Sensitivity of presynaptic p H on synaptic transmission
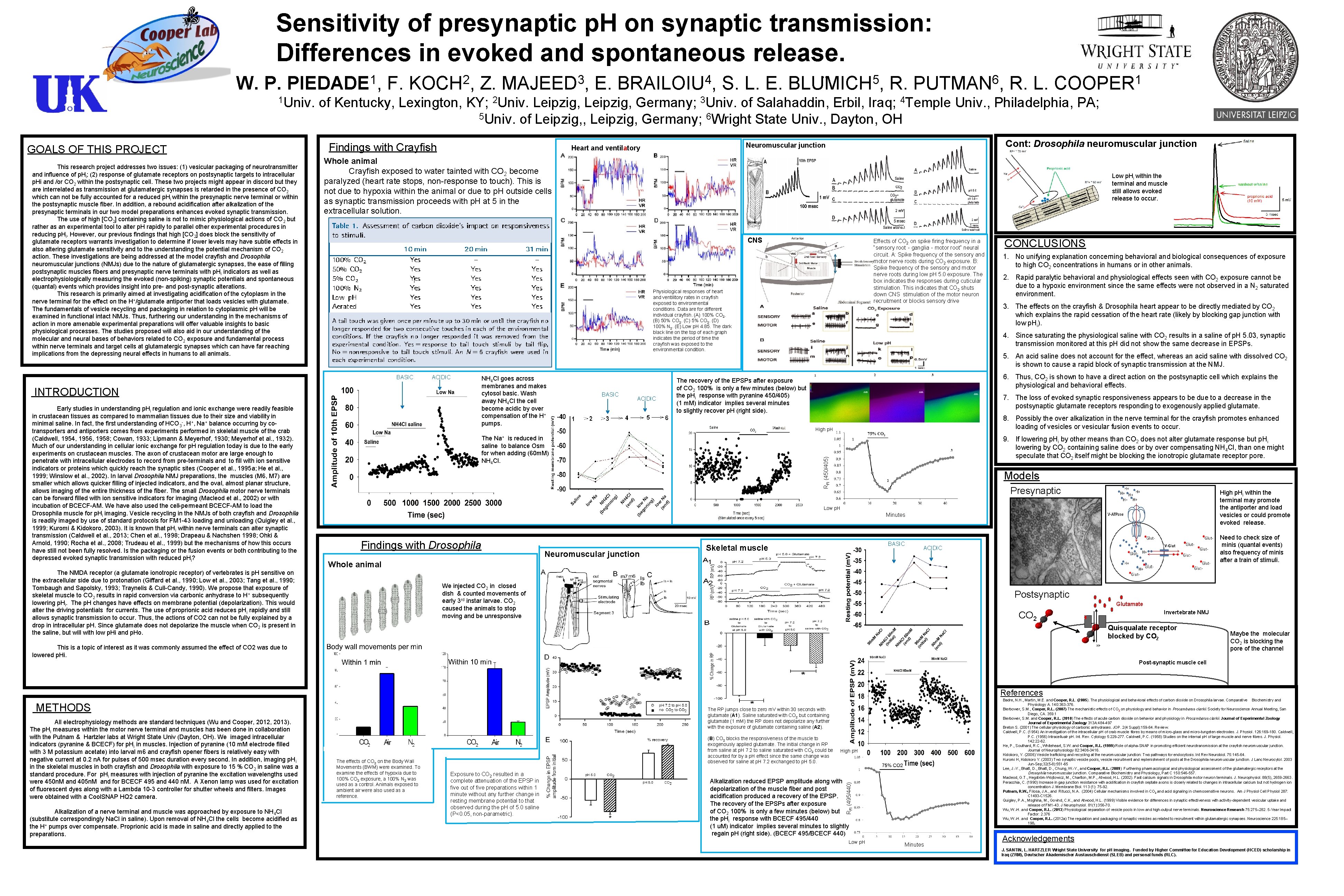
- Slides: 1
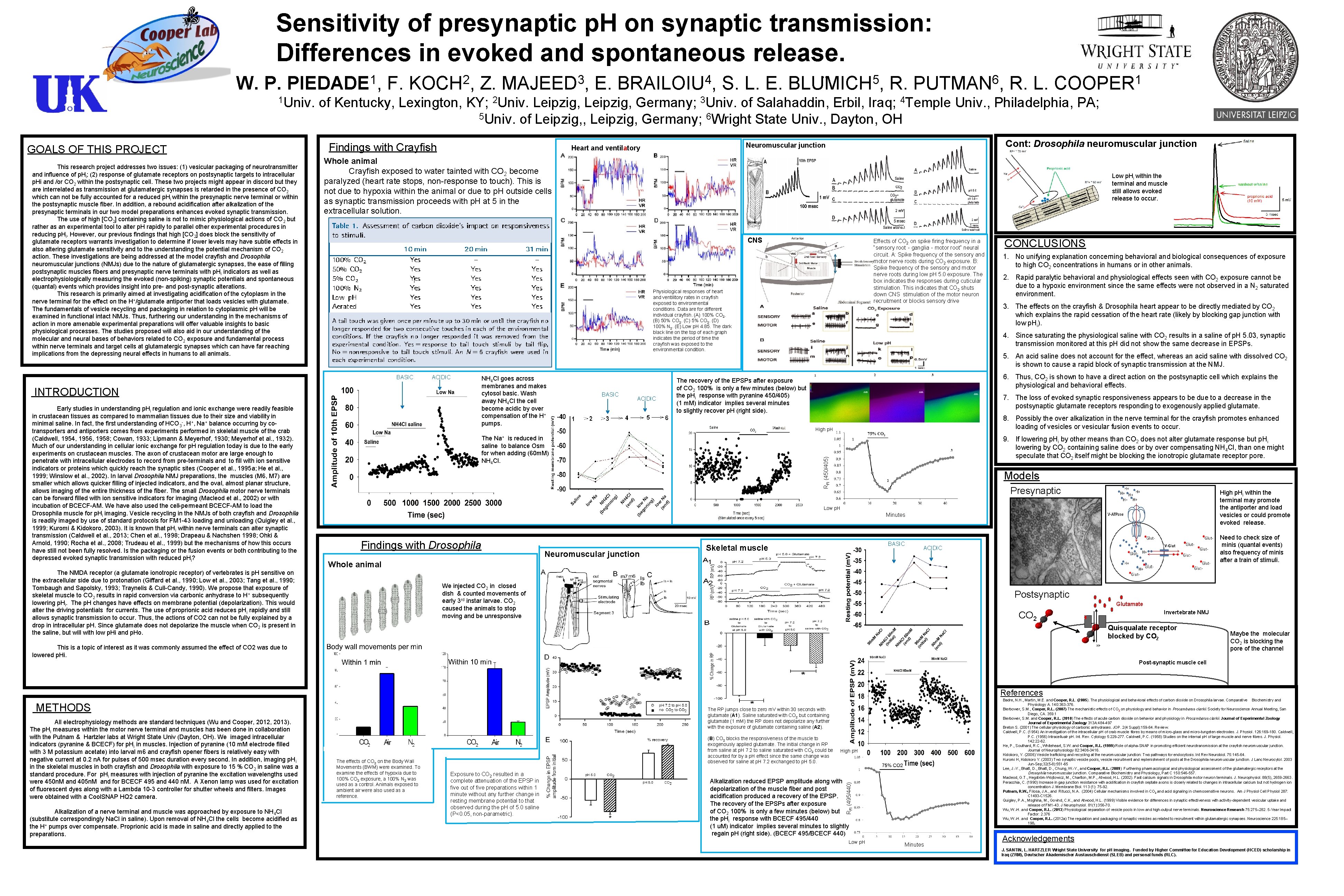
Sensitivity of presynaptic p. H on synaptic transmission: Differences in evoked and spontaneous release. 1 Univ. GOALS OF THIS PROJECT This research project addresses two issues: (1) vesicular packaging of neurotransmitter and influence of p. Hi; (2) response of glutamate receptors on postsynaptic targets to intracellular p. Hi and /or CO 2 within the postsynaptic cell. These two projects might appear in discord but they are interrelated as transmission at glutamatergic synapses is retarded in the presence of CO 2 which can not be fully accounted for a reduced p. Hi within the presynaptic nerve terminal or within the postsynaptic muscle fiber. In addition, a rebound acidification after alkalization of the presynaptic terminals in our two model preparations enhances evoked synaptic transmission. The use of high [CO 2] containing saline is not to mimic physiological actions of CO 2 but rather as an experimental tool to alter p. H rapidly to parallel other experimental procedures in reducing p. Hi. However, our previous findings that high [CO 2] does block the sensitivity of glutamate receptors warrants investigation to determine if lower levels may have subtle effects in also altering glutamate sensitivity and to the understanding the potential mechanism of CO 2 action. These investigations are being addressed at the model crayfish and Drosophila neuromuscular junctions (NMJs) due to the nature of glutamatergic synapses, the ease of filling postsynaptic muscles fibers and presynaptic nerve terminals with p. Hi indicators as well as electrophysiologically measuring the evoked (non-spiking) synaptic potentials and spontaneous (quantal) events which provides insight into pre- and post-synaptic alterations. This research is primarily aimed at investigating acidification of the cytoplasm in the nerve terminal for the effect on the H+/glutamate antiporter that loads vesicles with glutamate. The fundamentals of vesicle recycling and packaging in relation to cytoplasmic p. H will be examined in functional intact NMJs. Thus, furthering our understanding in the mechanisms of action in more amenable experimental preparations will offer valuable insights to basic physiological processes. The studies proposed will also aid in our understanding of the molecular and neural bases of behaviors related to CO 2 exposure and fundamental process within nerve terminals and target cells at glutamatergic synapses which can have far reaching implications from the depressing neural effects in humans to all animals. F. 2 KOCH , Z. E. 4 BRAILOIU , S. L. E. 5 BLU MICH , R. 6 PUTMAN , R. L. 1 COOPER of Kentucky, Lexington, KY; 2 Univ. Leipzig, Germany; 3 Univ. of Salahaddin, Erbil, Iraq; 4 Temple Univ. , Philadelphia, PA; 5 Univ. of Leipzig, , Leipzig, Germany; 6 Wright State Univ. , Dayton, OH Findings with Crayfish Cont: Drosophila neuromuscular junction Neuromuscular junction Heart and ventilatory Whole animal Crayfish exposed to water tainted with CO 2 become paralyzed (heart rate stops, non-response to touch). This is not due to hypoxia within the animal or due to p. H outside cells as synaptic transmission proceeds with p. H at 5 in the extracellular solution. Low p. Hi within the terminal and muscle still allows evoked release to occur. CNS Effects of CO 2 on spike firing frequency in a “sensory root‐ganglia‐motor root” neural circuit. A: Spike frequency of the sensory and motor nerve roots during CO 2 exposure. B: Spike frequency of the sensory and motor nerve roots during low p. H 5. 0 exposure. The box indicates the responses during cuticular stimulation. This indicates that CO 2 shuts down CNS stimulation of the motor neuron recruitment or blocks sensory drive Physiological responses of heart and ventilitory rates in crayfish exposed to environmental conditions. Data are for different individual crayfish. (A) 100% CO 2. (B) 50% CO 2. (C) 5% CO 2. (D) 100% N 2. (E) Low p. H 4. 85. The dark black line on the top of each graph indicates the period of time the crayfish was exposed to the environmental condition. BASIC ACIDIC INTRODUCTION Early studies in understanding p. Hi regulation and ionic exchange were readily feasible in crustacean tissues as compared to mammalian tissues due to their size and viability in minimal saline. In fact, the first understanding of HCO 3 -, H+, Na+ balance occurring by cotransporters and antiporters comes from experiments performed in skeletal muscle of the crab (Caldwell, 1954, 1956, 1958; Cowan, 1933; Lipmann & Meyerhof, 1930; Meyerhof et al. , 1932). Much of our understanding in cellular ionic exchange for p. H regulation today is due to the early experiments on crustacean muscles. The axon of crustacean motor are large enough to penetrate with intracellular electrodes to record from pre-terminals and to fill with ion sensitive indicators or proteins which quickly reach the synaptic sites (Cooper et al. , 1995 a; He et al. , 1999; Winslow et al. , 2002). In larval Drosophila NMJ preparations, the muscles (M 6, M 7) are smaller which allows quicker filling of injected indicators, and the oval, almost planar structure, allows imaging of the entire thickness of the fiber. The small Drosophila motor nerve terminals can be forward filled with ion sensitive indicators for imaging (Macleod et al. , 2002) or with incubation of BCECF-AM. We have also used the cell-permeant BCECF-AM to load the Drosophila muscle for p. Hi imaging. Vesicle recycling in the NMJs of both crayfish and Drosophila is readily imaged by use of standard protocols for FM 1 -43 loading and unloading (Quigley et al. , 1999; Kuromi & Kidokoro, 2003). It is known that p. Hi within nerve terminals can alter synaptic transmission (Caldwell et al. , 2013; Chen et al. , 1998; Drapeau & Nachshen 1998; Ohki & Arnold, 1990; Rocha et al. , 2008; Trudeau et al. , 1999) but the mechanisms of how this occurs have still not been fully resolved. Is the packaging or the fusion events or both contributing to the depressed evoked synaptic transmission with reduced p. Hi? 3 MAJEED , NH 4 Cl goes across membranes and makes cytosol basic. Wash away NH 4 Cl the cell become acidic by over compensation of the H+ pumps. BASIC ACIDIC 1. No unifying explanation concerning behavioral and biological consequences of exposure to high CO 2 concentrations in humans or in other animals. 2. Rapid paralytic behavioral and physiological effects seen with CO 2 exposure cannot be due to a hypoxic environment since the same effects were not observed in a N 2 saturated environment. 3. The effects on the crayfish & Drosophila heart appear to be directly mediated by CO 2 which explains the rapid cessation of the heart rate (likely by blocking gap junction with low p. Hi). 5. An acid saline does not account for the effect, whereas an acid saline with dissolved CO 2 is shown to cause a rapid block of synaptic transmission at the NMJ. 6. Thus, CO 2 is shown to have a direct action on the postsynaptic cell which explains the physiological and behavioral effects. The recovery of the EPSPs after exposure of CO 2 100% is only a few minutes (below) but the p. Hi response with pyranine 450/405) (1 m. M) indicator implies several minutes to slightly recover p. H (right side). 7. The loss of evoked synaptic responsiveness appears to be due to a decrease in the postsynaptic glutamate receptors responding to exogenously applied glutamate. 8. Possibly the over alkalization in the nerve terminal for the crayfish promotes enhanced loading of vesicles or vesicular fusion events to occur. High p. H The Na+ is reduced in saline to balance Osm for when adding (60 m. M) NH 4 Cl. CONCLUSIONS 4. Since saturating the physiological saline with CO 2 results in a saline of p. H 5. 03, synaptic transmission monitored at this p. H did not show the same decrease in EPSPs. 9. If lowering p. Hi by other means than CO 2 does not alter glutamate response but p. Hi lowering by CO 2 containing saline does or by over compensating NH 4 Cl, than one might speculate that CO 2 itself might be blocking the ionotropic glutamate receptor pore. RFl (450/405) W. P. 1 PIEDADE , Models Presynaptic High p. Hi within the terminal may promote the antiporter and load vesicles or could promote evoked release. Low p. H Minutes Findings with Drosophila Neuromuscular junction BASIC Skeletal muscle Need to check size of minis (quantal events) also frequency of minis after a train of stimuli. ACIDIC Whole animal The NMDA receptor (a glutamate ionotropic receptor) of vertebrates is p. H sensitive on the extracellular side due to protonation (Giffard et al. , 1990; Low et al. , 2003; Tang et al. , 1990; Tombaugh and Sapolsky, 1993; Traynelis & Cull-Candy, 1990). We propose that exposure of skeletal muscle to CO 2 results in rapid conversion via carbonic anhydrase to H+ subsequently lowering p. Hi. The p. H changes have effects on membrane potential (depolarization). This would alter the driving potentials for currents. The use of proprionic acid reduces p. Hi rapidly and still allows synaptic transmission to occur. Thus, the actions of CO 2 can not be fully explained by a drop in intracellular p. H. Since glutamate does not depolarize the muscle when CO 2 is present in the saline, but will with low p. Hi and p. Ho. We injected CO 2 in closed dish & counted movements of early 3 rd instar larvae. CO 2 caused the animals to stop moving and be unresponsive Postsynaptic Glutamate Invertebrate NMJ CO 2 Quisqualate receptor blocked by CO 2 K+ This is a topic of interest as it was commonly assumed the effect of CO 2 was due to lowered p. Hi. Maybe the molecular CO 2 is blocking the pore of the channel Post-synaptic muscle cell References METHODS Alkalization of a nerve terminal and muscle was approached by exposure to NH 4 Cl (substitute correspondingly Na. Cl in saline). Upon removal of NH 4 Cl the cells become acidified as the H+ pumps over compensate. Proprionic acid is made in saline and directly applied to the preparations. The RP jumps close to zero m. V within 30 seconds with glutamate (A 1). Saline saturated with CO 2 but containing glutamate (1 m. M) the RP does not depolarize any further with the exposure of glutamate containing saline (A 2). The effects of CO 2 on the Body Wall Movements (BWM) were examined. To examine the effects of hypoxia due to 100% CO 2 exposure, a 100% N 2 was used as a control. Animals exposed to ambient air were also used as a reference. (B) CO 2 blocks the responsiveness of the muscle to exogenously applied glutamate. The initial change in RP from saline at p. H 7. 2 to saline saturated with CO 2 could be accounted for by a p. H effect since the same change was observed for saline at p. H 7. 2 exchanged to p. H 5. 0. Exposure to CO 2 resulted in a complete attenuation of the EPSP in five out of five preparations within 1 minute without any further change in resting membrane potential to that observed during the p. H of 5. 0 saline (P<0. 05, non-parametric). High p. H Alkalization reduced EPSP amplitude along with depolarization of the muscle fiber and post acidification produced a recovery of the EPSP. The recovery of the EPSPs after exposure of CO 2 100% is only a few minutes (below) but the p. Hi response with BCECF 495/440 (1 u. M) indicator implies several minutes to slightly regain p. H (right side). (BCECF 495/BCECF 440) RFl (495/440) All electrophysiology methods are standard techniques (Wu and Cooper, 2012, 2013). The p. Hi measures within the motor nerve terminal and muscles has been done in collaboration with the Putnam & Hartzler labs at Wright State Univ (Dayton, OH). We imaged intracellular indicators (pyranine & BCECF) for p. Hi in muscles. Injection of pyranine (10 m. M electrode filled with 3 M potassium acetate) into larval m 6 and crayfish opener fibers is relatively easy with negative current at 0. 2 n. A for pulses of 500 msec duration every second. In addition, imaging p. H i in the skeletal muscles in both crayfish and Drosophila with exposure to 15 % CO 2 in saline was a standard procedure. For p. Hi measures with injection of pyranine the excitation wavelengths used were 450 n. M and 405 n. M and for BCECF 495 and 440 n. M. A Xenon lamp was used for excitation of fluorescent dyes along with a Lambda 10 -3 controller for shutter wheels and filters. Images were obtained with a Cool. SNAP HQ 2 camera. Badre, N. H. , Martin, M. E. and Cooper, R. L. (2005). The physiological and behavioral effects of carbon dioxide on Drosophila larvae. Comparative Biochemistry and Physiology A. 140: 363 -376. Bierbower, S. M. , Cooper, R. L. (2007) The mechanistic effects of CO 2 on physiology and behavior in Procambarus clarkii. Society for Neuroscience Annual Meeting, San Diego, CA. 359. 1 Bierbower, S. M. and Cooper, R. L. (2010) The effects of acute carbon dioxide on behavior and physiology in Procambarus clarkii. Journal of Experimental Zoology 313 A: 484 -497 Breton S. (2001) The cellular physiology of carbonic anhydrases. JOP. 2(4 Suppl): 159 -64. Review. Caldwell, P. C. (1954) An investigation of the intracellular p. H of crab muscle fibres by means of micro-glass and micro-tungsten electrodes. J. Physiol. 126: 169 -180. Caldwell, P. C. (1956) Intracellualr p. H. Int. Rev. Cytology 5: 229 -277. Caldwell, P. C. (1958) Studies on the internal p. H of large muscle and nerve fibres. J. Physiol. 142: 22 -62. He, P. , Southard, R. C. , Whiteheart, S. W. and Cooper, R. L. (1999) Role of alpha-SNAP in promoting efficient neurotransmission at the crayfish neuromuscular junction. Journal of Neurophysiology 82: 3406 -3416. Kidokoro, Y. (2006) Vesicle trafficking and recycling at the neuromuscular junction: Two pathways for endocytosis. Int Rev Neurobiol. 75: 145 -64. Kuromi H, Kidokoro Y. (2003) Two synaptic vesicle pools, vesicle recruitment and replenishment of pools at the Drosophila neuromuscular junction. J Lanc Neurocytol. 2003 Jun-Sep; 32(5 -8): 551 -65 Lee, J. -Y. , Bhatt, D. , Chung, W. -Y. , and Cooper, R. L. (2009) Furthering pharmacological and physiological assessment of the glutamatergic receptors at the Drosophila neuromuscular junction. Comparative Biochemistry and Physiology, Part C 150: 546 -557. Macleod, G. T. , Hegström-Wojtowicz, M. , Charlton, M. P. , Atwood, H. L. (2002). Fast calcium signals in Drosophila motor neuron terminals. J. Neurophysiol. 88(5), 2659 -2663. Peracchia, C. (1990) Increase in gap junction resistance with acidification in crayfish septate axons is closely related to changes in intracellular calcium but not hydrogen ion concentration J. Membrane Biol. 113 (1): 75 -92. Putnam, R. W. , Filosa, J. A. , and Ritucci, N. A. (2004) Cellular mechanisms involved in CO 2 and acid signaling in chemosensitive neurons. Am J Physiol Cell Physiol 287: C 1493 -C 1526. Quigley, P. A. , Msghina, M. , Govind, C. K. , and Atwood, H. L. (1999) Visible evidence for differences in synaptic effectiveness with activity-dependent vesicular uptake and release of FM 1 -43. J Neurophysiol. 81(1): 356 -70. Wu, W. -H. and Cooper, R. L. (2013) Physiological separation of vesicle pools in low- and high-output nerve terminals. Neuroscience Research 75: 275– 282. 5 -Year Impact Factor: 2. 376 Wu, W. -H. and Cooper, R. L. (2012 a) The regulation and packaging of synaptic vesicles as related to recruitment within glutamatergic synapses. Neuroscience 225: 185– 198. Low p. H Minutes Acknowledgements J. SANTIN, L. HARTZLER Wright State University for p. H imaging. Funded by Higher Committee for Education Development (HCED) scholarship in Iraq (ZRM), Deutscher Akademischer Austauschdienst (SLEB) and personal funds (RLC).