Seismic methods in HC exploration Geophysical prospecting and
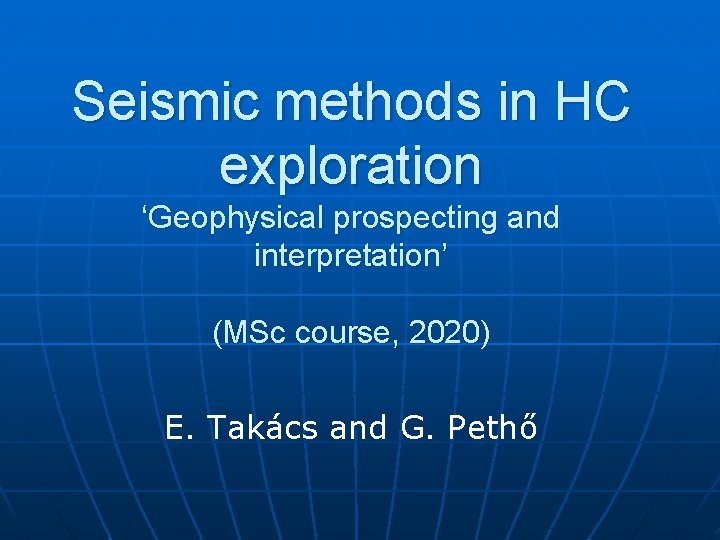
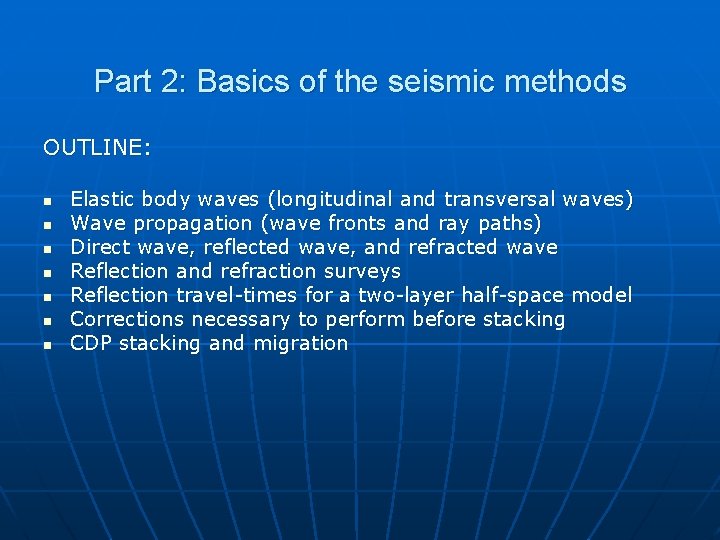
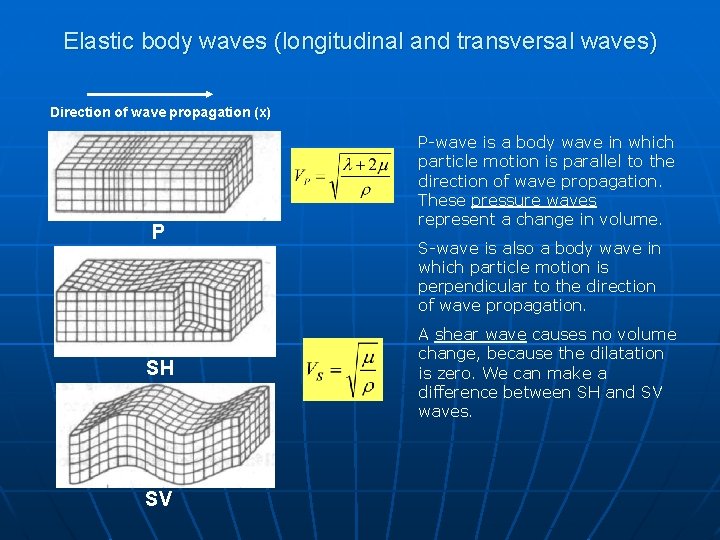
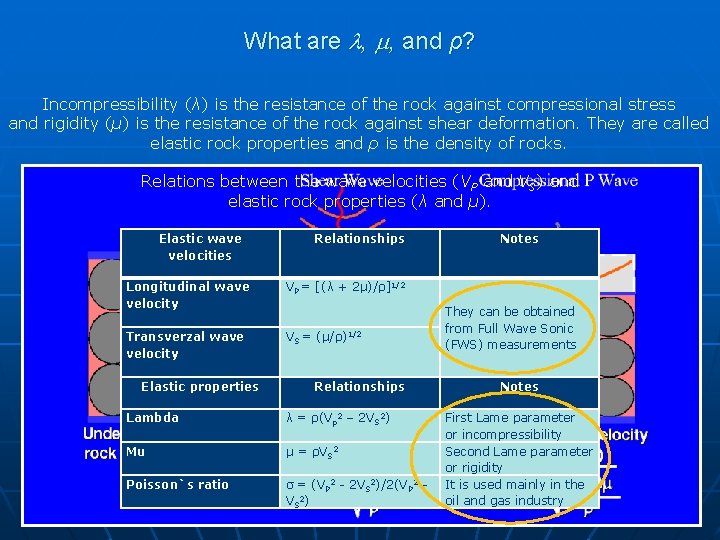
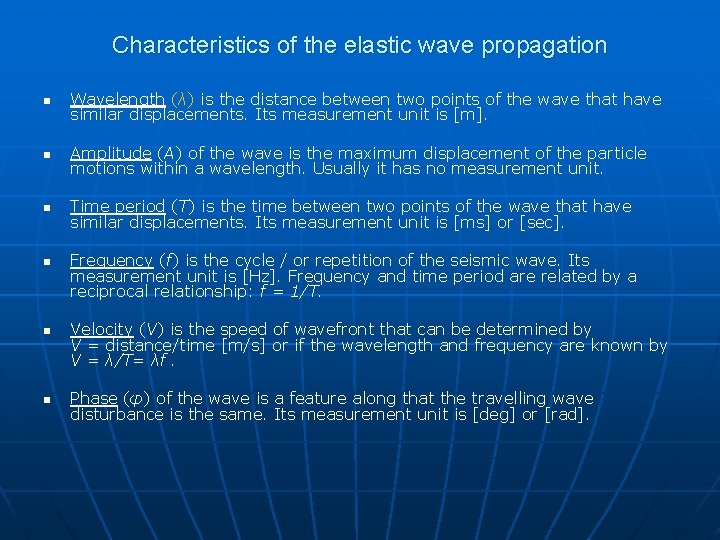
![P- and S-wave velocities in some materials Material P-wave velocity [m/s] S-wave velocity [m/s] P- and S-wave velocities in some materials Material P-wave velocity [m/s] S-wave velocity [m/s]](https://slidetodoc.com/presentation_image_h/0301b16b3b100373e5f876df5b7f0a77/image-6.jpg)
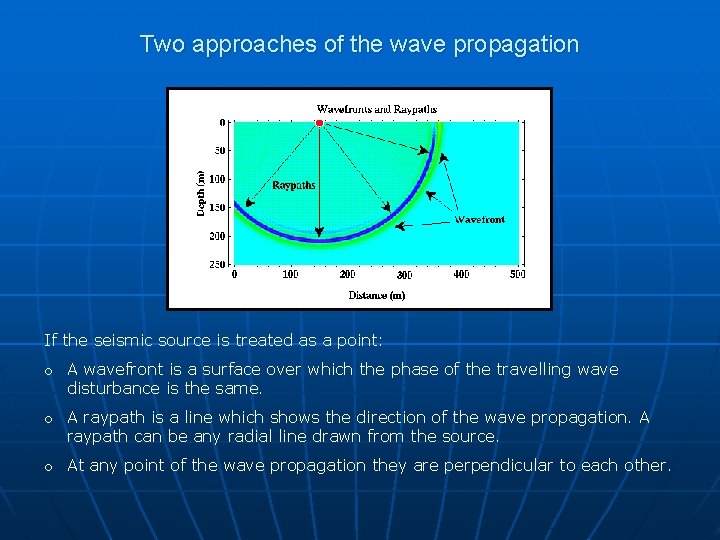
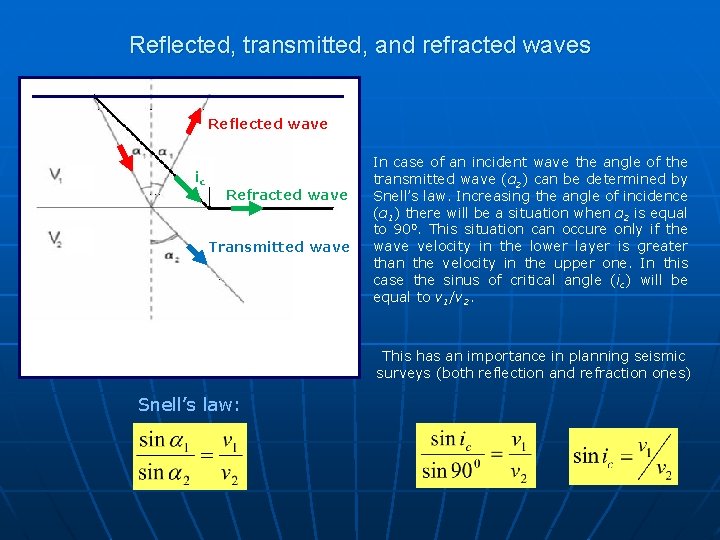
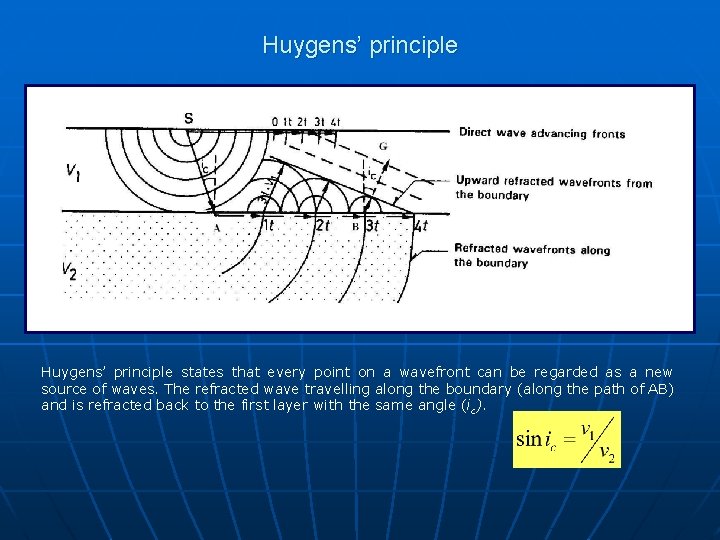
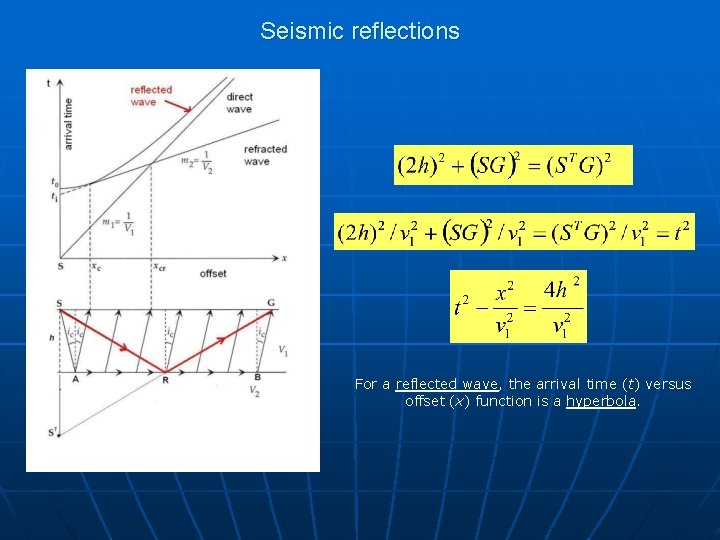
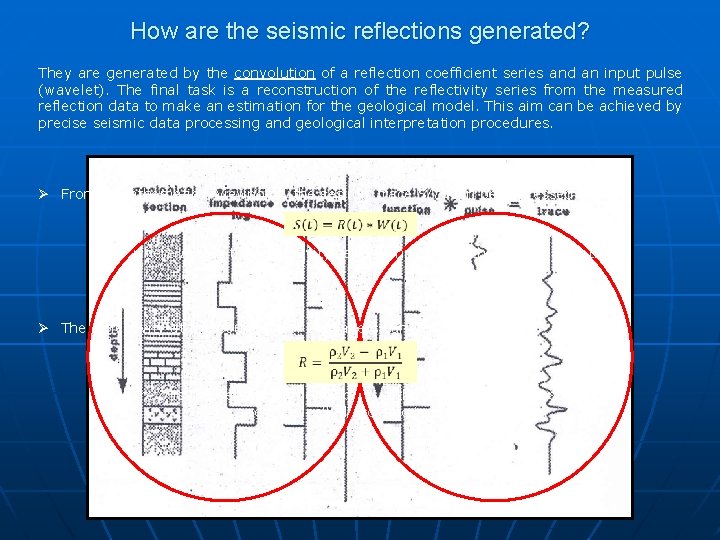
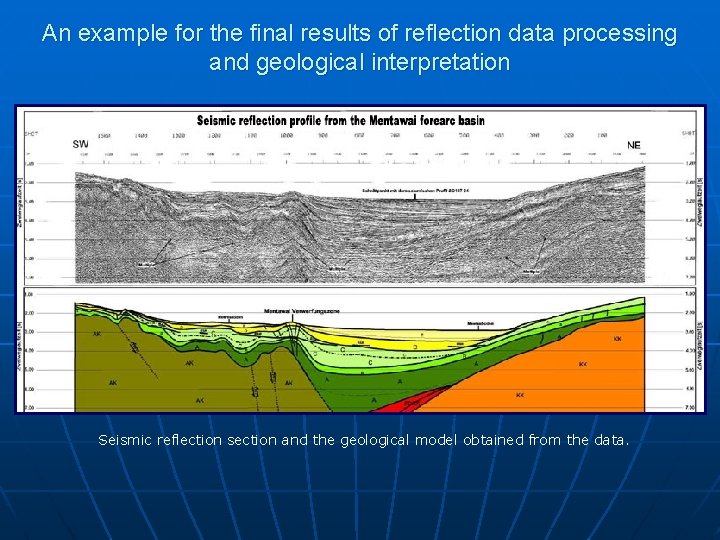
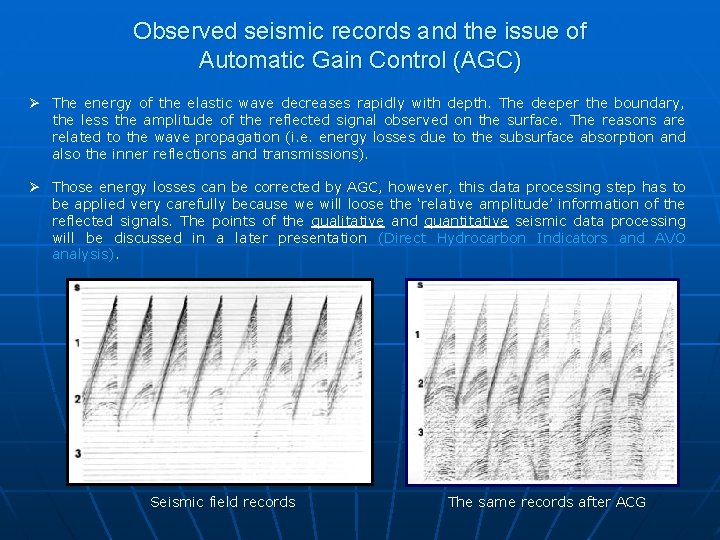
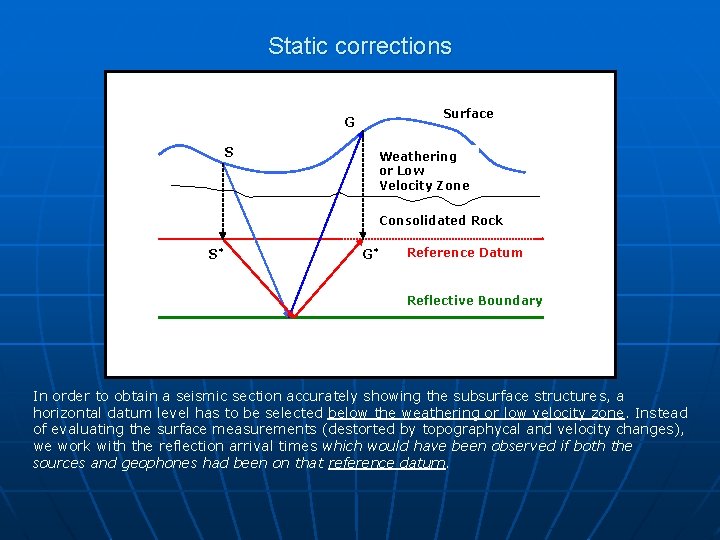
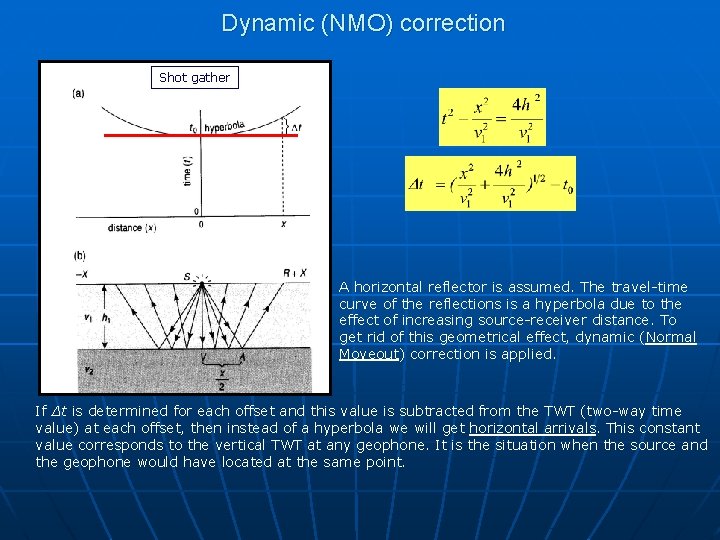
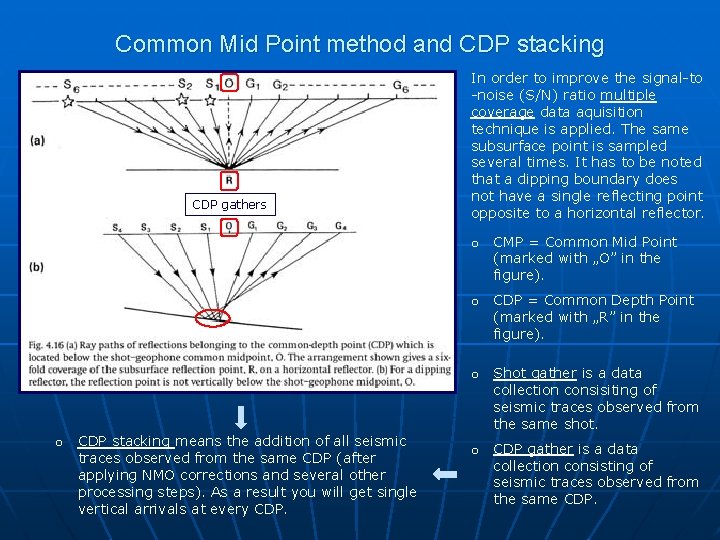
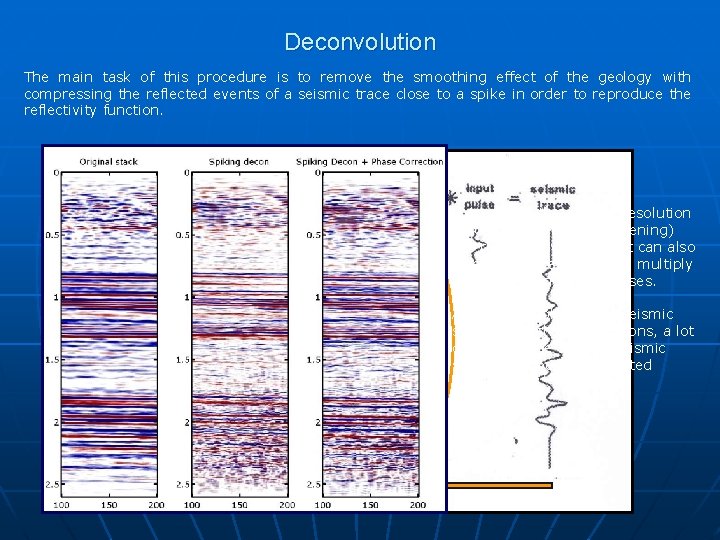
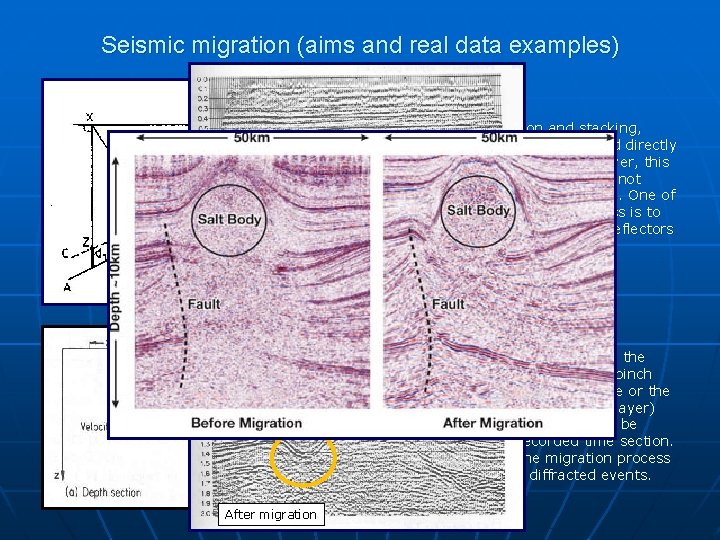
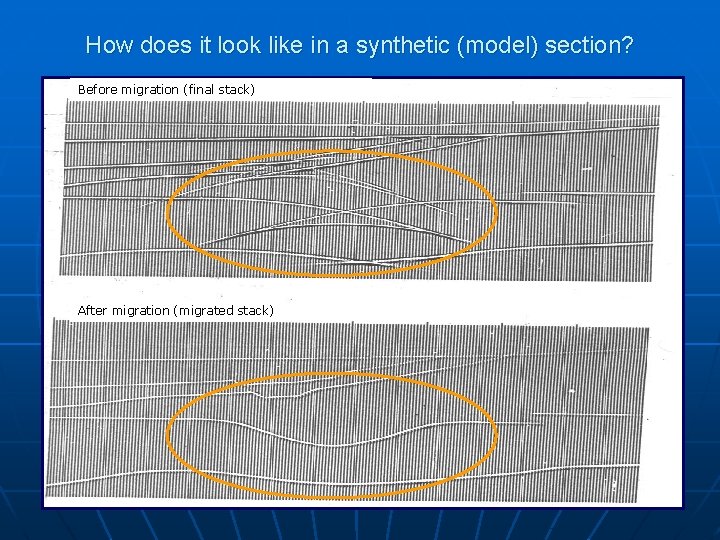
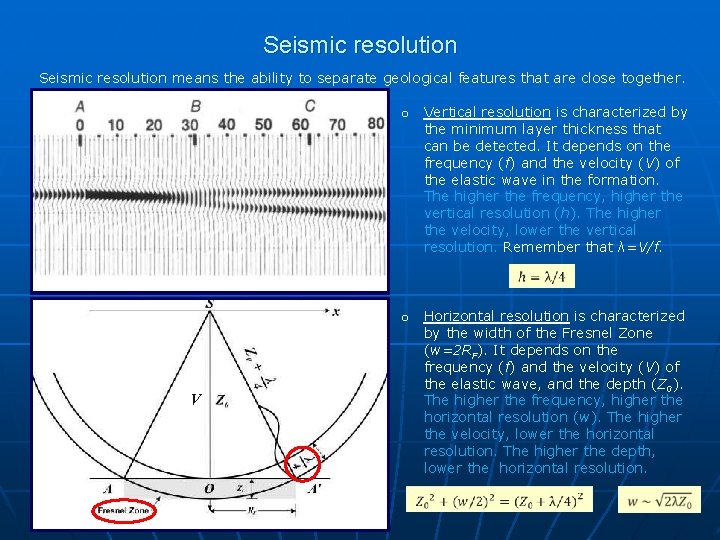
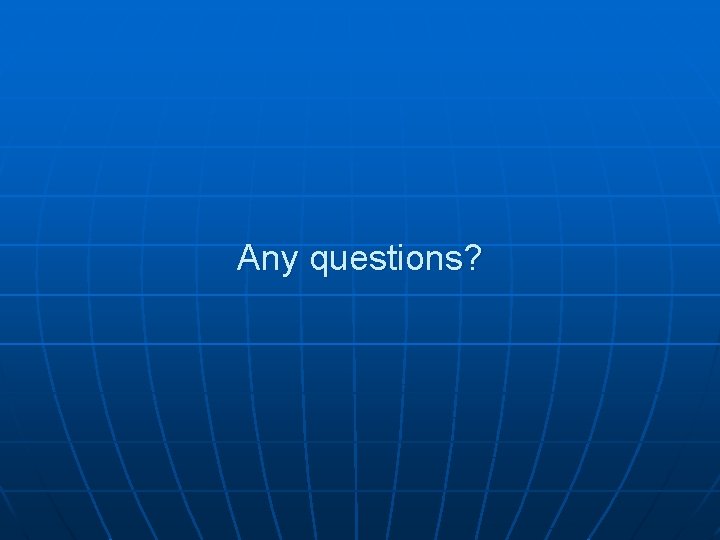
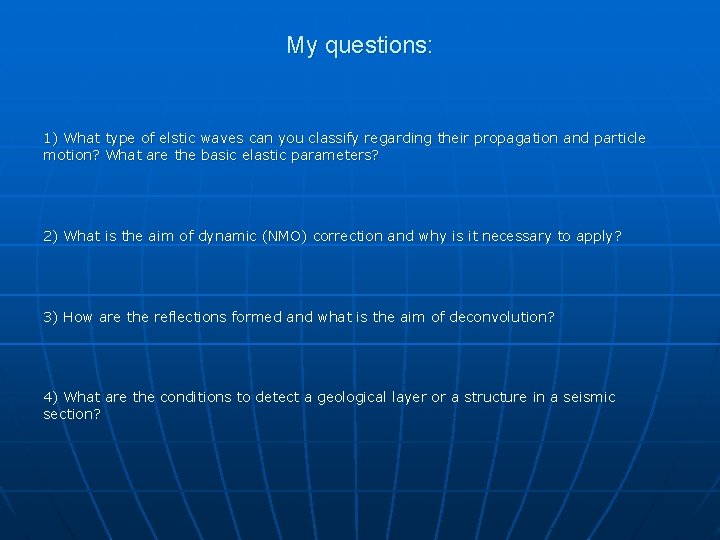
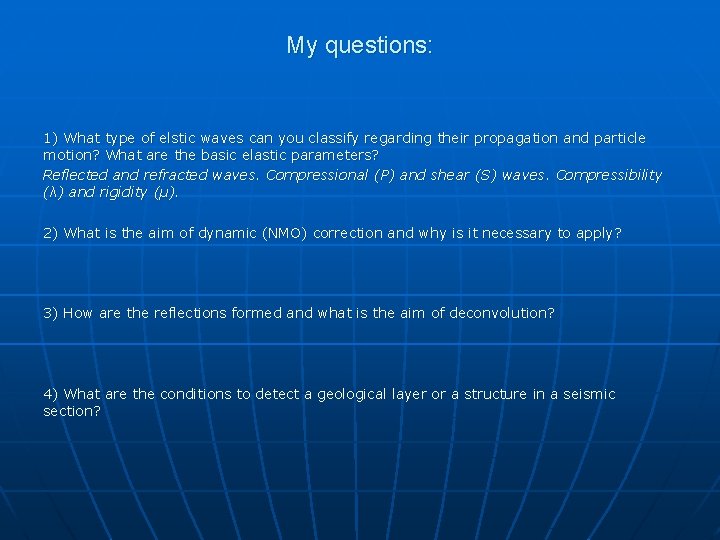
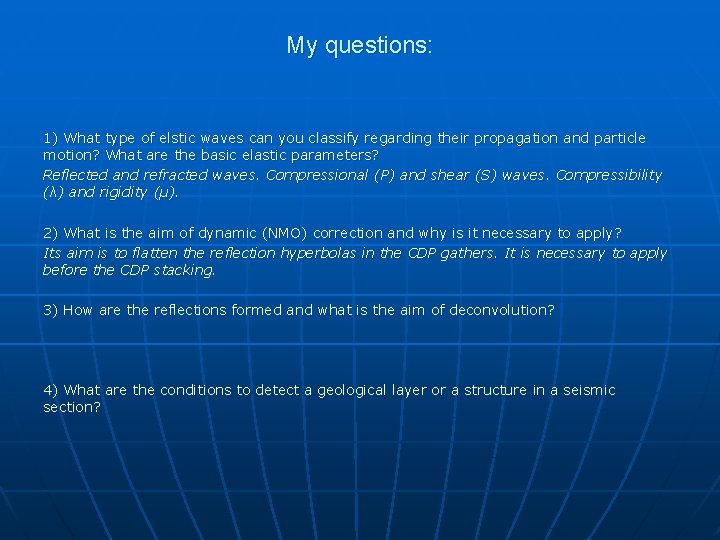
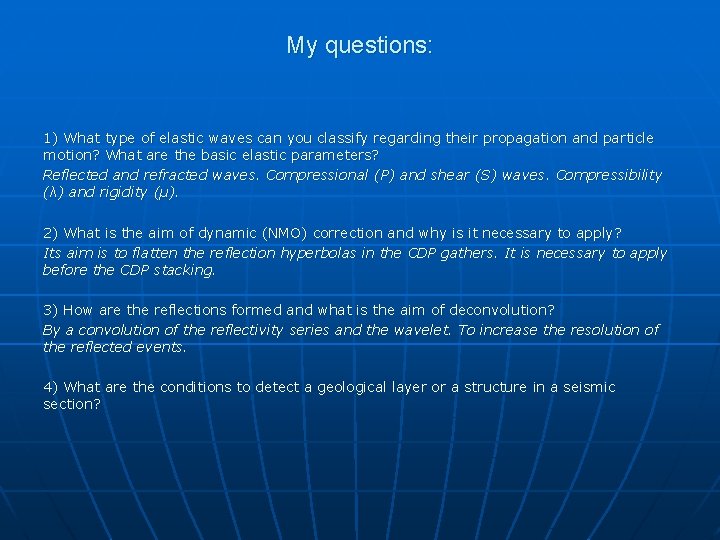
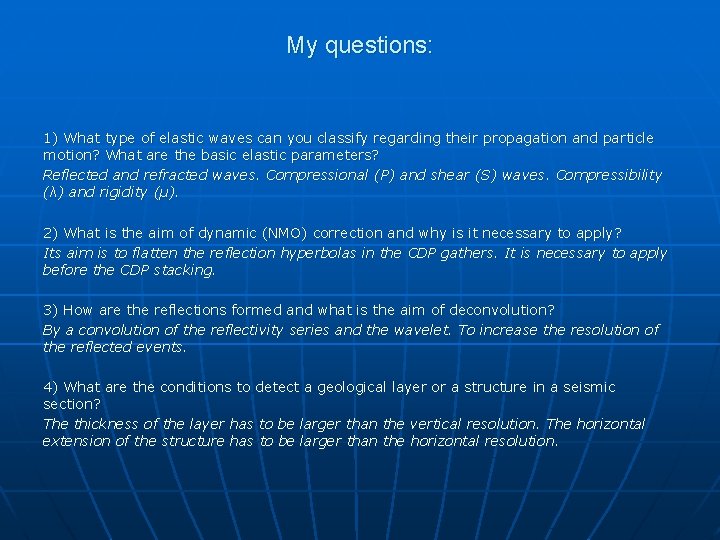
- Slides: 26
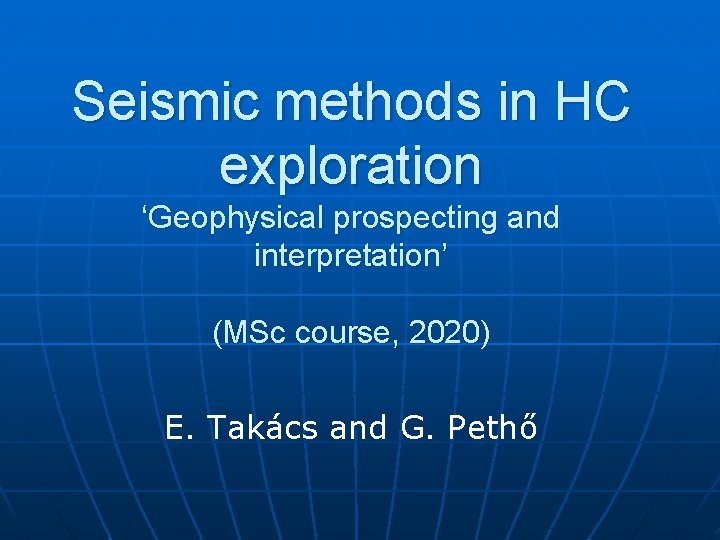
Seismic methods in HC exploration ‘Geophysical prospecting and interpretation’ (MSc course, 2020) E. Takács and G. Pethő
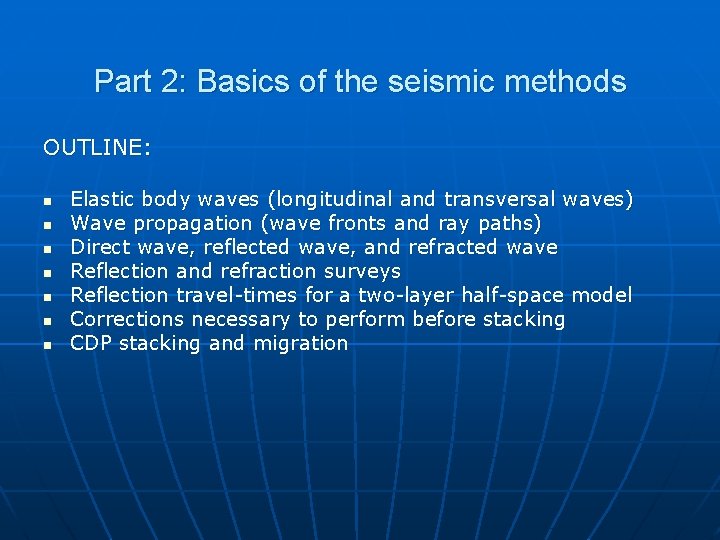
Part 2: Basics of the seismic methods OUTLINE: n n n n Elastic body waves (longitudinal and transversal waves) Wave propagation (wave fronts and ray paths) Direct wave, reflected wave, and refracted wave Reflection and refraction surveys Reflection travel-times for a two-layer half-space model Corrections necessary to perform before stacking CDP stacking and migration
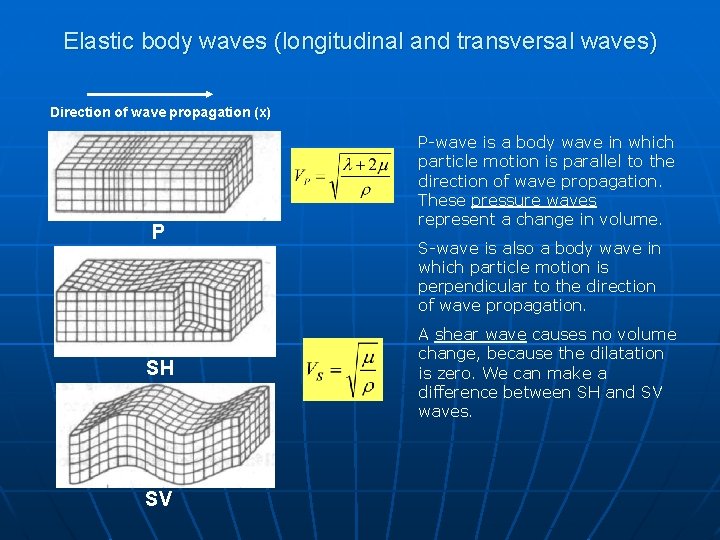
Elastic body waves (longitudinal and transversal waves) Direction of wave propagation (x) P SH SV P-wave is a body wave in which particle motion is parallel to the direction of wave propagation. These pressure waves represent a change in volume. S-wave is also a body wave in which particle motion is perpendicular to the direction of wave propagation. A shear wave causes no volume change, because the dilatation is zero. We can make a difference between SH and SV waves.
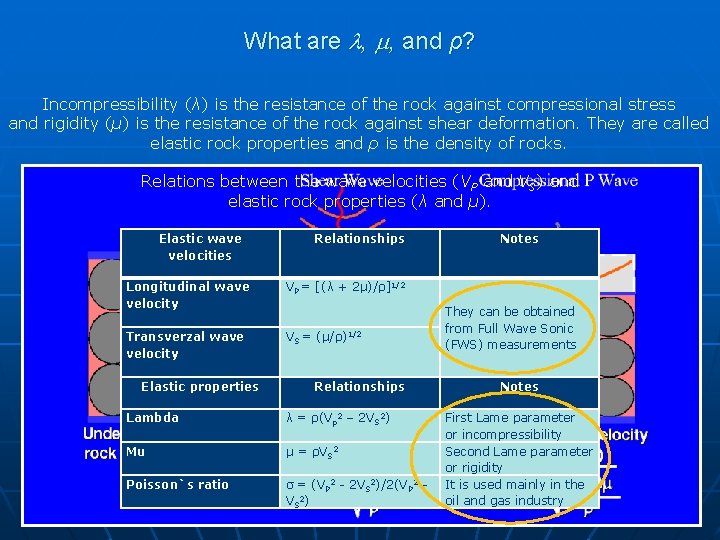
What are , , and ρ? Incompressibility (λ) is the resistance of the rock against compressional stress and rigidity (µ) is the resistance of the rock against shear deformation. They are called elastic rock properties and ρ is the density of rocks. Relations between the wave velocities (VP and VS) and elastic rock properties (λ and µ). Elastic wave velocities Relationships Longitudinal wave velocity Transverzal wave velocity Elastic properties VP = [(λ + 2μ)/ρ]1/2 Lambda λ = ρ(Vp 2 – 2 VS 2) Mu μ = ρVS 2 Poisson`s ratio σ = (VP 2 - 2 VS 2)/2(VP 2 - VS 2) VS = (μ/ρ)1/2 Relationships Notes They can be obtained from Full Wave Sonic (FWS) measurements Notes First Lame parameter or incompressibility Second Lame parameter or rigidity It is used mainly in the oil and gas industry
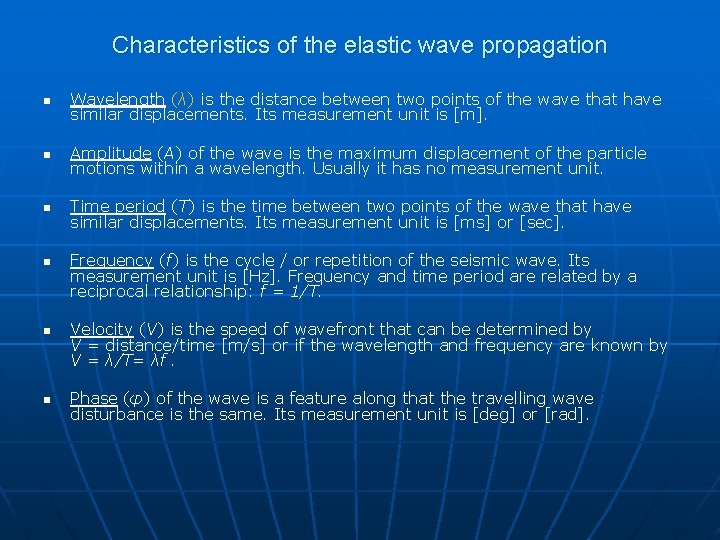
Characteristics of the elastic wave propagation n Wavelength (λ) is the distance between two points of the wave that have similar displacements. Its measurement unit is [m]. n Amplitude (A) of the wave is the maximum displacement of the particle motions within a wavelength. Usually it has no measurement unit. n Time period (T) is the time between two points of the wave that have similar displacements. Its measurement unit is [ms] or [sec]. n n n Frequency (f) is the cycle / or repetition of the seismic wave. Its measurement unit is [Hz]. Frequency and time period are related by a reciprocal relationship: f = 1/T. Velocity (V) is the speed of wavefront that can be determined by V = distance/time [m/s] or if the wavelength and frequency are known by V = λ/T= λf. Phase (φ) of the wave is a feature along that the travelling wave disturbance is the same. Its measurement unit is [deg] or [rad]. disturbance is the same
![P and Swave velocities in some materials Material Pwave velocity ms Swave velocity ms P- and S-wave velocities in some materials Material P-wave velocity [m/s] S-wave velocity [m/s]](https://slidetodoc.com/presentation_image_h/0301b16b3b100373e5f876df5b7f0a77/image-6.jpg)
P- and S-wave velocities in some materials Material P-wave velocity [m/s] S-wave velocity [m/s] 330 ----- Water 1400 -1500 ----- Petroleum 1300 -1400 ----- Granite 5500 -5900 2800 -3000 6400 3200 Sandstone 1400 -4300 700 -2800 Limestone 5900 -6100 2800 -3000 Sand (unsaturated) 200 -1000 80 -400 Sand (saturated) 800 -2200 320 -880 Clay 1000 -2500 400 -1000 Glacial till (saturated) 1500 -2500 600 -1000 Air Basalt The elastic rock properties (λ and µ) are more characteristic for the medium than the wave velocities (VP and VS).
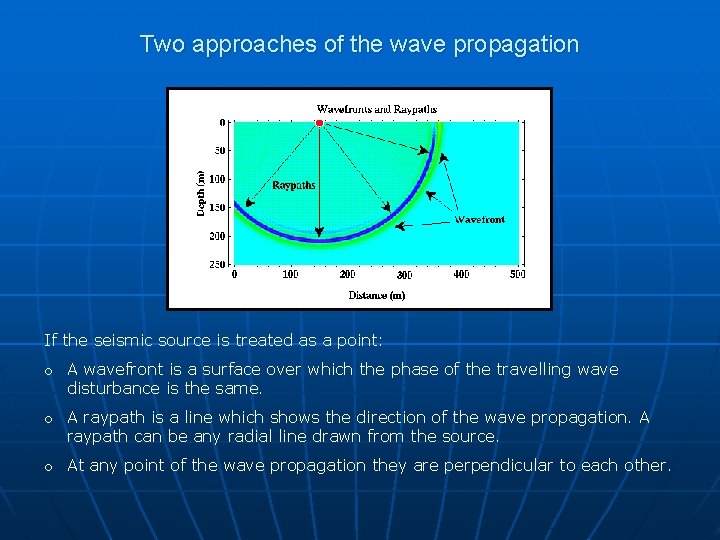
Two approaches of the wave propagation If the seismic source is treated as a point: o A wavefront is a surface over which the phase of the travelling wave disturbance is the same. o A raypath is a line which shows the direction of the wave propagation. A raypath can be any radial line drawn from the source. o At any point of the wave propagation they are perpendicular to each other.
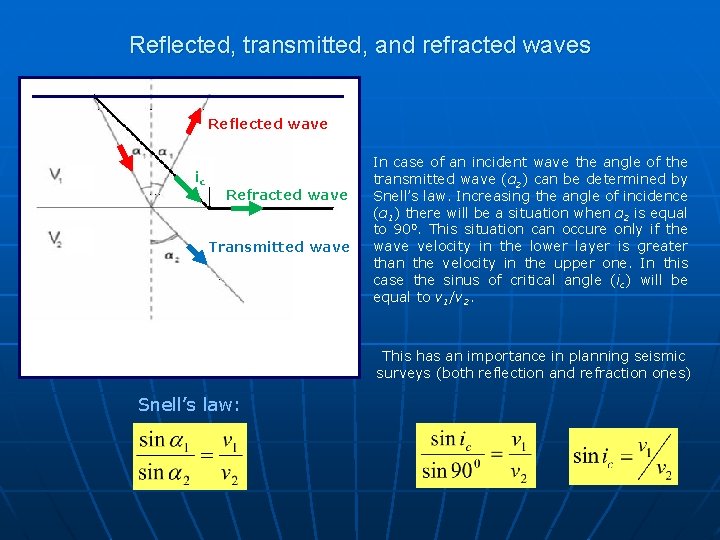
Reflected, transmitted, and refracted waves Reflected wave icc Refracted wave Transmitted wave In case of an incident wave the angle of the transmitted wave (α 2) can be determined by Snell’s law. Increasing the angle of incidence (α 1) there will be a situation when α 2 is equal to 900. This situation can occure only if the wave velocity in the lower layer is greater than the velocity in the upper one. In this case the sinus of critical angle (ic) will be equal to v 1/v 2. This has an importance in planning seismic surveys (both reflection and refraction ones) Snell’s law:
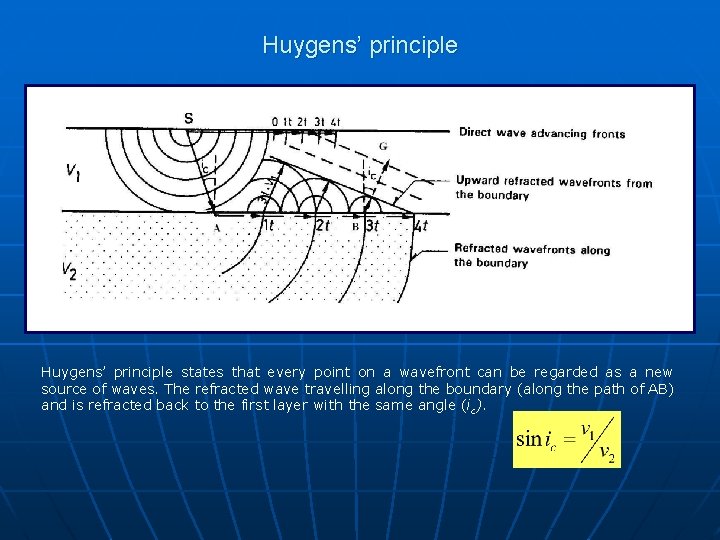
Huygens’ principle states that every point on a wavefront can be regarded as a new source of waves. The refracted wave travelling along the boundary (along the path of AB) and is refracted back to the first layer with the same angle (ic).
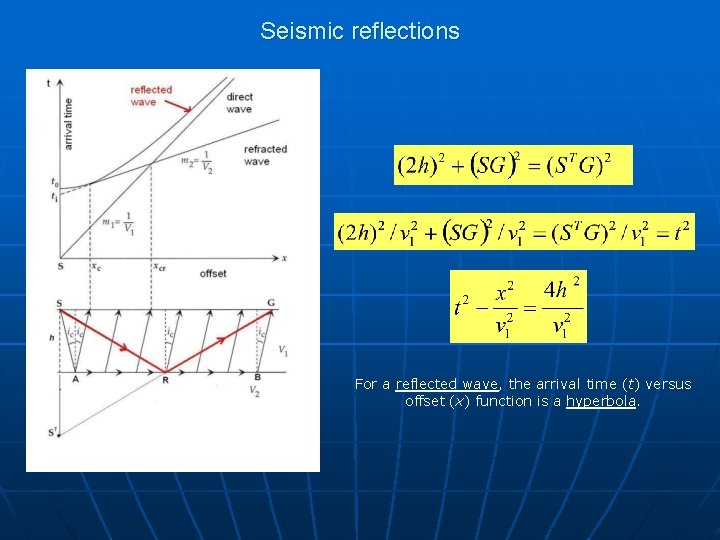
Seismic reflections For a reflected wave, the arrival time (t) versus offset (x) function is a hyperbola.
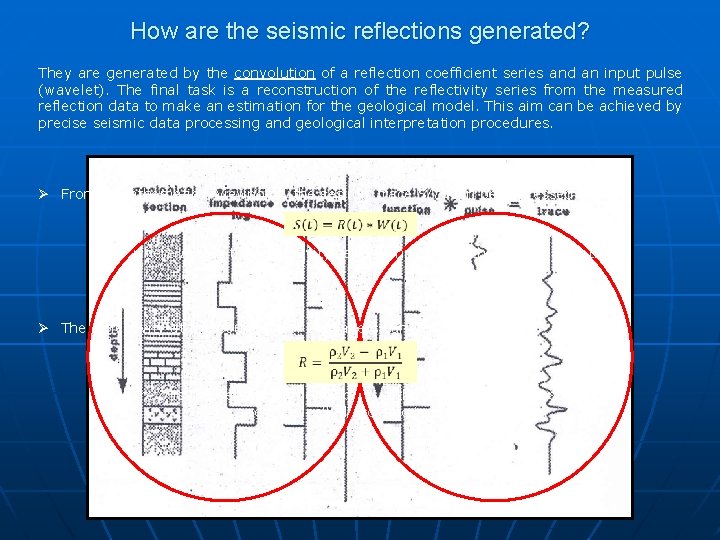
How are the seismic reflections generated? They are generated by the convolution of a reflection coefficient series and an input pulse (wavelet). The final task is a reconstruction of the reflectivity series from the measured reflection data to make an estimation for the geological model. This aim can be achieved by precise seismic data processing and geological interpretation procedures. Ø From a mathematical viewpoint, the sesimic trace S(t) can be expressed as where R(t) is the reflectivity series, and W(t) is the input wavelet. Ø The reflectivity at the boundary of two layers can be calculated by where ρ is the density of the rocks, V is the velocity of the elastic wave, and ρV is called acoustic impedance.
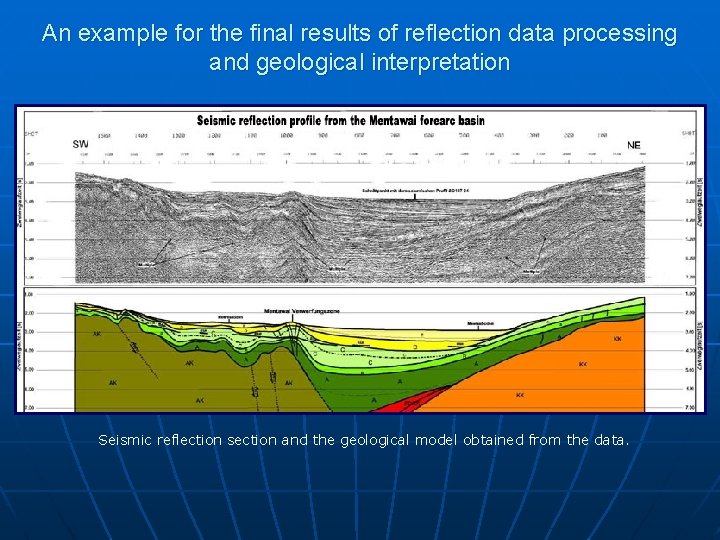
An example for the final results of reflection data processing and geological interpretation Seismic reflection section and the geological model obtained from the data.
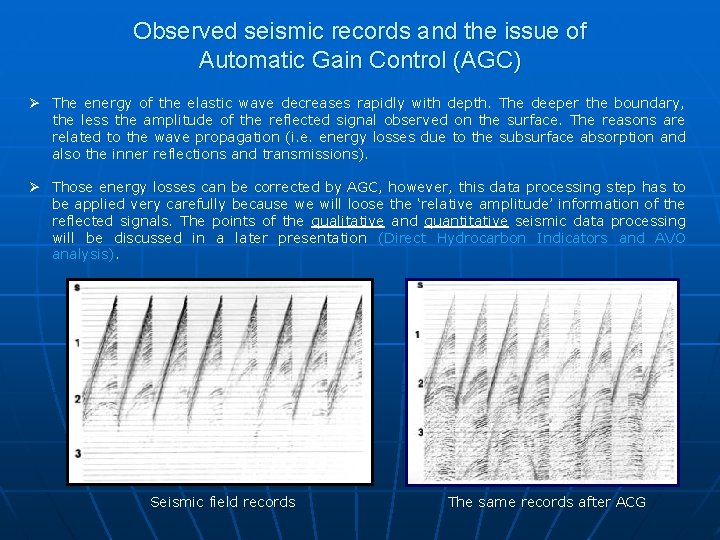
Observed seismic records and the issue of Automatic Gain Control (AGC) Ø The energy of the elastic wave decreases rapidly with depth. The deeper the boundary, the less the amplitude of the reflected signal observed on the surface. The reasons are related to the wave propagation (i. e. energy losses due to the subsurface absorption and also the inner reflections and transmissions). Ø Those energy losses can be corrected by AGC, however, this data processing step has to be applied very carefully because we will loose the ‘relative amplitude’ information of the reflected signals. The points of the qualitative and quantitative seismic data processing will be discussed in a later presentation (Direct Hydrocarbon Indicators and AVO analysis). Seismic field records The same records after ACG
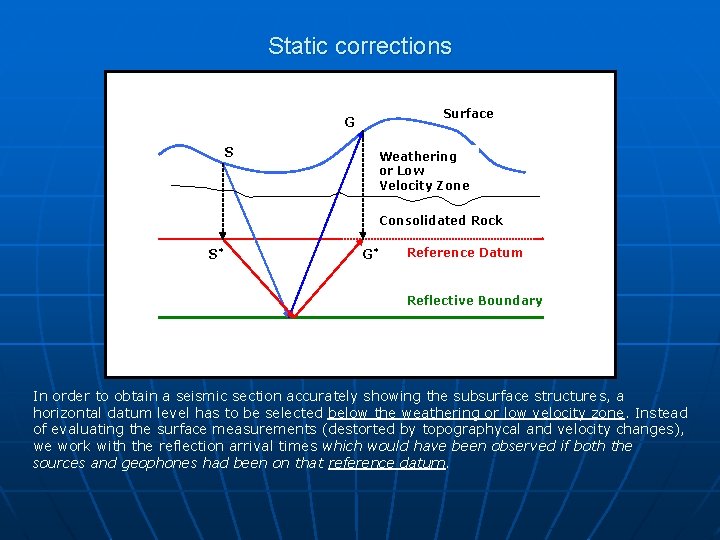
Static corrections Surface G S Weathering or Low Velocity Zone Consolidated Rock S* G* Reference Datum Reflective Boundary In order to obtain a seismic section accurately showing the subsurface structures, a horizontal datum level has to be selected below the weathering or low velocity zone. Instead of evaluating the surface measurements (destorted by topographycal and velocity changes), we work with the reflection arrival times which would have been observed if both the sources and geophones had been on that reference datum.
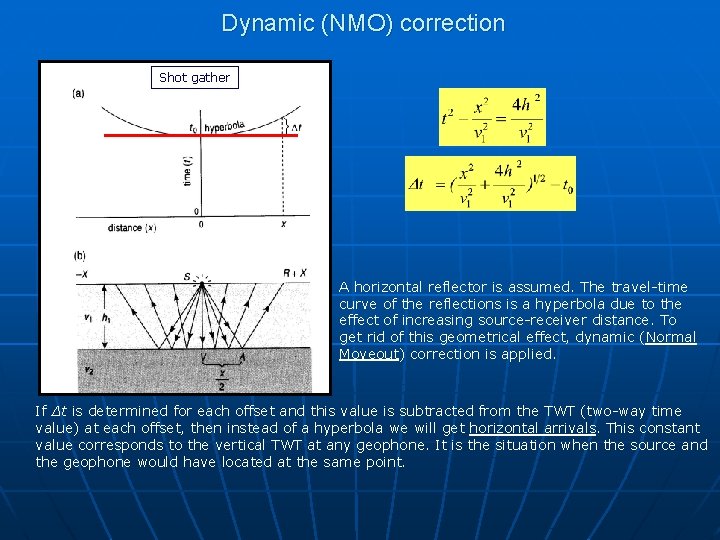
Dynamic (NMO) correction Shot gather A horizontal reflector is assumed. The travel-time curve of the reflections is a hyperbola due to the effect of increasing source-receiver distance. To get rid of this geometrical effect, dynamic (Normal Moveout) correction is applied. If ∆t is determined for each offset and this value is subtracted from the TWT (two-way time value) at each offset, then instead of a hyperbola we will get horizontal arrivals. This constant value corresponds to the vertical TWT at any geophone. It is the situation when the source and the geophone would have located at the same point.
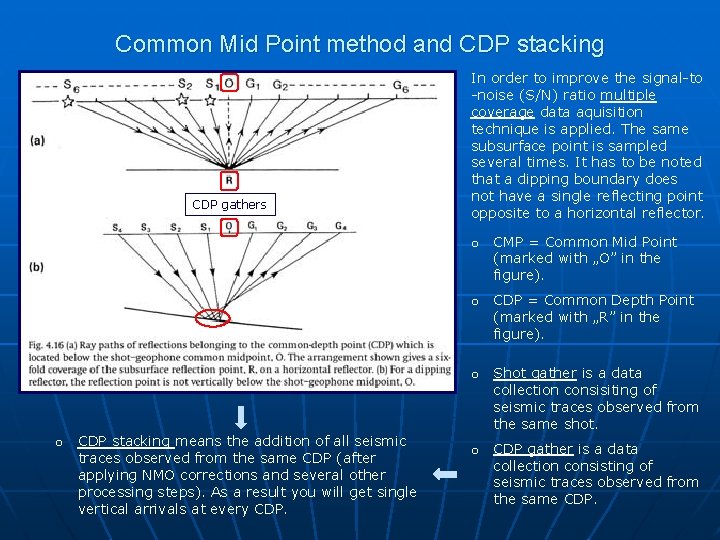
Common Mid Point method and CDP stacking CDP gathers In order to improve the signal-to -noise (S/N) ratio multiple coverage data aquisition technique is applied. The same subsurface point is sampled several times. It has to be noted that a dipping boundary does not have a single reflecting point opposite to a horizontal reflector. o CMP = Common Mid Point (marked with „O” in the figure). o CDP = Common Depth Point (marked with „R” in the figure). o Shot gather is a data collection consisiting of seismic traces observed from the same shot. o CDP stacking means the addition of all seismic traces observed from the same CDP (after applying NMO corrections and several other processing steps). As a result you will get single vertical arrivals at every CDP. o CDP gather is a data collection consisting of seismic traces observed from the same CDP.
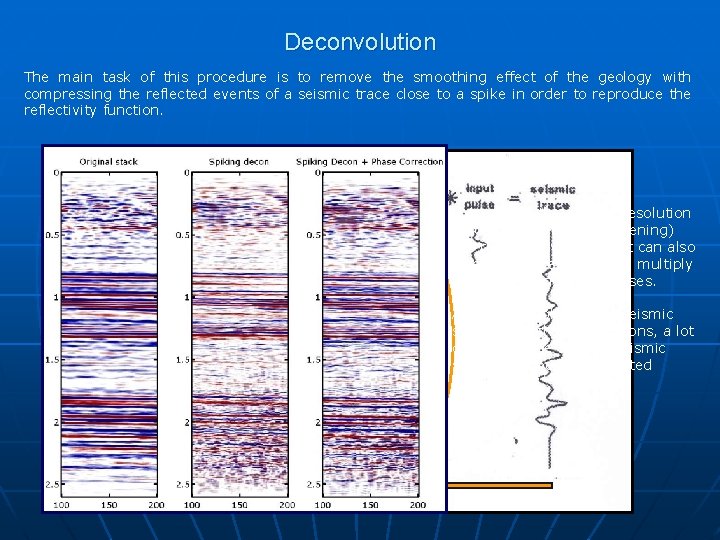
Deconvolution The main task of this procedure is to remove the smoothing effect of the geology with compressing the reflected events of a seismic trace close to a spike in order to reproduce the reflectivity function. It improves the vertical resolution by compressing (or shortening) the reflection wavelets. It can also attenuate reverberations, multiply reflections, and other noises. After deconvolution the seismic section has sharp reflections, a lot of noise in the original seismic section has been attenuated considerably.
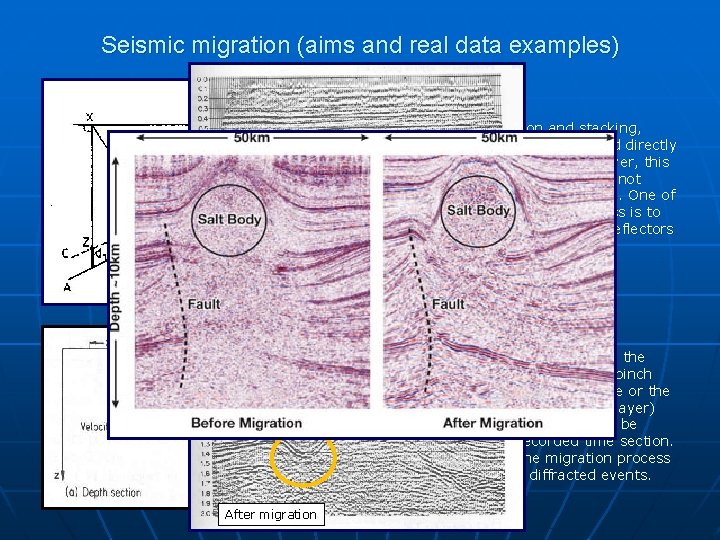
Seismic migration (aims and real data examples) After NMO correction and stacking, each reflection event is mapped directly beneath the CMP points. However, this vertical ray path assumption is not correct if the reflector has a dip. One of the aim of the migration process is to move the dipping (or curved) reflectors to their real position. Before migration If there are ‘diffractor points’ in the homogeneous half-space (e. g. pinch out of a layer, a narrow syncline or the termination points of a faulted layer) then ‘diffracted hyperbolas’ will be generated in the recorded time section. The other aim of the migration process is to ‘remove’ that diffracted events. After migration
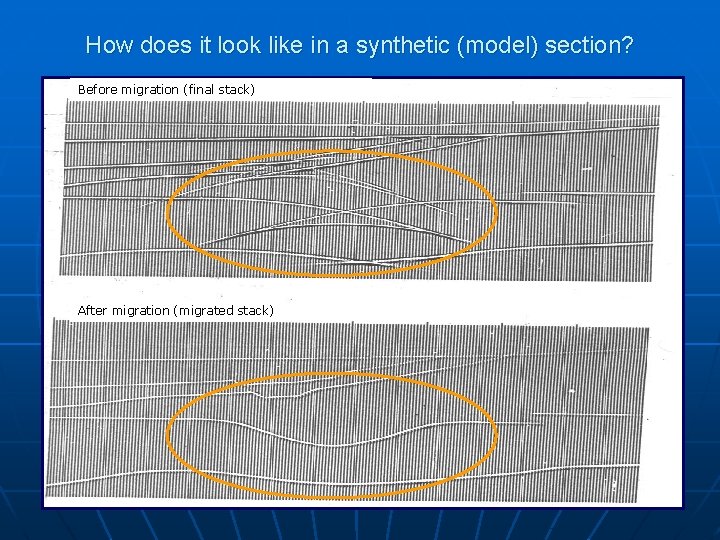
How does it look like in a synthetic (model) section? Before migration (final stack) After migration (migrated stack)
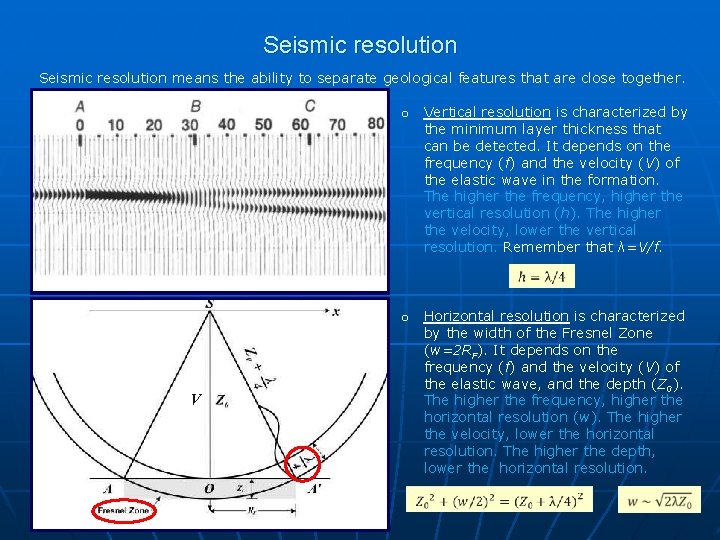
Seismic resolution means the ability to separate geological features that are close together. o Vertical resolution is characterized by the minimum layer thickness that can be detected. It depends on the frequency (f) and the velocity (V) of the elastic wave in the formation. The higher the frequency, higher the vertical resolution (h). The higher the velocity, lower the vertical resolution. Remember that λ=V/f. V o Horizontal resolution is characterized by the width of the Fresnel Zone (w=2 RF). It depends on the frequency (f) and the velocity (V) of the elastic wave, and the depth (Z 0). The higher the frequency, higher the horizontal resolution (w). The higher the velocity, lower the horizontal resolution. The higher the depth, lower the horizontal resolution.
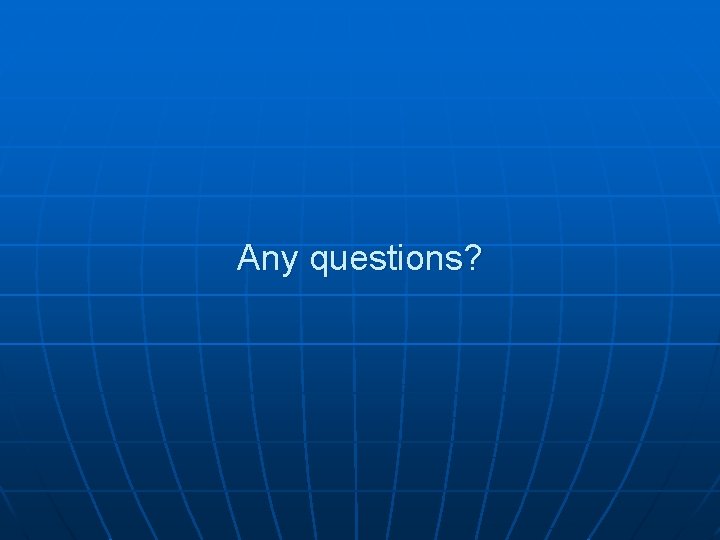
Any questions?
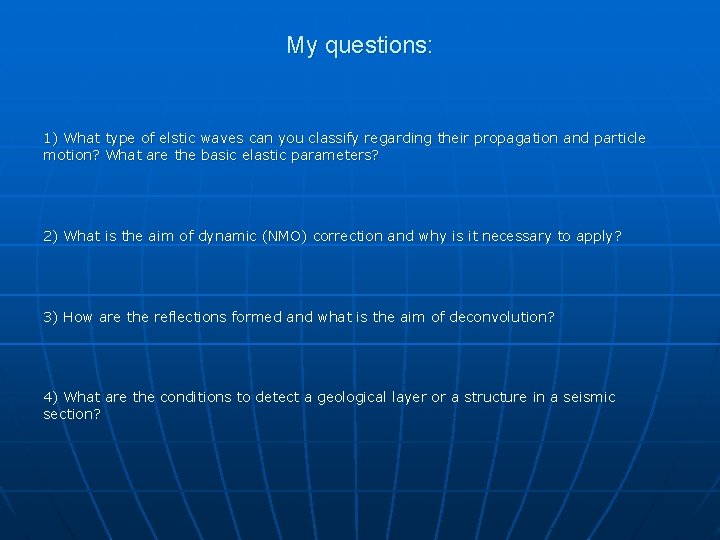
My questions: 1) What type of elstic waves can you classify regarding their propagation and particle motion? What are the basic elastic parameters? 2) What is the aim of dynamic (NMO) correction and why is it necessary to apply? 3) How are the reflections formed and what is the aim of deconvolution? 4) What are the conditions to detect a geological layer or a structure in a seismic section?
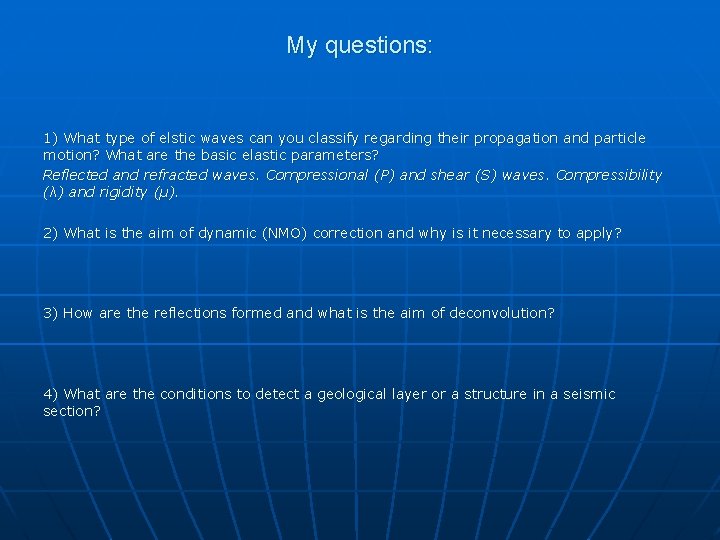
My questions: 1) What type of elstic waves can you classify regarding their propagation and particle motion? What are the basic elastic parameters? Reflected and refracted waves. Compressional (P) and shear (S) waves. Compressibility (λ) and rigidity (µ). 2) What is the aim of dynamic (NMO) correction and why is it necessary to apply? 3) How are the reflections formed and what is the aim of deconvolution? 4) What are the conditions to detect a geological layer or a structure in a seismic section?
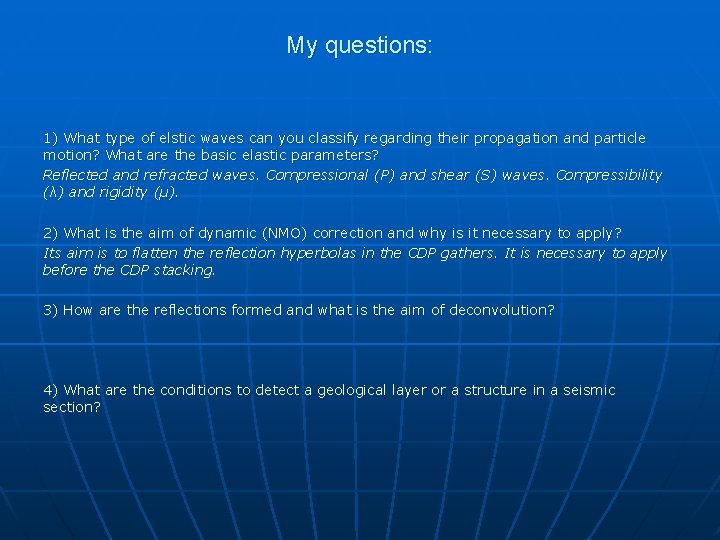
My questions: 1) What type of elstic waves can you classify regarding their propagation and particle motion? What are the basic elastic parameters? Reflected and refracted waves. Compressional (P) and shear (S) waves. Compressibility (λ) and rigidity (µ). 2) What is the aim of dynamic (NMO) correction and why is it necessary to apply? Its aim is to flatten the reflection hyperbolas in the CDP gathers. It is necessary to apply before the CDP stacking. 3) How are the reflections formed and what is the aim of deconvolution? 4) What are the conditions to detect a geological layer or a structure in a seismic section?
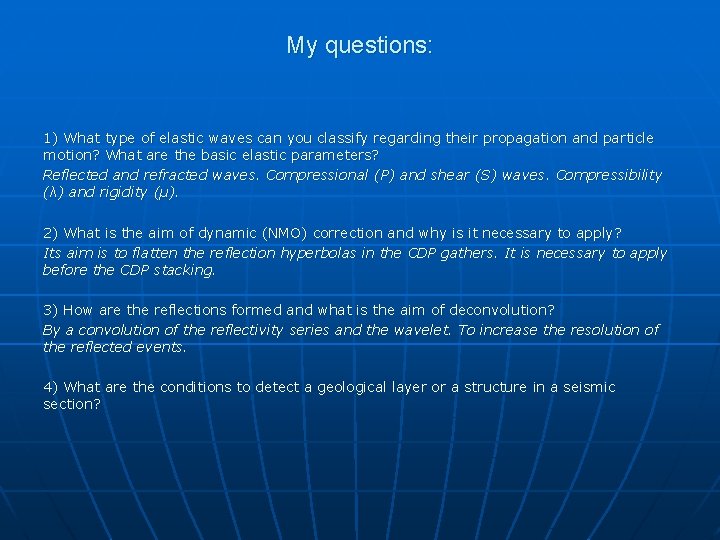
My questions: 1) What type of elastic waves can you classify regarding their propagation and particle motion? What are the basic elastic parameters? Reflected and refracted waves. Compressional (P) and shear (S) waves. Compressibility (λ) and rigidity (µ). 2) What is the aim of dynamic (NMO) correction and why is it necessary to apply? Its aim is to flatten the reflection hyperbolas in the CDP gathers. It is necessary to apply before the CDP stacking. 3) How are the reflections formed and what is the aim of deconvolution? By a convolution of the reflectivity series and the wavelet. To increase the resolution of the reflected events. 4) What are the conditions to detect a geological layer or a structure in a seismic section?
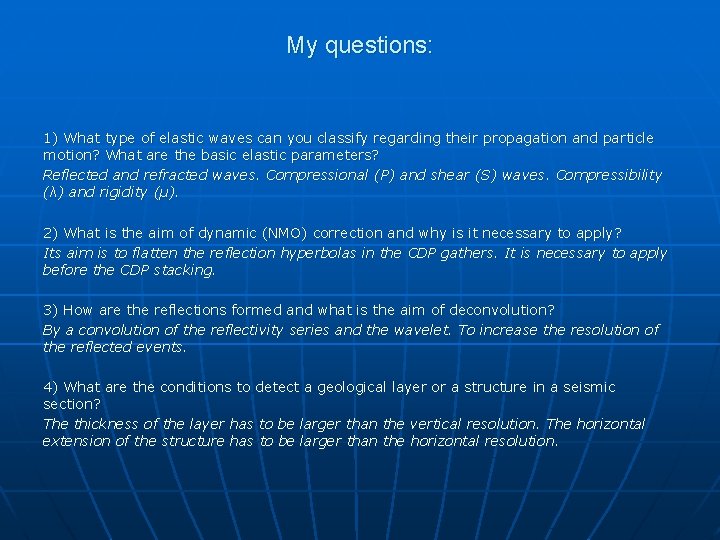
My questions: 1) What type of elastic waves can you classify regarding their propagation and particle motion? What are the basic elastic parameters? Reflected and refracted waves. Compressional (P) and shear (S) waves. Compressibility (λ) and rigidity (µ). 2) What is the aim of dynamic (NMO) correction and why is it necessary to apply? Its aim is to flatten the reflection hyperbolas in the CDP gathers. It is necessary to apply before the CDP stacking. 3) How are the reflections formed and what is the aim of deconvolution? By a convolution of the reflectivity series and the wavelet. To increase the resolution of the reflected events. 4) What are the conditions to detect a geological layer or a structure in a seismic section? The thickness of the layer has to be larger than the vertical resolution. The horizontal extension of the structure has to be larger than the horizontal resolution.
Latitude correction gravity formula
Gravity method of geophysical exploration
Ngdc
Reservoir geophysical corporation
Basilan and romblon tsunami
Geophysical fluid dynamics
Using bird dogs when prospecting for clients refers to
What are the objectives of strategic prospecting?
Prospecting and qualifying
Tsm prospecting
Prospecting
Social prospecting pro
Strategic prospecting
Prospecting tour
Prospecting in aviation
Sales prospecting playbook
Ikbs
Kepong prospecting ltd v schmidt
Wax pattern in fpd
Aircraft cable seismic manufacturer
Which seismic wave refracts and cannot penetrate the core
Robotics webquest
Module 10 expansion exploration and encounters
Spain god gold glory
Presidential elections exploration and announcement
Early european exploration and colonization resulted in
Exploration and evaluation assets shall be classified as