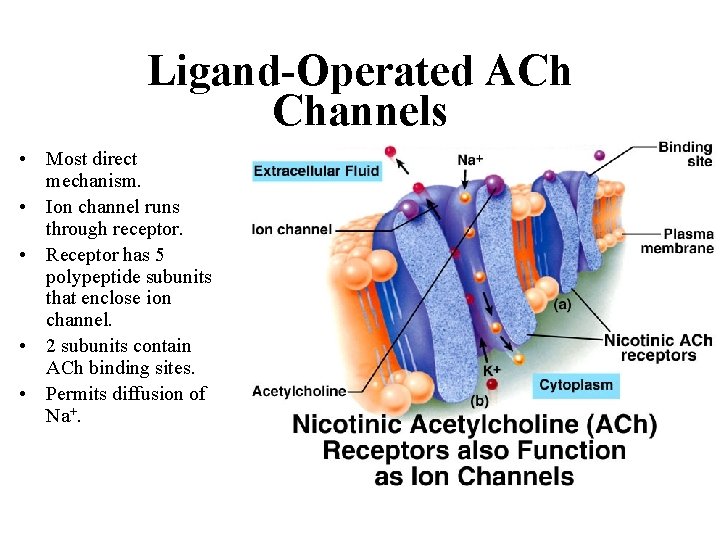
Ligand-Operated ACh Channels • Most direct mechanism. • Ion channel runs through receptor. • Receptor has 5 polypeptide subunits that enclose ion channel. • 2 subunits contain ACh binding sites. • Permits diffusion of Na+.
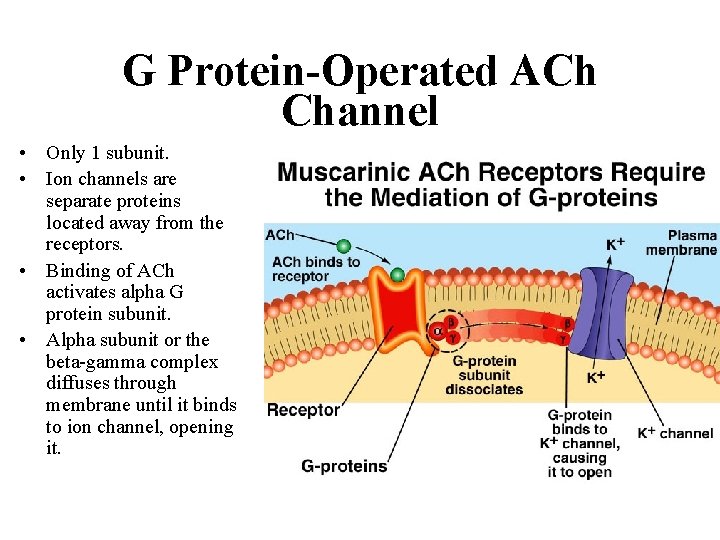
G Protein-Operated ACh Channel • Only 1 subunit. • Ion channels are separate proteins located away from the receptors. • Binding of ACh activates alpha G protein subunit. • Alpha subunit or the beta-gamma complex diffuses through membrane until it binds to ion channel, opening it.
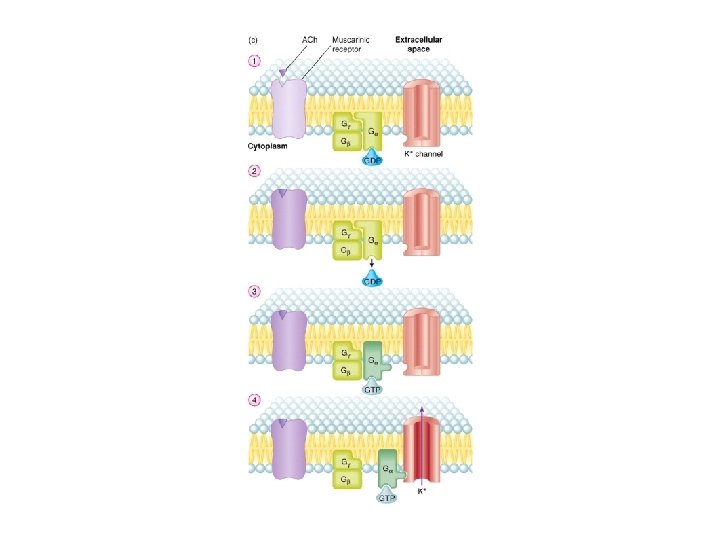
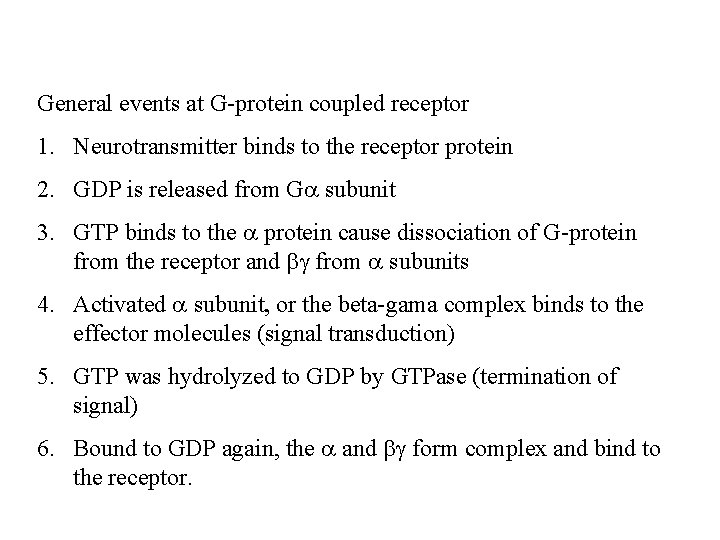
General events at G-protein coupled receptor 1. Neurotransmitter binds to the receptor protein 2. GDP is released from Ga subunit 3. GTP binds to the a protein cause dissociation of G-protein from the receptor and bg from a subunits 4. Activated a subunit, or the beta-gama complex binds to the effector molecules (signal transduction) 5. GTP was hydrolyzed to GDP by GTPase (termination of signal) 6. Bound to GDP again, the a and bg form complex and bind to the receptor.
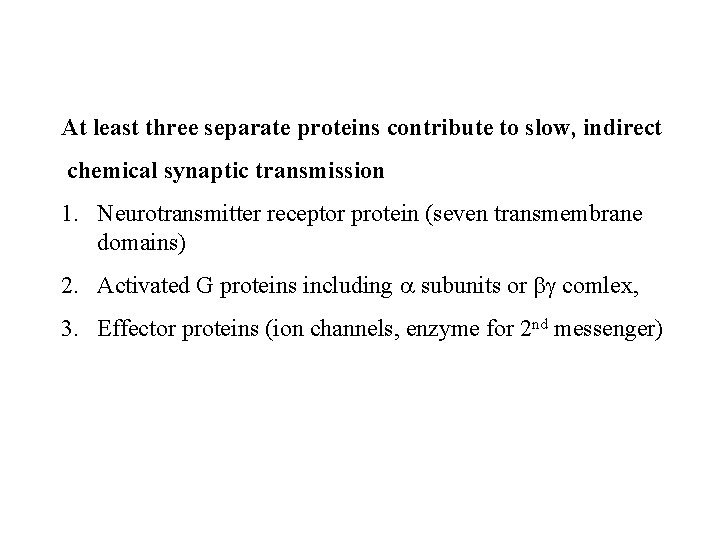
At least three separate proteins contribute to slow, indirect chemical synaptic transmission 1. Neurotransmitter receptor protein (seven transmembrane domains) 2. Activated G proteins including a subunits or bg comlex, 3. Effector proteins (ion channels, enzyme for 2 nd messenger)
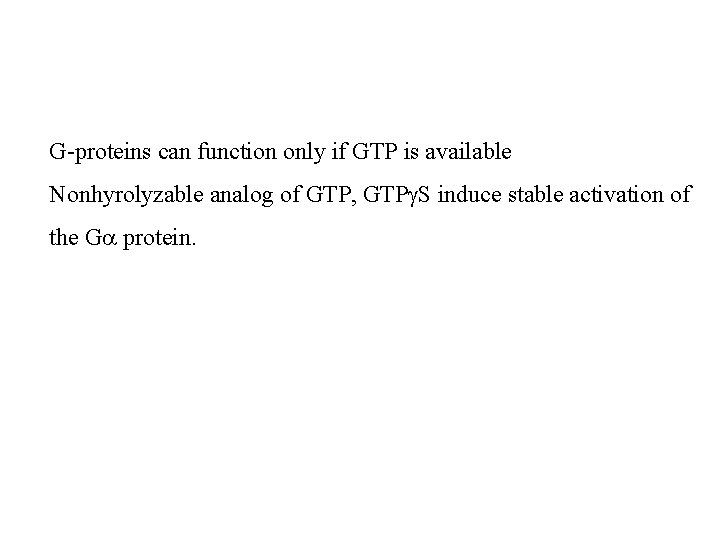
G-proteins can function only if GTP is available Nonhyrolyzable analog of GTP, GTPg. S induce stable activation of the Ga protein.
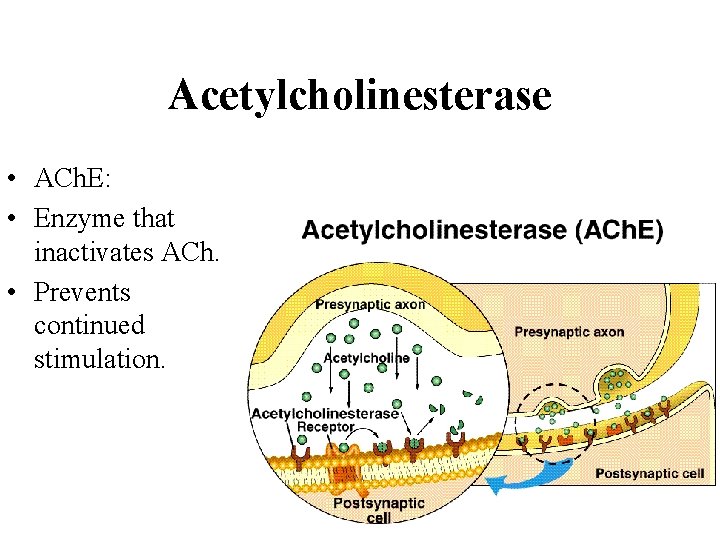
Acetylcholinesterase • ACh. E: • Enzyme that inactivates ACh. • Prevents continued stimulation.
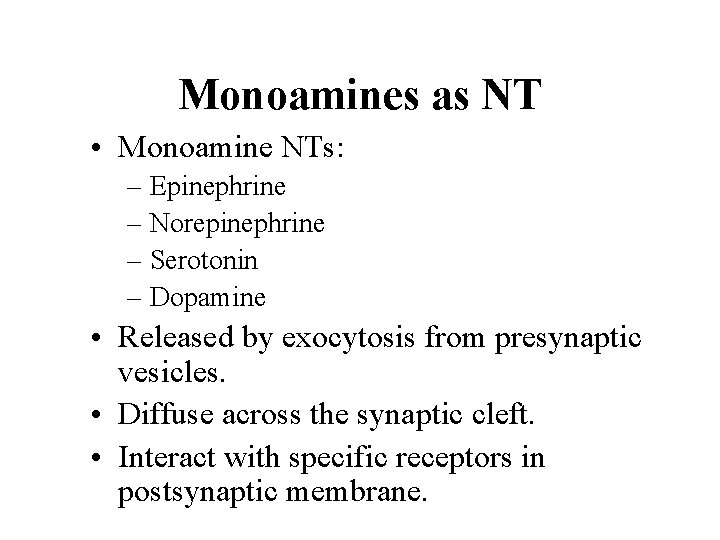
Monoamines as NT • Monoamine NTs: – Epinephrine – Norepinephrine – Serotonin – Dopamine • Released by exocytosis from presynaptic vesicles. • Diffuse across the synaptic cleft. • Interact with specific receptors in postsynaptic membrane.
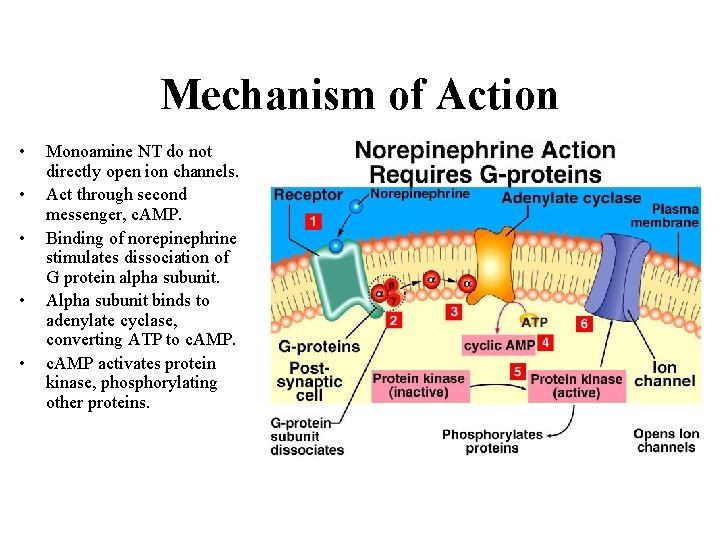
Mechanism of Action • • • Monoamine NT do not directly open ion channels. Act through second messenger, c. AMP. Binding of norepinephrine stimulates dissociation of G protein alpha subunit. Alpha subunit binds to adenylate cyclase, converting ATP to c. AMP activates protein kinase, phosphorylating other proteins.
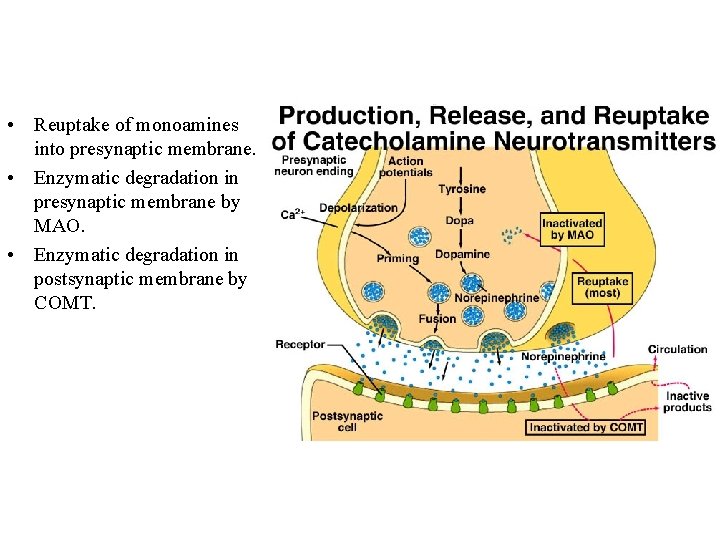
• Reuptake of monoamines into presynaptic membrane. • Enzymatic degradation in presynaptic membrane by MAO. • Enzymatic degradation in postsynaptic membrane by COMT.